DOI:
10.1039/C1SC00358E
(Edge Article)
Chem. Sci., 2011,
2, 1897-1901
Template-directed synthesis of π-conjugated porphyrin [2]rotaxanes and a [4]catenane based on a six-porphyrin nanoring†
Received
12th June 2011
, Accepted 2nd July 2011
First published on 28th July 2011
Abstract
[2]Rotaxanes consisting of butadiyne-linked porphyrin dimers threaded through a phenanthroline-containing macrocycle, can be synthesised by an active-metal template directed copper-mediated Glaser coupling, in yields of up to 61%, without requiring a large excess of the macrocycle. The crystal structure of one of these rotaxanes confirms that the macrocycle is clasped around the centre of the porphyrin dimer. A radial hexa-pyridyl template was used to convert the alkyne-terminated [2]rotaxane into a [4]catenane cyclic porphyrin hexamer in 62% yield, viapalladium-catalysed Glaser coupling. The related [7]catenane porphyrin dodecamer complex was also isolated as a by-product of this cyclisation, in 6% yield. These results illustrate the scope of template-directed synthesis for creating complex interlocked structures directly from simple starting materials.
Introduction
Templates have been widely used in the synthesis of large macrocycles,1,2 and topologically complex molecules such as rotaxanes and catenanes.3 The unusual dynamics of these mechanically interlocked molecules leads to potential applications in molecular machines, sensors and switches.4Rotaxane formation also provides a valuable strategy for modifying the properties of long conjugated π-systems, by generating ‘insulated molecular wires’.5 Recently Leigh and co-workers reported an active-metal template strategy for rotaxane synthesis, whereby a metal cation both orientates the individual components and catalyses the formation of a covalent bond to capture the interlocked structure.6 Saito and co-workers reported the synthesis of both rotaxanes and catenanes, from macrocyclic Cu(I) complexes, through a similar active-metal template Glaser coupling.7 We have previously used a hexapyridyl template to direct the synthesis of cyclic porphyrin nanorings;2 these fully π-conjugated nanorings have unusual electronic structures, and are models for the cyclic chlorophyll arrays found in natural photosynthetic light harvesting antenna. Rotaxanes with porphyrin stoppers are also of interest for mimicking the function of photosynthetic reaction centres.8 Here we report the preparation of butadiyne-linked porphyrin dimer rotaxanes by active-metal template-directed synthesis. We combine this methodology with the template-directed synthesis of porphyrin nanorings to achieve an efficient synthesis of a [4]catenane consisting of a fully π-conjugated six-porphyrin nanoring with three mechanically interlocked phenanthroline macrocycles. There are few previous reports of [4]catenanes,9 and the chemistry reported here provides an exceptionally direct route to interlocked architectures.
Results and discussion
We started by investigating the scope for active-metal templated copper-mediated Glaser coupling using the phenyl-terminated porphyrin monomer, P1a to synthesise rotaxane P2a⊂M. This reaction was carried out using the procedure developed by Saito and co-workers,7 by stirring P1a with the phenanthroline macrocycle M (0.75 equiv.), copper(I) iodide (0.50 equiv.), iodine (0.50 equiv.) and potassium carbonate (2.0 equiv.) in 1
:
1 toluene and THF for 5 days at 60 °C. After work-up with potassium cyanide, the desired rotaxane P2a⊂M was isolated in 42% yield, together with the non-interlocked porphyrin dimer thread P2a (30% yield), Scheme 1.
