DOI:
10.1039/C0MD00177E
(Concise Article)
Med. Chem. Commun., 2011,
2, 819-823
2-Anilinonicotinyl linked COMPOUND LINKS
Read more about this on ChemSpider
Download mol file of compound1,3,4-oxadiazole derivatives: Synthesis, antitumour activity and inhibition of tubulin polymerization†
Received
5th October 2010
, Accepted 12th February 2011
First published on 19th May 2011
Abstract
A series of 2-anilinonicotinyl linked 1,3,4-oxadiazoles was synthesized and evaluated for their antitumour activity against various cancer cell lines, inhibition of tubulin polymerization and cell cycle effects. Some of these compounds showed good antiproliferative activity with GI50 values ranging from 4.57 to 97.09 μM in the human cancer cell lines and one of the compounds 5m showed potent antitumour efficacy in all the cell lines tested. This compound also inhibited tubulin polymerization under both in vitro and in vivo conditions. Analysis of tubulin by Western blot experiments demonstrated that 5m depolymerizes microtubules by causing disturbances in the ratio of soluble versus polymerized tubulin in cells, leading to the cell cycle arrest at G2/M phase of the cell cycle followed by activation of caspase-3 activity and apoptotic cell death.
Introduction
There has been considerable interest in the development of novel small molecules which affect the tubulin polymerization.1 Recent studies indicated that the antitumour effect of several compounds correlated with their apoptosis inducing ability.2Microtubules are composed of dynamic polymers of tubulin involved in cellular processes, especially in induction of apoptosis.3 Rapidly dividing cells are more susceptible to tubulin polymerization inhibitors than non-dividing cells and impair microtubule dynamics and consequently arrest cells during mitosis.3 It is known that caspases, a cysteine protease family of proteins, play a critical role for the initiation and execution of apoptosis.4 Among the caspases, caspase-3, -6, and -7 are key effector caspases that cleave multiple protein substrates in cells leading to irreversible apoptotic cell death.5 The hallmark of microtubule-interfering drugs is mitotic arrest that eventually leads to apoptosis.6 The mode of action of tubulin inhibitors is that they bind to the tubulin binding sites thereby stabilizing or destabilizing microtubule assembly. Disruption of microtubule leads to cell cycle arrest at G2/M phase followed by apoptotic cell death.7 Various naturally occurring compounds which act as tubulin targeting agents are currently in clinical trials, which stabilize or destabilize microtubule by specifically binding to anyone of the three reported binding sites.8 COMPOUND LINKS
Read more about this on ChemSpider
Download mol file of compoundCombretastatin A-4 (CA4), a naturally occurring compound which possesses antitubulin properties is currently in phase I/II clinical trials against solid tumours.9 E7010 (ABT-751, 1, Fig. 1), a novel sulfonamide has shown to block cell cycle at mitosis by inhibition of tubulin polymerization.10 E7010 reversibly binds to the colchicine binding site of α-tubulin and displays antitumour activity against several types of human tumour xenographs.11,12 This compound showed good in vivo activity against rodent and human tumours13 and reached phase II clinical studies.14
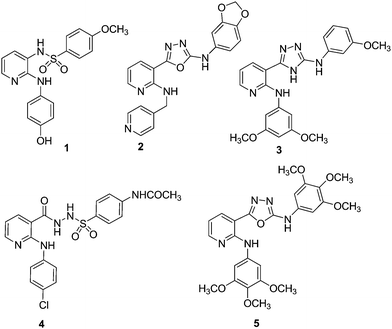 |
| Fig. 1 Chemical structures of E7010 (1), anilino-1,3,4-oxadiazole (2), anilino-1,3,4-triazoles (3), anilino sulfonyl hydrazide (4), 2-anilino-nicotinyl 1,3,4-oxadiazoles (5). | |
