DOI:
10.1039/C1RA00313E
(Paper)
RSC Adv., 2011,
1, 1045-1049
Fluorescent probe for Fe3+ and CN− in aqueous media mimicking a memorized molecular crossword puzzle†
Received
16th June 2011
, Accepted 31st July 2011
First published on 16th September 2011
Abstract
A fluorescent probe 4 possessing dansyl group showed “On-Off” selectivity toward Fe3+ ions and ratiometric selectivity toward CN− ions in mixed aqueous media, which mimics a sequence dependent molecular crossword puzzle with optical memory feature (in feedback loop).
Introduction
The trivalent form of iron, an essential element in human body,1 plays a significant role in many biological processes and is crucial for cellular metabolism such as oxygen metabolism, electron-transfer processes and DNA and RNA synthesis.2 The deficiency and excess of iron causes imbalance in iron transport and its storage which leads to various pathological disorders, organ malfunction and mortality.3 On the other hand, CN− ion is among the most toxic inorganic anions.4 It strongly inactivates the active site of cytochrome a3 in mitochondria and suppresses the transport of oxygen in mammalian cells,5 as a result even its small amount is very poisonous to human body. Despite its acute toxicity, cyanide or hydrogen cyanide are used in large number of applications like synthetic fibres and resins, herbicides, electroplating and gold extraction.6 Thus, the diversity of their functions, both beneficial and otherwise, makes the detection of iron and cyanide ions important. Recently, a few reports about the fluorogenic sensing of Fe3+ ions7 and ratiometric sensing of CN− ions8f–h in aqueous/mixed aqueous media have appeared in literature but none of these reports sought to use these sensors for the development of molecular photonic logic gates and devices which are an alternative to conventional silicon based devices which have limitation of miniaturization.9 Numerous chemical systems have been mimicked into various molecular level devices such as identification tags for small objects,10memory units11 and molecular keypad locks12 performing digital operations. Another electronic device which is less developed at molecular level is “molecular crossword puzzles”. The output signals of molecular crossword puzzle are dependent not only upon the appropriate combination of chemical inputs but also upon the exact sequence of these inputs. Tian et al.13 developed a molecular crossword puzzle by using appropriate combination of Cu2+ and Hg2+ ions as chemical inputs.
Thiacalix[4]arene
14 possessing four bridging sulfur atoms has superior binding properties toward transition metal ions than conventional calix[4]arene. Thus, there is much more potential in investigating the chemistry of thiacalix[4]arenes toward transition metal ions as artificial receptors, enzyme mimics or molecular level devices. Our research programme involves design, synthesis and evaluation of artificial receptors for soft metal ions15 and anions16 based on (thia)calix[4]arene. Recently, in a preliminary communication, we reported a fluorescent chemosensor based on thiacalix[4]arene of 1,3-alternate conformation which behaves as an “On–Off” reversible switch for two chemical inputs Hg2+ and K+ ions and mimics a molecular level keypad lock in the presence of F− ions.15b In continuation of this work, in the present manuscript, we have designed and synthesized dansyl-appended chemosensor 4 based on thiacalix[4]arene of 1,3-alternate conformation which shows high selectivity towards Fe3+ with quenching in fluorescence emission and CN− ions with ratiometric behaviour in mixed aqueous media. The chemosensor 4 shows high selectivity for Fe3+ and CN− ions with a detection limit17 of 5 × 10−7 mol L−1 and 2.5 × 10−6 mol L−1, respectively which is sufficiently low for the detection of a submillimolar concentration range of Fe3+ and CN− ions found in many chemical systems. The sequential addition of Fe3+ and CN− ions mimics the molecular crossword puzzle in which the output signals are dependent not only upon the appropriate combination of chemical inputs but also upon the exact sequence of these inputs.
Results and discussion
Synthesis of probe 4
The reaction of compound 115a with hydrazine monohydrate in dry tetrahydrofuran furnished thiacalix[4]arene-1,3-diamine 2 in 92% yield (Scheme 1). The condensation of 2 with 1.0 mol equiv of dansyl chloride 3 in dry dichloromethane in the presence of Et3N furnished 4 (45%) (Scheme 1). The structures of compounds 2 and 4 were confirmed from their spectroscopic and analytical data.
