DOI:
10.1039/C0MD00205D
(Concise Article)
Med. Chem. Commun., 2011,
2, 119-125
Received
5th November 2010
, Accepted 14th November 2010
First published on 6th December 2010
Abstract
A bifunctional chelator featuring (DO3A-AM)-Porphyrin units has been prepared. The high-field relaxivity of the Gd complex is similar to those of currently available contrast agents clearly showing that the first coordination sphere remains identical to the parent molecule [Gd(DO3A-AM)(H2O)], with one coordinated water molecule.
Introduction
Non-invasive imaging techniques are perfect tools for the diagnosis of many diseases, like cancers. The most routinely used analyses at the clinical stage are MRI (Magnetic Resonance Imaging),1–8SPECT (Single Photon Emission Computed Tomography), PET (Positron Emission Tomography).7,9–21 Some other, less well known, imaging techniques are developed at the pre-clinical stage and based on optical imaging, e.g. FRI (fluorescence reflectance imaging), FMT (fluorescence-mediated tomography) or MPM (multiphoton microscopy). Computed tomography and magnetic resonance imaging are primary anatomical imaging techniques that provide superior resolution, while PET and SPECT are nuclear medicine imaging modalities that image physiological processes occurring within the living system. Of the latter two, PET has advantages with respect to sensitivity and resolution.11,12,18 In general all of these techniques need contrast agents in order either to operate or to enhance the contrast between normal and diseased tissue, as well as to indicate organ function or blood flow. Actually, the main isotopes for medical SPECT and TEP imaging are gamma and positron emitters, respectively, including e.g.99mTc, 111In, 68Ga… 64Cu has also emerged as an important positron emitting radionuclide that has the potential for use in diagnostic imaging.13
Our research recently focused on the synthesis of new bimodal contrast agents for medical imaging. Conveniently, the use of bimodal contrast agents could allow the study of the same biological structures at different depths and resolutions, to obtain the most relevant diagnosis. The COMPOUND LINKS
Read more about this on ChemSpider
Download mol file of compoundwater soluble bimodal contrast agent that we wish to synthesize (Chart 1) should strongly complex two metallic species in order to avoid any issues related to the trans-chelation or trans-metalation inside the biological organism at physiological pH.
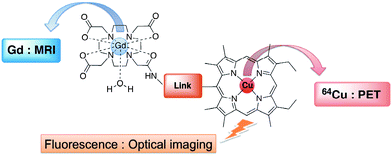 |
| Chart 1 | |
For those reasons, we decided to use on one hand a tetraazacycloalkane and on the other hand a porphyrin for potential MRI/TEP bimodality (e.g.Gd(DO3A-AM)22 as contrast agent in MRI and free-base COMPOUND LINKS
Read more about this on ChemSpider
Download mol file of compoundporphyrin as potential 64Cu complexing agent). Up to now, there are only a few examples of architectures incorporating porphyrins and tetraazacycloalkanes that have been reported in the literature, mainly for biomimetic studies.16,23–25 Indeed, it is well-known that the porphyrin macrocycles display interesting properties in terms of fluorescence, DNA targeting and tumoral tropism (it has been known for many years that porphyrin derivatives are selectively retained by tumors). Recently, paramagnetic metalloporphyrins were also developed as tumor seeking magnetic resonance imaging contrast agents, based on the tumoral “preferential uptake” property of porphyrins.8,17,26,27 In addition, 99mTc and 111In labeled porphyrins have also been reported.16,17,26 Interestingly many functionalized tetraazamacrocycles (e.g.DOTA derivatives) are commercially available and are widely used as contrast agents for MRI as well as for SPECT and PET imaging.7
Experimental section
Physicochemical characterization of compounds
1H NMR spectra were recorded on a Bruker Avance II 300 (300 MHz) or on a Bruker Avance DRX 600 (600 MHz) spectrometer at the “Plateforme d'Analyse Chimique et de Synthèse Moléculaire de l'Université de Bourgogne (PACSMUB)”; chemical shifts are expressed in ppm relative to COMPOUND LINKS
Read more about this on ChemSpider
Download mol file of compoundchloroform (7.26 ppm) or COMPOUND LINKS
Read more about this on ChemSpider
Download mol file of compoundwater (4.79 ppm). UV–Vis spectra were recorded on a Varian Cary 1 spectrophotometer. Mass spectra and accurate mass measurements (HR-MS) were obtained on a Bruker Daltonics Ultraflex II spectrometer in the MALDI/TOF reflectron mode using COMPOUND LINKS
Read more about this on ChemSpider
Download mol file of compounddithranol as a matrix or on a Bruker Micro TofQ instrument in ESI mode. Both measurements were made at the “Plateforme d'Analyse Chimique et de Synthèse Moléculaire de l'Université de Bourgogne (PACSMUB)”.