Oxadiazoles are known to possess a broad spectrum of biological activities15,16 including anticancer activity.17,18 A number of COMPOUND LINKS
Read more about this on ChemSpider
Download mol file of compoundoxadiazole derivatives depending upon the substituents and positions of heteroatoms namely 1,2,4- and 1,3,4-oxadiazoles are known to have anticancer effects.19–21 Recently, the potent anticancer activity of novel indolyl-1,3,4-oxadiazoles is disclosed22 and nicotinyl linked 1,3,4-oxadiazoles (2) exhibit anticancer activity through tubulin polymerization inhibition,23 and more recently these compounds are reported as a new class of dual vascular endothelial growth factor receptors 2 (VEGFR-2) kinase and tubulin inhibitors.24
In addition, another series of 1,3,4-oxadiazole derivatives are reported to exhibit antiproliferative, antimitotic, and microtubule destabilizing activity profiles comparable to that of COMPOUND LINKS
Read more about this on ChemSpider
Download mol file of compoundcombretastatin, COMPOUND LINKS
Read more about this on ChemSpider
Download mol file of compoundpodophyllotoxin and COMPOUND LINKS
Read more about this on ChemSpider
Download mol file of compoundnocodazole.25 Moreover, structurally related 2-anilinonicotinyl-1,3,4-triazoles (3) are also known to display anticancer and tubulin inhibitory effects.26,27 In continuation to our previous studies28–33 in search of potent anticancer agents, 2-anilinonicotinyl linked 1,3,4-oxadiazoles (5) were prepared by modifying the 2-anilinonicotinyl linked sulfonyl hydrazide scaffold (4),34 by the incorporation of an oxadiazole ring system. These were evaluated for their in vitro antiproliferative activity, tubulin polymerization and cell cycle as well apoptotic effects.
Results and discussion
Chemistry
COMPOUND LINKS
Read more about this on ChemSpider
Download mol file of compound2-Chloronicotinic acid ethylester (6) and substituted anilines (7a–d) were refluxed in COMPOUND LINKS
Read more about this on ChemSpider
Download mol file of compoundethylene glycol to provide the coupled product of COMPOUND LINKS
Read more about this on ChemSpider
Download mol file of compound2-anilinonicotinic acid esters (8a–d),35 which on treatment with COMPOUND LINKS
Read more about this on ChemSpider
Download mol file of compoundhydrazine hydrate in COMPOUND LINKS
Read more about this on ChemSpider
Download mol file of compoundethanol afforded 2-anilinonicotinic acid hydrazides (9a–d) in quantitative yields (Scheme 1).36 Treatment of 9a–d with substituted aryl isothiocyanates afford the corresponding thiosemicarbazides (10a–m) by employing the literature procedure. The synthesis of the final products (5a–m) was carried out by the reaction of thiosemicarbazides (10a–m) in COMPOUND LINKS
Read more about this on ChemSpider
Download mol file of compoundpyridine and COMPOUND LINKS
Read more about this on ChemSpider
Download mol file of compoundtosyl chloride using THF as the solvent under reflux conditions.37
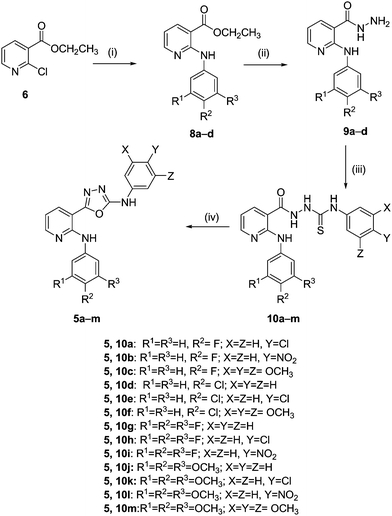 |
| Scheme 1 Reagents and conditions: (i) substituted anilines (7a–d), COMPOUND LINKS
Read more about this on ChemSpider
Download mol file of compoundethylene glycol, 160 °C, 8 h; (ii) NH2NH2·H2O, COMPOUND LINKS
Read more about this on ChemSpider
Download mol file of compoundethanol, reflux 6 h; (iii) aryl isothiocyanates, dry COMPOUND LINKS
Read more about this on ChemSpider
Download mol file of compoundbenzene, rt, 8 h; (iv) TsCl, COMPOUND LINKS
Read more about this on ChemSpider
Download mol file of compoundpyridine, THF, reflux 2–3 h. | |
Biological evaluation
(A) Antitumour activity.