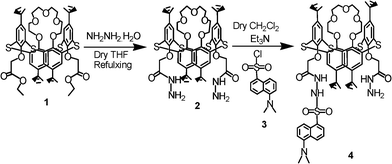 |
| Scheme 1 Synthesis of compound 4. | |
Binding studies
The binding behaviour of compound 4 was studied toward different cations and anions by fluorescence and 1H NMR spectroscopy. In the fluorescence spectrum, compound 4 shows high fluorescence emission band at 531 nm when excited at 338 nm (Fig. 1). Among all the metal ions studied, compound 4 showed high selectivity for Fe3+ ions. Upon addition of increasing amounts of Fe3+ ion as its perchlorate salt (0–150 equiv) to the solution of compound 4, there was significant quenching with small blue shift in fluorescence emission which is attributed to the 4.Fe3+ complex (Fig. 1). This remarkable quenching induced by Fe3+ ions is ascribed to reverse PET from dansyl moieties to oxygen atoms of which the electron density was diminished upon metal complexation (Supporting Information, Fig. S7)†. Under the same conditions as used above for Fe3+, we also tested the fluorescence response of receptor 4 to other metal ions such as Li+, Na+, K+, Ba2+, Mg2+, Ni2+, Cu2+, Zn2+, Cd2+, Ag+, Pb2+, Co2+, Hg2+, Fe2+ besides Fe3+, it was observed that no significant fluorescence change of 4 occurred in the presence of these metal ions (300 equiv) (Supporting Information, Fig. S8)†. Fitting the changes in fluorescence spectra of 4 with Fe3+ ions using SPECFIT18 program gave a best fit and demostrated that a 1
:
1 stoichiometry of the host and guest was the most stable species in the solution with a binding constant (logβ11) of 4.36 ± 0.02.
In addition to cation binding properties, we also investigated the sensing properties of 4 toward different anions such as such as F−, Cl−, Br−, I−, CN−, CH3COO−, HSO4−, NO3−, and H2PO4− using tetrabutylammonium as counter cation in 10% aqueous ethanol as amide moiety are known to interact with anions.15b Among all the anions tested, compound 4 showed high selectivity towards CN− ions. Upon addition of increasing amounts of CN− ion (0–100 equiv) to the solution of compound 4 in 10% aqueous ethanol, a significant decrease in fluorescence emission at 531 nm with the formation of a new blue shifted band centred at 460 nm, was observed (Fig. 2). The relative intensity ratio of emission at 460 nm to 531 nm (I460/I531) of free ligand was 0.43 and it increases by 8 fold to 3.46 with addition of 100 equiv of CN− ions in 10% aqueous ethanol (Supporting Information, Fig. S10)†. The ratiometric response of 4 on addition of cyanide ions in 10% aqueous ethanol is due to the deprotonation of the sulphonamide group (SO2NH) (Supporting Information, Scheme S1)†. Similar results were obtained when strong base such as tetrabutylammonium hydroxide was specifically employed. The intermolecular interactions of receptor 4 with cyanide ions were evaluated by 1H NMR spectroscopy. It was found that on addition of 0.5 equiv of cyanide to a solution of compound 4 in CDCl3, the weakening of signal due to SO2NH proton takes place, but there is no change in the chemical shift of SO2NH proton (Supporting Information, Fig. S11)†. With further addition of 0.5 equiv of cyanide, the SO2NH proton completely disappeared (Supporting Information, Fig. S11)†, which indicates that the deprotonation of the SO2NH proton is taking place in the presence of cyanide ions. The deprotonated species 4A (Supporting Information, Scheme S1)† of compound 4 probably gets solvated by the protic solvent (10% aqueous ethanol) which probably decreases the energy of HOMO and hence increases the HOMO–LUMO energy gap in deprotonated species and results in the formation of blue shifted band at 460 nm. Under the same conditions as used above for CN−, we also tested the fluorescence response of receptor 4 to other anions such as F−, Cl−, Br−, I−, CH3COO−, HSO4−, NO3−, and H2PO4− besides CN−, it was observed that no significant change in the fluorescence behaviour of 4 occurred in the presence of these anions (100 equiv) (Supporting Information, Fig. S10)†.