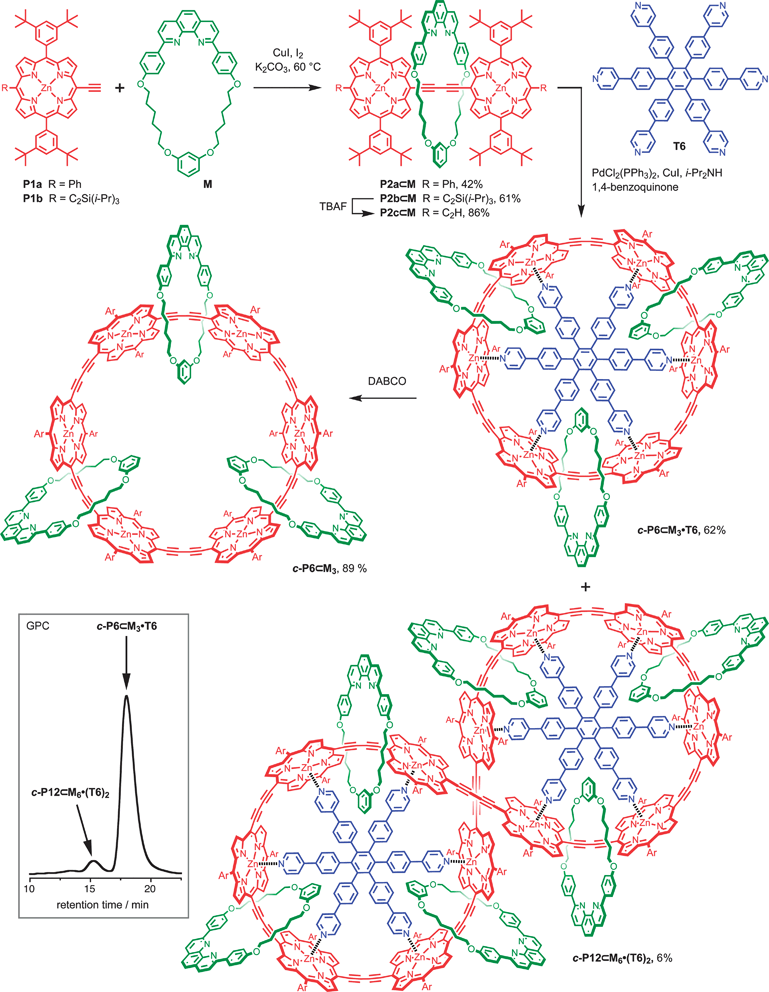 |
| Scheme 1 Synthesis of P2⊂M and cc-P6⊂M33. The insert shows the analytical GPC trace of the crude reaction mixture from the palladium-catalysed coupling of rotaxane P2c⊂M in the presence of template T6 (solvent: toluene; column: PLgel 3 μm mixed-E, 2 × 300 mm, 7.5 mm diameter). | |
Crystals of rotaxane P2a⊂M suitable for X-ray analysis were grown by slow diffusion of methanol into a CHCl3/1% pyridine solution.‡ The asymmetric unit contains one molecule of P2a⊂M, with a molecule of methanol coordinated to each zinc centre, and two molecules of chloroform, as well as other disordered solvent. The solid-state structure of the rotaxane is shown in Fig. 1. The porphyrin dimer is slightly twisted; the angle between the 24-atom planes of the two porphyrins is 27.88(4)°. The porphyrin dimer unit is also slightly nonlinear; the angle between the zinc atoms and the centroid of the two central carbon atoms of the butadiyne is 171.73(7)°. These features contrast with the three previously reported crystal structures of meso-butadiyne-linked porphyrin dimers, all of which have crystallographic inversion centres at the centre of the dimer, so that the porphyrin units are exactly coplanar and colinear.10 The phenanthroline units form tightly packed π-stacks, with a plane-plane distance of 3.47(2) Å, generating dimers of rotaxane units in the crystal.
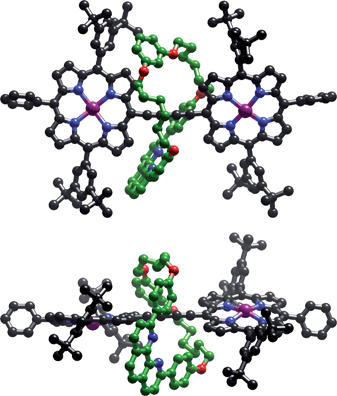 |
| Fig. 1 Two orthogonal views of rotaxane P2a⊂M in the crystal (hydrogen atoms, coordinated methanol molecules and chloroform solvent molecules omitted for clarity). | |
Further evidence for the close proximity of the macrocycle to the centre of the porphyrin dimer is provided by comparison of the 1H NMR spectra of P2a⊂M, P2a and M (Fig. 2). In the rotaxane, up-field shifts are observed for the macrocycle protons Hg, Hh and Hj, and for protons H2 and H5 on the porphyrin, while proton Hf on the macrocycle is strongly de-shielded (Δδ = 0.9 ppm). NOEs are observed between the macrocycle and porphyrinβprotons H1 and H2 on the axle of the rotaxane.