The 2,3,7,8,12,18-hexamethyl-5-(4-azidomethylphenyl)-13,17-diethylporphyrinato-Zn(II) 3 was prepared as recently described by our group.28
8,12-Diethyl-2,3,7,13,17,18-hexamethyl-20-p-(amino-methyl)phenylporphyrin
4
The zinc(II) p-azidomethylphenylporphyrin was first demetalated. Shielded from light, a solution of 2,3,7,8,12,18-hexamethyl-5-(4-azidomethylphenyl)-13,17-diethylporphyrinato-Zn 3 (270 mg, 0.418 mmol) in 50 mL of COMPOUND LINKS
Read more about this on ChemSpider
Download mol file of compounddichloromethane was stirred vigorously at room temperature and during 2 h with 50 mL of 1 M hydrochloric acid solution. The demetalation reaction was monitored by UV-visible and MALDI/TOF mass spectrometry. The organic layer was then washed three times with saturated sodium hydrogencarbonate solution, dried over MgSO4 and evaporated under reduced pressure. The free-base porphyrin 3 was not further purified and used directly in the following reduction step.
The free-base p-azidomethylphenylporphyrin 3 (242 mg, 0.416 mmol) was dissolved in 50 mL of THF in the presence of 120 mg (0.530 mmol) of COMPOUND LINKS
Read more about this on ChemSpider
Download mol file of compoundtriphenylphosphine and 30 μL of COMPOUND LINKS
Read more about this on ChemSpider
Download mol file of compoundwater. The reaction mixture was heated under reflux for 7 h, shielded from light. The solvent was removed and the crude product was dissolved in a CH2Cl2–MeOH solution (97
:
3) purified by column chromatography over COMPOUND LINKS
Read more about this on ChemSpider
Download mol file of compoundalumina (CH2Cl2–COMPOUND LINKS
Read more about this on ChemSpider
Download mol file of compoundacetic acid, 75
:
25). The purple band was collected, washed with saturated sodium hydrogencarbonate solution and dried over MgSO4. The title compound 4 was isolated as a purple microcrystalline solid in 40% yield (92 mg, 0.165 mmol). UV/Vis (CH2Cl2): λmax (nm) (ε × 10−3 L mol−1 cm−1) = 402 (121), 500 (10), 535 (5), 570 (5), 624 (2) nm. 1H NMR (300 MHz, COMPOUND LINKS
Read more about this on ChemSpider
Download mol file of compoundCDCl3) δ (ppm): −3.2 (s, 2H, NHporph); 1.85 (t, J = 7.4 Hz, 6H, -CH2-CH3); 2.43 (s, 6H, -CH3); 3.50 (s, 6H, -CH3); 3.61 (s, 6H, -CH3); 4.03–4.10 ppm (s, 2H, -NH2 overlapped with q, J = 7.4 Hz, 4H, -CH2-CH3); 4.22 (s, 2H, -CH2-NH2); 7.64 (d, J = 8.0 Hz, 2H, HAr); 7.98 (d, J = 8.0 Hz, 2H, HAr); 9.92 (s, 1H, Hmeso); 10.14 ppm (s, 2H, Hmeso). MS (MALDI-TOF) m/z = 556.16 [M + H]+ 556.34 calcd for C37H41N5.
8,12-Diethyl-2,3,7,13,17,18-hexamethyl-20-(benzyl-acetamide-N-DO3A-(tris-tert-butylester))porphyrin
6
p-(Aminomethyl)phenylporphyrin
4 (91.0 mg, 0.163 mmol), DOTA-tris(tBu)ester 5 (94.4 mg, 0.164 mmol), COMPOUND LINKS
Read more about this on ChemSpider
Download mol file of compound1-ethyl-3-(3-dimethylaminopropyl)carbodiimide hydrochloride (EDC·HCl) (41.0 mg, 0.213 mmol) and hydroxybenzotriazole (COMPOUND LINKS
Read more about this on ChemSpider
Download mol file of compoundHOBt) (28.7 mg, 0.212 mmol) were dissolved in 40 mL of DMF. The reaction mixture was then stirred at room temperature for 24 h, shielded from light. The solvent was removed under vacuum and the crude product was redissolved in 50 mL of CH2Cl2. The organic phase was washed three times with COMPOUND LINKS
Read more about this on ChemSpider
Download mol file of compoundwater then the solvent was removed. The crude product was dissolved in a CH2Cl2–MeOH (90
:
10) solution and purified by chromatography over COMPOUND LINKS
Read more about this on ChemSpider
Download mol file of compoundalumina (CH2Cl2–COMPOUND LINKS
Read more about this on ChemSpider
Download mol file of compoundacetic acid (80
:
20) as eluent). The purple band was collected, washed with saturated COMPOUND LINKS
Read more about this on ChemSpider
Download mol file of compoundsodium hydrogencarbonate solution and dried over MgSO4. COMPOUND LINKS
Read more about this on ChemSpider
Download mol file of compoundPorphyrin 6 was isolated as a purple microcrystalline solid in 45% yield (82 mg, 0.073 mmol). UV/Vis (CH2Cl2): λmax (nm) (ε × 10−3 L mol−1 cm−1) = 403 (130), 503 (10), 535 (5), 570 (5), 624 (2) nm. 1H NMR (400 K, 600 MHz, COMPOUND LINKS
Read more about this on ChemSpider
Download mol file of compoundDMSO-d6) δ (ppm): −3.16 (s, 2H, NHporph); 1.46 (s, 27H, -C(CH3)3); 1.88 (t, J = 7.4 Hz, 6H, -CH2-CH3); 2.43 (s, 6H, -CH3); 2.60 (s broad, 16H, -N–CH2-CH2-N-); 3.22 (s, 6H, -N–CH2-COOtBu); 3.32 (s, 2H, -N–CH2-CON-); 3.50 (s, 6H, -CH3); 3.63 (s, 6H, -CH3); 4.09 (q, J = 7.4 Hz, 4H, -CH2-CH3); 4.76 (d, J = 6.0 Hz, 2H, Ar–CH2-NH-CO-); 7.72 (d, J = 8.0 Hz, 2H, HAr); 7.96 (d, J = 8.0 Hz, 2H, HAr); 8.71 (t, J = 6.0 Hz,1H, Ar-CH2-NH-CO-); 10.02 (s, 1H, Hmeso); 10.18 ppm (s, 2H, Hmeso). MS (MALDI-TOF) m/z = 1132.60 [M + Na]+ 1132.69 calcd for C65H91N9O7Na. HR-MS (ESI) m/z = 1132.6917 [M + Na]+ 1132.6939 calcd for C65H91N9O7Na.
