Influence of polarity of the peripheral substituents of porphyrin molecules on the photocatalytic activity of Cu(II) porphyrin modified TiO2 composites
Received
13th October 2011
, Accepted 6th January 2012
First published on 9th January 2012
Abstract
Two novel porphyrins, 5,10,15-tri-phenyl-20-[4-(2-(4-formoxyl) phenoxy)ethoxy]phenyl porphyrin and 5,10,15-tri-phenyl-20-[4-(2-(4-hydroxyimino) phenoxy)ethoxy]phenyl porphyrin, and their corresponding copper(II) complexes were synthesized and spectroscopically characterized. The Cu(II) porphyrin–TiO2 composites were also prepared and characterized. The photoactivity of the composites was investigated by carrying out the degradation of 4-nitrophenol (4-NP) in aqueous solution under UV-visible light. It was found that the photoactivity of Cu(II) porphyrin–TiO2 composites was evidently influenced by the interaction between porphyrin molecules and hydroxyls anchored on the TiO2. Furthermore, the photoactivity of Cu(II) porphyrin–TiO2 composites was also affected by dispersion of composites in 4-NP solution.
1 Introduction
Photocatalysis is a promising method for the treatment and purification of polluted water.1,2 As a kind of economical and environment-friendly catalyst, the porphyrin-sensitized TiO2 has been proposed for the oxidative degradation of various kinds of organic pollutants in water.3–5 It is not only because the porphyrins have good chemical stability, but also they can provide more photoinduced electrons that transfer from the excited porphyrins to the conduction band of TiO2.6–8
In recent years increasing interest has been centered on enhancing photodegradation efficiency of porphyrin–TiO2 composites. Several factors have been proved to influence the efficiency, including the center metal, the peripheral substituents of porphyrin molecules, axial ligand, the crystal form and specific surface area of TiO2.9–15 In fact, different polarity of the substituents of porphyrin molecules may change the surface properties of TiO2 nanoparticles through physical or chemical adsorption of the composites. Furthermore, the electron transfer on the surface of TiO2 is influenced by the interaction between porphyrin molecules and hydroxyls anchored on the TiO2, leading to the change in the photocatalytic efficiency.
For these reasons, two porphyrins with different polarity of substituents, 5,10,15-tri-phenyl-20-[4-(2-(4-formoxyl)-phenoxy)-ethoxy] phenyl porphyrin (1b), 5,10,15-tri-phenyl-20-[4-(2-(4-hydroxyimino) phenoxy)-ethoxy]phenyl porphyrin (1c), and their corresponding copper(II) porphyrins (2b, 2c) were synthesized and characterized in this paper (Fig. 1). The CuPp–TiO2 photocatalysts were prepared and characterized by infrared spectroscopy (IR), X-ray diffraction (XRD), UV-vis diffuse reflectance spectrum (DRS), scanning electron microscopy (SEM) and energy-dispersive X-ray spectroscopy (EDS). The photocatalytic activity of the catalysts was evaluated by measuring the degradation of 4-nitrophenol (4-NP), and Cu(II) tetraphenyl porphyrin (CuTPP) was also used to impregnate TiO2, denoted CuTPP–TiO2, and it served for comparative purposes. The correlative factors influencing the photoactivity were also investigated.