The synthesized compounds 5a–m were evaluated for the antiproliferative activity against five human cancer cell lines viz., breast adenocarcinoma (MDA-MB-231), liver (HepG-2), lung adenocarcinoma (A549), cervix (HeLa) and prostate (DU-145) using sulforhodamine B (SRB) assay. Growth inhibitory activities (GI50 values) were presented as micromolar concentrations in Table 1. Results indicate that most of the compounds exhibited significant growth inhibitory effects in almost all the cell lines with GI50 values ranging from 4.57 to 97.0 μM concentrations. Keeping the 2-anilinonicotinyl ring unchanged, we explored the modifications on the oxadiazole anilino ring with electron donating and withdrawing substituents. In case of compounds 5a–c, chloro substitutent containing compound at position-4 of oxadiazole-anilino ring (5a), exhibited significant cytotoxicity against lung and cervix cancer cell lines with GI50 values of 10.6 and 8.6 μM, respectively. However, electron deficient group, 4-nitro (5b) or electron rich, 3,4,5-trimethoxy substituents (5c) completely abolished the activity. Replacement of 4-fluoro substituent with 4-chloro group or 3,4,5-trifluoro substitutients 5d–h on the nicotinyl aniline ring has shown moderate to good activity. Compound (5m) with trimethoxy substituent on both anilino rings exhibited highest potency with GI50 values ranging from 4.57–10.69 μM towards all the tested cell lines. Replacement of 3,4,5-trimethoxy groups on the oxadiazole phenyl ring with 4-nitro group (5l) showed selectivity against lung cancer cell line and cervix cancer cell line with GI50 value of 10.57 and 8.46 μM, respectively. Whereas, replacement of 3,4,5-trimethoxy groups with 4-chloro group (5k) showed selectivity against lung and cervix cancer cell line with GI50 values of 6.45 and 6.67 μM respectively. Removal of 3,4,5-trimethoxy subsituents from the anilino moiety linked to the oxadiazoles led to reduced potency of compound 5j. Compound 5m is found to be most potent with GI50 value of 4.57 μM against A549 cell line and good inhibitory activity against all the cell lines tested and interestingly all the synthesized compounds are active in HeLa cancer line with GI50 values ranging from 5.1–8.6 μM. COMPOUND LINKS
Read more about this on ChemSpider
Download mol file of compoundNocodazole, which was used as a positive control in this assays showed <1 μM GI50 values in all the cell lines whereas, E7010 exhibited GI50 values of 8.51 and 5.25 μM, against MDA-MB-231 and HepG-2 cell lines, respectively.38 Overall, the results demonstrate that eight (5a, 5d, 5e, 5g, 5h, 5i, 5j & 5m) out of thirteen compounds exhibited good antitumour properties in at least three cell lines out of five tested. Although the focus of the present investigation is to identify the antitubulin agents, there are other potential antitumour compounds in this series (5a, 5d, 5e, 5g, 5h, 5i & 5j) whose mechanism of action is not yet known and further studies are in progress to identify their primary site of action.
Table 1
GI50 valuesa (μM) of compounds 5a–m and their CLogPb values
Compd |
MDA-MB-231
c |
HepG-2d |
A549
e |
HeLaf |
DU-145
g |
ClogP |
Each data represents mean ±S.D. from three different experiments performed in triplicates.
ClogP values calculated using ChemDrawUltra 11.0.
MDA-MB-231: human breast adenocarcinoma cell line.
HepG-2: human liver cancer cell line.
A549: human lung adenocarcinoma epithelial cell line.
HeLa: human cervix cancer cell line.
DU-145: human prostate cancer cell line.
