DOI:
10.1039/C1RA00815C
(Paper)
RSC Adv., 2012,
2, 930-940
ZnO/Ag nanohybrid: synthesis, characterization, synergistic antibacterial activity and its mechanism†
Received
29th September 2011
, Accepted 4th October 2011
First published on 28th November 2011
Abstract
A highly homogeneous ZnO/Ag nanohybrid has been synthesized by a novel route, employing chitosan as mediator by purely electrostatic interaction. By employing various techniques such as powder XRD, UV-visible, IR spectroscopy and electron (SEM, TEM) microscopy, the formation of the nanohybrid has been established. The synergistic antibacterial effect of ZnO/Ag nanohybrid on Gram-positive and Gram-negative bacteria is found to be more effective, compared to the individual components (ZnO and Ag). Cytotoxicity experiments are carried out and the results are correlated to the solubility of the nanohybrid. A possible mechanism has been proposed for the antibacterial activity of ZnO/Ag nanohybrid, based on TEM studies on bacteria, carried out by employing the microtome technique and by EPR measurements on the hybrid.
Introduction
Although tremendous achievements have been made in the field of personal hygiene and medicine, the access to safe drinking water in many parts of the world still remains a dream or has become an expensive commodity.1 According to WHO-2004 report, at least one-sixth of the world’s population lack access to safe drinking water.2 The consequences are alarming as millions of people, mostly children under the age of five, die annually. Water borne bacteria, fungi and viruses are responsible for the transmission of diseases. The age old method of treating water with chemicals, such as chlorine for the purification of drinking water, results in secondary carcinogenic disinfection by-products (DBPs).3–5 This has lead to pondering about the use of alternatives, wherein the DBPs formation is negligible, but act as an effective antibacterial agent. Perhaps nanotechnology offers us a handle on this type of environmental problem. Nanomaterials have unique properties, because of their large surface to volume ratio and are found to be excellent adsorbents, catalysts and sensors.6–8 Nanomaterials such as Ag nanoparticles (Ag NPs),9–13TiO2 nanoparticles (TiO2 NPs),14–16ZnO nanoparticles (ZnO NPs),17–19SiO2 nanoparticles (SiO2 NPs),20,21 and polysaccharide chitosan nanoparticles22 have shown good antimicrobial activity. Among these ZnO NPs exhibit as an effective antibacterial agent against Gram-positive, such as Staphylococcus aureus (S. aureus) compared to Gram-negative.23,24 On the other hand Ag NPs are known to have more bactericidal effect on Gram-negative bacteria like Escherichia coli (E. coli) compared to Gram-positive.25,26 Our approach here is to combine these two, and to have a hybrid system that can function synergistically on both types of bacteria in the purification of water. Very little work has been reported in this direction, for example, W. Lu et al.,27 have reported the preparation of ZnO/Ag hybrid by a hydrothermal method and have studied the antibacterial activity against E. coli and S. aureus. Koga et al.,28 have synthesized ZnO/Ag by ion-exchange between Ag and Zn species and simultaneous ZnO-mediated photo-reduction and have tested the antibacterial activity for repeated use against bacterium E. coli. Li et al.,29 have fabricated blend films of chitosan/Ag/ZnO (CS/Ag/ZnO) by sol-cast transformation and have reported the antibacterial studies. Though antibacterial mechanisms of the individual components such as Ag nano,30,31ZnO17,32,33 are proposed earlier but no experimental findings to show the mechanism of the antibacterial activity of the hybrid are reported. Others have synthesized ZnO/Ag nanostructures by various other routes and have studied different properties. For example Xiang et al.,34 have prepared ZnO/Ag nanostructures by a photochemical method and studied the gas sensing properties. Zheng et al.,35 have synthesized ZnO/Ag by a hydrothermal method for the study of photocatalytic activity of materials. Song et al.,36 have synthesized ZnO/Ag composite microsphere for Raman studies. Lee et al.,37 have studied the optical properties of ZnO/Ag composite nanocrystals, prepared by mixing Ag NPs with sodium hydroxide containing Zn(NO3)2. Some of these procedures reported involve lengthy steps in order to have purely biocompatible material. Here, we report a very simple and faster method to fabricate ZnO/Ag nanohybrid with chitosan as mediator. The approach is the sequential deposition of positively charged chitosan polyelectrolyte over ZnO particles and then attaching negatively charged citrate capped Ag+ ions over positively charged chitosan by electrostatic interactions. Surface silver ions are then reduced by sodium borohydride resulting in Ag nanoparticles over the ZnO surface, thus finally forming the ZnO/Ag nanohybrid. Based on the minimum inhibition concentration (MIC) and cytotoxicity results, a particular composition of the nanohybrid was chosen for a detailed study. The prepared samples were characterized by X-ray diffraction (XRD), scanning electron microscopy (SEM), transmission electron microscopy (TEM) and X-ray photoelectron spectroscopy (XPS). The antibacterial activity of ZnO NPs, Ag NPs and ZnO/Ag nanohybrid are tested individually against E. coli and S. aureus in saline water. ZnO/Ag nanohybrid synergistically kills both the bacteria in solution. A qualitative antibacterial test in the form of inhibition zones was also evaluated by disc diffusion assay. Cytotoxicity experiments are also carried out and the results are correlated to the solubility of the nanohybrid. A possible mechanism of the antibacterial activity of the hybrid was proposed based on the TEM studies of the interaction between bacteria and ZnO/Agx nanohybrid, carried out by employing microtome technique and reactive hydroxyl radical results obtained by EPR studies on the hybrid.
Experimental section
Materials
Zinc acetate di-hydrate, silver nitrate, sodium borohydride, (S.D Fine-Chem Pvt.Ltd., India), diethylene glycol (Merck, India) chitosan (Mw∼2
000
000; Sigma Aldrich, Germany), sodium citrate di-hydrate (Rankem, India) were used as such without any further purification. For the purpose of antimicrobial assays of the ZnO/Ag nanohybrid, nutrient broth (Hi-Media Laboratories Ltd, India) was used. Standard reference strains, Escherichia coliMTCC 1302, and Staphylococcus aureus ATCC 25923 (supplied by M.S.Ramaiah Hospital, Bangalore) were used for the bacterial studies.
Synthesis of ZnO submicron particles
ZnO submicron particles were prepared by hydrolysis of zinc salts in polyol on refluxing at 180 °C, similar to the procedure described by Zhang et al.38 Typically, 0.9855 gm of zinc acetate di-hydrate was added to 45 ml of diethylene glycol (DEG) with vigorous stirring for 30 min at room temperature. The mixture was then refluxed in an oil bath at 180 °C for 1 h on stirring. A milky white precipitate appeared at the end of the reaction indicating the formation of ZnO. The as-obtained milky precipitate was centrifuged, separated and washed with ethanol several times by a repeated sonication-centrifugation process. Finally, the precipitate was dried in a vacuum-air oven at 60 °C for 6 h.
