DOI:
10.1039/C0MD00175A
(Concise Article)
Med. Chem. Commun., 2011,
2, 371-377
Glycosylated porphyrin derivatives and their photodynamic activity in cancer cells†
Received
2nd October 2010
, Accepted 28th December 2010
First published on 11th February 2011
Abstract
The present study reports the design and synthesis of nine C2-symmetric 5,15-[bis(arayl)]-10α,20β-[bis(1,2:3,4-di-O-isopropylidene-α-D-galactopyranose-6-yl)]porphyrins (3–11) bearing electron donating or electron withdrawing substituents and a D2-symmetric 5α,10β,15α,20β-tetrakis(1,2:3,4-di-O-isopropylidene-α-D-galactopyranose-6-yl)porphyrin (12). In the system we design, the C6 of pyranose sugar is elegantly fused into the porphyrin core as mesocarbon, which renders a new type of photodynanic inducers. The biological effects of these derivatives were assessed in HeLa and HCT116 human cancer cells. In particular, the tetra-glycofused structure 12 exhibited the highest cellular uptake and photocytotoxicity. Unlike the reported sugar-porphyrin conjugates, which normally localize in mitochondria or endoplasmic reticulum, the unique glycofused porphyrins in this study were dominantly localized in lysosomes. The measurement of the dual flurorescence of annexin V-FITC/PI by flow cytometry revealed that the cell death was caused by apoptosis. Further PARP cleavage study suggested that apoptosis induced by the treatment of compound 12 was via caspase-dependent apoptotic pathway in cancer cells.
Introduction
Photodynamic therapy (PDT)1 is a rapidly growing method used to treat various cancers including multidrug resistance2 (MDR) phenotype tumor cells by using non-toxic photosensitizers (PSs) and innocuous visible light in the presence of molecular oxygen. This technique is based on the generation of cytotoxic reactive oxygen species (ROS) by a PS under light irradiation.3 Currently, a few potent PSs such as porphyrins,4phthalocyanines,5COMPOUND LINKS
Read more about this on ChemSpider
Download mol file of compoundperylene,6chlorin derivatives7 are commonly used in photodynamic therapy. They are suitable PSs due to their light absorption in the visible range of spectrum, but early generation of these molecules has obvious drawbacks such as low tissue selectivity, low sensitizing efficiency, low solubility, high systemic toxicity, etc. Therefore, the development of new PS that targets the abnormal cells selectively and generates cytotoxic ROS efficiently is one of the current strategies that are being explored.
Conjugation of COMPOUND LINKS
Read more about this on ChemSpider
Download mol file of compoundporphyrin with cancer cell recognizing biomolecules is an active area receiving much attention, especially the use of biological active sugar motifs as a conjugate. Previous studies described the roles of saccharides in cell recognition, with COMPOUND LINKS
Read more about this on ChemSpider
Download mol file of compoundporphyrin-saccharide derivatives exhibiting much higher binding affinity to human cancer cell lines than their non-saccharide counterparts,8,9 with the sugar moieties enhancing uptake by cancer cells. Intelligibly, such glycoconjugate porphyrin is thus a potential avenue for targeted photosensitizers towards tumor cells.8 In this work, we aim to develop a new series of directly linked sugar-COMPOUND LINKS
Read more about this on ChemSpider
Download mol file of compoundporphyrin conjugates and investigate their potential phototoxicity, cellular localization studies, and in vitro apoptotic activities.
Results and discussion
Rational design of glycofused porphyrins as PSs
COMPOUND LINKS
Read more about this on ChemSpider
Download mol file of compoundPorphyrin derivatives are commonly used PSs due to their light absorption in the visible range of the spectrum and efficient phototoxicity towards cancer cells.10 In the past decade, great efforts have been made to search for more efficient photosensitizing molecules by modification of the porphyrin core and peripheral structure of phthalocyanines. Not surprisingly, tumor-recognizing elements, such as monoclonal antibodies have also been extensively explored to gain tissue selectivity and reduce systemic toxicity. Synthesis of sugar-COMPOUND LINKS
Read more about this on ChemSpider
Download mol file of compoundporphyrin conjugates were reported sporadically, but in most cases, the biologically active sugars were included into the peripheral structures with a linker between sugar moiety and the photosensitizing core structure (such as Fig. 1, structure A). Van Nostrum and coworkers demonstrated the peripheral and axial substitution of phthalocyanines with COMPOUND LINKS
Read more about this on ChemSpider
Download mol file of compoundsolketal protected sugar groups (Fig. 1, structure B) facilitates an increased cellular uptake of cancer cells.11 The COMPOUND LINKS
Read more about this on ChemSpider
Download mol file of compoundsolketal group was thought to act as a targeted micelle, resulting in a higher intracellular concentration of the PS and a concomitant increase in photodynamic effect.