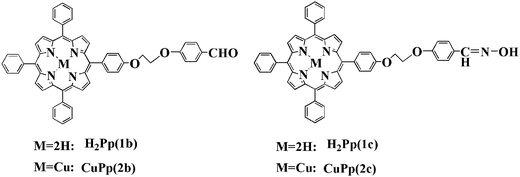 |
| Fig. 1 The structure of porphyrins and their copper porphyrins. | |
2 Experimental
2.1 Reagents and materials
4-Hydroxybenzaldehyde, 4-(2-bromoethoxy)benzaldehyde and 1,2-dibromoethane were obtained from Sinopharm Chemical Reagent Co. Ltd, other reagents were obtained from Tianjin Chemical Reagents Company. They were used without further purification except for pyrrole, which was distilled before use. TiO2 was purchased from Acros Organics, USA (anatase phase, BET specific surface area 9 m2 g−1), used in preparation of loaded samples and applied as a photocatalyst in photoreactivity experiments. Synthesis and purification of H2TPP and CuTPP were carried out according to the literature procedure.16
2.2 Equipment
A Model XPA-VII photocatalytic reactor (Xujiang Electromechanical Plant, Nanjing, China) was used for the degradation of 4-NP. Elemental analysis (C, H and N) was performed by a Vario EL-III CHNOS instrument. FT-IR spectra were obtained with samples in a KBr matrix for the title complexes on A BEQUZNDX-550 series FT-IR spectrophotometer in the range of 4000–400 cm−1. UV-vis spectra were recorded by a Shimadzu UV-1800 UV-vis-NIR spectrophotometer. 1H NMR spectra were recorded at room temperature using a Varian Inova 400 MHZ apparatus and tetra-methylsilane (TMS) for reference. Mass spectrometry (MS) analysis was carried out on a matrix assisted laser desorption/ionization time of flight mass spectrometer (MALDI-TOF MS, Krato Analytical Company of Shimadzu Biotech, Manchester, Britain) using a standard procedure involving 1 mL of the sample solution. The morphologies of the samples were analyzed by scanning electron microscopy (Quanta 400FEG) with energy dispersive X-ray spectrometry. The X-ray diffraction measurement was performed with a Bruker D8 diffractometer using graphite monochromatic copper radiation (Cu Kα) at 40 kV, 30 mA over the 2θ range of 20–70°.
2.3 Synthesis of porphyrins and copper porphyrins
5-(4-Hydroxyphenyl)-10,15,20-triphenylporphyrin (1a).
4-Hydroxybenzaldehyde (15 mmol, 1.83 g), benzaldehyde (45 mmol, 4.6 mL) and pyrrole (60 mmol, 4.15 mL) reacted in refluxing propionic acid for about 50 min.17 The crude product was purified on a silica-gel column with dichloromethane as eluent. Yield: 25%. Mp: >250 °C. Anal. calcd. (found) for C44H30N4O (mol. wt: 630.74), %: C, 83.75 (83.79); H, 4.78 (4.79); N, 8.91 (8.88). MS: m/z 630.2 ([M+H]+) amu. UV-vis(CH2Cl2): λmax/nm, 420 (Soret band), 517, 550, 590, 654 (Q bands). FT-IR: ν, cm−1, 3429, 3316, 3053, 1610, 1472, 1349, 1217, 1073, 966, 800, 702.
5,10,15-Triphenyl-20-[4-(2-(4-formoxyl)phenoxy)ethoxy]phenyl porphyrin (1b).
The hydroxy-derivatized porphyrin 1a (0.2 mmol, 0.126 g) and 4-(2-bromoethoxy) benzaldehyde (2 mmol, 0.458 g) were mixed together in 10 mL of DMF. The solution was stirred in the presence of K2CO3 (0.15–0.2 g) for 12 h at 60 °C in the darkness. The DMF was evaporated under a vacuum drier after the reaction was complete, and the residue was dissolved in CH2Cl2 and purified on a silica-gel column using dichloromethane as eluent. Yield: 85%. Mp: >250 °C. Anal. calcd. (found) for C53H38N4O3 (mol. wt: 778.89), %: C, 81.71 (81.73); H, 4.96 (4.92); N, 7.21 (7.19). MS: m/z 779.71 ([M+H]+) amu. UV-vis(CH2Cl2): λmax/nm, 418 (Soret band), 515, 550, 590, 646 (Q bands). FT-IR: ν, cm−1, 3419, 2797, 2734, 1687, 1601, 1577, 1507, 1253, 965, 830, 800. 1H NMR (CDCl3, 400 MHz): δ, ppm 9.90 (s, 1H, aldehyde-H), 8.85 (m, 8H, β position of the pyrrole moiety), 8.22 (m, 6H, ortho-phenyl-H), 7.84 (m, 9H, meta- and para-phenyl-H), 7.75–7.20 (d, d, 4H, substituted phenyl-H), 5.28 (m, 4H, phenyl-H), 4.42, 3.61 (t, t, 2H, 2H, CH2-CH2), −2.76 (s, 2H, imino-H).