Synthesis of ZnO/Ag nanohybrid
The pristine ZnO powder was modified as follows to obtain ZnO/Ag nanohybrid; Ag NPs were attached to ZnO with chitosan as mediator. Typically, 0.0025 gm (30.7 μMole) of ZnO was added to 1 ml of 0.1% chitosan aqueous solution (prepared by dissolving weighed amount of chitosan in Millipore water by drop wise addition of 0.1% acetic acid) and stirred for an hour, centrifuged and washed with Millipore water to remove unbound chitosan. Further, a solution containing 1 ml of 1.0 mM AgNO3 and 1.0 mM citrate was added and stirred for about an hour, centrifuged and washed with Millipore water to remove unbound AgNO3. Both these steps were repeated several times to ensure maximum binding of chitosan onto ZnO and the Ag ions onto chitosan. Subsequently, 10 μl of 40 mM NaBH4 in ice-cool water was added at a time to reduce Ag+ ion to metallic Ag° on stirring and it was continued for a few more minutes, till the appearance of yellow colour on ZnO, forming ZnO/Ag1 nanohybrid. The nanohybrid was then sonicated, centrifuged and washed several times with ethanol. Finally, the precipitate was dried in a vacuum-air oven at 45 °C for 24 h. This procedure was repeated with 4.0 mM, 7.0 mM and 10.0 mM of AgNO3 and sodium citrate, keeping the amount of ZnO (0.0025 gm) the same to obtain different compositions of ZnO/Ag nanohybrid. These samples are designated as, ZnO/Ag4, ZnO/Ag7 and ZnO/Ag10, respectively.
Antibacterial study
Minimum inhibition concentration (MIC)
The MIC is the lowest concentration at which a material exhibits antimicrobial activity and this was done by serial dilution. Sterile test tubes were taken separately with 9.9 ml of saline water, inoculated with corresponding microorganisms (fresh culture, mid-log phase, OD600 ∼ 0.045–0.050) and diluted up to 108 CFU/ml. To this, different concentrations (25–200 μg ml−1) of ZnO NPs, Ag NPs and ZnO/Ag nanohybrid were added to individual test tubes. After 4 h of incubation at 37 °C, 0.1 ml each of this solution was taken and plated in sterile nutrient agar plates. Then the plates were incubated at 37 °C overnight and colonies were counted to determine the MIC.
Zone of inhibition (ZOI)
The bacterial lawn was prepared on sterile Muller Hinton agar plates by using a sterile cotton swab. Four 10 mm diameter sterile filter papers were taken and coated with 100 μl of ZnO/Ag nanohybrid, ZnO solution, Ag NPs and water, respectively, and were placed at the centre of the lawn carefully without touching the other parts and incubated at 37 °C for 24 h. The zone around the film was then photographed on subsequent days.
In vitro cytotoxicity experiment
In vitro cytotoxicity assays for the NPs were carried out by exposing human embryonic kidney cell line (HEK 293) in microtiter plates to various dilutions of NPs in aqueous saline solution. Concentrations ranging from 10 μg ml−1 to 150 μg ml−1 of ZnO, ZnO/Agx nanohybrid were taken in saline solution and thoroughly sonicated. These are then pipetted into 96-well microtiter plates. Further 0.1 ml freshly trypsinized HEK 293 cells cultured as monolayer in Dulbecco's Modified Eagle Medium (DMEM) (containing 10% FCS and antibiotic solution) to active growth phase (18
000 cell per well) were added and incubated for 20 h at 35 °C in 5% CO2 atmosphere. Wells containing an equal number of cells, without NPs, were left as controls. After incubation, the detached cells, medium, and NPs were removed by vigorous shaking. The remaining live cells were fixed in 2% solution of formalin in 0.067 M phosphate-buffered saline (PBS) (pH 7.2) for 1 min. The plates were stained with 0.4% trypan blue dye-PBS solution for 20 min. Excess stain was removed through rinsing with water, and the plates were air dried. For quantification, the stain was eluted from the wells with four successive 50 μl samples of 50% ethanol and diluted in 0.9 ml of PBS. Absorbance of the pooled washings was recorded at 595 nm in a spectrophotometer. Percentage of cells surviving the treatment was computed for various doses of NPs by considering the optical density (OD595) for control as a hundred percent.
Characterization
Powder XRD patterns of ZnO and ZnO/Ag nanohybrid are recorded by a Philips XRD ‘X’ PERT PRO diffractometer using Cu-Kα radiation (λ = 1.5438 Å) as X-ray sources with a scan rate of 4° min−1.
The amount of silver in each of the ZnO/Agx nano hybrid samples was determined by ICP emission spectroscopy using a Perkin-Elmer optima 2100 Inductively Coupled Plasma-Optical Emission (ICP-OES) spectrometer at λ = 328 nm by initially dissolving a known amount of ZnO/Agx samples in 100 μl dilute nitric acid (∼3.5 M) and there after suitable dilution was made with Millipore water.
To find the solubility of ZnO NPs, chitosan modified ZnO NPs and ZnO/Ag4 in water, an equal amount of these samples were taken separately and vortexed overnight in a known volume of Millipore water. They were then centrifuged and filtered through filter paper of pore diameter ∼100 nm (Amicon ultrafiltration disc) and an estimation of the Zn content was made by an ICP-OES spectrometer at λ = 2138 nm.
UV-visible
absorption spectra of the samples were recorded in a quartz cuvette, prepared by thoroughly sonicating in water for a few hours, using Perkin-Elmer Lambda 35 UV-Visible spectrophotometer.
IR
absorption spectra were recorded in a FT-IR SPECTRUM 1000 PERKIN ELMER spectrometer on thoroughly dried samples using KBr (dilutant) pellets. The ratio of sample to dilutant was kept constant throughout in order to compare the spectra.
The morphological studies of as synthesized ZnO NPs and ZnO/Ag nanohybrid were carried out using a FE-SEMSIRION field emission scanning electron microscope (SEM). A drop of well dispersed particles in water was cast onto a piece of silicon wafer and air dried. A thin gold coating was applied to avoid charging during scanning and a detailed microscopic study was carried out.
The size and morphology of ZnO/Agx nanohybrid were analyzed by TEM in TECNAI F 30 transmission electron microscope. Proper care was taken to suitably mount the samples onto the Cu grid.