8,12-Diethyl-2,3,7,13,17,18-hexamethyl-20-(benzyl-acetamide-N-DO3A-(tris-COOH))porphyrin
7
DO3A-AM-(tris-tert-butylester)porphyrin
6 (50.0 mg, 0.045 mmol) was dissolved in 5 mL of CH2Cl2 in the presence of 1 mL of COMPOUND LINKS
Read more about this on ChemSpider
Download mol file of compoundtrifluoroacetic acid (the solution turns from purple to green). The reaction mixture was stirred at room temperature for 24 h, shielded from light. The crude product was redissolved in 5 mL of CH2Cl2 then evaporated under vacuum. This procedure was repeated 4 times in order to totally remove TFA and tBuOH. The isolated compound was then dissolved in Milli-Q COMPOUND LINKS
Read more about this on ChemSpider
Download mol file of compoundwater and freeze-dried. The title compound 7 was isolated in quantitative yield (42 mg, 0.044 mmol) as a brown-purple powder. UV/Vis (COMPOUND LINKS
Read more about this on ChemSpider
Download mol file of compoundH2O): λmax (nm) (ε × 10−3 L mol−1 cm−1) = 403 (30), 503 (5), 535 (3), 570 (3), 624 (1.5) nm. 1H NMR (400 K, 600 MHz, COMPOUND LINKS
Read more about this on ChemSpider
Download mol file of compoundDMSO-d6) δ (ppm): 1.88 (t, J = 7.4 Hz, 6H, -CH2-CH3); 2.44 (s, 6H, -CH3); 3.15 (m, 16H, -N–CH2-CH2-N-); 3.51 (s, 6H, -CH3); 3.63 (s, 6H, -CH3); 3.71 (s, 4H, -N–CH2-COOH); 3.72 (s, 2H, -N–CH2-COOH); 3.86 (s, 2H, -N–CH2-CON-); 4.09 (q, J = 7.4 Hz, 4H, -CH2-CH3); 4.76 (d, J = 6.0 Hz, 2H, Ar–CH2-NH-CO-); 7.74 (d, J = 8.0 Hz, 2H, HAr); 8.00 (d, J = 8.0 Hz, 2H, HAr); 8.71 (t, J = 6.0 Hz, 1H, Ar-CH2-NH-CO-); 10.04 (s, 1H, Hmeso); 10.21 (s, 2H, Hmeso). MS (MALDI-TOF) m/z = 942.40 [M + H]+ 941.52 calcd for C53H68N9O7. HR-MS (ESI) m/z = 940.5058 [M − H]− 940.5090 calcd for C53H66N9O7.
8,12-Diethyl-2,3,7,13,17,18-hexamethyl-20-(benzyl-acetamide-N-DO3A gadolinium)-porphyrin
8
The DO3A-AM-porphyrin 7 (16.0 mg, 0.0168 mmol) was dissolved in Milli-Q COMPOUND LINKS
Read more about this on ChemSpider
Download mol file of compoundwater (3 mL) and the pH adjusted to 8 with two COMPOUND LINKS
Read more about this on ChemSpider
Download mol file of compoundNaOH aqueous solutions (1M and 0.1M). Then, a solution of Gd(NO3)3·5H2O (7.9 mg, 0.0182 mmol) in Milli-Q COMPOUND LINKS
Read more about this on ChemSpider
Download mol file of compoundwater (1 mL) was added, followed by adjustment of the pH to 6.7. The reaction mixture was heated at 50 °C for 12 h, while maintaining the pH to 6.7.
The resulting solution led, after cooling to room temperature, to the formation of a dark red microcrystalline solid, which was separated by filtration, washed with Milli-Q COMPOUND LINKS
Read more about this on ChemSpider
Download mol file of compoundwater, and dried under vacuum to yield the gadolinium DO3A-AM-porphyrin complex 8 in 75% yield (14.0 mg, 0.0127 mmol). Anal. Calcd for [Gd(L)] C53H64N9O7Gd·4H2O·3NaOH·0.2tBuOH: C, 49.58; H, 5.72; N, 9.67. Found: C, 49.47; H, 5.96; N, 9.29%. UV–Vis (COMPOUND LINKS
Read more about this on ChemSpider
Download mol file of compoundH2O): λmax (nm) (ε × 10−3 L mol−1 cm−1) = 380 (42), 509 (6), 540 (4), 576 (3.5), 630 (1.7) nm. HR-MS (MALDI-TOF) m/z = 1097.4158 [M + H]+ 1097.4255 calcd for C53H65GdN9O7. HR-MS (ESI) m/z = 1119.4015 [M + Na]+ 1119.4074 calcd for C53H64GdN9O7Na.