Since the compound 4 shows optical sensing toward Fe3+ and CN− ions by different modes, we investigated the “On-Off” switching process and sequence dependence of emission output between these chemical inputs. In the first sequence, Fe3+ was added first to the solution of compound 4 which results in fluorescence quenching i.e. output signal is “0” for its “off state” and sequential addition of CN− ions (300 equiv) lead to formation of blue shifted emission band at 460 nm i.e. output signal is “1” for its “on state” (Fig. 3A). The addition of CN− ions (0–200 equiv) initially remove the Fe3+ from the 4.Fe3+ complex and further addition of CN− ions results in blue shifted band at 460 nm which is attributed to the 4A species. Further addition of Fe3+ ions to the above solution results in fluorescence quenching due to complexation of CN− ions with Fe3+ ions and the equilibrium shifts toward the 4.Fe3+ complex. On the other hand, in the reverse input sequence, the addition of 100 equiv of CN− ions forms a blue shifted emission band at 460 nm i.e. output signal is “1” for its “on state” of receptor 4 (Fig. 3B) which is due to formation of the 4A species. The sequential addition of Fe3+ ion leads to strong complexation between negatively charged nitrogen and Fe3+ ion which results in complete quenching i.e. output signal is “0” for its “off state” (Fig. 3B).
The “On-Off” switching behaviour of receptor 4 can be demonstrated with the help of binary logic. The two chemical inputs of Fe3+ and CN− are designated as InF and InC respectively and considered as “1” when they are present and “0” if they are absent. The output signals were measured as the fluorescence emissions at 460 nm and 531 nm of the receptor 4, being “1” when fluorescence emission is high and “0” when fluorescence emission is low. For the first input sequence, the addition of InF (Fe3+) followed by InC (CN−) leads to fluorescence emission at 460 nm and output was “1” (On state, Table 1). As shown in the sequential logic circuit constructed (Fig. 4), the output at 460 nm gets “on” only when it acts as an input of OR gate together with InF. This output signal is memorized by the logic circuit so that complete signal transduction take place. On reversal of input sequence, the addition of InC (CN−) followed by InF (Fe3+) gave quenched fluorescence emission at 460 nm and output was “0” (Off state) mimicking INHIBIT gate demonstrating the inhibition of fluorescence emission (Supporting Information, Fig. S12)†.
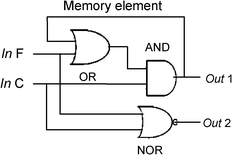 |
| Fig. 4 Sequential logic circuit which displays the memory unit for the first sequence with two input strings InF and InC at λ 460 nm and 531 nm of molecular crossword puzzle. | |
Table 1 Truth table for the first sequence (addition of first input InF followed by second input InC)
InF (Fe3+) |
InC (CN−) |
Output 1 (460 nm) |
Output 2 (531 nm) |
0 |
0 |
0 |
1 |
1 |
0 |
0 |
0 |
0 |
1 |
1 |
0 |
1 |
1 |
1 |
0 |
The contrasting mode selectivity of receptor 4 towards Fe3+ and CN− ions helps us to develop the sequence dependent logic circuit capable of memory functions. The receptor 4 acts as a molecular crossword puzzle with different sequences of two chemical inputs (Fe3+ and CN− ions). Fig. 5 describes a molecular crossword puzzle that gets “solved” by inserting the right sequence of two input keys Fe3+ and CN− ion. The inputs Fe3+ and CN− ion are designated as “A” and “S”, respectively. The output signals are designated as “K” (strong fluorescence emission at 460 nm, On-state) and “D” (quenched fluorescence emission at 460 nm, Off-state). The first input sequence, “A” followed by “S” results in strong fluorescence emission at 460 nm (On state) with TRUE output signal “1” and the puzzle gets solved with code “ASK”. The other sequence “S” followed by “A” was unable to solve the puzzle as there is “Off” fluorescence emission at 460 nm. This sequence with wrong code “SAD” fails to solve the crossword puzzle. Thus, an exact sequence would be possible by assigning the correct order of input signals so that only one sequence would solve the crossword puzzle.
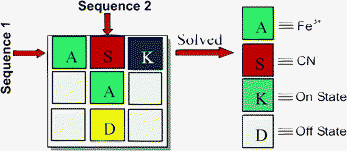 |
| Fig. 5 Sequence dependent crossword puzzle that solved with “ASK” code. | |
Conclusions
In conclusion, a fluorescent probe 4 showed high selectivity towards Fe3+ and CN− ions in contrasting modes which mimics a molecular crossword puzzle with optical memory feature.