5,10,15-Triphenyl-20-[4-(2-(4-hydroxyimino)phenoxy)ethoxy]phenyl porphyrin (1c).
Magnesium sulfate (4 mmol, 0.5 g) and triethylamine (0.004 mmol, 0.5 mL) were added to a solution of 1b (0.1 mmol, 0.078 g) and hydroxylamine hydrochloride (1 mmol, 0.07 g) in dichloromethane, and the mixture was stirred for 24 h at room temperature, followed by filtration of MgSO4 and evaporation of the solvent. Further purification of the residue was carried out on a silica-gel column with dichloromethane as eluent. Yield: 65%. Mp: >250 °C. Anal. calcd. (found) for C53H39N5O3 (mol. wt: 793.91), %: C, 80.21 (80.18); H, 4.98 (4.95); N, 8.77 (8.82). MS: m/z 794.67 ([M+H]+) amu. UV-vis(CH2Cl2): λmax/nm, 418 (Soret band), 515, 550, 590, 646 (Q bands). FT-IR: ν, cm−1, 3427, 2924, 1606, 1512, 1240, 1174, 970, 800. 1H NMR (CDCl3, 400 MHz): δ, ppm 8.84 (m, 8H, β position of the pyrrole moiety), 8.22 (m, 6H, ortho-phenyl-H), 8.08 (s, 1H, -OH), 7.76 (m, 9H, meta- and para-phenyl-H), 7.75–7.25 (d, d, 4H, substituted phenyl-H), 6.92 (s, 1H, –CH=N),4.61 (m, 4H, phenyl-H), 4.30, 3.64 (t, t, 2H, 2H, CH2-CH2), -2.77 (s, 2H, imino-H).
Copper(II) 5,10,15-triphenyl-20-[4-(2-(4-formoxyl)phenoxy)ethoxy]phenyl porphyrin (2b).
An excess of ten times of Cu(OAC)2 was added to the solution of 1b in dichloromethane and ethanol with stirring at room temperature for 12 h. After the filtration of unreacted solid salt and evaporation of solvent, the crude product was purified on a silica-gel column with dichloromethane as eluent. Yield: 95%. Mp: >250 °C. Anal. calcd. (found) for C53H36N4O3Cu (mol. wt: 840.42), %: C, 75.78 (75.74); H, 4.30 (4.32); N, 6.65 (6.67); Cu, 7.54 (7.56). MS: m/z 841.71 ([M+H]+) amu. UV-vis(CH2Cl2): λmax/nm, 415 (Soret band), 539, 573 (Q bands). FT-IR: ν, cm−1, 3437, 2925, 2803, 2730, 1685, 1601, 1504, 1251, 1161, 827.
Copper(II) 5,10,15-triphenyl-20-[4-(2-(4-hydroxyimino)phenoxy)ethoxy]phenyl porphyrin (2c).
2c was prepared in the same method as that of 2b except that 1c was used instead of 1b. Yield: 95%. Mp: >250 °C. Anal. calcd. (found) for C53H37N5O3Cu (mol. wt: 855.44), %: C, 74.39 (74.41); H, 4.37 (4.36); N, 8.22 (8.19); Cu, 7.44 (7.43). MS: m/z 858.45 ([M+H]+) amu. UV-vis(CH2Cl2): λmax/nm, 415 (Soret band), 539, 573 (Q bands). FT-IR: ν, cm−1, 3435, 2924, 1602, 1508, 1236, 1174, 999, 800.
2.4 Preparation of the CuPp–TiO2 photocatalysts
Preparation procedures of the CuPp–TiO2 (2b–TiO2 and 2c–TiO2) photocatalysts18 are as follows: 6 μmol amounts of 2b or 2c in 30 mL CH2Cl2 solution and 1 g TiO2 were added. The suspension was stirred for 12 h at room temperature. Then the solvent was removed using a rotary evaporator and the photocatalyst was collected.