Copper(II) 8,12-diethyl-2,3,7,13,17,18-hexamethyl-20-(benzyl-acetamide-N-DO3A-gadolinium)porphyrin
9
The (DO3A-AM)Gd–H2(Porphyrin) 8 (5.0 mg, 4.6 μmol) was dissolved in 2 mL of COMPOUND LINKS
Read more about this on ChemSpider
Download mol file of compoundDMSO. COMPOUND LINKS
Read more about this on ChemSpider
Download mol file of compoundCopper nitrate trihydrate (1.0 mg, 4.1 μmol) was then added and the solution was heated at 50 °C for 2 h, shielded from light. The solvent was then removed under vacuum and the solid thus obtained was washed with COMPOUND LINKS
Read more about this on ChemSpider
Download mol file of compoundMeOH. The non-soluble solid was then filtered to afford the title compound as a red microcrystalline solid (4.5 mg, 3.9 μmol, 85% yield). UV/Vis (COMPOUND LINKS
Read more about this on ChemSpider
Download mol file of compoundH2O): λmax (nm) (ε × 10−3 L mol−1 cm−1) = 390 (25), 536 (2.5), 569 (3.0) nm. HR-MS (ESI) m/z = 1158.3312 [M + H]+ 1158.3385 calcd for C53H63CuGdN9O7.
Relaxivity measurements
Solutions of [Gd(L)] for relaxivity measurements were prepared by dissolving the (DO3A-AM)Gd–H2(Porphyrin) 8 complex into an H2O–DMSO 99
:
1 mixture (pH = 7.5, CGd = 0.15–0.20 mM) (Table 1). The exact Gd(III) ion concentration was determined by emission spectrometry on a Vista AX CCD Simultaneous ICP-AES, Varian.
Table 1 Relaxivity measurements of (DO3A-AM)Gd–H2(Porphyrin) 8 and (DO3A-AM)Gd-(Porphyrin)Cu 9 at different frequencies (T = 298 K)
Gd complex |
Relaxivity, r1/in mM−1s−1 |
300 MHz |
60 MHz |
20 MHz |
(DO3A-AM)Gd–H2(Porph) 8 (average values measured for two concentrations) |
4.15 ± 0.2 |
11.9 ± 1.0 |
18.9 ± 1.0 |
(DO3A-AM)Gd-(Porph)Cu
9 (c = 1.63.10−4 mol L−1) |
3.85 ± 0.2 |
— |
18.8 ± 1.0 |
A solution of the reference complex [Gd(DOTA)]− was also prepared in situ by dissolving equimolar amounts of the corresponding ligand H4DOTA (CheMatech) and Gd(NO3)3·5H2O into an H2O–DMSO 99
:
1 mixture followed by adjustment of the pH with COMPOUND LINKS
Read more about this on ChemSpider
Download mol file of compoundNaOH aqueous solutions (1M and 0.1M) (pH = 7.5, CGd = 0.24 mM, Cligand = 0.28 mM). Gd(III) solutions were prepared by dissolving the appropriate amount of Gd(NO3)3·5H2O in COMPOUND LINKS
Read more about this on ChemSpider
Download mol file of compoundwater. The exact Gd(III) ion concentration was determined by colorimetric titration in acetate buffer (pH = 4.5) using standardized H2Na2edta solutions (Aldrich) and COMPOUND LINKS
Read more about this on ChemSpider
Download mol file of compoundxylenol orange as the indicator. It has to be noted that, for each sample, the absence of free gadolinium was checked by the xylenol orange test.29
The longitudinal relaxation times T1 were measured at 20 MHz (0.47 T) and 60 MHz (1.41 T) and at 298 K on a Bruker Minispec “mqvar” ND2318. Relaxation measurements were also performed at 300 MHz (7.07 T) on a Bruker Avance II 300 MHz NMR spectrometer (Table 1).
Results and discussion
Synthesis and physicochemical characterization
We wish to report herein a very efficient and facile synthesis of an asymmetrical DO3A-AM-Porphyrin and its metal complexes. The structures of the DO3A-AM-Porphyrin derivatives are shown in Chart 2. The key p-azidomethyl-phenyl-porphyrin 3 was first prepared in a two step synthesis starting from a,c-biladiene 2 and COMPOUND LINKS
Read more about this on ChemSpider
Download mol file of compoundp-azidomethylbenzaldehyde 1.30 Interestingly, this key precursor could also be used in “click chemistry” reaction as described recently by our group.28,31 The azido group was further reduced in 40% yield by a phosphine mediated reduction in THF in the presence of COMPOUND LINKS
Read more about this on ChemSpider
Download mol file of compoundwater to give the p-(aminomethyl)phenylporphyrin 4. We then decided upon an amide bond to link the porphyrin moiety to the DOTA-like macrocycle. Indeed, amide bonds are biocompatible and they play a major role in the elaboration and composition of biological systems, representing for example the main chemical bonds that link amino acid building blocks together to give proteins.32Amide bonds are generally prepared by reaction of carboxylic acids and amines; however, the reaction usually requires drastic conditions (e.g. high temperatures >200 °C to remove COMPOUND LINKS
Read more about this on ChemSpider
Download mol file of compoundwater).32 For these reasons, it is usually necessary to activate the carboxylic acid function, here the single -COOH functional group of the DOTA-(tris-tert-butylester) 5. The coupling reagent we used (Scheme 1) was based on a carbodiimide (e.g.1-ethyl-3-(3-dimethylaminopropyl)carbodiimide, EDC·HCl) in the presence of COMPOUND LINKS
Read more about this on ChemSpider
Download mol file of compound1-hydroxy-1H-benzotriazole (COMPOUND LINKS
Read more about this on ChemSpider
Download mol file of compoundHOBt). The reaction mixture was stirred at room temperature during 24 h and, after classical workup, the expected DO3A-AM-(tris-tert-butylester)porphyrin 6 was isolated in 45% yield. The formation of the amide bond between the two macrocycles was clearly demonstrated by 1H NMR and by MALDI-TOF and ESI mass spectrometry (HR-MS), with the appearance in both spectra of a single ionic pattern corresponding to [M + Na]+ (See Experimental Section).