The interaction between bacteria and ZnO/Agx nanohybrid were also observed in TEM by preparing thin slices of bacteria embedded in a polymer by microtome. After contact with ZnO/Ag nanohybrid for 4 h, the corresponding bacterial suspension was washed twice and fixed with 2% glutaraldehyde for 1.5 h. Samples were then post fixed with 1% lead acetate and dehydrated in acetone series (35, 50, 70, 80, 95 and 100%) for 3 min each. After dehydration by 100% acetone, samples were then embedded in (SPURR resin) of suitable size. Ultra thin sections were cut using an ultra microtome (RMC MT-X) and stained with uranyl acetate and lead citrate. The thin sections were taken on to the Cu grid and micrographs were obtained using the JEOL (100 CX II) TEM at 100 KV.
The powdered ZnO/Ag nanohybrid samples were pressed into 0.5 mm thick and 8.0 mm diameter pellets and placed in an ultra high vacuum chamber at 10−9 torr for chemical analysis. X-ray photoelectron spectra (XPS) were recorded on a Thermoscientific Multilab 2000 equipment employing Al Kα X-rays (1486.6 eV).
Hydroxyl radicals were detected by an EPR-spin trapping technique using spin trapper 5,5-dimethyl-1-pyrroline-N-oxide (DMPO, 0.02 M) (Sigma Aldrich). Aqueous suspensions of ZnO, ZnO/chitosan and ZnO/Agx nanohybrid were drawn separately into three quartz capillaries (one end closed) along with DMPO. The capillaries were then placed in the EPR tube and spectra were recorded on a Bruker emx X-band EPR spectrometer.
Results and discussion
ZnO/Ag nanohybrid
ZnO/Ag nanohybrid is nothing but a combination of a semiconductor material and metal particles. In the solution method of synthesis generally two steps are involved. First semiconductor particles are prepared from a sol solution and then appropriate metal salts are added and reduced by a suitable reducing agent.34,39–41 During this process many times it ends up in the formation of self-nucleated and isolated metal nanoparticles along with the metal-semiconductor hybrids. To avoid this problem a simple technique being adopted to fabricate ZnO/Ag nanohybrid, with chitosan as a mediator as depicted in Scheme 1. Also, the added advantages of using chitosan are: (i) Invariably ZnO surface have large OH−groups,42,43 thus chitosan is being a cationic polymer can easily be fabricated over ZnO surface. (ii) Chitosan has lots of cationic groups (–NH3+), which can freely attach to the negatively charged surface like citrate stabilized Ag NPs, and thus decreasing the possibility of the formation of self nucleated Ag NPs. (iii) Chitosan is biodegradable44,45 and also a biocompatible 46,47polymer.
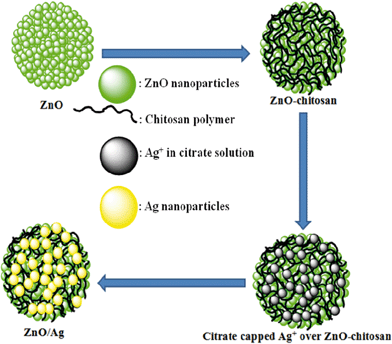 |
| Scheme 1 Schematic illustration of synthesis of ZnO/Ag nanohybrid, using chitosan as mediator. | |
Initially, cationic polyelectrolyte, chitosan was deposited over the ZnO surface, followed by negatively charged citrate capped Ag+, which is reduced to Ag by the addition of ice cooled NaBH4 solution as shown in the equation;
Ag+ + BH4− + 3H2O→Ag + H3BO3 +7/2 H2 |
We have chosen ZnO/Ag4 nanohybrid composition as a representative sample for all our discussion. Fig. 1 shows the powder XRD patterns of as-prepared ZnO/Ag1, ZnO/Ag4, ZnO/Ag7 and ZnO/Ag10 nanohybrid. The recorded diffraction peaks can be categorized into two sets. Peaks marked with ‘@’ match well with Bragg reflections of the standard wurtzite structure of ZnO (JCPDS file no. 36–1451), while the others marked ‘*’ agree well with face-centered cubic (FCC) silver (JCPDS file no. 04-0783).
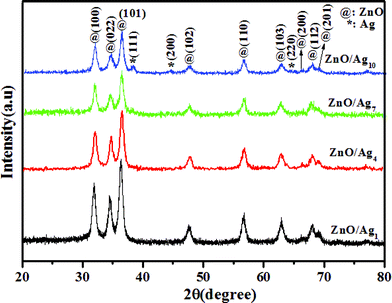 |
| Fig. 1
Powder XRD patterns of ZnO/Ag1, ZnO/Ag4, ZnO/Ag7 and ZnO/Ag10 nanohybrid. | |
For further clarity a very slow scan rate (0.1° min−1) powder XRD was run for the ZnO/Ag4 sample and the diffraction pattern obtained is shown in the supporting information (Fig. S1†).
The estimated Ag present in the ZnO/Ag nanohybrids modified with 1, 4, 7 and 10 mM AgNO3, by ICP-OES is given in Table 1. As expected, when the initial concentration of AgNO3 increased, the amount of Ag deposited on to the ZnO also increased. However, the amount of Ag deposited is considerably small, compared to the original concentration of Ag+ in solution.
Table 1 Estimated Ag by ICP-OES in ZnO/Agx nanoybrid
ZnO/Agx |
Ag (%) |
ZnO/Ag1 |
0.97 |
ZnO/Ag4 |
3.79 |
ZnO/Ag7 |
6.63 |
ZnO/Ag10 |
9.10 |
Optical properties
The optical absorption of pristine ZnO, ZnO/Ag4 nanohybrid and Ag NPs in aqueous solution recorded in the UV-visible range, are shown in Fig. 2. A sharp peak at 365 nm for pure ZnO can be attributed to the excitonic absorption of ZnO.35,36,48 The plasmon peak around 410 nm corresponds to spherical Ag NPs, while in the ZnO/Ag4 nanohybrid, these two peaks merge, resulting in a weak excitonic peak and a broad absorption in the range of 400–600 nm with a red shift (Fig. 2b). As the silver concentration increased the broadening of the peak also increases, see supporting information (Fig. S2†). The most probable reason for the red shift of surface plasmon absorption of Ag NPs in the ZnO/Ag4 nanohybrid is due to the strong interfacial coupling between neighbouring ZnO and Ag NPs. It is well known that the position of plasmon absorption of metal NPs depends on the electron density of metal,49 which has the relation
λ = [4π2c2meff ε0/Ne2]1/2 |
Where λ is the plasmon absorption wavelength, meff and N are the effective mass and density of free electrons of the metal respectively. When electron density N of metal is decreased, the wave length (λ) of the plasmon absorption increases, resulting in red shift of plasmon absorption. Thus the red shift in ZnO/Ag nanohybrids is due to the transfer of electrons from the Ag NPs to ZnO, causing a decrease in electron density of Ag NPs (see XPS results). This happens because the work function (Φs) of ZnO (5.2 eV) is more than that work function (Φm) of silver (4.26 eV) and the Fermi energy level (Efs) of ZnO is lower than that of Ag (Efm), resulting in the transfer of electrons from Ag to ZnO, until the two systems attain equilibrium and form the new Fermi energy level (Ef)37 as illustrated in Fig. 3.