2.5 Photodegradation setup and photodegradation experiment
The photodegradation experiment was carried out by using a Model XPA-VII photocatalytic reactor consisting twelve 50 mL quartz tubes irradiated by a 400 W, 80 V Halogen lamp providing UV-vis light. The average light intensity on the sample surface was about 615 mW·cm−2. The temperature inside the reactor was maintained at 25 °C with a continuous circulation of water running through an interlayer.
The reacting suspension consisted of 4-NP (10−4 mol L−1, 50 mL) and the photocatalyst (0.01 g) was magnetically stirred with air bubbled into the suspension for 30 min before irradiation. The photodegradation lasted 66 min. ∼3 mL of the suspension was taken out to be centrifuged every 6 min during the irradiation and the solution of 4-NP was obtained by collecting the supernatant liquid. The quantitative determination of 4-NP was carried out by measuring its absorption at 317 nm with a Shimadzu UV-1800 UV-vis spectrophotometer.
3 Results and discussion
3.1 Synthesis of the porphyrins (1a,1b,1c) and copper(II) porphyrins CuPp (2b, 2c)
The synthetic routes are illustrated in Scheme 1. The porphyrin 1a was synthesized using the Adler–Longo method. The porphyrin 1b was synthesized by reaction of 1a porphyrin with 4-(2-bromoethoxy)benzaldehyde in DMF in the presence of K2CO3. The porphyrin 1c was synthesized through 1b porphyrin reacting with hydroxylamine hydrochloride in the presence of magnesium sulfate and triethylamine with a yield of 65%. Successively, the CuPp (2b, 2c) were obtained by the reaction of free porphyrin H2Pp (1b, 1c) with Cu(OAc)2 in CH2Cl2/C2H5OH with yields of about 95%.
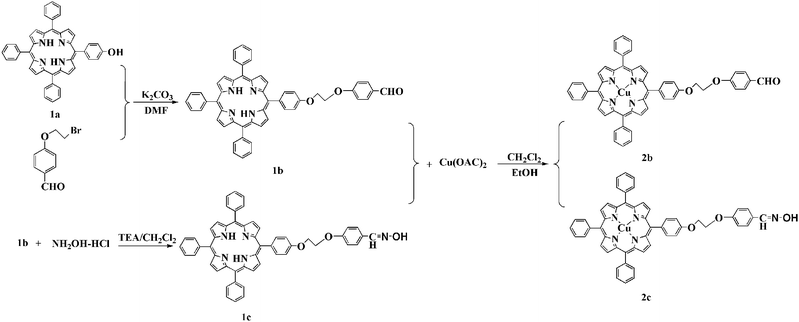 |
| Scheme 1 Synthesis of the porphyrins (1b,1c) and copper(II) porphyrins CuPp (2b,2c). | |
The UV-vis spectra of H2Pps (1b, 1c) show almost the same Soret and Q bands. The insertion of Cu(II) into the porphyrin ring caused a violet shift of 3 nm of the corresponding Soret bands for UV-vis spectra as well as a decreasing number of Q bands, which due to the symmetry of the porphyrin ring increases when the hydrogen ions of N–H are replaced by Cu(II). Mass spectroscopy data of 1b, 1c, 2b, 2c perfectly correspond to the expected [M+H]+m/z values. 1H NMR spectra were also consistent with the structure of the isolated 1b, 1c. The main change in the IR spectra of copper porphyrins compared with free base porphyrins is the disappearance of stretching vibration of N–H.
3.2 Morphology of photocatalysts
The SEM images of the different photocatalysts microspheres are shown in Fig. 2. It can be observed that the microsphere of CuPp–TiO2 possesses a similar morphology as bare TiO2, which indicates that the TiO2 microspheres are basically unchanged before and after loading with the copper porphyrin. The EDS results of the CuPp (2c)–TiO2 photocatalyst (as shown in Fig. 3) revealed that the Cu element presented on the surface of TiO2. The Cu element existing in TiO2 particles is probably derived from CuPp.