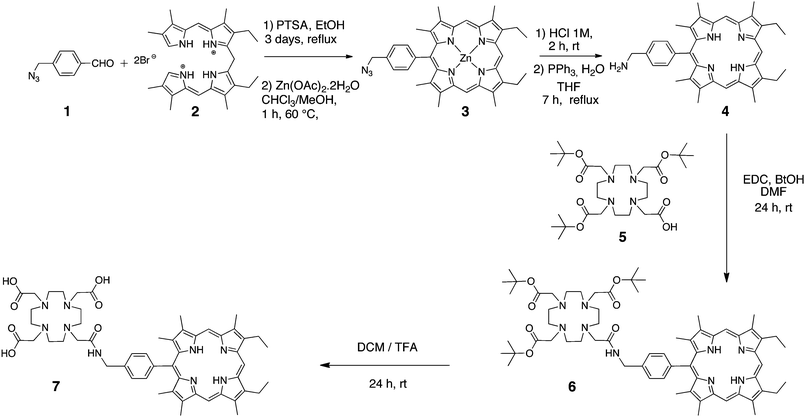 |
| Scheme 1 | |
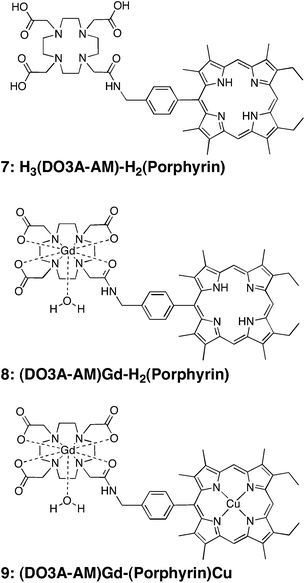 |
| Chart 2 | |
The three tert-butyl functionalities of compound 6 were then easily cleaved on treating with TFA, at room temperature and for 24 h, to produce quantitatively the related analogue, H3(DO3A-AM)-H2(Porphyrin) 7, with three carboxylic acid substituents. Interestingly, it is noteworthy that no cleavage (by hydrolysis) of the amide link has been observed during the TFA-mediated removal of the tert-butyl ester functionalities. The successful formation of H3(DO3A-AM)-H2(Porphyrin) 7 was confirmed by the HRMS (ESI) spectrum (Fig. 1), which exhibited a pseudo-molecular ion, [M + H]+ at 940.5058 Da (3 ppm deviation with respect to calculated mass). The 1H NMR spectrum (see Fig. 2) also showed the disappearance of the –NH2 peak at about 4.03–4.10 ppm (s, 2H, -NH2 overlapped with q, 4H, -CH2-CH3) and appearance of a new signal at about 8.71 ppm (s, 1H, Ar-CH2-NH-CO-). This strong downfield shift is easily explained by the strong deshielding effect of the amide function. At the same time, the Ar–CH2-N is deshielded and appears as a doublet at 4.76 ppm (compared to 4.22 ppm for the Ar-CH2-NH2 of the p-(aminomethyl)phenylporphyrin precursor 4). It has to be noted that, at 300 K, the 1H NMR spectrum of the H3(DO3A-AM)-H2(Porphyrin) 7 displays many broad and unresolved resonances (due to the presence of different conformational isomers in slow exchange in solution) and it was necessary to increase the temperature to 400 K to obtain higher resolution (Fig. 2).
![Experimental and calculated ESI HR-MS [M + H]+ ionic pattern of H3(DO3A-AM)-H2(Porphyrin) 7.](/image/article/2011/MD/c0md00205d/c0md00205d-f1.gif) |
| Fig. 1 Experimental and calculated ESI HR-MS [M + H]+ ionic pattern of H3(DO3A-AM)-H2(Porphyrin) 7. | |
The gadolinium complex of the H3(DO3A-AM)-H2(Porphyrin) 7 was classically prepared by dissolving 0.92 eq. of the free-base ligand in Milli-Q COMPOUND LINKS
Read more about this on ChemSpider
Download mol file of compoundwater (18 mΩ) at pH adjusted to 8 with NaOH solution (Scheme 2). Then, a solution of 1.1 eq. of Gd(NO3)3·5H2O in Milli-Q COMPOUND LINKS
Read more about this on ChemSpider
Download mol file of compoundwater was added, followed by further adjustment of the pH to 6.7. After heating the reaction mixture at 50 °C for 12 h then cooling to room temperature, the corresponding gadolinium complex was filtered from the solution, and washed several times with Milli-Q COMPOUND LINKS
Read more about this on ChemSpider
Download mol file of compoundwater to get rid of the NaNO3 salts and the Gd(water) in excess. The gadolinium (DO3A-AM)-porphyrin complex was obtained in 45% yield, as a dark red microcrystalline solid. The HRMS spectrum of 8 confirmed that full complexation occurs as no free ligand 7 was observed (Fig. S1†).