The absorption spectra of P2a⊂M and P2a in chloroform are almost identical in the region 400–750 nm, which indicates that the threaded macrocycle does not substantially change the distribution of porphyrin–porphyrin torsional angles in solution. The rotaxane P2a⊂M exhibits an absorption band at 250–350 nm due to the phenanthroline moiety of the macrocycle. The absorption spectrum of P2a⊂M overlaps well with its excitation spectrum, in both the macrocycle and porphyrin absorption regions (see ESI†). The close proximity of the rotaxane components evidently leads to efficient energy transfer from the phenanthroline to the porphyrin axle.
In order to gain access to a new class of catenanes, we prepared the alkyne-terminated [2]rotaxane P2c⊂M. Coupling of the TIPS-protected porphyrin P1b in the presence of macrocycle M using the reaction conditions described above gave rotaxane P2b⊂M in 61% yield, together with a 7% yield of the unthreaded dimer P2b. Deprotection of the TIPS-protected acetylenes afforded rotaxane P2c⊂M. Palladium catalysed oxidative coupling of P2c⊂M in the presence of template T6 (0.33 equiv.)2b gave [4]catenane cc-P6⊂M33·T6 in 62% (Scheme 1). Molecular mechanics calculations indicate that this catenane has a very compact structure (Fig. 3), but the high yield achieved in this synthesis shows that the threaded phenanthroline macrocycles do not hinder cyclisation. The 2D NMR spectra of P6⊂M33·T6 reveal similar macrocycle–porphyrin NOEs to those observed in the rotaxanes, as well as additional NOEs between the macrocycle and template T6 (see ESI†). The catenane-template complex remains intact under MALDI mass spectrometry and an excellent match with the theoretical mass and isotope pattern is obtained (see ESI†). The equilibrium constant for formation of cc-P6⊂M33·T6 is too high to measure directly, but could be determined by analysing the thermodynamics of template displacement with quinuclidine. The threaded macrocycles do not significantly change the stability of the complex. The UV-vis titration showed simple isosbestic behaviour and the binding isotherms fit the calculated curve with a displacement constant of Kb = 5.2 × 10−2M−5 corresponding to logKf = 38 ± 1, which is similar to the stability constant of cc-P6·T6.2b The free [4]catenane cc-P6⊂M33 was prepared from cc-P6⊂M33·T6 by addition of a large excess of the competing ligand DABCO (1,4-diazabicyclo[2.2.2]octane) and isolated in 89% yield.
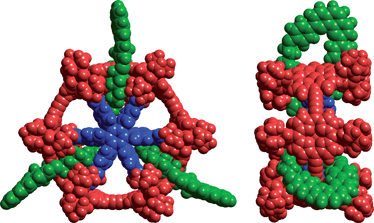 |
| Fig. 3 Calculated structure of cc-P6⊂M33·T6 (MM+ force field). | |
The 12-porphyrin “figure-of-eight” [7]catenane complex cc-P12⊂M66·(T6)22 was also isolated as a by-product (6% yield) during the synthesis of cc-P6⊂M33·T6. 1H NMR spectroscopy, MALDI-TOF MS, GPC molecular weight determination and UV-vis absorption spectroscopy all confirm the identity of this [7]catenane (see ESI†). Its 1H NMR spectrum is consistent with the expected D2 symmetry and closely resembles the spectrum of the analogous non-catenated complex cc-P12⊂(T6)22.2c A partial assignment of the spectrum was achieved by reference to this compound and by using 2D NMR techniques.
Conclusion
In summary, we have shown that active-metal templates can be used to prepare conjugated porphyrin dimer [2]rotaxanes in good yield, and that radial templates can be used to convert these rotaxanes into large π-conjugated catenanes. There are very few previous reports of catenanes based on fully π-conjugated macrocycles.11 This work illustrates the scope of template-directed synthesis for creating complex interlocked structures in a controlled manner from simple starting materials, particularly using Glaser coupling.12Porphyrin-based molecular wires exhibit remarkable properties such as wire-like charge transport,13 ultrafast energy migration,14 strong two-photon absorption15 and bright near-infrared luminescence.16 They are also promising chromophores for photodynamic therapy.17 The possibility to encapsulate these π-systems by rotaxane and catenane formation may provide valuable control over their properties, as it offers a unique approach to engineering intermolecular interactions. This chemistry may also be useful for modifying the reactivity of carbon-rich materials, such as carbyne, through rotaxane and catenane formation.18
Acknowledgements
This work has been supported in part by the DARPA ZOE program. We thank the EPSRC Mass Spectrometry Service (Swansea) for mass spectra, D. V. Kondratuk and M. C. O'Sullivan for valuable advice and B. Odell for assistance with NMR spectroscopy.