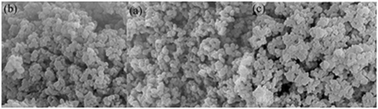 |
| Fig. 2 SEM images of bare TiO2 (a), 2b–TiO2 (b) and 2c–TiO2 (c). | |
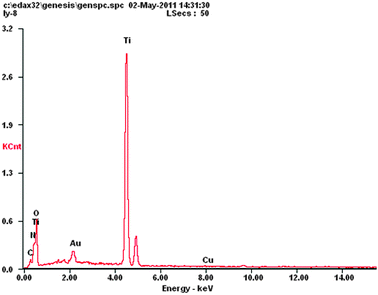 |
| Fig. 3 EDS spectrum of the 2c–TiO2 composite. | |
3.3 Diffuse reflectance spectra of photocatalysts
Fig. 4(a) shows the diffuse reflectance spectra of the bare TiO2, 2b–TiO2 and 2c–TiO2 photocatalysts recorded in the range of 300–750 nm. The absorption spectra of 2b and 2c are also shown in Fig. 4(b) for comparison. Obviously, there is no absorption above 400 nm for bare TiO2, while 2b–TiO2 and 2c–TiO2 exhibit the feature peaks of copper porphyrins observed in CH2Cl2 solution, indicating that 2b, 2c have been successfully loaded onto the TiO2 surface, maintaining the porphyrin structure framework. Furthermore, the Soret bands of 2b or 2c–TiO2 photocatalysts have a significant red-shift compared with the spectra of 2b, 2c in CH2Cl2 solution (Table 1), indicating the interaction between the porphyrin molecules and TiO2.
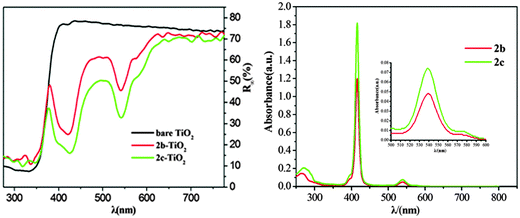 |
| Fig. 4 (a) The diffuse reflectance spectra of bare TiO2 and CuPp–TiO2 photocatalysts. (b) UV-vis spectra of CuPp (2b, 2c) in CH2Cl2. | |
Table 1 UV-vis data of the CuPps and diffuse reflectance spectral data of photocatalysts
Compounds |
|
λ
max/nm |
|
2b
|
415 |
539 |
573 |
2b–TiO2 |
422 |
542 |
|
2c
|
415 |
539 |
573 |
2c–TiO2 |
426 |
542 |
|
3.4 FT-IR spectra of photocatalysts
The FT-IR spectra of bare TiO2, 2b–TiO2 and 2c–TiO2 photocatalysts are displayed in Fig. 5. The absorption peak at 3430 cm−1 is deemed as the stretching vibrations of surface hydroxyl groups of TiO2 and the band around 1630 cm−1 is attributed to the bending vibration of the Ti–OH bond.19 In addition, several absorption peaks around 2925 cm−1 and 1130 cm−1 are observed in 2b–TiO2, 2c–TiO2 photocatalysts which are associated with the stretching vibrations of C–H and C–C, respectively, indicating the presence of CuPp on the surface of TiO2. Further observation shows that the intensity of peaks at 3430 cm−1 decreases in the 2b–TiO2 and the 2c–TiO2 compared with bare TiO2, indirectly reflecting the different quantity of hydroxyl groups on the surface of TiO2.
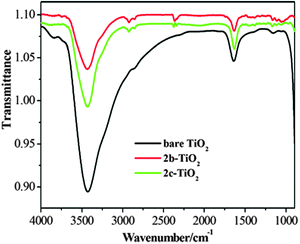 |
| Fig. 5 FT-IR spectra of bare TiO2 and CuPp–TiO2 composites. | |
3.5 XRD characterization of CuPp–TiO2 photocatalysts
The XRD patterns of the bare TiO2 and 2b–TiO2, 2c–TiO2 photocatalysts are shown in Fig. 6. No differences are found in these photocatalysts, and the prominent diffraction peaks at 25.5°, 37.5°, 47.7°, 53.4° and 62.8° are observed which were attributed to anatase TiO2.20 These results indicated that the 2b, 2c porphyrins which were loaded on the surface of TiO2 microsphere had no effect on the crystal structure of TiO2 powders.