GI50 value not attained at the concentration used.
|
5a
|
>100 |
53.4 ± 7.76 |
10.62 ± 0.78 |
8.6 ± 0.98 |
>100 |
5.82 |
5b
|
—h |
—h |
—h |
—h |
—h |
4.89 |
5c
|
—h |
—h |
—h |
—h |
—h |
4.23 |
5d
|
>100 |
17.01 ± 1.47 |
8.78 ± 1.43 |
5.38 ± 0.49 |
26.61 ± 2.34 |
5.66 |
5e
|
97.09 ± 10.56 |
20.54 ± 1.83 |
14.34 ± 2.1 |
6.99 ± 0.84 |
15.99 ± 2.32 |
6.39 |
5f
|
58.62 ± 7.6 |
45.03 ± 3.92 |
—h |
—h |
11.90 ± 1.84 |
4.80 |
5g
|
20.67 ± 2.72 |
11.16 ± 1.62 |
5.73 ± 0.76 |
5.1 ±.73 |
9.83 ± 1.63 |
5.25 |
5h
|
>100 |
29.34 ± 2.32 |
12.32 ± 1.93 |
7.62 ± 1.04 |
12.3 ± 1.83 |
5.98 |
5i
|
28.17 ± 3.4 |
11.59 ± 0.84 |
10.64 ± 1.56 |
7.96 ± 1.22 |
10.18 ± 1.36 |
4.39 |
5j
|
15.42 ± 2.16 |
13.82 ± 1.74 |
12.63 ± 1.67 |
6.94 ± 0.87 |
12.52 ± 1.64 |
4.05 |
5k
|
—h |
—h |
6.45 ± 0.87 |
6.67 ± 0.92 |
11.86 ± 1.46 |
4.78 |
5l
|
—h |
—h |
10.57 ± 10.87 |
8.46 ± 1.45 |
—h |
3.85 |
5m
|
10.69 ± 1.34 |
5.57 ± 0.64 |
4.57 ± 0.42 |
5.55 ± 0.63 |
7.06 ± 0.96 |
3.19 |
Nocodazole
|
0.15 |
0.76 |
0.21 |
0.75 |
0.19 |
— |
These compounds possess aqueous solubility (CLogP) in the range of 3.19–6.39. Compounds having CLogP values greater than 4 displayed weak or moderate cytotoxic effect, whereas compound 5m with lowest CLogP value of 3.19 showed the most potent cytotoxicity in this series.
(C)
Cell cycle analysis and apoptosis (Flow cytometry).
The compounds that exhibit effects on tubulin assembly are known to cause the alteration of cell cycle parameters with preferential G2/M blockade, therefore flow cytometry analysis was carried out to determine the effect of these compounds. A549 cells were exposed to 2 μM concentration of compounds 5a–m along with positive control nocadazole at 1 μM for 48 h, The results demonstrate that while the distribution of cells at G1, S and G2/M phase were 78%, 10% and 10% in controls, the values were found to be 65%, 15% and 19% respectively in cells treated with 2 μM of compound 5m. Similar effects were observed when cells were treated with the positive control, nocadazole, where the distributions of cells were found to be 55%, 25% and 18% for G1, S, G2/M. Under similar experimental conditions the compound 5k did not induce cell cycle arrest at G2/M phase (see ESI, Table 2†). The effect of 5m on cell cycle arrest was found to be dose dependent, as treatment of A549 cells with compound 5m at 1, 2 and 4 μM increased the percentage of cells at G2/M phase to 20, 24, & 29 respectively (see ESI, Table 3†). The data indicate that the induction of cellular apoptosis by 5m is probably by virtue of its inhibitory effect on tubulin polymerization as there was an increase in cell cycle arrest at metaphase. In brief, the effect of compound 5m on cell cycle progression correlated with its antiproliferative activity and tubulin inhibition.
(D) Analysis of free versus polymerized tubulin by Western blotting.
In order to extend the in vitro effects of the compounds on tubulin polymerization to the cellular effects, Western blot analysis of α-tubulin in A549 cells was performed by treating COMPOUND LINKS
Read more about this on ChemSpider
Download mol file of compoundnocodazole (1 μM), COMPOUND LINKS
Read more about this on ChemSpider
Download mol file of compoundtaxol (1 μM) and different concentrations of 5m (0.1 to 10 μM) for 24 h. Free and polymerized tubulin was isolated as described in the general procedure (see ESI†). Results indicate that while the tubulin in the supernatant fraction showed an increased level of free tubulin in a dose dependent manner after treatment with various concentrations of 5m, there was a steady decrease in the polymerized tubulin in the pellet (Fig. 3a). COMPOUND LINKS
Read more about this on ChemSpider
Download mol file of compoundTaxol,41 which was used as the negative control, stabilized the tubulin assembly as the amount of tubulin in the pellet was predominant than the cytosolic fraction. Densitometry analysis was performed for band intensities obtained from Western blot. Percentage of supernatant to pellet fractions were calculated and shown in Fig. 3b. There were nearly 2-, 6- and 10-fold increase in tubulin content of supernatant fraction of 5m at 0.1, 1 and 10 μM concentrations, respectively, compared to untreated controls. However, nocadazole treatment increased the proportion of soluble tubulin to nearly 6-fold under similar experimental conditions.
