DOI:
10.1039/C1PY00093D
(Communication)
Polym. Chem., 2011,
2, 1648-1653
A new benzoxazine containing uracil, complementary functionality†
Received
3rd March 2011
, Accepted 31st May 2011
First published on 10th June 2011
Abstract
A new benzoxazine, uracil containing benzoxazine (UBz), has been successfully synthesized. The FTIR and DSC measurement of the poly(UBz)/poly(BA-a) blends indicated that hydrogen bonding interactions between uracil groups would result in a decrease in the exothermic peak temperature. In addition, the PL measurement confirmed that the poly(UBz)/poly(BA-a) blend possessed complementary behavior.
Introduction
Polybenzoxazine cured from benzoxazine monomers is a type of addition-cure phenolic system possessing several attractive properties as compared with conventional phenolic resins, such as low dimension change and no byproducts release during curing (curing without the addition of strong acid catalyst), good mechanical properties, high glass transition temperature, high char yield, low surface free energy, etc.1–3 Since Holly and Cope synthesized the benzoxazine monomers in the 1940s,4benzoxazines with diverse functionalities and other additional properties have been developed. Recently, syntheses of new benzoxazines with specific functionalities have attracted great interest because these new benzoxazines can be attached to different moieties easily and improve the properties of the resulting polybenzoxazine. Yagci et al. introduced the proparagyl group onto a benzoxazine and successfully attached it to the modified polyvinylchloride (PVC) through the click chemistry.5 They also synthesized a benzoxazine containing ethanol group which can be used as an initiator to prepare a naphthoxazine functionalized poly(ε-caprolactone) through ring opening polymerization.6 To improve the properties of polybenzoxazines, silicon-containing and poly(ether ester) (PEE) containing benzoxazines were prepared by Liu and Yagci, the new polybenzoxazines possessed enhanced glass transition temperature and flexibility.7,8 In addition, Chang and Lin9 and Ishida et al.10 prepared benzoxazines with diamine functionalities, opening new strategies to synthesize benzoxazines and polybenzoxazines. Other benzoxazines with functionalities such as allyl,11acetylene,12nitrile,13malemide,14 and coumarine15groups have also been prepared for specific uses.
In the present study, we introduced a supramolecular functionality, uracil, to benzoxazine. Recently, supramolecular functionalities possessing highly directional and sufficiently strong non-covalent host–guest pairs (arrays of hydrogen bonds) have attracted great attention.16–19 Uracil (U), a functionality of RNA, is one of the supramolecular functionalities which has the ability to associate with adenine (A) of both RNA and DNA through complementary hydrogen bonding interaction, and also can self-associate through self-complementary interaction.20,21 In our previous studies, several polymers with supramolecular functionalities have been synthesized and indicated that they possessed unique properties and had the potential for practical use.21,22 In this study, uracil based benzoxazine (UBz) was successfully synthesized and incorporated into BA-a benzoxazine. The influence of the self-complementary interaction on exothermic peak temperature of the UBz/BA-a blends was investigated. In addition, the PL measurement was employed and indicated that the uracil groups within poly(UBz)/poly(BA-a) blends possess complementary behavior.
Experimental
Materials
1,10-Dibromodecane, 1,4-dibromodecane, 4-nitrophenol, uracil, adenine, potassium bicarbonate, paraformaldehyde, phenol, 1-pyrenemethanol, sodium hydride, anhydrous magnesium sulfate, palladium on activated carbon (unreduced, 10% Pd/C), and hydrazine hydrate (80%), were purchased from Sigma-Aldrich. N,N-Dimethylformamide (DMF) and tetrahydrofuran (THF) (Sigma-Aldrich) were vacuum-distilled prior to use and all other chemicals were reagent grade from Sigma-Aldrich and used as received. BA-a benzoxazine was supplied by Shikoku Corp.