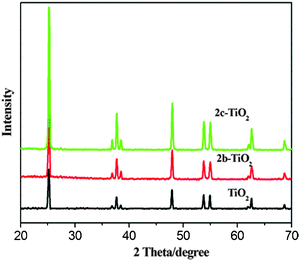 |
| Fig. 6 XRD patterns of the bare TiO2 and CuPp–TiO2 composites. | |
3.6 Photocatalytic activity of photocatalysts
The photocatalytic activities for the degradation of 4-NP in aqueous solution under halogen lamp irradiation using the prepared 2b–TiO2, 2c–TiO2 and CuTPP–TiO2 photocatalysts were evaluated as Fig. 7 illustrates. The blank group showed no effects on the degradation of 4-NP, indicating that the 4-NP was difficult to photo-degrade in the absence of a photocatalyst. Under halogen lamp irradiation, all three composites, 2b–TiO2, 2c–TiO2 and CuTPP–TiO2, showed much enhanced photoactivity than bare TiO2, and in particular 2c–TiO2 exhibited the highest efficiency of all samples, in the following order:
2c–TiO2 > 2b–TiO2 > CuTPP–TiO2 > TiO2 (bare) |
3.7 The influence of polarity of the substituents of porphyrin on the photoactivity
During the preparation of photocatalysts, it is found that the 2c is hardly washed out from the surface of TiO2 nanoparticles using dichloromethane as solvent, but 2b is easily separated from the surface of TiO2 under the same conditions. From the above phenomena, it is estimated that 2c has stronger interaction with hydroxyls located on the surface of TiO2. The results correspond to the larger polarity of peripheral substituent of 2c than that of 2b.
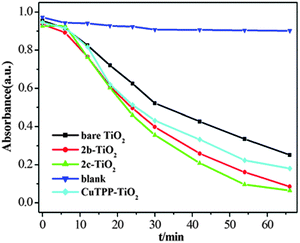 |
| Fig. 7 4-NP concentration vs. irradiation time using different photocatalysts. | |
It can be illustrated that the photocatalytic efficiency of the CuPp–TiO2 nanocomposites was better than that of the bare TiO2. Under halogen lamp irradiation, copper porphyrins produce photoinduced electrons which can transfer to the conduction band of the TiO2, then the oxygen molecules on the TiO2 surface obtain the electrons and produce reactive species O2˙− and ˙OH for the degradation of 4-NP. The larger the polarity of the substituent group for the copper porphyrin is, the stronger the interaction with the TiO2 will be, and thus accelerates the transfer process of the photoinduced electrons. 2c has the largest polarity, then 2b, and CuTPP has the least polarity. As a result, the 2c–TiO2 composite shows the highest photocatalytic efficiency. It can be included that the polarity of the substituent group on the porphyrin is an important factor for the photocatalytic efficiency.
Further observation of degradation of 4-NP shows that the photocatalytic efficiency of 2b–TiO2 is a little better than that of 2c–TiO2 during the beginning 20 min. This may be due to the dispersion of the composites in the solution. As shown in Fig. 8, the 2b–TiO2 composite shows much better dispersion than that of the 2c–TiO2 composite before irradiating, which corresponds to the smaller polarity of substituent of 2b and weaker interaction with TiO2 nanoparticles. The result indicates that the larger polarity of substituents of porphyrin molecules may reduce the hydrophilicity of TiO2 nanoparticles, leading to the reduction of dispersion.
 |
| Fig. 8 Illustration for 2b–TiO2 photocatalyst (A, C), 2c–TiO2 photocatalyst (B, D) (upper: before irradiation; down: after irradiation). | |
After irradiating for about 20 min, the dispersion of the 2c–TiO2 composite became much better, and then the polarity of the substituents of porphyrin molecules became the main factor for the photocatalytic efficiency, so 2c–TiO2 showed the highest photocatalytic efficiency finally.