(E)
Activation of caspases.
It was of interest to understand the origin of cytotoxicity for 5m as in several instances cell cycle arrest at G2/M phase is known to induce cellular apoptosis. Caspases plays a crucial role in the induction of apoptosis4 and amongst them caspase-3 happens to be one of the effector caspase5 which is in turn activated by caspase-9. Hence, A549 cells were treated with different concentrations of 5m along with the positive control COMPOUND LINKS
Read more about this on ChemSpider
Download mol file of compoundnocodazole and examined the activation of caspase-9 and -3 as described in the procedure. Results indicate that there was nearly 1.5–4 fold induction in caspase-9 and -3 activities in cells treated with 0.5, 1.25 μM and 2.5 μM compound 5m (Fig. 4). COMPOUND LINKS
Read more about this on ChemSpider
Download mol file of compoundNocodazole (1 μM) induced caspase activities to nearly 4-fold compared to controls, under similar conditions.
Conclusion
A new series of 2-anilino substituted nicotinyl 1,3,4 oxadiazoles was synthesized and evaluated for their antiproliferative and inhibition of tubulin polymerization activities. These compounds exhibited significant antiproliferative activity with GI50 values ranging from 4.57–97.09 μM. Most of the compounds were specifically active in the cervical cancer cell line with GI50 values 5.1–8.6 μM. The lead compound 5m exhibits significant antiproliferative activity with GI50 values ranging from 4.57–10.69 μM and showed to be potent to inhibit tubulin polymerization in both in vitro assay as well as in cells as observed by the increase in the ratio of soluble to polymerized tubulin. Moreover, this compound also possesses reasonably good aqueous solubility with ClogP value of 3.19. Further studies indicated that cell cycle arrest at G2/M phase followed by the activation of caspases as the mechanism of cytoxicity for compound 5m. The compound 5m has shown comparable inhibition of tubulin polymerization to related compounds.23 These mechanistic studies provide more insight for the further development of tubulin inhibitors as potential anticancer agents.
Acknowledgements
The authors (Y.V.V.S and M.A) thank UGC and (M.K.R) thank CSIR, New Delhi, India for the award of research fellowships.
Notes and references
- F. Pellegrini and D. R. Bubman, Cancer Invest., 2005, 23, 264–273 CrossRef.
- M.-A. Belaud-Rotureau, F. Durrieu, G. Labroille, F. Lacombe, O. Fitoussi, P. Agape, G. Marit, J. Reiffers and F. Belloc, Leuk. Res., 1997, 21, 163–172 CrossRef CAS.
- M. A. Jordan, J. A. Hadfield, N. J. Lawrence and A. T. McGown, Med. Res. Rev., 1998, 18, 259–296 CrossRef CAS.
- S. Kumar, Cell Death Differ., 2007, 14, 32–43 CrossRef CAS.
- N. A. Thornberry, Chem. Biol., 1998, 5, R97–R103 Search PubMed.
- R. O. Carlson, Expert Opin. Invest. Drugs, 2008, 17, 707–722 Search PubMed.
- E. Pasquier and M. Kavallaris, IUBMB Life, 2008, 60, 165–170 Search PubMed.
- M. Kavallaris, Nat. Rev. Cancer, 2010, 10, 194–204 Search PubMed.
- J. K. Buolamwini, Curr. Opin. Chem. Biol., 1999, 3, 500–509 Search PubMed.