Notes and references
-
(a) S. Anderson, H. L. Anderson and J. K. M. Sanders, Acc. Chem. Res., 1993, 26, 469–475 CrossRef CAS;
(b) S. Anderson, H. L. Anderson and J. K. M. Sanders, J. Chem. Soc., Perkin Trans. 1, 1995, 2255–2267 RSC;
(c) S. Rucareanu, A. Schuwey and A. Gossauer, J. Am. Chem. Soc., 2006, 128, 3396–3413 CrossRef CAS;
(d) J. Li, A. Ambroise, S. I. Yang, J. R. Diers, J. Seth, C. R. Wack, D. F. Bocian, D. Holten and J. S. Lindsey, J. Am. Chem. Soc., 1999, 121, 8927–8940 CrossRef CAS.
-
(a) M. Hoffmann, C. J. Wilson, B. Odell and H. L. Anderson, Angew. Chem., Int. Ed., 2007, 46, 3122–3125 CrossRef CAS;
(b) M. Hoffmann, J. Kärnbratt, M.-H. Chang, L. M. Herz, B. Albinsson and H. L. Anderson, Angew. Chem., Int. Ed., 2008, 47, 4993–4996 CrossRef CAS;
(c) M. C. O'Sullivan, J. K. Sprafke, D. V. Kondratuk, C. Rinfray, T. D. W. Claridge, A. Saywell, M. O. Blunt, J. N. O'Shea, P. H. Beton, M. Malfois and H. L. Anderson, Nature, 2011, 469, 72–75 CrossRef CAS.
-
(a) C. O. Dietrich-Buchecker, J.-P. Sauvage and J. P. Kintzinger, Tetrahedron Lett., 1983, 24, 5095–5098 CrossRef CAS;
(b) C. A. Hunter, J. Am. Chem. Soc., 1992, 114, 5303–5311 CrossRef CAS;
(c) D. B. Amabilino and J. F. Stoddart, Chem. Rev., 1995, 95, 2725–2828 CrossRef CAS;
(d)
Molecular Catenanes, Rotaxanes and Knots, ed. J.-P. Sauvage and C. O. Dietrich-Buchecker, Wiley-VCH., Weinheim, 1999 Search PubMed;
(e) K. S. Chichak, S. J. Cantrill, A. R. Pease, S.-H. Chiu, G. W. V. Cave, J. L. Atwood and J. F. Stoddart, Science, 2004, 304, 1308–1312 CrossRef CAS;
(f) M. S. Vickers and P. D. Beer, Chem. Soc. Rev., 2007, 36, 211–225 RSC;
(g) H. Li, A. C. Fahrenbach, S. K. Dey, S. Basu, A. Trabolsi, Z. Zhu, Y. Y. Botros and J. F. Stoddart, Angew. Chem., Int. Ed., 2010, 49, 8260–8265 CrossRef CAS.
-
(a) P. Gaviña and J.-P. Sauvage, Tetrahedron Lett., 1997, 38, 3521–3524 CrossRef;
(b) V. Balzani, A. Credi, S. Silvi and M. Venturi, Chem. Soc. Rev., 2006, 35, 1135–1149 RSC;
(c) H. Tian and Q.-C. Wang, Chem. Soc. Rev., 2006, 35, 361–374 RSC;
(d) W. R. Browne and B. L. Feringa, Nat. Nanotechnol., 2006, 1, 25–35 CrossRef CAS;
(e) S. Saha and J. F. Stoddart, Chem. Soc. Rev., 2007, 36, 77–92 RSC;
(f) E. R. Kay, D. A. Leigh and F. Zerbetto, Angew. Chem., Int. Ed., 2007, 46, 72–191 CrossRef CAS.