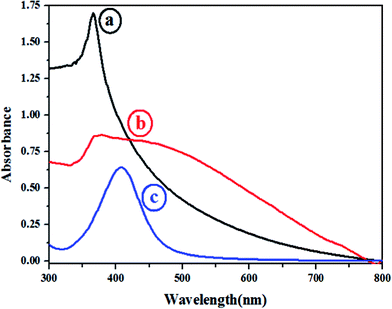 |
| Fig. 2
UV-Visible
spectra of (a) unmodified ZnO, (b) ZnO/Ag4 nanohybrid and (c) pure Ag NPs. | |
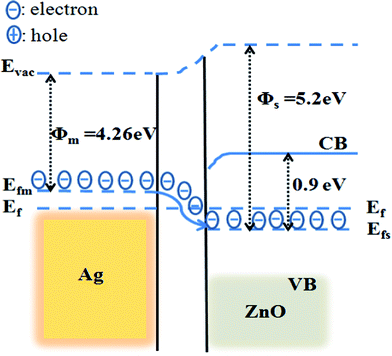 |
| Fig. 3 The energy band structure of Ag metal and ZnO showing the uniform Fermi energy level, induced by electron transfer from Ag to ZnO. | |
The FT-IR spectrum of ZnO in Fig. 4a shows a strong O–H stretching peak at 3398 cm−1 indicating the presence of a sufficient amount of hydroxyl groups at the surface of as prepared ZnO. Upon addition of cationic chitosan polymer onto ZnO, there appears a peak at 3360 cm−1 which may be assigned to the combination of N–H and O–H stretching of chitosan (Fig. 4b). Moreover in case of ZnO/chitosan the most prominent peaks at 1556 cm−1 and 1393 cm−1 corresponding to N–H bending and C–H bending of chitosan, respectively, clearly shows the ZnO surface being modified with cationic chitosan. Similarly in case of ZnO/Ag4 nanohybrid these peaks have reduced intensity (Fig. 4c), indicating the interaction between cationic chitosan and citrate capped Ag NPs. Similar observations were made by Bai et al.,50 in their studies on the absorption of Pb on to chitosan.
In order to obtain detailed information about the microstructure and morphology of as synthesized ZnO/Ag4 nanohybrid, TEM and SEM studies were carried out. Fig. 5 shows SEM and TEM images of ZnO/Ag4 along with pristine ZnO. Both SEM and TEM images reveal that the ZnO particles are spherical and polydispersed with size varying between 150–250 nm (Fig. 5a, 5c). On close observation one would see that the individual ZnO submicron particles are made up of an aggregation of a large number of ZnO NPs of size ∼13 nm and the surface of the submicron particles of ZnO/Ag4 nanohybrid are rough compared to the surface of pristine ZnO (Fig. 5a–b). A low-magnified TEM image of this sample shows a sufficient yield of ZnO/Ag4 nanohybrid consisting of metallic Ag NPs (highlighted by circles, Fig. 5d) on ZnO submicron sized particles. Self-nucleated or isolated Ag NPs are hardly observed. The high-resolution TEM (HRTEM) (Fig. 5f) of the region marked as ‘α’ (Fig. 5d) shows the lattice fringes of both ZnO and Ag. The lattice fringes may be assigned to 0.235 nm of (111) plane of FCC Ag and similarly another set of lattice fringes of width 0.256 nm is assigned to (002) plane of ZnO. These micrographic studies clearly indicate the formation of ZnO/Ag4 nanohybrid. The EDAX pattern confirms the presence of the elements Zn, O and Ag (Fig. 5e)
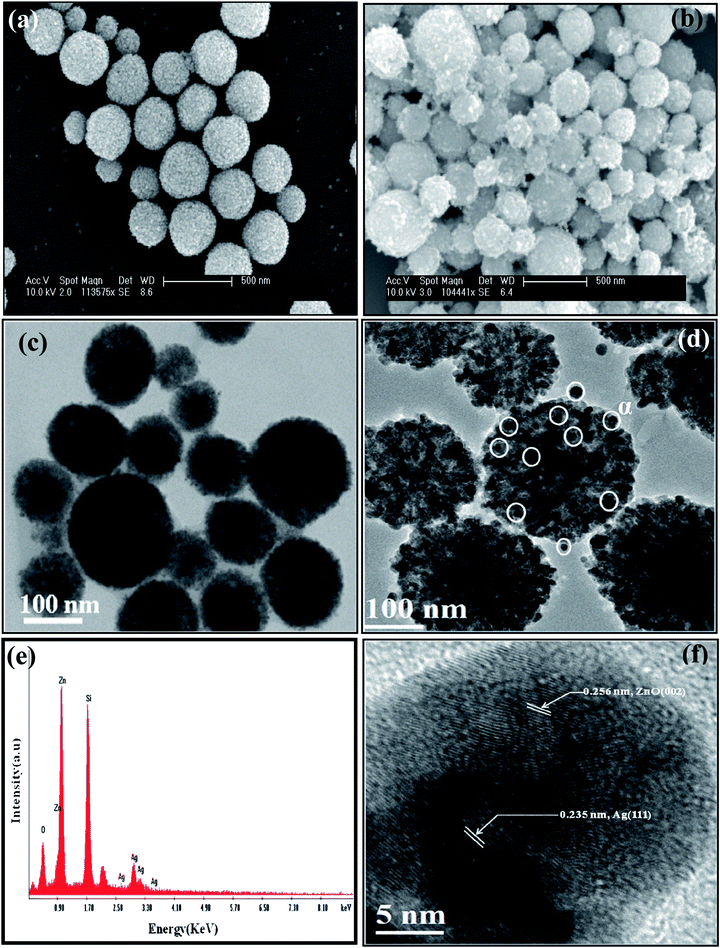 |
| Fig. 5
Electron micrographs: (a) and (b) SEM images (scale bar: 500 nm) of ZnO and ZnO/Ag4 nanohybrid, respectively, (c) and (d) respective TEM images (scale bar: 100 nm) (e) EDAX spectrum obtained from SEM for ZnO/Ag4 nanohybrid, and (f) HRTEM image showing lattice fringes of both ZnO and Ag. | |
Based on powder XRD, microscopic and optical studies, it confirms the deposition of Ag NPs onto ZnO submicron particles, forming ZnO/Ag nanohybrid. For further characterization and to know the surface structure of ZnO/Ag4 nanohybrid, XPS studies are carried out and the results are shown in Fig. 6. Fig. 6a shows the scan survey spectra of the sample. The peaks can be ascribed to Zn, O, C, N and Ag (inset: Fig. 6a, zoomed images).