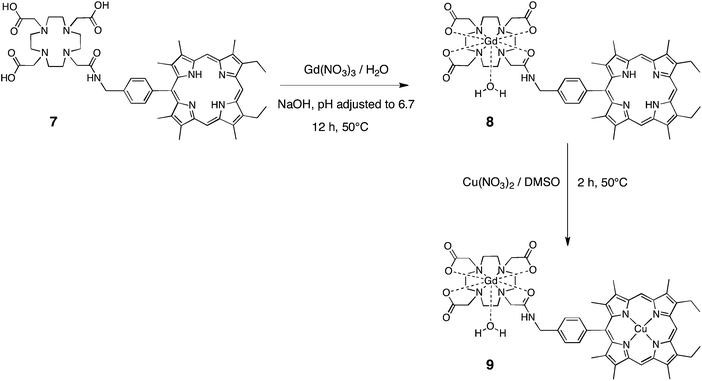 |
| Scheme 2 | |
The next step in the synthesis of the bimodal imaging contrast agent was the metalation of the porphyrin macrocycle. At this stage, it is worthy to note that the metal to be inserted in the cavity should present a higher affinity for the porphyrin macrocycle than for the tetraazamacrocycle, mainly in order to avoid any transmetalation of the gadolinium complex and the release of free Gd(water), which is toxic.
As significant research effort has been devoted to the development of ligands that can stably coordinate 64Cu (t1/2 = 12.7 h), we first wished to prepare a heterobimetallic Cu–Gd complex, soluble in COMPOUND LINKS
Read more about this on ChemSpider
Download mol file of compoundwater, as potential bimodal agent for MRI and PET imaging. Insertion of Cu(II) into the porphyrin macrocycle of (DO3A-AM)Gd–H2(Porphyrin) 8 was achieved by heating at 50 °C the free-base (DO3A-AM)Gd-Porphyrin with COMPOUND LINKS
Read more about this on ChemSpider
Download mol file of compoundcopper(II) nitrate trihydrate in COMPOUND LINKS
Read more about this on ChemSpider
Download mol file of compoundDMSO for 2 h to afford the copper–gadolinium (DO3A-AM)Gd-(Porphyrin)Cu 9 in 85% yield (Scheme 2). The uncomplexed free-base (DO3A-AM)Gd-Porphyrin 8 was removed by washings with COMPOUND LINKS
Read more about this on ChemSpider
Download mol file of compoundMeOH, the efficiency of those washings was monitored by ESI mass spectrometry (Fig. S2†). This bismetallic complex was characterized by HRMS (Fig. 3) mass spectrometry and UV-visible spectroscopy (Fig. S3†). Indeed, UV-visible spectroscopy, recorded in COMPOUND LINKS
Read more about this on ChemSpider
Download mol file of compoundwater, clearly confirmed that the sequential metalation took place as indicated in Scheme 2. The UV–visible spectrum of the (DO3A-AM)Gd-(Porphyrin)Cu 9 shows, in COMPOUND LINKS
Read more about this on ChemSpider
Download mol file of compoundwater, a red-shift of the Soret band by ca. 10 nm and the presence of only two visible bands at 536 and 569 nm, characteristic of the copper insertion into the porphyrin core (Fig. S2†). It is noteworthy that no transmetalation of the gadolinium complex has been observed by mass spectrometry (MALDI/TOF and ESI) and that the bimetallic complex 9 remains stable in COMPOUND LINKS
Read more about this on ChemSpider
Download mol file of compoundwater and at physiological pH (7.4).
![Experimental and calculated ESI HR-MS [M + H]+ ionic pattern of (DO3A-AM)Gd-(Porphyrin)Cu 9.](/image/article/2011/MD/c0md00205d/c0md00205d-f3.gif) |
| Fig. 3 Experimental and calculated ESI HR-MS [M + H]+ ionic pattern of (DO3A-AM)Gd-(Porphyrin)Cu 9. | |
Relaxivity measurements
The efficiency of a Gd(III) complex to improve the contrast in magnetic resonance images is gauged by its longitudinal relaxivity r1.33r1 represents the paramagnetic relaxation enhancement of the longitudinal relaxation rate of the water protons as a result of 1mM of this Gd(III) complex at a given temperature and magnetic field:
where T1 is the longitudinal relaxation time.