- N. Koyanagi, T. Nagasu, F. Fujita, T. Watanabe, K. Tsukahara, Y. Funahashi, M. Fujita, T. Taguchi, H. Yoshino and K. Kitoh, Cancer Res., 1994, 54, 1702–1706 CAS.
- A. Yokoi, J. Kuromitsu, T. Kawai, T. Nagasu, N. H. Sugi, K. Yoshimatsu, H. Yoshino and T. Owa, Mol. Cancer Ther., 2002, 1, 275–286 Search PubMed.
- K. Yoshimatsu, A. Yamaguchi, H. Yoshino, N. Koyanagi and K. Kitoh, Cancer Res., 1997, 57, 3208–3213 CAS.
- M. Kavallaris, N. M. Verrills and B. T. Hill, Drug Resist. Updates, 2001, 4, 392–401 Search PubMed.
- A. M. Mauer, E. E. Cohen, P. C. Ma, M. F. Kozloff, L. Schwartzberg, A. I. Coates, J. Qian, A. E. Hagey and G. B. Gordon, J. Thorac. Oncol., 2008, 3, 631–636 Search PubMed.
- M. Islam, A. A. Siddiqui, R. Rajesh, A. Bakht and S. Goyal, Acta. Pol. Pharm., 2008, 65, 441–447 Search PubMed.
- R. Chawla, A. Arora, M. K. Parameswaran, P. Chan, D. Sharma, S. Michael and T. K. Ravi, Acta. Pol. Pharm., 2010, 67, 247–253 Search PubMed.
- D. Kumar, G. Patel, E. O. Johnson and K. Shah, Bioorg. Med. Chem. Lett., 2009, 19, 2739–2741 Search PubMed.
- H. Z. Zhang, S. Kasibhatla, J. Kuemmerle, W. Kemnitzer, K. Ollis-Mason, L. Qiu, C. Crogan-Grundy, B. Tseng, J. Drewe and S. X. Cai, J. Med. Chem., 2008, 48, 5215–5223 Search PubMed.
- W. Kemnitzer, J. Kuemmerle, H. Z. Zhang, S. Kasibhatla, B. Tseng, J. Drewe and S. X. Cai, Bioorg. Med. Chem. Lett., 2009, 19, 4410–4415 Search PubMed.
- A. Kumar, S. S. D'Souza, S. L. Gaonkar, K. M. Rai and B. P. Salimath, Invest. New Drugs, 2008, 26, 425–435 CrossRef CAS.
- K. A. Jessen, N. M. English, J. Yu Wang, S. Maliartchouk, S. P. Archer, L. Qiu, R. Brand, J. Kuemmerle, H. Z. Zhang, K. Gehlsen, J. Drewe, B. Tseng, S. X. Cai and S. Kasibhatla, Mol. Cancer Ther., 2005, 4, 761–771 CrossRef CAS.
- D. Kumar, S. Sundaree, E. O. Johnson and K. Shah, Bioorg. Med. Chem. Lett., 2009, 19, 4492–4494 Search PubMed.
- X. Ouyang, E. L. Piatnitski, V. Pattaropong, X. Chen, H. He, A. S. Kiselyov, A. Velankar, J. Kawakami, M. Labelle, L. Smith., J. Lohman, S. P. Lee, A. Malikzay, J. Fleming, J. Gerlak, Y. Wang, R. L. Rosler, K. Zhou, S. Mitelman and M. Camara, Bioorg. Med. Chem. Lett., 2006, 16, 1191–1196 CrossRef CAS.
- E. L. Piatnitski, A. S. Kiselyov, X. Ouyang, X. Chen, V. Pattaropong, Y. Wang, M. C. Tuma and J. F. Doody, ACS Med. Chem. Lett., 2010 DOI:10.1021/ml1001568.
- A. S. Kiselyov, M. N. Semenova, N. B. Chernyshova, A. Leitao, A. V. Samet, K. A. Kislyi, M. M. Raihstat, T. Oprea, H. Lemcke, M. Lantow, D. G. Weiss, N. N. Ikizalp, S. A. Kuznetsov and V. V. Semenov, Eur. J. Med. Chem., 2010, 45, 1683–1697 Search PubMed.
