DOI:
10.1039/C1RA00679G
(Paper)
RSC Adv., 2012,
2, 151-155
Na3PO4-catalyzed aminochlorination reaction of β-nitrostyrenes in water†
Received
5th September 2011
, Accepted 6th September 2011
First published on 27th October 2011
Abstract
A facile and green aminochlorination of β-nitrostyrenes has been developed by using t-butyl N,N-dichlorocarbamate (BocNCl2) as a nitrogen/chlorine source in water at room temperature, which tolerated a wide range of substrates and resulted in the highest efficiency so far.
Introduction
Among all the methodologies for the preparation of vicinal haloamines, aminohalogenation and related reactions of functionalized olefins has become one of the most useful and attractive tools to create carbon–nitrogen and carbon–halogen bonds in tandem fashion at the same time.1–4 The resulting 1,2-haloamines from this reaction belong to an important class of building blocks in both organic synthesis and medicinal chemistry.5 In the past decades, the studies on aminohalogenation were focused on the development of high regio- and stereoselective systems. Many metal- and organocatalyzed aminohalogenation reactions have been reported for several functionalized alkenes, including α,β-unsaturated carboxylic esters,6 α,β-unsaturated nitriles,7 α,β-unsaturated ketones,8 β-nitrostyrenes9,19 and so on.10 In particular, β-nitrostyrenes were developed as a new class of substrates for aminohalogenation in the past five years, and the type of nitrogen sources for aminohalogenation was greatly improved, because β-nitrostyrenes could react well with sulfonamide, amide and carbamate resulting in the corresponding products.9 Although the alkene substrates, nitrogen sources and catalysts11 for aminohalogenation have been carefully studied, the media for such transformations still remains unexplored. Actually, the media showed a great effect on the formation of the final products in these processes. In 2004, ionic liquids were used as media for aminohalogenation, and the reaction gave higher chemical yields, but 12 h was still needed for the completion of the reaction.12 Water was also reported for the aminobromination of olefins with chloramine-T as the nitrogen source, and could shorten the reaction time, but only moderate yields were obtained.13 These examples became the pioneering attempts for green aminohalogenation reactions.
In recent years, there has been an intense drive towards developing green chemistry using more environmentally acceptable solvents.14 Water, compared to common organic reaction media, is an environment friendly, cheap, imflammable reaction medium which has often shown an unprecedented effect on the rate and selectivity of organic reactions that cannot be attained in conventional organic solvents.15 Thus, the development of an efficient procedure using water as the reaction medium has received high priority in the design of green processes.16 As a part of our ongoing efforts to develop efficient an aminohalogenation system, we surprisingly found that aminochlorination of β-nitrostyrenes could result in high efficiency when the reaction was performed in water. Herein, we would like to present our findings on the aminochlorination of β-nitrostyrenes with tert-butyl N,N-dichlorocarbamate (BocNCl2) as a nitrogen/chlorination source in water resulting in the highest efficiency so far (Scheme 1).
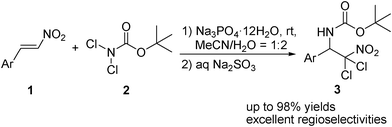 |
| Scheme 1 Aminochlorination with BocNCl2 in water medium. | |
Results and discussion
Earlier work from our laboratory showed that the aminohalogenation of functionalized olefins could give 5%–10% higher chemical yields with the using of 4 Å sieves to remove the trance amount of water from the system.7,8b However, recently reported aminohalogenation of β-nitrostyrenes could proceed smoothly without the aid of 4 Å sieves, even without the protection of the inert gas.9d Moreover, we found that the catalysts for these reactions, such as KOH and K2CO3, could not dissolve well in the organic solvents used (CH2Cl2 and CH3CN). On the basis of these results and aiming at developing a green aminohalogenation process, we tried to investigate the potential of using water as a medium for aminochlorination. Thus, β-nitrostyrene 1a and BocNCl2 was mixed in MeCN/H2O (v/v = 1
:
1) at room temperature without use of any catalyst. The reaction did not happen and most of the starting materials remained even after the reaction was extended to a quite long time (entry 1, Table 1). Then, several transition metal catalysts (entries 2–5) and organocatalysts (entries 9–11) were employed in this reaction, but no desired products were observed at all. A further scan of the catalysts found that the transformation could proceed smoothly in the presence of potassium or sodium salts resulting in excellent chemical yields (entries 6–8 and 12–14), and the best result was obtained from the catalyst Na3PO4. Particularly, these reactions proceeded very quickly, complete within 5 min in the water media. As we know, previous reported aminohalogenation reactions in common organic solvents usually need several hours, sometime two days to consume the starting materials. In the current system, water was believed to play a significant role in this transformation. It was assumed that water can dissolve the catalyst Na3PO4, which makes the process become a homogeneous reaction and therefore accelerate the reaction rate obviously.