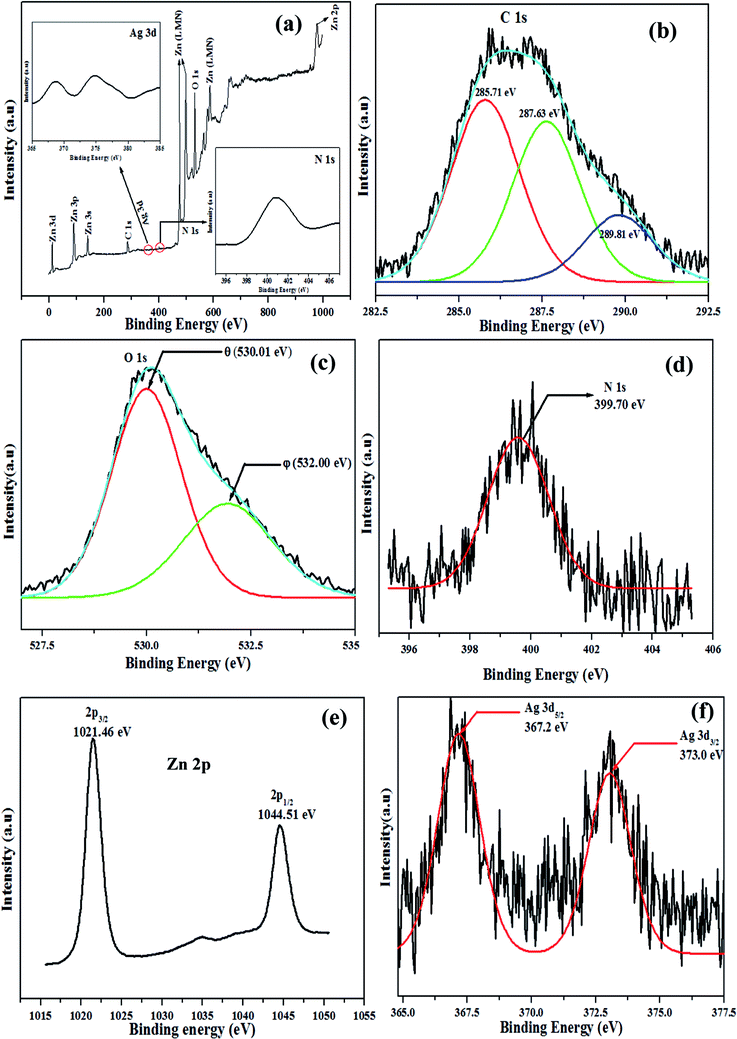 |
| Fig. 6 Typical XPS spectra of ZnO/Ag4 nanohybrid (a) the survey spectra and high resolution spectra of (b) C 1 s, (c) O 1 s, (d) N 1 s, (e) Zn 2p, (f) Ag 3d. | |
The high-resolution XPS spectra of elements C, O, N, Zn and Ag are shown in Fig. 6b–f, respectively. On deconvolution, the carbon 1s peak (Fig. 6b) was analyzed in terms of three components; 285.7 eV due to C–H and C–C moieties, 287.6 eV because of C–O–C and C–OH linkages and 289.8 eV may be assigned to both C
O and [–O–C–O–] moieties of the chitosan polymer.51Fig. 6c shows the high resolution spectrum of O1s having two kinds of oxygen species. One of the deconvoluted peaks marked ‘θ’ (530.01 eV) is closely associated with lattice oxygen of ZnO, while the other peak marked with ‘φ’ (532.00 eV) is attributed to oxygen of surface hydroxyl groups.52 The N1s peak (Fig. 6d) at 399.7 eV can be assigned to N–C
O and –NH2 binding of chitosan.53 The peak position at 1021.46 eV (Fig. 6e) of Zn 2p3/2 in ZnO/Ag4, confirms that Zn exists in the sample mainly in the form of Zn2+.54Fig. 6f shows the Ag 3d XPS spectrum of the ZnO/Ag4 nanohybrid. The binding energy (BE) of Ag 3d5/2 (367.2 eV) shifts notably to the lower value compared to Ag° (BE of Ag 3d5/2 for Ag° is about 368.2 eV).55 This suggests that the electron density of Ag in ZnO/Ag4 nanohybrid has decreased, further confirming the reason for red shift of the Ag plasmon band in the ZnO/Ag nanohybrid (Fig. 2).
Antibacterial activity
One of our interests is to study the inorganic materials for antimicrobial activity in the purification of water for safe drinking. Keeping this in mind the antimicrobial activity of ZnO/Ag nanohybrid was tested in saline water containing microbes S. aureus and E. coli individually as model systems which is close to microbes contaminated drinking water.18 On the other hand when the nutrient broth (NB) media was used, the probability of adherence of particles to the protein present in NB will be more,18 which causes difficulty while conducting microscopic and EPR studies. The corresponding minimum inhibition concentrations (MIC) are tabulated in Table 2. The results of MIC for ZnO/Ag nanohybrid in all compositions studied is much less than that of pure ZnO (as prepared) and Ag NPs (as prepared), indicating the synergistic effect of the nanohybrid. Further for qualitative assessment of antibacterial effect, it is evaluated by disc diffusion assay and the zone of inhibition (ZOI) and is shown in Fig. 7a–b. From the photographs one can clearly observe ZOI formed by ZnO/Ag nanohybrid is more compared to individual components, suggesting that ZnO/Ag nanohybrid is an efficient antibacterial material for both Gram-negative and Gram-positive bacteria.
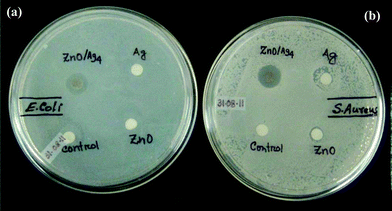 |
| Fig. 7 Photographs showing disc diffusion assay. Clear zone formed against the growth of (a) E. coli and (b) S. aureus. | |
Table 2 Minimum inhibition concentration (MIC) for ZnO, Ag and ZnO/Ag nanohybrid
ZnO/Agx |
MIC (μg ml−1) |
S. aureus
|
E. coli
|
Ag NPs are synthesized by NaBH4 and capped with sodium citrate: (AgNO3) : 1 mM and NaBH4 : 40 mM and citrate : 1 mM).44
|
ZnO (as prepared) |
100 |
127 |
ZnO/Ag1 |
23 |
35 |
ZnO/Ag4 |
18 |
25 |
ZnO/Ag7 |
10 |
20 |
ZnO/Ag10 |
07 |
12 |
Ag (as prepared)a |
46 |
37 |
In vitro cytotoxicity
The cyctotoxicity of materials synthesized is shown in Fig. 8. The degree of toxicity of ZnO, ZnO/Ag7 and ZnO/Ag10 are found to be almost the same, whereas ZnO/Ag1 and ZnO/Ag4 shows less toxicity. The IC50 (half maximal inhibitory concentration) of ZnO and ZnO/Ag4 are 6.23 μg ml−1 and 14.81 μg ml−1, respectively, which indicates that the ZnO toxicity is more than twice that of ZnO/Ag4. This can be explained by the solubility of ZnO and modified ZnO. Brunner et al.,56 has suggested that the solubility of oxide nanomaterials strongly influences their toxicity. The higher the solubility of a compound, the higher will be the in vitro toxicity of mammalian cells. The solubility of ZnO (chitosan modified) and ZnO/Ag4 are almost the same, but much less than ZnO (unmodified) as seen in the solubility chart (inset, Fig. 8) obtained from ICP-OES study. The solubility of the modified zinc oxide synthesized by us is much less than the reported values for ZnO NPs.57 This infers the fact that ZnO (chitosan modified) and ZnO/Ag4 may be less toxic to mammalian cells than nano sized ZnO studied.