- X. Ouyang, X. Chen, E. L. Piatnitski, A. S. Kiselyov, H. Y. He, Y. Mao, V. Pattaropong, Y. Yu, K. H. Kim, J. Kincaid, L. I. I. Smith, W. C. Wong, S. P. Lee, D. L. Milligan, A. Malikzay, J. Fleming, J. Gerlak, D. Deevi, J. F. Doody and H. Chiang, Bioorg. Med. Chem. Lett., 2005, 15, 5154–5159 Search PubMed.
- J. A. Stefely, R. Palchaudhuri, P. A. Miller, R. J. Peterson, G. C Moraski, P. J. Hergenrother and M. J. Miller, J. Med. Chem., 2010, 53, 3389–3395 Search PubMed.
- A. Kamal, K. S. Reddy, M. N. A. Khan, V. C. R. N. C. Rajesh, M. J. Ramaiah, S. N. C. V. L. Pushpavalli, C. Srinivas, M. P. Bhadra, M. Chourasia, G. N. Sastry, A. Juvekar, S. Zingde and M. Barkume, Bioorg. Med. Chem., 2010, 18, 4747–4761 Search PubMed.
- A. Kamal, J. S. Reddy, M. J. Ramaiah, D. Dastagiri, E. V. Bharathi, M. A. Azhar, F. Sultana, S. N. C. V. L. Pushpavalli, M. P. Bhadra, A. Juvekar, S. Sen and S. Zingde, Eur. J. Med. Chem., 2010, 45, 3924–3937 Search PubMed.
- A. Kamal, G. Balakishan, G. Ramakrishna, T. B. Shaik, K. Sreekanth, M. Balakrishna, D. Rajender, D. Dastagiri and S. V. Kalivendi, Eur. J. Med. Chem., 2010, 45, 3870–3884 Search PubMed.
- A. Kamal, G. Ramakrishna, P. Raju, A. Viswanath, M. J. Ramaiah, G. Balakishan and M. P. Bhadra, Bioorg. Med. Chem. Lett., 2010, 20, 4865–4869 Search PubMed.
- A. Kamal, M. N. A. Khan, Y. V. V. Srikanth and S. V. C. R. N. C. Rajesh, Chem. Biol. Drug Des., 2009, 73, 687–693 Search PubMed.
- A. Kamal, M. N. A. Khan, Y. V. V. Srikanth, K. S. Reddy, A. Juvekar, S. Sen, N. Kurian and S. Zingde, Bioorg. Med. Chem., 2008, 16, 7804–7810 Search PubMed.
- A. Kamal, M. N. A. Khan, K. S. Reddy and K. Rohini, Bioorg. Med. Chem., 2007, 15, 1004–1013 Search PubMed.
- R. Jouhari and P. Quinn, Heterocycles, 1994, 38, 2243–2246 Search PubMed.
- T. I. Fand and P. E. Spoerri, J. Am. Chem. Soc., 1952, 74, 1345 Search PubMed.
- S. J. Dolman, F. Gosselin, P. D. O'Shea and I. W. Davies, J. Org. Chem., 2006, 71, 9548–9551 Search PubMed.
- N. Nakatsu, Y. Yoshida, K. Yamazaki, T. Nakamura, S. Dan, Y. Fukui and T. Yamori, Mol. Cancer Ther, 2005, 4, 399–412 Search PubMed.
- D. Bonne, C. Heusele, C. Simon and D. Pantaloni, J. Biol. Chem., 1985, 260, 2819–2825 CAS.
- K. Huber, P. Patel, L. Zhang, H. Evans, A. D. Westwell, P. M. Fischer, S. Chan and S. Martin, Mol. Cancer Ther., 2008, 7, 143–151 Search PubMed.
- H. Tu, A. Huang, T. Hour, S. Yang, Y. Pu and C. Lin, Bioorg. Med. Chem., 2010, 18, 2089–2098 Search PubMed.
Footnote |
† Electronic supplementary information (ESI) available: Spectral data of compounds 8a–d, 9a–d, 10a–m and 5a–m and experimental procedures for synthesis and biological evaluations. See DOI: 10.1039/c0md00177e |
|
This journal is © The Royal Society of Chemistry 2011 |