|
Entry |
Catalyst |
Time |
Yield (%)b |
Reaction conditions: β-nitrostyrene (0.5 mmol), BocNCl2 (1.25 mmol), catalyst (20 mol%), MeCN (2.5 mL), H2O (2.5 mL).
Isolated yields.
No reaction was observed.
|
1 |
None |
48 h |
NRc |
2 |
Mn(OAc)2 |
48 h |
NRc |
3 |
Ni(OAc)2 |
48 h |
NRc |
4 |
Pd(OAc)2 |
48 h |
NRc |
5 |
CuI |
48 h |
NRc |
6 |
KOH |
5 min |
84 |
7 |
K2CO3 |
5 min |
81 |
8 |
K3PO4·3H2O |
5 min |
85 |
9 |
Ph3P |
48 h |
NRc |
10 |
DMAP |
48 h |
NRc |
11 |
2,2’-Bpy |
48 h |
NRc |
12 |
NaOH |
5 min |
79 |
13 |
Na2CO3 |
5 min |
81 |
14 |
Na3PO4·12H2O |
5 min |
89 |
The following reaction condition screening was carried out with Na3PO4 as catalyst in water medium (Table 2). As shown in Table 2, an excellent result was obtained from the reaction in MeCN/H2O (89% yield, entry 1). We found that the reactions proceeded very slowly and gave poor yields, when THF/H2O, Et2O/H2O, CH2Cl2/H2O or DMF/H2O were used as solvents (entries 2–5), as well as methanol and ethanol (entries 6 and 7). In search for a greener solvent, the reaction was performed with a higher ratio of water. The ratio of MeCN/water can be increased to 1
:
2, and the reaction also gave high efficiency (entry 8). Further increasing the ratio of H2O resulted in longer reaction time and lower chemical yield (entry 9). It was interesting that the aminochlorination reaction could even occur in pure water, although with a low chemical yield (entry 10). Finally, the loading amount of BocNCl2 was examined and it was found that the use of 2.5 equiv of BocNCl2 gave the highest yield (88%, entry 8).
Table 2 Optimization of reaction conditionsa
After establishing the optimized reaction conditions, we explored the scope and generality of the process by using a library of β-nitrostyrenes as substrates to investigate the scope for the current system (Table 3). As shown in Table 3, the alkenes substrates were converted into the corresponding haloamines with excellent regioselectivities for all cases under aqueous conditions within 12 min, and no other by-products were observed. Generally, these β-nitrostyrenes with electron-deficient substituents could participate better in the reaction and usually complete within 5 min. Especially, for the case of strong electron-withdrawing substituents, such as fluoro (entries 4 and 9), trifluoromethyl (entry 12) and nitrile (entry 13), the transformation could finish in 4 min, giving excellent chemical yields (84%–96%).
Table 3 Scope of the aminochlorination reaction in MeCN/H2Oa

|
Entry |
Ar |
Time |
Product |
Yield (%)b |
Reaction conditions: β-nitrostyrene (0.5 mmol), BocNCl2 (1.25 mmol), Na3PO4·12H2O (20 mol%), MeCN (1.6 mL), H2O (3.2 mL).