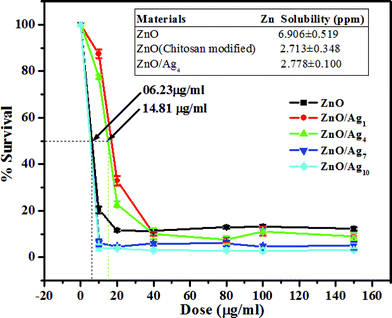 |
| Fig. 8 Plot of percentage survival of HEK-293 cellsvs. different doses of nanomaterials. Inset showing the solubility table of ZnO, modified ZnO and ZnO/Ag4. | |
A plausible mechanism for antimicrobial activity
The germicidal effect of Ag and ZnO NPs on microorganisms is very well known but the bactericidal mechanism of both Ag and ZnO is still not fully understood.30–33 The mechanisms of antibacterial property of nanostructures till now proposed can be classified broadly into two,58,59 (i) oxidative stress generated by particles in solutions17,32,33 and (ii) physical attack of nanoparticles on the bacteria. Other forms of mechanism like (a) deactivation of enzymatic activity of bacterial respiratory chain,60,61 (b) bacterial structural changes by the nanoparticles,30,32,33 (c) interaction with bacterial DNA31,62etc., reported in literature are nothing but the consequences of (ii). However, there are no reports on the mechanism of a hybrid system, like ZnO/Ag. Here an attempt is made to give a plausible mechanism based on our own TEM and EPR studies. We have examined the interaction of ZnO/Ag4 nanohybrid with both types of bacterial strains by TEM. Fig. 9a–b shows the TEM micrographs of healthy E. coli and S. aureus, respectively. The attachment and internalization of ZnO/Ag4 nanohybrid on to the cell walls of E. coli and S. aureus are seen in respective micrographs (Fig. 9c and 9d). Interestingly, in the case of E. coli, there is an extensive attachment of Ag NPs around the whole cell wall (Fig. 9c), which is further confirmed by HRTEM (Fig. 9e) taken on one such particle (shown by an arrow, Fig. 9c), and the distance of 0.235 nm measured between two adjacent lattice fringes, corresponds to Ag (111). Also one can observe in a few cases Ag NPs have penetrated into the cell wall of the bacteria as shown by the arrows in Fig. 9c. Based on the arguments put forth in optical studies and from XPS analysis, it is seen that Ag NPs on ZnO surface in ZnO/Ag hybrid are slightly electro positive and at biological pH = 7.4, the overall surface of the bacteria is negatively charged due to the dissociation of an excess number of carboxylic acid and other groups present in the membrane.63,30 These two oppositely charged species attract electro-statically each other upon interaction which supports the fact that Ag NPs of the hybrid attaches to the cell wall surface of E. coli preferentially than the ZnO particles as seen in the TEM pictures (Fig. 9c). Further, these particles gradually interact with the cell membrane leading to the enhancement of permeability of the cell membrane; as a result some of the Ag NPs could enter into the cell and eventually causing cell death, similar to the observation made in the case of individual Ag NPs.31
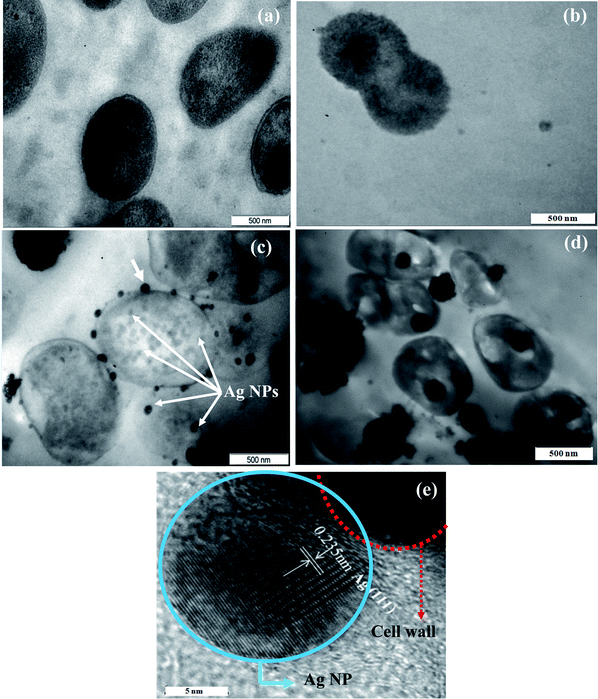 |
| Fig. 9
TEM images: (a) and (b) of healthy E. coli and S. aureus, respectively. Thin-section TEM images demonstrating the effect of ZnO/Ag4 nanohybrid on (c) E. coli and (d) S. aureus, respectively. (e) HRTEM image taken on one Ag nanoparticle attached to E. colicell wall (shown by an arrow Fig. 9c) | |
On the other hand, Ag NPs of the hybrid do not directly attach to S. aureus but the hybrid ZnO/Ag particles as a whole are found inside the cell (Fig. 9d) on penetration, similar to pure ZnO reported earlier.17 This is understandable as S. aureuscell surface is less negatively charged compared to E. coli,64,65 and hence less susceptible to direct attack by Ag NPs present in ZnO/Ag nanohybrid.