- M. J. Frampton and H. L. Anderson, Angew. Chem., Int. Ed., 2007, 46, 1028–1064 CrossRef CAS.
-
(a) V. Aucagne, K. D. Hänni, D. A. Leigh, P. J. Lusby and D. B. Walker, J. Am. Chem. Soc., 2006, 128, 2186–2187 CrossRef CAS;
(b) J. Berná, J. D. Crowley, S. M. Goldup, K. D. Hänni, A.-L. Lee and D. A. Leigh, Angew. Chem., Int. Ed., 2007, 46, 5709–5713 CrossRef;
(c) J. D. Crowley, S. M. Goldup, A.-L. Lee, D. A. Leigh and R. T. McBurney, Chem. Soc. Rev., 2009, 38, 1530–1541 RSC;
(d) J. D. Crowley, S. M. Goldup, N. D. Gowans, D. A. Leigh, V. E. Ronaldson and A. M. Z. Slawin, J. Am. Chem. Soc., 2010, 132, 6243–6248 CrossRef CAS.
-
(a) S. Saito, E. Takahashi and K. Nakazono, Org. Lett., 2006, 8, 5133–5136 CrossRef CAS;
(b) Y. Sato, R. Yamasaki and S. Saito, Angew. Chem., Int. Ed., 2009, 48, 504–507 CrossRef CAS.
-
(a) J. A. Faiz, V. Heitz and J.-P. Sauvage, Chem. Soc. Rev., 2009, 38, 422–442 RSC;
(b) M.-J. Blanco, M. Consuelo Jiménez, J.-C. Chambron, V. Heitz, M. Linke and J.-P. Sauvage, Chem. Soc. Rev., 1999, 28, 293–305 RSC.
-
(a) F. Bitsch, C. O. Dietrich-Buchecker, A.-K. Khemiss, J.-P. Sauvage and A. Van Dorsselaer, J. Am. Chem. Soc., 1991, 113, 4023–4025 CrossRef CAS;
(b) D. B. Amabilino, P. R. Ashton, A. S. Reder, N. Spencer and J. F. Stoddart, Angew. Chem., Int. Ed. Engl., 1994, 33, 433–437 CrossRef.
-
(a) P. N. Taylor, J. Huuskonen, G. Rumbles, R. T. Aplin, E. Williams and H. L. Anderson, Chem. Commun., 1998, 909–910 RSC;
(b) Y.-J. Chen, S.-S. Chen, S.-S. Lo, T.-H. Huang, C.-C. Wu, G.-H. Lee, S.-M. Peng and C.-Y. Yeh, Chem. Commun., 2006, 1015–1017 RSC;
(c) A. Berlicka, L. Latos-Graźyński and T. Lis, Inorg. Chem., 2005, 44, 4522–4533 CrossRef CAS.
- P. Bäuerle, M. Ammann, M. Wilde, G. Götz, E. Mena-Osteritz, A. Rang and C. A. Schalley, Angew. Chem., Int. Ed., 2007, 46, 363–368 CrossRef.
- P. Siemsen, R. C. Livingston and F. Diederich, Angew. Chem., Int. Ed., 2000, 39, 2632–2657 CrossRef CAS.
-
(a) K. Susumu, P. R. Frail, P. J. Angiolillo and M. J. Therien, J. Am. Chem. Soc., 2006, 128, 8380–8381 CrossRef CAS;
(b) G. Sedghi, K. Sawada, L. J. Esdaile, M. Hoffmann, H. L. Anderson, D. Bethell, W. Haiss, S. J. Higgins and R. J. Nichols, J. Am. Chem. Soc., 2008, 130, 8582–8583 CrossRef CAS.
- M.-H. Chang, M. Hoffmann, H. L. Anderson and L. M. Herz, J. Am. Chem. Soc., 2008, 130, 10171–10178 CrossRef CAS.
- M. Drobizhev, Y. Stepanenko, Y. Dzenis, A. Karotki, A. Rebane, P. N. Taylor and H. L. Anderson, J. Phys. Chem. B, 2005, 109, 7223–7236 CrossRef CAS.