Isolated yields.
|
1 |
C6H5 |
5 min |
3a
|
88 |
2 |
2-OCH3C6H4 |
10 min |
3b
|
97 |
3 |
2-ClC6H4 |
5 min |
3c
|
98 |
4 |
3-FC6H4 |
4 min |
3d
|
87 |
5 |
3-ClC6H4 |
5 min |
3e
|
73 |
6 |
3-BrC6H4 |
5 min |
3f
|
86 |
7 |
4-CH3C6H4 |
12 min |
3g
|
83 |
8 |
4-OCH3C6H4 |
10 min |
3h
|
77 |
9 |
4-FC6H4 |
4 min |
3i
|
85 |
10 |
4-ClC6H4 |
5 min |
3j
|
94 |
11 |
4-BrC6H4 |
10 min |
3k
|
78 |
12 |
4-CF3C6H4 |
4 min |
3l
|
84 |
13 |
4-CNC6H4 |
4 min |
3m
|
96 |
14 |
3-Br,4-OCH3C6H3 |
12 min |
3n
|
90 |
The reaction time was dramatically shortened compared to reactions in common organic solvents,9 and to the best of our knowledge, this is the shortest time for the aminohalogenation of functionalized olefins until now.4–13
Furthermore, we tried to develop a more simple multicomponent system for such an aminochlorination with water as medium. The combination of BocNH2/NCS was used as nitrogen/halogen source (Scheme 2), taking place of BocNCl2. This combination of BocNH2/NCS also can work as nitrogen/chlorination source in MeCN/H2O, forming the desired haloamine product. Although the yield was lower and the reaction time was extended to 48 h, the advantage of such a multicomponent reaction lies in the commercial availability of all the reagents.
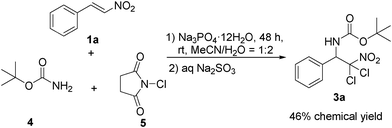 |
| Scheme 2 Aminochlorination with BocNH2/NCS in MeCN/H2O. | |
Presumably, the aminohalogenation usually proceeds through three ways: (i) halonium intermediates,8f,9a–9b,9d (ii) aziridinium intermediate,6b,6c (iii) radical intermediate,17 (iv) Michael addition.9c The mechanism involving the Michael addition intermediate presumably explains the regiochemistry obtained from the current system (Scheme 3). The existence of water can bring the catalyst Na3PO4 into the system and therefore accelerate the activation process of nitrogen BocNCl2, forming the intermediate A. Then, A does a Michael addition to β-nitrostyrene 1a giving intermediate B, followed by the trapping of Cl+ to form intermediate C, a normal monohaloamino product. Under the action of base, Na3PO4, deprotonation of the monohaloamino product occurs resulting in intermediate D. The final step is the formation of dichlorinated compound Evia electrophilic chlorination of precursor D. The final product 3a is obtained by treatment with aqueous Na2SO3.
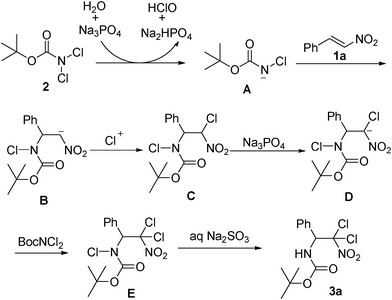 |
| Scheme 3 Proposed mechanism for the aminochlorination. | |
Conclusions
In conclusion, a facile and green aminochlorination process has been developed by using BocNCl2 as the nitrogen/chlorination source with water as the medium at room temperature. This reaction proceeded very fast and was complete within 4 min, tolerating a wide scope of substrates with good to excellent yields. Further study on the attempt of this green process to other functionalized alkenes substrates is presently under progress.
Experimental section
General information
All aminochlorination reactions were performed in vials at room temperature without protection of inert gases. BocNCl218 was prepared according to the reported methods. The other chemicals were used as obtained from commercial sources without further purification. Flash chromatography was performed using silica gel 60 (200–300 mesh). Thin layer chromatography was carried out on silica gel 60 F-254 TLC plates of 20 cm × 20 cm. Melting points are uncorrected. IR spectra were collected on Bruker Vector 22 in KBr pellets. 1H and 13C NMR (TMS used as internal standard) spectra were recorded with a Bruker ARX 300 spectrometer. High resolution mass spectra for all the new compounds were done by Micromass Q-Tof instrument (ESI†).