Concentration-dependent measurements of the longitudinal relaxation times T1 of two water solutions of 8 (namely 1.56 × 10−4 and 2.08 × 10−4 mol L−1) were performed in COMPOUND LINKS
Read more about this on ChemSpider
Download mol file of compoundH2O–DMSO 99
:
1 mixtures at pH = 7.4, at 25 °C and at different fields, giving average relaxivity values of 4.2, 11.9 and 18.9 mM−1s−1, at 300 MHz, 60 MHz and 20 MHz, respectively (Table 1). It has to be noted that a 99
:
1 COMPOUND LINKS
Read more about this on ChemSpider
Download mol file of compoundH2O:COMPOUND LINKS
Read more about this on ChemSpider
Download mol file of compoundDMSO mixture has been used, at pH 7.4, for T1 measurements, in order to solve solubility issues. However, in these conditions, the molar fraction of COMPOUND LINKS
Read more about this on ChemSpider
Download mol file of compoundDMSO (1%) is sufficiently small to consider that the solvation of the complex is the same as in pure COMPOUND LINKS
Read more about this on ChemSpider
Download mol file of compoundwater. Therefore, the dynamics and relaxivity of the complex should not be affected by the presence of 1% COMPOUND LINKS
Read more about this on ChemSpider
Download mol file of compoundDMSO. The relaxivity at 300 MHz is similar to that measured for [Gd(DOTA)(H2O)]− in the same conditions (r1 = 3.61 mM−1s−1 at 300 MHz, in COMPOUND LINKS
Read more about this on ChemSpider
Download mol file of compoundH2O–DMSO 99
:
1, pH = 7.5, 25 °C). These high-field relaxivities are typical values for gadolinium complexes with one COMPOUND LINKS
Read more about this on ChemSpider
Download mol file of compoundwater molecule in the first coordination sphere.
However, the medium-field relaxivity of 8, (DO3A-AM)Gd–H2(Porphyrin), is significantly higher than those of commercially available contrast agents (r1 = 4.2 mM−1s−1 and 4.3 mM−1s−1 for [Gd(DOTA)(H2O)]− and [Gd(DTPA)(H2O)]2− at pH = 7.4 and 20 MHz).34–36 This is indicative of a slow molecular tumbling of the porphyrin conjugate, presumably because of its higher molecular weight and the presence of the porphyrin unit. As a comparison, a relaxivity of 14.8 mM−1s−1 at 20 MHz has been measured by Simonneaux et al. for a Gd2(DTPA)4(H2O)2-tetra-p-aminophenylporphyrin conjugate.37 Moreover, a similar relaxivity has been measured at 20 and 300 MHz for the copper complex 9 (Table 1), which further confirms the stability of the complex and the absence of any transmetalation at physiological pH. In the case of 9, the paramagnetic copper(II) does not contribute to the relaxivity as no COMPOUND LINKS
Read more about this on ChemSpider
Download mol file of compoundwater molecule is bound to the copper atom. Indeed, COMPOUND LINKS
Read more about this on ChemSpider
Download mol file of compoundporphyrin macrocycles usually lead to the formation of four-coordinate copper(II) complexes (the copper atom being coordinated by the 4N inner nitrogen of the porphyrin core). In addition to the particularly high relaxivity of 8 at clinical field, this makes our porphyrin-conjugate a very promising ligand for 64Cu radiolabelling and as a MRI/PET bimodal agent.
Conclusion
In summary, we have developed a concise and efficient synthetic strategy towards the first Gd(DO3A-AM)-Cu(porphyrin) 9water soluble heterobimetallic coordination conjugate in good yields. We are currently working on the development of new porphyrin and tetraazamacrocycle architectures by changing the nature and the number of the substituents around the porphyrin macro-ring. For example, it is possible to fine-tune the properties of the bimodal ligand, by adding e.g. hydro-soluble groups or other complexing agents. We can also introduce one grafting function for biological vectorization or surface anchoring. Other possibilities (e.g.CuI-catalyzed Huisgen cycloaddition, also called “Click Chemistry”)38 are being explored in order to interconnect the two chelating subunits in a final step (after metalation of both DOTA-like and COMPOUND LINKS
Read more about this on ChemSpider
Download mol file of compoundporphyrin macrocycles for example). The synthesis of those new architectures and evaluation of their relaxivities are currently in progress and will be reported in due course.
Acknowledgements
We are really thankful to Dr Frédéric Boschetti and CheMatech Company for furnishing the DOTA-tris(tBu)ester precursor, to Mélanie Bourdillon and Dr Michel Meyer for the ICP-AES analyses, to Bruker Optics for the T1 measurements (at 20 and 60 MHz) and to Marie-José Penouilh for the HR-MS mass spectra. Support was provided by the CNRS, the “Université de Bourgogne” and the “Conseil Régional de Bourgogne” through the 3MIM integrated project (“Marquage de Molécules par les Métaux pour l'Imagerie Médicale”). The French Ministry of Research is acknowledged for PhD scholarship (AE).
Notes and references
- P. Caravan, J. J. Ellison, T. J. McMurry and R. B. Lauffer, Chem. Rev., 1999, 99, 2293–2352 CrossRef CAS.
- E. Terreno, D. D. Castelli, A. Viale and S. Aime, Chem. Rev., 2010, 110, 3019–3042 CrossRef CAS.
- E. J. Werner, A. Datta, C. J. Jocher and K. N. Raymond, Angew. Chem., Int. Ed., 2008, 47, 8568–8580 CrossRef CAS.
- L. Frullano, J. Rohovec, J. A. Peters and C. F. G. C. Geraldes, Top. Curr. Chem., 2002, 221, 25–64 CAS.
- H. Gries, Top. Curr. Chem., 2002, 221, 1–24 CAS.