-
(a) T. V. Duncan, K. Susumu, L. E. Sinks and M. J. Therien, J. Am. Chem. Soc., 2006, 128, 9000–9001 CrossRef CAS;
(b) O. Fenwick, J. K. Sprafke, J. Binas, D. V. Kondratuk, F. Di Stasio, H. L. Anderson and F. Cacialli, Nano Lett., 2011, 11, 2451–2456 CrossRef CAS.
-
(a) H. A. Collins, M. Khurana, E. H. Moriyama, A. Mariampillai, E. Dahlstedt, M. Balaz, M. K. Kuimova, M. Drobizhev, V. X. D. Yang, D. Phillips, A. Rebane, B. C. Wilson and H. L. Anderson, Nat. Photonics, 2008, 2, 420–424 CrossRef CAS;
(b) M. Balaz, H. A. Collins, E. Dahlstedt and H. L. Anderson, Org. Biomol. Chem., 2009, 7, 874–888 RSC;
(c) K. Ogawa and K. Kobuke, Org. Biomol. Chem., 2009, 7, 2241–2246 RSC.
-
(a) F. Diederich, Nature, 1994, 369, 199–207 CrossRef CAS;
(b) S. M. E. Simkins, B. M. Kariki and L. R. Cox, J. Organomet. Chem., 2006, 691, 5517–5523 CrossRef;
(c) W. A. Chalifoux and R. R. Tykwinski, Nat. Chem., 2010, 2, 967–971 CrossRef CAS.
- J. Cosier and A. M. Glazer, J. Appl. Crystallogr., 1986, 19, 105–107 CrossRef CAS.
- L. Palatinus and G. Chapuis, J. Appl. Crystallogr., 2007, 40, 786–790 CrossRef CAS.
- P. W. Betteridge, J. R. Carruthers, R. I. Cooper, K. Prout and D. J. Watkin, J. Appl. Crystallogr., 2003, 36, 1487 CrossRef CAS.
- A. Spek, J. Appl. Crystallogr., 2003, 36, 7–13 CrossRef CAS.
- P. van der Sluis and A. L. Spek, Acta Crystallogr., Sect. A: Found. Crystallogr., 1990, 46, 194–201 CrossRef.
- R. I. Cooper, A. L. Thompson and D. J. Watkin, J. Appl. Crystallogr., 2010, 43, 1100–1107 CrossRef CAS.
Footnotes |
† Electronic supplementary information (ESI) available. CCDC reference number 829502. For ESI and crystallographic data in CIF or other electronic format see DOI: 10.1039/c1sc00358e |
‡ Diffraction data for a typical dark green metallic crystal of the rotaxane P2a⊂M were collected at 100 K19 using an Oxford Diffraction (Agilent) SuperNova (λ = 1.54180 Å). Cell parameters were determined and refined and raw frame data were integrated using CrysAlisPro. The structure was solved with SuperFlip20 and refined by full-matrix least squares on F2 using CRYSTALS.21 All non-hydrogen atoms were refined with anisotropic displacement parameters; geometric restraints were required for the disordered components and thermal restraints were necessary to maintain sensible ADPs; diffuse disordered solvent was modelled by treating the discrete Fourier transform of the void region as contributions to the A and B parts of the calculated structure factors with PLATON/SQUEEZE;22hydrogen atoms were generally visible in the difference map and treated in the usual manner;24 interplane dihedral angle calculated with PLATON.22,23 See the SI/CIF for full refinement details; crystallographic data (excluding structure factors) have been deposited with the Cambridge Crystallographic Data Centre (CCDC 829502) and can be obtained viahttp://www.ccdc.cam.ac.uk/data_request/cif. Single Crystal Data: C158H164Cl6N10O6Zn2, Mr = 2640.57, triclinic, P , a = 19.7916(3) Å, b = 20.7295(3) Å, c = 22.2512(3) Å, α = 90.5711(12)°, β = 99.9660(13)°, γ = 117.6227(15)°, V = 7923.7(2) Å, data/restraints/parameters: 33183/7392/2102, Rint = 0.094, final R1 = 0.1145, wR2 = 0.3360 (I > 2σ(I)), Δρmin,max = −1.52,+3.03 e.Å3. |
|
This journal is © The Royal Society of Chemistry 2011 |
Click here to see how this site uses Cookies. View our privacy policy here.