Aminochlorination of β-nitrostyrenes with BocNCl2 with water as medium
Into a reaction vial were taken β-nitrostyrenes (0.5 mmol), Na3PO4·12H2O (0.1 mmol), BocNCl2 (1.25 mmol), MeCN (1.6 mL) and H2O (3.2 mL). The reaction mixture was stirred at room temperature for 12 min, and then the reaction was quenched with saturated Na2SO3 (3.0 mL). The organic layer was taken and the aqueous layer was extracted with EtOAc (2 × 20 mL). The combined organic layers were dried with anhydrous Na2SO4, filtered and the solvent was removed to give the crude product 3, which was purified by TLC plate (hexane/EtOAc, 8
:
1).19
tert-Butyl 2,2-dichloro-2-nitro-1-phenylethylcarbamate (3a).
White solid, yield 88%, mp 105–106 °C. 1H NMR (CDCl3, 300 MHz): δ = 7.42 (s, 5 H), 5.99 (d, J = 10.5 Hz, 1 H), 5.56 (d, J = 10.5 Hz, 1 H), 1.45 (s, 9 H).
tert-Butyl 2,2-dichloro-1-(2-methoxyphenyl)-2-nitroethylcarbamate (3b).
White solid, yield 97%, mp 152–154 °C. 1H NMR (CDCl3, 300 MHz): δ = 7.33–7.42 (m, 2 H), 6.94–7.03 (m, 2 H), 6.28 (d, J = 10.5 Hz, 1 H), 6.08 (d, J = 9.6 Hz, 1 H), 3.88 (s, 3 H)? 1.46 (s, 9 H). 13C NMR (CDCl3, 75 MHz): δ = 157.7, 154.3, 130.9, 130.6, 120.8, 116.5, 112.8, 111.6, 81.0, 61.0, 55.6, 28.2. IR (KBr): ν = 3253, 3142, 2978, 1697, 1585, 1365, 1249, 1160, 756 cm−1. HRMS [M+Na+]: calcd for C14H18N2O5Cl2Na: 387.0485, found: 387.0490.
tert-Butyl 2,2-dichloro-1-(2-chlorophenyl)-2-nitroethylcarbamate (3c).
White solid, yield 98%, mp 168–170 °C. 1H NMR (CDCl3, 300 MHz): δ = 7.48–7.51 (m, 2 H), 7.33–7.40 (m, 2 H), 6.75 (d, J = 10.2 Hz, 1 H), 5.58 (d, J = 10.2 Hz, 1 H), 1.44 (s, 9 H).
tert-Butyl 2,2-dichloro-1-(3-fluorophenyl)-2-nitroethylcarbamate (3d).
White solid, yield 87%, mp 115–117 °C. 1H NMR (CDCl3, 300 MHz): δ = 7.35–7.43 (m, 1 H), 7.10–7.24 (m, 3 H), 5.99 (d, J = 8.7 Hz, 1 H), 5.55 (s, 1 H), 1.45 (s, 9 H).
tert-Butyl 2,2-dichloro-1-(3-chlorophenyl)-2-nitroethylcarbamate (3e).
White solid, yield 73%, mp 136–138 °C. 1H NMR (CDCl3, 300 MHz): δ = 7.33–7.45 (m, 4 H), 5.97 (d, J = 9.3 Hz, 1 H), 5.58 (s, 1H), 1.45 (s, 9 H).
tert-Butyl 1-(3-bromophenyl)-2,2-dichloro-2-nitroethylcarbamate (3f).
White solid, yield 86%, mp 152–154 °C. 1H NMR (CDCl3, 300 MHz): δ = 7.55–7.60 (m, 2 H), 7.39 (d, J = 7.2 Hz, 1 H), 7.31 (d, J = 7.8 Hz, 1 H), 5.96 (d, J = 9.0 Hz, 1 H), 5.57 (d, J = 8.7 Hz, 1 H), 1.45 (s, 9 H).
tert-Butyl 2,2-dichloro-2-nitro-1-p-tolylethylcarbamate (3g).