4-Nitrophenol (1.39g, 10 mmol) and potassium carbonate (1.66g, 12 mmol) were dissolved in 50 mL of acetone under an argon atmosphere with magnetic stirring at 50 °C for 1 h. 1,10-Dibromodecane (6 g, 20 mmol) was then added to the solution and the suspension was maintained at 50 °C for 24 h. The solution was cooled to 30 °C and filtered to remove most of the salt and then dried under vacuum. The product (slightly yellow powder, Mp = 55.4 °C) was obtained through crystallization from petroleum ether (yield: 83%), see Fig. 1.
Synthesis of 1-[10-(4-nitro-phenoxy)-decyl]-1H-pyrimidine-2,4-dione (Compound 2)
Compound 1 (7.79g, 20 mmol), uracil (4.48g, 40 mmol) and potassium carbonate (5.53g, 40 mmol) were added to 200 mL of DMF under an argon atmosphere and stirred at 75 °C for 48 h. The solution was cooled to 30 °C and filtrated to remove most of the salt and then dried under vacuum. The product (slightly yellow powders, Mp = 120.2 °C) was obtained through crystallization from the dichloromethane (yield: 63%), see Fig. 2.
Synthesis of 1-[10-(4-amino-phenoxy)-decyl]-1H-pyrimidine-2,4-dione (Compound 3)
Compound 2 (7.69g, 20 mmol), 10% Pd/C (0.15g) and 50 mL of hydrazine monohydrate were added to 150 mL of ethanol under an argon atmosphere and stirred at 95 °C for 3 h. The solution was filtered to remove the Pd/C catalyst and then the product (white powders, Mp = 168.5 °C) was obtained through crystallization from ethanol (yield: 89%), see Fig. 3.
Synthesis of 1-{[10-[4-(4a,8a-dihydro-4H-benzo[e][1,3]oxazin-3-yl)-phenoxyl]-decyl}-1H-pyrimidine-2,4-dione (UBz)
Compound 3 (0.15 g, 0.4 mmol), phenol (0.18 g, 2 mmol) and paraformaldehyde (0.0275 g) were dissolved in 10 ml of chloroform. The suspension was stirred at 125 °C for 12 h under an argon atmosphere. After filtration, the solution was partitioned between chloroform and 1 N sodium bicarbonate and then the final product, UBz (yellow viscous liquid), was obtained through chromatography (yield: 35%), see Fig. 4 and Scheme 1.
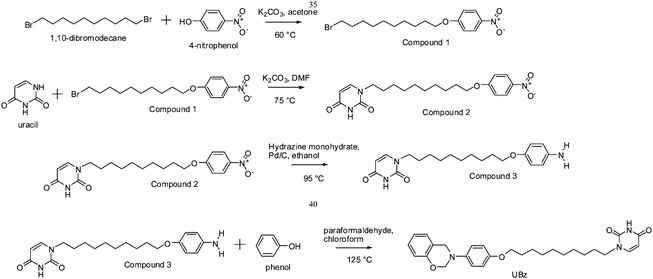 |
| Scheme 1 The series of reactions to synthesize UBz. | |
A-pyrene shown in Scheme 2 was synthesized as we reported and illustrated in the ESI.†
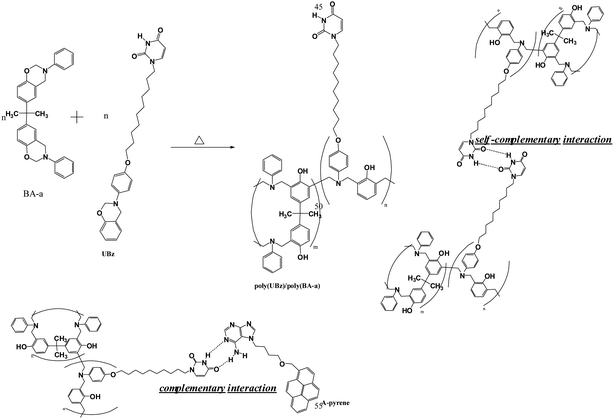 |
| Scheme 2 The structures of the poly(UBz)/poly(BA-a) blend, the self-complementary interaction between uracil groups and the complementary interaction between uracil and A-pyrene. | |
Blend preparation
Desired amounts of UBz and BA-a were dissolved in THF and stirred at 30 °C overnight. The compositions of UBz/BA-a blends are summarized in Table 1. The solutions were then cast onto Teflon dishes and heated at 40 °C for 4 h to remove most of the solvent. Finally, these samples were dried under vacuum at 40 °C for an additional 8 h. To cure BA-a and UBz/BA-a blends, they were heated at 210 °C for 2 h in an oven and the DSC thermograms obtained after heating indicated that the curing reaction was complete (see ESI†).