Experimental
General information
The 1H and 13C NMR spectra were recorded on JEOL 300 MHz spectrometer using TMS as internal standard and CDCl3 as solvent. FAB Mass spectra were recorded on JEOL XS102/DA-6000 mass spectrometer using xenon (6 kV, 10mA) as FAB gas. Infrared spectra were recorded on a Pye Unicam SP3-3 Infrared spectrophotometer. The UV/vis and fluorescence spectra were recorded with Shimadzu UV-2450 spectrophotometer and CARY-varian 100 spectrofluorophotometer, respectively. Stock solutions (0.1M) of metal perchlorate salts and tetrabutylammonium anion salts were prepared in ethanol:H2O (9
:
1, v/v). Stock solution (0.1mM) of compound 4 was prepared in THF:ethanol (9.5
:
0.5, v/v). The working solution (5μM) of compound 4 was prepared from stock solution in ethanol (containing 5% THF) and H2O (9
:
1, v/v). For all measurements of fluorescence spectra, excitation wavelength was at 344 nm and slit width at 10 nm. UV/vis and fluorescence spectra were performed by using 5.0 μM of compound 4 in ethanol:water:THF (8.85
:
1
:
0.15, v/v) and varying the concentration of guests (cations and anions).
Synthesis of compound 2.
The solution of compound 1 (300 mg, 0.28 mmol) and hydrazine monohydrate (143 mg, 2.84 mmol) in tetrahydrofuran (20 ml) was refluxed for 24h. After completion of reaction (TLC), the solvent was evaporated under vacuo. The crude product was dissolved in CH2Cl2 (50 ml), washed thrice with water (50 ml), dried over anhydrous sodium sulphate and distilled under reduced pressure to give crude product which was recrystallized from dichloromethane and methanol (1
:
1, v/v) to give 270 mg (92.4%) of compound 2 as white solid. m. p. > 240 °C, IR (KBr pallet, cm−1) 1668, 3452; 1H NMR (300 MHz, CDCl3) δ (ppm): 1.25 (18H, s, C(CH3)3 x 2), 1.37 (18H, s, C(CH3)3 x 2), 3.00 (4H, t, J= 8.4 Hz, OCH2 x 2), 3.39 (4H, t, J= 3.9 Hz, OCH2 x 2), 3.48 (4H, d, J= 4.2 Hz, NCH2 x 2), 3.62 (4H, t, J= 3.0 Hz, OCH2 x 2), 3.96 (4H, t, J= 9.0 Hz, OCH2 x 2), 4.56 (4H, s, OCH2 x 2), 7.35 (4H, s, ArH x 4), 7.41 (4H, s, ArH x 4), 7.55 (2H, s, NH x 2); 13C NMR (75 MHz, CDCl3) δ (ppm): 31.3 (CH3)3, 34.4 (C(CH3)3), 65.5 (OCH2), 68.2 (OCH2), 70.0 (OCH2), 71.4 (OCH2), 73.5 (OCH2), 127.0 (ArC), 127.2 (ArC), 127.3 (ArC), 127.5 (ArC), 147.4 (ArC), 147.6 (ArC), 154.4 (ArC), 156.2 (ArC), 168.5 (CO); FAB-MS: m/z 1024 M+; Anal. Calcd for C52H70N4O9S4: C, 61.03; H, 6.89, N, 5.47, S, 12.53, Founds: C, 60.89; H, 6.56, N, 5.31, S, 12.27.
Synthesis of compound 4.