White solid, yield 83%, mp 108–110 °C. 1H NMR (CDCl3, 300 MHz): δ = 7.32 (d, J = 7.8 Hz, 2 H), 7.23 (d, J = 8.4 Hz, 2 H), 5.95 (d, J = 9.9 Hz, 1 H), 5.58 (d, J = 9.0 Hz, 1 H), 2.38 (s, 3 H), 1.45 (s, 9 H).
tert-Butyl 2,2-dichloro-1-(4-methoxyphenyl)-2-nitroethylcarbamate (3h).
White solid, yield 77%, mp 113–114 °C. 1H NMR (CDCl3, 300 MHz): δ = 7.35 (d, J = 8.7 Hz, 2 H), 6.93 (d, J = 9.0 Hz, 2 H), 5.93 (d, J = 9.3 Hz, 1 H), 5.54 (d, J = 9.9 Hz, 1 H), 3.83 (s, 3 H), 1.45 (s, 9 H).
tert-Butyl 2,2-dichloro-1-(4-fluorophenyl)-2-nitroethylcarbamate (3i).
White solid, yield 85%, mp 109–110 °C. 1H NMR (CDCl3, 300 MHz): δ = 7.40–7.44 (m, 2 H), 7.08–7.13 (m, 2 H), 5.97 (d, J = 9.6 Hz, 1 H), 5.55 (d, J = 9.9 Hz, 1 H), 1.44 (s, 9 H).
tert-Butyl 2,2-dichloro-1-(4-chlorophenyl)-2-nitroethylcarbamate (3j).
White solid, yield 94%, mp 101–102 °C. 1H NMR (CDCl3, 300 MHz): δ = 7.38 (s, 4 H), 5.96 (d, J = 8.7 Hz, 1 H), 5.54 (s, 1 H), 1.44 (s, 9 H).
tert-Butyl 1-(4-bromophenyl)-2,2-dichloro-2-nitroethylcarbamate (3k).
White solid, yield 78%, mp 106–107 °C. 1H NMR (CDCl3, 300 MHz): δ = 7.57 (d, J = 8.4 Hz, 2 H), 7.32 (d, J = 8.4 Hz, 2 H), 5.95 (d, J = 9.9 Hz, 1 H), 5.52 (s, 1 H), 1.44 (s, 9 H).
tert-Butyl 2,2-dichloro-2-nitro-1-(4-(trifluoromethyl)phenyl)ethylcarbamate (3l).
White solid, yield 84%, mp 118–119 °C. 1H NMR (CDCl3, 300 MHz): δ = 7.70 (d, J = 8.4 Hz, 2 H), 7.60 (d, J = 8.1 Hz, 2 H), 6.06 (d, J = 9.0 Hz, 1 H), 5.60 (d, J = 9.3 Hz, 1 H), 1.44 (s, 9 H).
tert-Butyl 2,2-dichloro-1-(4-cyanophenyl)-2-nitroethylcarbamate (3m).
White solid, yield 96%, mp 129–130 °C. 1H NMR (CDCl3, 300 MHz): δ = 7.74 (d, J = 7.5 Hz, 2 H), 7.60 (d, J = 5.7 Hz, 2 H), 6.04 (d, J = 5.1 Hz, 1 H), 5.63 (d, J = 4.8 Hz, 1 H), 1.43 (s, 9 H).
tert-Butyl 1-(3-bromo-4-methoxyphenyl)-2,2-dichloro-2-nitroethylcarbamate (3n).
White solid, yield 90%, mp 169–171 °C. 1H NMR (CDCl3, 300 MHz): δ = 7.62 (s, 1 H), 7.34 (d, J = 7.2 Hz, 1 H), 6.92 (d, J = 8.7 Hz, 1 H), 5.91 (d, J = 7.8 Hz, 1 H), 5.51 (d, J = 7.8 Hz, 1 H), 3.92 (s, 3 H), 1.45 (s, 9 H).
Aminochlorination of β-nitrostyrene with BocNH2 and NCS with water as medium
Into a reaction vial were taken β-nitrostyrenes (0.5 mmol), Na3PO4·12H2O (0.1 mmol), BocNH2 (1.5 mmol), NCS (1.5 mmol), MeCN (1.6 mL) and H2O (3.2 mL). The reaction mixture was stirred at room temperature for 48 h, and then the reaction was quenched with saturated Na2SO3 (3.0 mL). The organic layer was taken and the aqueous layer was extracted with EtOAc (2 × 20 mL). The combined organic layers were dried with anhydrous Na2SO4, filtered and the solvent was removed to give the crude product, which was purified by TLC plate (hexane/EtOAc, 8
:
1).