Table 1 Compositions of the UBz/BA-a blends
|
UBz (wt%) |
BA-a (wt%) |
UBz/BA-a (10/90) |
10 |
90 |
UBz/BA-a (20/80) |
20 |
80 |
UBz/BA-a (50/50) |
50 |
50 |
UBz/BA-a (80/20) |
80 |
20 |
UBz/BA-a (90/10) |
90 |
10 |
Absorption test
Desired amounts of BA-a and UBz/BA-a blends were dissolved in THF to form 1 wt% solutions. The solution was cast onto quartz glass using spin coating (1500 rpm for 30 s) and then the sample was cured as described above. Before the PL measurement, the resulting poly(BA-a) and poly(UBz)/poly(BA-a) blends were immersed in 10 ml of dichloromethane solution of A-pyrene (0.25 wt%) for 5 mins and washed three times with dichloromethane.
Results and discussion
In this study, uracil was incorporated into benzoxazine through series of reactions for the first time. The precursor, compound 3, was designed to have an amine group (for forming benzoxazine), a uracil group, and a long alkyl chain (for increasing its solubility with low polarity solvents, such as chloroform and 1,3-dioxane, which were usually employed for synthesizing benzoxazines). Although compound 3 possesses a long alkyl chain, the presence of highly polar amine and uracil groups still caused difficulty in dissolving in non-polar solvents. Therefore, in the final step in the synthesis of UBz, the amount of phenol used was five times that of compound 3. The excess phenol plays a role as solvent to dissolve the highly polar compound 3, which is the key to the synthesis.
Fig. 5 illustrates the DSC thermograms of UBz, BA-a, and UBz/BA-a blends. The exothermic peak temperatures of UBz and BA-a are both near 247 °C and the exothermic peak temperatures of the UBz/BA-s blends remain unchanged, disregarding the UBz content in the blends, except in the UBz/BA-a (10/90) blend. The UBz/BA-a (10/90) blend has a slightly lower exothermic peak temperature at 232 °C. In a previous study, Ronda et al. indicated that the incorporation of electron-donating or withdrawing groups to benzoxazines would result in a change in their exothermic peak temperatures.23 In the present study, the uracil group on UBz did not appreciably influence the exothermic peak temperature as compared with BA-a, probably due to the existence of the long alkyl chain between uracil and the benzoxazine ring. However, for the UBz/BA-a (10/90) blend, certain association between the UBz and BA-a may play a role in slightly lowering exothermic peak temperature.