- V. Jacques and J. F. Desreux, Top. Curr. Chem., 2002, 221, 123–164 CAS.
-
A. E. Merbach and E. Toth, The chemistry of contrast agents in medical magnetic resonance imaging, John Wiley & Sons, Ltd, New York, 2001 Search PubMed.
- D. D. Schwert, J. A. Davies and N. Richardson, Top. Curr. Chem., 2002, 221, 123–164 CAS.
- M. Shokeen and C. J. Anderson, Acc. Chem. Res., 2009, 42, 832–841 CrossRef CAS.
- T. J. Wadas, E. H. Wong, G. R. Weisman and C. J. Anderson, Chem. Rev., 2010, 110, 2858–2902 CrossRef CAS.
- S. M. Ametamey, M. Honer and P. A. Schulbiger, Chem. Rev., 2008, 108, 1501–1516 CrossRef CAS.
- M. Fani, J. P. André and H. R. Maecke, Contrast Media Mol. Imaging, 2008, 3, 67–77 Search PubMed.
- T. J. Wadas, E. H. Wong, G. R. Weisman and C. J. Anderson, Curr. Pharm. Des., 2007, 13, 3–16 CrossRef CAS.
- S. Liu, Adv. Drug Delivery Rev., 2008, 60, 1347–1370 CrossRef CAS.
- S. Liu and D. S. Edwards, Bioconjugate Chem., 2001, 12, 7–34 CrossRef CAS.
- S. Murugesan, S. J. Shetty, T. S. Srivastava, O. P. D. Noronha and A. M. Samuel, Appl. Radiat. Isot., 2001, 55, 641–646 CrossRef CAS.
- Y. Ni, Curr. Med. Imaging Rev., 2008, 4, 96–112 Search PubMed.
- K. Tanaka and K. Fukase, Org. Biomol. Chem., 2008, 6, 815–828 RSC.
- D. W. Townsend, Phys. Med. Biol., 2008, 53, R1–R39 CrossRef CAS.
- N. Yicheng, Curr. Med. Imaging Rev., 2008, 4, 96–112 Search PubMed.
- Z. Zhang, K. Liang, S. Bloch, M. Berezin and S. Achilefu, Bioconjugate Chem., 2005, 16, 1232–1239 CrossRef CAS.
- D. D. Dischino, E. J. Delaney, J. E. Emswiler, G. T. Gaughan, J. S. Prasad, S. K. Srivastava and M. F. Tweedle, Inorg. Chem., 1991, 30, 1265–1269 CrossRef CAS.
- A. Beeby, R. S. Dickins, S. FitzGerald, L. J. Govenlock, C. L. Maupin, D. Parker, J. P. Riehl, G. Siligardi and J. A. G. Williams, Chem. Commun., 2000, 1183–1184 RSC.
- C. Comte, C. P. Gros, R. Guilard, R. G. Khoury and K. M. Smith, J. Porphyrins Phthalocyanines, 1998, 2, 377–382 CrossRef CAS.
-
S. Koeller, Ph.D. Thesis, Université de Bourgogne, 1993.
- N. Foster, D. V. Woo, F. Kaltovich, J. Emrich and C. Ljungquist, J. Nucl. Med., 1985, 756–760 CAS.
- B. Ma, G. Li, P. Kanter, D. Lamonica, Z. Grossman and R. K. Pandey, J. Porphyrins Phthalocyanines, 2003, 7, 500–507 CAS.
- A. Takai, M. Chkounda, A. Eggenspiller, C. P. Gros, M. Lachkar, J.-M. Barbe and S. Fukuzumi, J. Am. Chem. Soc., 2010, 132, 4477–4489 CrossRef CAS.
- G. Brunisholz and M. Randin, Helv. Chim. Acta, 1959, 42, 1927–1938 CrossRef CAS.
- J.-M. Barbe, G. Canard, S. Brandès and R. Guilard, Eur. J. Org. Chem., 2005, 21, 4601–4611 CrossRef.
- A. Takaï, C. P. Gros, J.-M. Barbe and S. Fukuzumi, Phys. Chem. Chem. Phys., 2010, 12, 12160–12168 RSC.
- E. Valeur and M. Bradley, Chem. Soc. Rev., 2009, 38, 606–631 RSC.
- E. Toth, L. Helm and A. E. Merbach, Top. Curr. Chem., 2002, 221, 61–102 CAS.
- M. F. Ferreira, A. F. Martins, J. A. Martins, P. M. Ferreira, E. Tóth and C. F. G. C. Geraldes, Chem. Commun., 2009, 42, 6475–6477 Search PubMed.
- P. Hermann, J. Kotek, V. Kubíček and I. Lukeš, Dalton Trans., 2008, 3027–3047 RSC.
- A. Nonat, M. Giraud, C. Gateau, P. H. Fries, L. Helm and M. Mazzanti, Dalton Trans., 2009, 8033–8046 RSC.
- F. Hindré, M. Le Plouzennec, J. D. de Certaines, M. T. Foultier, T. Patrice and G. Simonneaux, J. Magn. Reson. Imaging, 1993, 3, 59–65 CrossRef CAS.
-
J. Lahann, Click Chemistry for Biotechnology and Materials Science, Wiley-VCH, Chichester, 2009 Search PubMed.
|
This journal is © The Royal Society of Chemistry 2011 |