Dansyl chloride
3 (13.2 mg, 0.048 mmol) was added dropwise to a solution of thiacalix[4]arene-1,3-diamine 2 (50 mg, 0.048 mmol) and Et3N (12.3 mg, 0.048 mmol) in dry CH2Cl2 (30 ml). The reaction was stirred at room temperature for 12 h. After completion of reaction (TLC), the reaction mixture was diluted with CH2Cl2 (50 ml), washed thrice with water (50 ml), dried over anhydrous sodium sulphate and distilled under reduced pressure to give crude product which was recrystallized from dichloromethane and methanol (1
:
1, v/v) to give 33 mg (45%) of compound 4 as a light yellow solid. m. p. 158–160 °C, IR (KBr pallet, cm−1) 1665, 1H NMR (300 MHz, CDCl3) δ (ppm): 1.23 (18H, s, C(CH3)3 x 2), 1.34 (9H, s, C(CH3)3), 1.38 (9H, s, C(CH3)3), 2.86 (6H, s, NCH3 x 2), 3.04–3.11 (4H, m, OCH2 x 2), 3.30 (2H, br, OCH2), 3.43–3.52 (4H, m, OCH2 x 2), 3.66 (2H, br, OCH2), 3.85–3.96 (4H, m, OCH2 x 2), 4.29 (2H, s, OCH2), 4.31 (2H, s, OCH2), 4.86 (2H, br, NH2), 5.69 (1H, s, NH), 7.09 (1H, d, J= 7.5 Hz, ArH), 7.24 (4H, s, ArH x 4), 7.31–7.45 (2H, m, ArH x 2), 7.53 (4H, s, ArH x 4), 8.15 (1H, d, J= 6.0 Hz, ArH), 8.43 (1H, d, J= 8.7 Hz, ArH), 8.55 (1H, d, J= 8.1 Hz, ArH), 10.72 (1H, s, NH). 13C NMR (75 MHz, CDCl3) δ (ppm): 31.2 (CH3)3, 31.3 (CH3)3, 31.4 (CH3)3, 34.3 (C(CH3)3), 34.62 (C(CH3)3), 34.65 (C(CH3)3), 66.1 (OCH2), 69.9 (OCH2), 71.4 (OCH2), 73.4 (OCH2), 96.1 N(CH3)3, 123.7 (ArC), 126.6 (ArC), 127.0 (ArC), 127.1 (ArC), 127.7 (ArC), 127.8 (ArC), 127.9 (ArC), 148.3 (ArC), 149.1 (ArC), 150.4 (ArC), 152.3 (ArC), 155.6 (ArC), 158.3 (ArC), 161.6 (CO); FAB-MS: m/z 1257 M+; Anal. Calcd for C64H81N5O11S5: C, 61.17; H, 6.50 N, 5.57, S, 12.76, Founds: C, 60.83; H, 6.27 N, 5.39, S, 12.43.
Acknowledgements
We are thankful to CSIR [01(2326)/09/EMR-II], UGC [33-276/2007(SR)] and DST [SR/FT/CS/10-2006] New Delhi for financial support and Guru Nanak Dev University for the laboratory facilities.
References
- R. Meneghini, Iron homeostasis, oxidative stress, and DNA damage, Free Radical Biol. Med., 1997, 23, 783 CrossRef CAS.
-
B. F. Matzanke, G. Muller-Matzanke and K. N. Raymond, Iron Carriers and Iron Proteins; VCH Publishers: New York, 1989, 5 Search PubMed.
-
Iron and Human Disease; R. B. Lauffer, Ed.; CRC Press: Boca Raton, FL, 1992.
-
K. W. Kulig, Cyanide Toxicity; U.S. Department of Health and Human Services: Atlanta, GA, 1991 Search PubMed.
-
(a)
Cyanide in Biology; B. Vennesland, E. F. Comm, J. Knownles, J. Westly, F. Wissing, ed.; Academic: London, 1981 Search PubMed;
(b) A. E. Lindasy, A. R. Greenbaum and D. O’Hare, Anal. Chim. Acta, 2004, 511, 185 CrossRef.
-
G. C. Miller, A. Pritsos, Cyanide: Social, Industrial and Economic Aspects. Proceeding of the TMS Annual Meeting, 2001; p. p 73 Search PubMed.
-
(a) B. Wang, J. Hai, Z. Liu, Q. Wang, Z. Yang and S. Sun, Angew. Chem., Int. Ed., 2010, 49, 4576 CrossRef CAS;
(b) Y. Xiang and J. A. Tong, Org. Lett., 2006, 8, 1549 CrossRef CAS;
(c) J. L. Bricks, A. Kovalchuk, C. Trieflinger, M. Nofz, M. Buschel, A. I. Tolmachev, J. Daub and K. Rurack, J. Am. Chem. Soc., 2005, 127, 13522 CrossRef CAS;
(d) L. J. Fan and W. E. Jones, J. Am. Chem. Soc., 2006, 128, 6784 CrossRef CAS;
(e) J. Mao, L. N. Wang, W. Dou, X. L. Tang, Y. Yan and W. S. Liu, Org. Lett., 2007, 9, 4567 CrossRef CAS;
(f) C. R. Lohani and K. H. Lee, Sens. Actuators, B, 2010, 143, 649 CrossRef.