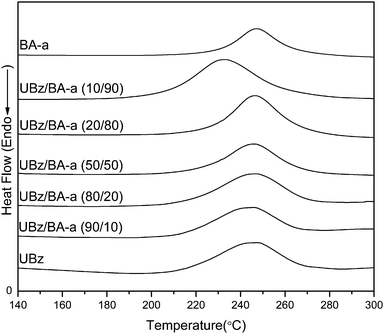 |
| Fig. 5
DSC thermograms of UBz, BA-a, and UBz/BA-a blends. | |
Fig. 6(a) and (b) illustrate the FTIR spectra of the C
O stretching of poly(UBz) (obtained from various temperatures) and poly(UBz)/poly(BA-a) blends, respectively. Since the uracil group possesses both imide and amide functionalities, several characteristic bands within the C
O stretching region are resulting from the self-complementary interactions (Scheme 2) as shown in Fig. 6(a). Upon heating from 30 to 180 °C, the intensities of the bands at 1660 and 1680 cm−1 of poly(UBz) are gradually decreased, representing the dissociation of hydrogen bonded C
O groups. Fig. 6(b) shows that the fraction of the hydrogen bonded C
O of the uracil group of poly(UBz)/poly(BA-a) (10/90) blend is higher than those of poly(UBz) and other blends, which is an interesting consequence. We surmise that the relatively higher fraction of hydrogen bonded uracil results in change in the exothermic peak temperature.24
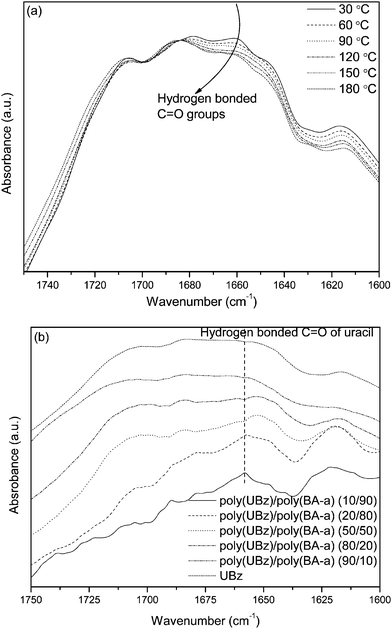 |
| Fig. 6
FTIR spectra of (a) poly(UBz) obtained from various temperatures and (b) poly(UBz)/poly(BA-a) blends. | |
Besides the self-complementary interaction between uracil groups, uracil can also strongly associate with adenine (A) of both RNA and DNA through the complementary hydrogen bonding interaction (Scheme 2).20–22 In our previous studies, the A–U complementary interaction has been studied and indicated that it possesses unique selectivity and can result in specific microstructures.20,21 In order to confirm that the uracil groups within the poly(UBz)/poly(BA-a) blends possess the complementary behavior, the absorption test and PL measurement were employed. Previous studies have indicated that the equilibrium constant of A–U interaction is higher than that of A–A and U–U interactions.25 It can be expected that the A–U interaction is relatively more preferable as carrying out the absorption test. Fig. 3 compares the PL spectra of poly(BA-a) and poly(UBz)/poly(BA-a) (50/50) blend after immersion in dichloromethane solution of A-pyrene, a band centered at ca. 470 occurred from the poly(UBz)/poly(BA-a) (50/50) blend which can be attributed to the characteristic of pyrene.26 This result indicates that the A-pyrene was absorbed on the surface of the poly(UBz)/poly(BA-a) (50/50) blend while no absorption was observed for the immersed poly(BA-a). Since the surface after the absorption test was washed several times with dichloromethane, the difference in the PL spectra shown in Fig. 7 indicates that the presence of uracil within poly(UBz)/poly(BA-a) blends contributed sites which can form complementary interactions with the adenine derivative and polybenzoxazine. Although the absorption test was carried out through the interface between uracil containing benzoxazine film and adenine containing solution, the complementary interaction still resulted in successful absorption of A-pyrene onto the poly(UBz)/poly(BA-a) blend surface. To our knowledge, this is the first time polybenzoxazine was modified with functionality which possessed complementary behavior.
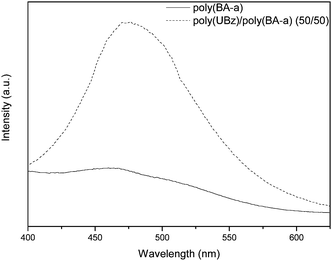 |
| Fig. 7 The PL spectra of poly(UBz)/poly(BA-a) and poly(BA-a) after absorption test. | |
Conclusions
In this study, a supramolecular functionality, uracil, has been successfully incorporated into benzoxazine. The consequence from FTIR and DSC measurements indicated that the relatively higher fraction of hydrogen bonded uracil groups within UBz/BA-a blends could result in a decrease in the exothermic peak temperature. In addition, the absorption test revealed that the presence of the uracil group of poly(UBz) led polybenzoxazine to possess complementary behavior, which is expected to expand the application of polybenzoxazine.
References
- N. N. Ghosh, B. Kiskan and Y. Yagci, Prog. Polym. Sci., 2007, 32, 1344 CrossRef CAS.