4 Conclusion
Two new copper porphyrin modified TiO2 composites have been prepared and characterized. The XRD and FT-IR spectra demonstrated that CuPps were loaded onto the surface of TiO2 microsphere and did not influence the phase of TiO2. These composites exhibited higher photoactivities compared with the bare TiO2 when they were employed for the photodegradation of 4-nitrophenol in water. Furthermore, it is found that photodegradation efficiency has been evidently influenced by the polarity of substituents of porphyrins in two aspects, one is the interaction with TiO2, and the other is the dispersion of photocatalysts in the solution. The larger the polarity of the substituent group for the porphyrin is, the stronger the interaction with the TiO2 will be, and the higher the photoactivity gets. The strong polarity of porphyrin substituents also makes the dispersion of composites worse, and results in a lower photocatalytic efficiency. But the interaction with TiO2 is the main factor for the photodegradation efficiency.
Acknowledgements
The authors acknowledge the research grant provided by the National Nature Science Foundation of China (Project Nos.20971103) and The International Cooperation Project of Shaanxi province (2008KW-33) that resulted in this article.
References
- P. R. Gogate and A. B. Pandit, Adv. Environ. Res., 2004, 8, 501 CrossRef CAS.
- M. R. Hoffmann, S. T. Martin, W. Y. Choi and D. W. Bahnemann, Chem. Rev., 1995, 95, 69 CrossRef CAS.
- G. Granados-Oliveros, E. A. Páez-Mozo, F. M. Ortega, C. Ferronato and J. M. Chovelon, Appl. Catal., B, 2009, 89, 448 CrossRef CAS.
- G. Mele, R. D. Sole, G. Vasapollo, E. García-López, L. Palmisano and M. J. Schiavello, Catal., 2003, 217, 334 CAS.
- Y. Cho and W. Choi, Environ. Sci. Technol., 2001, 35, 966 CrossRef CAS.
- F. Watson, A. Marton, A. M. Stux and G. J. Meyer, J. Phys. Chem. B, 2004, 108, 11680 CrossRef.
- A. F. Nogueira, L. F. Furtado, A. L. Formiga, M. Nakamura, K. Araki and H. Toma, Inorg. Chem. Commun., 2004, 43, 396 CAS.
- M. Grätzel, J. Photochem. Photobiol., C, 2003, 4, 145 CrossRef.
- M. Y. Duan, J. Li, G. Mele, C. Wang, X. F. Lü and G. Vasapollo, J. Phys. Chem. C, 2010, 114, 7857 CAS.
- A. Molinari, A. Maldotti, A. Bratovcic and G. Magnacca, Catal. Today, 2011, 161, 64 CrossRef CAS.
- N. A. Stephenson and A. T. Bell, J. Mol. Catal. A: Chem., 2007, 275, 54 CrossRef CAS.
- M. Y. Chang, Y. H. Hsieh, T. C. Cheng, K. S. Yao, M. C. Wei and C. Y. Chang, Thin Solid Films, 2009, 517, 3888 CrossRef CAS.
- H. Y. Huang, X. T. Gu, J. H. Zhou, K. Ji, H. L. Liu and Y. Y. Feng, Catal. Commun., 2009, 11, 58 CrossRef CAS.
- T. Ma, K. Inoue, H. Noma, K. Yao and E. Abe, J. Photochem. Photobiol., A, 2002, 152, 207 CrossRef CAS.
- W. M. Campbell, A. K. Burrell, D. L. Officer and K. W. Jolley, Coord. Chem. Rev., 2004, 248, 1363 CrossRef CAS.
-
K. M. Smith, Porphyrins and Metalloporphyrins, Elsevier, New York, 1977 Search PubMed.
- F. D'Souza, G. R. Deviprasad, M. E. El-Khouly, M. Fujitsuka and O. Ito, J. Am. Chem. Soc., 2001, 123, 5277 CrossRef CAS.
- C. Wang, J. Li and G. Mele,
et al.
, Appl. Catal., B, 2007, 76, 218 CrossRef CAS.
- X. F. Lü, J. Li, C. Wang and M. Y. Duan,
et al.
, Appl. Surf. Sci., 2010, 257, 795 CrossRef.
- C. Wang, J. Li and G. Mele,
et al.
, Dyes Pigm., 2010, 84, 183 CrossRef CAS.
|
This journal is © The Royal Society of Chemistry 2012 |
Click here to see how this site uses Cookies. View our privacy policy here.