Further, EPR measurements were carried out in order to find any release of free radicals from the compounds prepared. A quartet peak was observed between 3300–3360 Gauss (Fig. 10) for ZnO and ZnO/chitosan composite, which corresponds to hydroxyl free radicals (OH˙), similar to the spectra observed by Gadenken et al.33 These hydroxyl radicals induce oxidative stress in bacteria causing cell death. It is known that oxidative stress is more toxic to S. aureus than E. coli.66
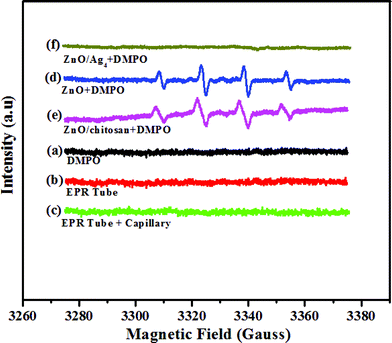 |
| Fig. 10
EPR spectra of (a) DMPO in saline, (b) EPR tube, (c) EPR tube + capillary, (d) ZnO + DMPO and (e) ZnO/chitosan + DMPO, (f) ZnO/Ag4 nanohybrid + DMPO. | |
Hence, the MIC of pure ZnO, in the case of S. aureus is less compared to E. coli. However, no OH˙ signals were observed for any of the prepared ZnO/Ag nanohybrid at their MIC concentrations. This indicates that the presence of silver at a concentration level studied quenches the formation of OH˙ radicals. Thus one may conclude that the antibacterial activity of the nanohybrid on Gram-positive bacteria like S. aureus is mainly due to a physical attack on the cell wall of the bacteria as shown in the TEM (Fig. 9) and not by the production of free radicals such as OH˙, as in the case of individual ZnO NPs.17,32,33
Conclusion
In conclusion, the method adopted for the synthesis of ZnO/Ag nanohybrid using chitosan as mediator is very simple. The Ag NPs in ZnO/Ag nanohybrid are stable and well distributed. Due to the strong interaction between metallic Ag and semiconductor ZnO, the ZnO/Ag nanohybrid showed enhanced and synergistic antibacterial activities for both Gram-negative and Gram-positive bacteria. Cytotoxicity experiments on the modified ZnO NPs is found to be less than the unmodified ZnO, mainly because of the reduced solubility of the modified ZnO NPs. From TEM and ESR studies the possible mechanism for the killing of bacteria is found to be mainly due to the physical interaction of particles with the bacteria.
Acknowledgements
The authors thank the Nano Centre (IISc) for electron microscope facilities and XPS central facility. H.N.V. thanks the IISc for internal funding.
References
- M. A. Shannon, P. W. Bohn, M. Elimelech, J. G. Georgiadis, B. J. Marinas and M. A. Mayes, Nature, 2008, 452, 301–310 CrossRef CAS.
- M. A. Montgomery and M. Elimelech, Environ. Sci. Technol., 2007, 41, 17–24 CrossRef.
- S. W. Krasner, H. S. Weinberg, S. D. Richardson and S. J. Pastor, Environ. Sci. Technol., 2006, 40, 7175–7185 CrossRef CAS.
- S. D. Richardson, A. D. Thruston and T. W. Collette, Environ. Sci. Technol., 1994, 28, 592–599 CAS.
- Q. Li, S. Mahendra, D. Y. Lyon, L. Brunet, M. V. Liga, D. Li and P. J. Alvarez, Water Res., 2008, 42, 4591–4602 CrossRef CAS.
- Q. Zhang, P. T. Chou, B. Russo, A. S. Jenekhe and G. Cao, Angew. Chem., Int. Ed., 2008, 47, 2402–2406 CrossRef CAS.
- A. P. Berseth, G. A. Harter, R. Zidan, A. Blomqvist, M. C. Araujo, H. R. Scheicher, R. Ahuja and P. Jena, Nano Lett., 2009, 9(4), 1501–1505 CrossRef CAS.
- J. Zhang, S. Song, L. Wang, D. Pan and C. Fan, Nat. Protoc., 2007, 2(11), 2888–2895 Search PubMed.
- N. Musee, M. Thwala and N. Nota, J. Environ. Monit., 2011, 13, 1164–1183 RSC.
- S. K. Gogoi, P. Gopinath, A. Paul, A. Ramesh, S. S. Ghosh and A. Chattopadhyay, Langmuir, 2006, 22, 9322–9328 CrossRef CAS.
- K. Hyeyoung and J. Jang, Langmuir, 2008, 24, 2051–2056 CrossRef CAS.
- M. D. Eby, M. N. Schaeublin, K. E. Farrington, M. S. Hussain and G. R. Johnson, ACS Nano, 2003, 3, 984–994 Search PubMed.
- P. S. Vijayakumar and B. L. V. Prasad, Langmuir, 2009, 25, 11741–11747 CrossRef CAS.
- Q. Wang, H. Yu, L. Zhong, J. Liu, J. Sun and J. Shen, Chem. Mater., 2006, 18, 1988–1994 CrossRef CAS.
- H. Kong, J. Song and J. Jang, Environ. Sci. Technol., 2010, 44, 5672–5676 CrossRef CAS.
- L. Brunet, D. Y. Lyon, E. M. Hotze, P. J. J. Alveraz and M. R. Weisner, Environ. Sci. Technol., 2009, 43, 4355–4360 CrossRef CAS.
- G. Applerot, A. Lipovski, R. Dror, P. Nina, Y. Nitzan, R. Lubart and A. Gedanken, Adv. Funct. Mater., 2009, 19, 842–852 CrossRef.
- I. Perelshtein, G. Applerot, N. Perkas, E. Wehrschetz-Sigl, A. Hasmann, G. M. Guebitz and A. Gedanken, ACS Appl. Mater. Interfaces, 2009, 1, 361–366 Search PubMed.
- H. Koga, T. Kitaoka and W. Hiroyuki, J. Mater. Chem., 2009, 19, 2135–2140 RSC.
- J. Jang and Y. Kim, Chem. Commun., 2008, 4016–4018 RSC.
- M. J. Rosemary, I. MacLaren and T. Pradeep, Langmuir, 2006, 22, 10125–10129 CrossRef CAS.
- I. M. Helander, E. L. Nurmiaho-Lassila, R. Ahvenainen, J. Rhoades and S. Roller, Int. J. Food Microbiol., 2001, 71, 235–244 Search PubMed.
- O. Yamamoto, K. S. Nakakoshi, T. Sasamoto, H. Nakagawa and K. Miura, Carbon, 2001, 39, 1643–1651 Search PubMed.
- J. Sawai, J. Microbiol. Methods, 2003, 54, 177–182 CrossRef CAS.
- F. Zeng, C. Hou, S. Z. Wu, X. X. Liu, Z. Tong and S. N. Yu, Nanotechnology, 2007, 18, 055605–05612 Search PubMed.
- S. Shrivastava, T. Bera, A. Roy, G. Singh, P. Ramachandrarao and D. Dash, Nanotechnology, 2007, 18, 225103–225111 CrossRef.
- W. Lu, G. Liu, S. Gao, S. Xing and J. Wang, Nanotechnology, 2008, 19, 445711–445720 CrossRef.