- Y. Yagci, B. Kiskan and N. N. Ghosh, J. Polym. Sci., Part A: Polym. Chem., 2009, 47, 5565 Search PubMed.
- C. F. Wang, Y. C. Su, S. W. Kuo, C. F. Huang, Y. C. Sheen and F. C. Chang, Angew. Chem., Int. Ed., 2006, 45, 2248 CrossRef CAS.
- F. W. Holly and A. C. Cope, J. Am. Chem. Soc., 1944, 66, 1875 CrossRef CAS.
- B. Kiskan, G. Demiray and Y. Yagci, J. Polym. Sci., Part A: Polym. Chem., 2008, 46, 3512 CrossRef CAS.
- B. Kiskan and Y. Yagci, Polymer, 2005, 46, 11690 CrossRef CAS.
- Y. L. Liu, C. W. Hsu and C. I. Chou, J. Polym. Sci., Part A: Polym. Chem., 2007, 45, 1007 Search PubMed.
- B. Kiskan, Y. Yagci and H. Ishida, J. Polym. Sci., Part A: Polym. Chem., 2008, 46, 414 Search PubMed.
- S. L. Chang and C. H. Lin, J. Polym. Sci., Part A: Polym. Chem., 2010, 48, 2430 Search PubMed.
- T. Agag, C. R. Arza, F. H. J. Maurer and H. Ishida, Macromolecules, 2010, 43, 2748 Search PubMed.
- T. Agag and T. Takeichi, Macromolecules, 2003, 36, 6010 Search PubMed.
- H. J. Kim, Z. Brunovska and H. Ishida, Polymer, 1999, 40, 6565 Search PubMed.
- Z. Brunovska and H. Ishida, J. Appl. Polym. Sci., 1999, 73, 2937 Search PubMed.
- H. Ishida and S. Ohba, Polymer, 2005, 46, 5588 Search PubMed.
- B. Kiskan and Y. Yagci, J. Polym. Sci., Part A: Polym. Chem., 2007, 45, 1670 Search PubMed.
- W. H. Binder and R. Zirbs, Adv. Polym. Sci., 2007, 207, 1 CAS.
- L. Brunsveld, B. J. B. Folmer, E. W. Meijer and R. P. Sijbesma, Chem. Rev., 2001, 101, 4071 CrossRef CAS.
- A. Ciferri, Macromol. Rapid Commun., 2002, 23, 511 CrossRef CAS.
- J. M. Lehn, Polym. Int., 2002, 51, 825 CrossRef CAS.
- C. C. Cheng, Y. C. Yen, Y. S. Ye and F. C. Chang, J. Polym. Sci., Part A: Polym. Chem., 2009, 46, 6388 Search PubMed.
- I. H. Lin, C. C. Cheng, Y. C. Yen and F. C. Chang, Macromolecules, 2010, 43, 1245 Search PubMed.
- Y. C. Yen, C. C. Cheng, S. W. Kuo and F. C. Chang, Macromolecules, 2010, 43, 2634 Search PubMed.
- R. Andreu, J. A. Reina and J. C. Ronda, J. Polym. Sci., Part A: Polym. Chem., 2008, 46, 3353 Search PubMed.
- H. M. Lin, S. Y. Wu, P. Y. Huang, C. F. Huang, S. W. Kuo and F. C. Chang, Macromol. Rapid Commun., 2002, 23, 511 CrossRef CAS.
- A. J. Wilson, Soft Matter, 2007, 3, 409 RSC.
-
(a) S. D. Kim and J. M. Torkelson, Macromolecules, 2002, 35, 5943 CrossRef CAS;
(b) Y. H. Kim, D. K. Yoon, E. H. Lee, Y. K. Ko and H. T. Jung, J. Phys. Chem. B, 2006, 110, 20836 CrossRef CAS.
Footnote |
† Electronic supplementary information (ESI) available. See DOI: 10.1039/c1py00093d |
|
This journal is © The Royal Society of Chemistry 2011 |