-
(a) Z. Xu, X. Chen, H. N. Kim and J. Yoon, Chem. Soc. Rev., 2010, 39, 127 RSC;
(b) H. J. Kim, K. C. Ko, J. H. Lee, J. Y. Lee and J. S. Kim, Chem. Commun., 2011, 47, 2886 RSC;
(c) X. Chen, S.-W. Nam, G.-H. Kim, N. Song, Y. Jeong, I. Shin, S. K. Kim, J. Kim, S. Park and J. Yoon, Chem. Commun., 2010, 46, 8953 RSC;
(d) C.-Y. Liu and W.-L. Tseng, Chem. Commun., 2011, 47, 2550 RSC;
(e) S.-Y. Chung, S.-W. Nam, J. Lim, S. Park and J. Yoon, Chem. Commun., 2009, 2866 Search PubMed;
(f) S. Saha, A. Ghosh, P. Mahato, S. Mishra, S. K. Mishra, E. Suresh, S. Das and A. Das, Org. Lett., 2010, 12, 3406 CrossRef CAS;
(g) G. Qian, X. Li and Z. Y. Wang, J. Mater. Chem., 2009, 19, 522 RSC;
(h) X. Lou; L. Zhang, J. Qin and Z. Li, Chem. Commun., 2008, 5848 Search PubMed.
-
(a) K. Szacilowski, Chem. Rev., 2008, 108, 3481 CrossRef CAS;
(b)
Molecular Devices and Machines. A Journey into the Nano world; V. Balzini, M. Venturi, A. Credi, Ed.; Wiley-VCH: Wienheim, 2003.
- A. P. de Silva, M. R. James, B. O. F. McKinney, P. A. Pears and S. M. Weir, Nat. Mater., 2006, 5, 787 CrossRef.
-
(a) G. Periyasamy, J. P. Collin, J. P. Sauvage, R. D. Levine and F. Remacle, Chem.–Eur. J., 2009, 15, 1310 CrossRef CAS;
(b) G. de Ruiter, E. Tartakovsky, N. Oded and M. E. van der Boom, Angew. Chem. Int. Ed., 2010, 49, 169 CAS.
-
(a) D. Margulies, C. E. Felder, G. Melman and A. Shanzer, J. Am. Chem. Soc., 2007, 129, 347 CrossRef CAS;
(b) M. Suresh, A. Ghosh and A. Das, Chem. Commun., 2008, 3906 Search PubMed.
- Z. Guo, W. Zhu, L. Shen and H. Tian, Angew. Chem., Int. Ed., 2007, 46, 5549 CrossRef CAS.
- N. Morohashi, F. Narumi, N. Iki, T. Hattori and S. Miyano, Chem. Rev., 2006, 106, 5291 CrossRef CAS.
-
(a) M. Kumar, R. Kumar and V. Bhalla, Org. Lett., 2011, 13, 366 CrossRef CAS;
(b) M. Kumar, R. Kumar and V. Bhalla, Chem. Commun., 2009, 7384 Search PubMed;
(c) M. Kumar, A. Dhir and V. Bhalla, Org. Lett., 2009, 11, 2567 CrossRef CAS;
(d) M. Kumar, A. Dhir and V. Bhalla, Chem. Commun., 2010, 6744 Search PubMed.
-
(a) M. Kumar, R. Kumar and V. Bhalla, Tetrahedron, 2009, 65, 4340 CrossRef CAS;
(b) J. N. Babu, V. Bhalla, M. Kumar, R. K. Puri and R. K. Mahajan, New J. Chem., 2009, 33, 675 RSC.
- G. L. Long and J. D. Winefordner, Anal. Chem., 1983, 55, 712A CrossRef CAS.
- H. Gampp, M. Maeder, C. J. Meyer and A. D. Zuberbulher, Talanta, 1985, 32, 95 CrossRef CAS.
|
This journal is © The Royal Society of Chemistry 2011 |