Acknowledgements
We gratefully acknowledge the financial support from the National Natural Science Foundation of China (No. 21102071) and the Fundamental Research Funds for the Central Universities (No. 1107020522 and No. 1082020502). The Jiangsu 333 program (for Pan) is also acknowledged.
References
-
J. E. Kemp, In Comprehensive Organic Synthesis, Vol. 3; B. M. Trost and I. Fleming, ed.; Pergamon Press: Oxford, 1991, 469–513 Search PubMed.
-
(a) Y. Y. Yeung, X. Gao and E. J. Corey, J. Am. Chem. Soc., 2006, 128, 9644 CrossRef CAS;
(b) D. A. Griffith and S. J. Danishefsky, J. Am. Chem. Soc., 1991, 113, 5863 CrossRef CAS;
(c) J. Lessard, H. Driguez and J. P. Vermes, Tetrahedron Lett., 1970, 11, 4887 CrossRef.
-
(a) F. A. Daniher and P. E. Butler, J. Org. Chem., 1968, 33, 4336 CrossRef CAS;
(b) F. A. Daniher and P. E. Butler, J. Org. Chem., 1968, 33, 2637 CrossRef CAS;
(c) F. A. Daniher, M. T. Melchior and P. E. Butler, Chem.Commun., 1968, 931 RSC.
- For recent review on aminohalogenation, see:
G. Li, S. R. S. Saibabu Kotti and C. Timmons, Eur. J. Org. Chem., 2007, 2745 Search PubMed.
- J. Qui and R. B. Silverman, J. Med. Chem., 2000, 43, 706 CrossRef.
-
(a) G. Li, H. X. Wei, S. H. Kim and M. Neighbors, Org. Lett., 1999, 1, 395–397 CrossRef CAS;
(b) H. X. Wei, S. H. Kim and G. Li, Tetrahedron, 2001, 57, 3869–3873 CrossRef CAS;
(c) G. Li, H. X. Wei and S. H. Kim, Tetrahedron, 2001, 57, 8407–8411 CrossRef CAS.
- J. L. Han, S. J. Zhi, L. Y. Wang, Y. Pan and G. Li, Eur. J. Org. Chem., 2007, 1332 CrossRef CAS.
-
(a) H. Sun, G. Q. Zhang, S. J. Zhi, J. L. Han, G. Li and Y. Pan, Org. Biomol. Chem., 2010, 8, 4236 RSC;
(b) D. Chen, C. Timmons, S. Chao and G. Li, Eur. J. Org. Chem., 2004, 3097–3101 CrossRef CAS;
(c) Z. G. Chen, J. F. Wei, R. T. Li, X. Y. Shi and P. F. Zhao, J. Org. Chem., 2009, 74, 1371 CrossRef CAS;
(d) J. F. Wei, L. H. Zhang, Z. G. Chen, X. Y. Shi and J. J. Cao, Org. Biomol. Chem., 2009, 7, 3280–3284 RSC;
(e) X. L. Wu, J. J. Xia and G. W. Wang, Org. Biomol. Chem., 2008, 6, 548–553 RSC;
(f) V. V. Thakur, S. K. Talluri and A. Sudalai, Org. Lett., 2003, 5, 861 CrossRef CAS.
-
(a) S. J. Zhi, J. L. Han, C. Lin, G. H. An, Y. Pan and G. Li, Synthesis, 2008, 1570 CAS;
(b) S. J. Zhi, H. Sun, C. Lin, G. Q. Zhang, G. Li and Y. Pan, Sci. China Chem., 2010, 53, 140–146 CrossRef CAS;
(c) Z. G. Chen, Y. Wang, J. F. Wei, P. F. Zhao and X. Y. Shi, J. Org. Chem., 2010, 75, 2085–2088 CrossRef CAS;
(d) S. J. Zhi, H. B. Mei, G. Q. Zhang, H. Sun, J. L. Han, G. Li and Y. Pan, Sci. China Chem., 2010, 53, 1946–1952 CrossRef CAS.