- H. Koga, T. Kitaoka and H. Wariishi, J. Mater. Chem., 2009, 19, 2135–2140 RSC.
- L-H. Lee, J. C. Deng, H. R. Deng, Z. L. Liu and X. L. Li, Chem. Eng. J., 2010, 160, 378–382 Search PubMed.
- I. Sondi and B. Salopek-Sondi, J. Colloid Interface Sci., 2004, 275, 177–182 CrossRef CAS.
- W. Yang, C. Shen, Q. Ji, H. An, J. Wang, Q. Liu and Z. Zhang, Nanotechnology, 2009, 20, 085102–085108 Search PubMed.
- M. L.i, L. Zhu and D. Lin, Environ. Sci. Technol., 2011, 45, 1977–1983 Search PubMed.
- A. Lipovsky, Z. Tzitrinovich, H. Friedmann, G. Applerot, A. Gedanken and R. Lubart, J. Phys. Chem. C, 2009, 113, 15997–16001 Search PubMed.
- Q. Xiang, G. Menga, Y. Zhanga, J. Xua, P. Xub, Q. Pana and W. Yuc, Sens. Actuators, B, 2010, 143, 635–640 CrossRef.
- Y. Zheng, L. Zheng, Y. Zhan, X. Lin, Q. Zheng and K. Wei, Inorg. Chem., 2007, 46, 6980–6986 CrossRef CAS.
- W. Song, Y. Wang, H. Hu and B. Zhao, J. Raman Spectrosc., 2007, 38, 1320–1325 Search PubMed.
- M. K. Lee, T. G. Kim, W. Kim and Y. M. Sung, J. Phys. Chem. C, 2008, 112, 10079–10082 CrossRef CAS.
- Q. Zhang, T. P. Chou, B. Russo, S. A. Janice and G. Cao, Adv. Funct. Mater., 2008, 18, 1654–1660 CrossRef CAS.
- A. Dawson and P. V. Kamat, J. Phys. Chem. B, 2001, 105, 960–966 CrossRef CAS.
- H. Tada, K. Teranishi, Y. Inubushi and S. Ito, Langmuir, 2000, 16, 3304–3309 CrossRef CAS.
- H. Zhang, X. Lib and G. Chen, J. Mater. Chem., 2009, 19, 8223–8231 RSC.
- E. Tang, G. Cheng, X. Ma, X. Pang and Q. Zhao, Appl. Surf. Sci., 2006, 252, 5227–5232 Search PubMed.
- B. Variansyah, J. D. Kim, B. K. Min, Y. H. Shin, Y. W. Lee and J. Kim, J. Supercrit. Fluids, 2010, 52, 76–83 CrossRef CAS.
- C. I. Dias, J. F. Mano and N. M. Alvesa, J. Mater. Chem., 2008, 18, 2493–2499 RSC.
- H. Xie, S. Zhang and S. Li, Green Chem., 2006, 8, 630–633 RSC.
- C. Chang, S. Chen and L. Zhang, J. Mater. Chem., 2011, 21, 3865–3871 RSC.
- K. H. Chung, M. Y. Cho, M. H. Sung, H. Poo and Y. T. Lim, Chem. Commun., 2011, 47 Search PubMed.
- B. K. Woo, W. Chen, A. G. Joly and R. Sammynaiken, J. Phys. Chem. C, 2008, 112, 14292–14296 CrossRef CAS.
- T. Hirakawa and P. V. Kamat, J. Am. Chem. Soc., 2005, 127, 3928–3934 CrossRef CAS.
- L. Jin and R. Bai, Langmuir, 2002, 18, 9765–9770 CrossRef CAS.
- A. G. Karakecili, C. Satriano, M. Gumusderelioglu and G. Marletta, J. Appl. Polym. Sci., 2007, 106, 3884–3888 Search PubMed.
- N. S. Ramgir, I. S. Mulla and V. K. Pillai, J. Phys. Chem. B, 2006, 110, 3995–4001 CrossRef CAS.
- C. M. Whelan, M. R. Smyth, J. C. Barnes, M. D. Brown and C. A. Anderson, Appl. Surf. Sci., 1998, 134, 144–158 CrossRef.
- F. Li, J. Wu, Q. Qin, Z. Li and X. Huang, Superlattices Microstruct., 2010, 47, 232–240 Search PubMed.
-
J. F. Moulder, W. F. Stickle, P. E. Sobol, K. D. Bomben, Handbook of X-ray Photo electronspectroscopy: A reference Book of Standard Spectra for identification and Interpretation of XPS Data, Physical Electronics, Reissue edition, Physical Electronics, USA 18725, Lakedrive East, Chanhassen, MN 55317, 1995 Search PubMed.
- T. J. Brunner, P. Wick, P. Manser, P. Spohn, R. N. Grass, L. K. Limbach, A. Bruinink and W. J. Stark, Environ. Sci. Technol., 2006, 40, 4371–4381 Search PubMed.
- S. W. Bian, I. A. Mudunkotuwa, T. Rupasinghe and V. H. Grassian, Langmuir, 2011, 27, 6059–6068 Search PubMed.
- W.-R. Li, X. B. Xie, Q. S. Shi, H. Y. Zeng, Y. S. O-Yang and Y-B Chen, Appl. Microbiol. Biotechnol., 2010, 85, 1115–1122 Search PubMed.
- C. Marambio-Jones and E. M. V. Hoek, J. Nanopart. Res., 2010, 12, 1531–1551 CrossRef CAS.
- H. J. Klasen, Burns, 2000, 30, 1.
- K. J. Kim, W. S. Sung, B. K. Suh, S. K. Moon, J. S. Choi, J. G. Kim and D. G. Lee, BioMetals, 2009, 22, 235–242 Search PubMed.
- M. Rai, A. Yadav and A. Gade, Biotechnol. Adv., 2009, 27, 76–83 CrossRef CAS.
- J. R. Morones, J. L. Elechiguerra, A. Camacho, K. Holt, J. B. Kouri and J. T. Ramirez, Nanotechnology, 2005, 16, 2346–2353 CrossRef CAS.
- R. Sonohara, N. Muramatsu, H. Ohshima and T. Kondo, Biophys. Chem., 1995, 55, 273–277 CrossRef CAS.
- N. Mitik-Dineva, J. Wang, V. K. Truong, P. Stoddart, F. Malherbe, R. J. Crawford and E. P. Ivanova, Curr. Microbiol., 2009, 58, 268–273 Search PubMed.
- M. C. Becerra, A. J. Eraso and I. Albesa, Luminescence, 2003, 18, 334–340 Search PubMed.
Footnote |
† Electronic supplementary information (ESI) available. See DOI: 10.1039/c1ra00815c |
|
This journal is © The Royal Society of Chemistry 2012 |
Click here to see how this site uses Cookies. View our privacy policy here.