- Q. Li, M. Shi, C. Timmons and G. Li, Org. Lett., 2006, 8, 625 CrossRef CAS.
-
(a) Z. G. Chen, L. Y. Zhou, W. L. Li, J. M. Zhou and C. N. Wang, Acta Chim. Sinica, 2011, 69, 1093–1100 CAS;
(b) Z. G. Chen, J. F. Wei, M. Z. Wang, L. Y. Zhou, C. J. Zhang and X. Y. Shi, Adv. Synth. Catal., 2009, 351, 2358–2368 CrossRef CAS;
(c) J. F. Wei, Z. G. Chen, W. Lei, L. H. Zhang, M. Z. Wang, X. Y. Shi and R. T. Li, Org. Lett., 2009, 11, 4216–4219 CrossRef CAS;
(d) T. M. Shaikh, P. U. Karabal, G. Suryavanshi and A. Sudalai, Tetrahedron Lett., 2009, 50, 2815–2817 CrossRef CAS;
(e) S. Minakata, Y. Yoneda, Y. Oderaotoshi and M. Komatsu, Org. Lett., 2006, 8, 967–969 CrossRef CAS.
- S. R. S. Saibabu Kotti, X. Xu, Y. N. Wang, A. D. Headley and G. G. Li, Tetrahedron Lett., 2004, 45, 7209–7212 CrossRef.
-
(a) X. L. Wu and G. W. Wang, Tetrahedron, 2009, 65, 8802–8807 CrossRef CAS;
(b) X. L. Wu and G. W. Wang, J. Org. Chem., 2007, 72, 9398–9401 CrossRef CAS.
-
(a) R. A. Sheldon, Green Chem., 2005, 7, 267–278 RSC;
(b) B. C. Ranu, A. Saha and R. Dey, Curr. Opin. Drug Discov. Devel., 2010, 13, 658–668 CAS;
(c) T. Erdmenger, C. G. Sanchez, J. Vitz, R. Hoogenboom and U. S. Schubert, Chem. Soc. Rev., 2010, 39, 3317–3333 RSC.
-
(a)
C. J. Li and T. H. Chan, in Organic Reactions in Aqueous Media, Wiley, New York, 1997 Search PubMed;
(b)
Organic Synthesis in Water, ed. P. A. Grieco, Blackie Academic and Professional, London, 1998 Search PubMed;
(c) A. Chanda and V. V. Fokin, Chem. Rev., 2009, 109, 725–748 CrossRef CAS.
-
(a) Y. Gu and F. Jerome, Green Chem., 2010, 12, 1127 RSC;
(b) D. Alves, M. Sachini, R. G. Jacob, E. J. Lenardao, M. E. Contreira, L. Savegnago and G. Perin, Tetrahedron Lett., 2011, 52, 133 CrossRef CAS;
(c) L. C. Goncalves, G. F. Fiss, G. Perin, D. Alves, R. G. Jacob and E. J. Lenardao, Tetrahedron Lett., 2010, 51, 6772–6775 CrossRef CAS.
-
(a) T. A. Foglia and D. Swern, J. Org. Chem., 1966, 31, 3625 CrossRef CAS;
(b) T. A. Foglia and D. Swern, J. Org. Chem., 1968, 33, 766 CrossRef CAS;
(c) B. Crookes, T. P. Seden and R. W. Turner, Chem.Commun., 1968, 324 Search PubMed.
- R. E. White and P. Kovacic, J. Am. Chem. Soc., 1975, 97, 1180 CrossRef CAS.
- For the known compounds, the 1H NMR spectra and melting point have been confirmed to be identical to the literature:
H. B. Mei, J. L. Han, G. G. Li and Y. Pan, RSC Adv., 2011, 1, 429-433 Search PubMed.
Footnote |
† Electronic Supplementary Information (ESI) available: Experimental procedures, full spectroscopic data for new compounds, and copies of 1H NMR and 13C NMR spectra. See DOI: 10.1039/c1ra00679g/ |
|
This journal is © The Royal Society of Chemistry 2012 |
Click here to see how this site uses Cookies. View our privacy policy here.