This biocompatible sugar unit is used to enhance the cellular uptake through over-expressed COMPOUND LINKS
Read more about this on ChemSpider
Download mol file of compoundglucose transporters in cancer cells.12 The isopropylidene protecting group renders the compound metabolically stable and increase the cell availability of phthalocyanine conjugate that can be cleaved in vivo to form hydrophilic free hydroxyl units.13 The molecular design in this study was also inspired by a naturally occurring sugar-porphyrin conjugate, tolylporphyrin A14 (Fig. 1, structure C). It has a C2 symmetric structural skeleton and the sugar moieties are directly linked to COMPOUND LINKS
Read more about this on ChemSpider
Download mol file of compoundporphyrin. This structural skeleton has the ability to reverse the multidrug resistance (MDR) tumor cells.2,14 This suggests that conjugating pattern of sugar moieties with porphyrin core also plays an important role for their biological activity. Based on all above and our experience in carbohydrate chemistry and molecular design,15 we designed the glycofused porphyrins as shown in Fig. 1D. In structure D, two para meso positions of porphyrin structure were elegantly fused with the C6 of isopropylidene protected galactose, and the other two para meso postions of the porphyrin structure were still decorated with aryl substitutions (3–11). The potential efficacy of our design is also strongly supported by Banfi et al. and Ferrand et al. works.4 They showed that diaryl porphyrin derivatives are more effective than corresponding tetraaryl porphyrin derivatives in inducing photodynamic cell elimination of human colon adenocarcinoma cells. In addition, two para meso positions of porphyrin structure were incorporated with similar sugar units, giving us tetrasugar porphyrin (12). We decided to choose COMPOUND LINKS
Read more about this on ChemSpider
Download mol file of compoundgalactose as a model sugar because Griegel and co-workers16 recognized that human retinoblastoma cells express sugar receptors that exhibits a preferential affinity for galactose residues and renders easy assimilation.
Synthesis of glycofused porphyrins
Among the various resources from which a porphyrin ring can be constructed, the acid-catalyzed condensation of dipyrrylmethane units with aryl aldehydes represents a widely used route, which we have exploited to obtain meso bis-glycosylated diarylporphyrins in this study. This approach takes advantage of the accessibility of homochiral dinuclear C-glycosyl dipyrrylmethane unit by a protocol involving the direct condensation of sugar aldehyde with COMPOUND LINKS
Read more about this on ChemSpider
Download mol file of compoundpyrrole (Scheme 1). Thus, the condensation of 1,2:3,4-di-O-isopropylidene-α-D-galacto-hexadialdo-1,5-pyranose (1) with COMPOUND LINKS
Read more about this on ChemSpider
Download mol file of compoundpyrrole (1
:
5 molar ratio) and 0.1 equiv of BF3·Et2O was effected in COMPOUND LINKS
Read more about this on ChemSpider
Download mol file of compounddichloromethane at room temperature. The reaction was completed within 1 h, after quenching with NaHCO3(aq) and flash chromatography, the dipyrrylmethane sugar unit was obtained in 65% isolated yield. With significant quantity of dipyromethane sugar in hand, we proceeded to construct the macrocycle. The condensation of the 1,2:3,4-di-O-isopropylidene-5,5-dipyrryl-6-deoxy-α-D-galactopyranose (2) with various aromatic aldehydes and sugar aldehyde (1) was performed according to the procedures developed by Casiraghi and coworkers.17 The porphyrin-ring construction was carried out by using various aldehydes with COMPOUND LINKS
Read more about this on ChemSpider
Download mol file of compounddipyrryl methane (2) and BF3·Et2O in dry COMPOUND LINKS
Read more about this on ChemSpider
Download mol file of compounddichloromethane under argon atmosphere. The porphyrinogen intermediate was then oxidized by DDQ and further purification by flash chromatography yielded porphyrins 3–12 ranging from yields of 5% to 16% (Scheme 1). All the compounds are highly soluble in common organic solvents due to the protection with isopropylidene groups.
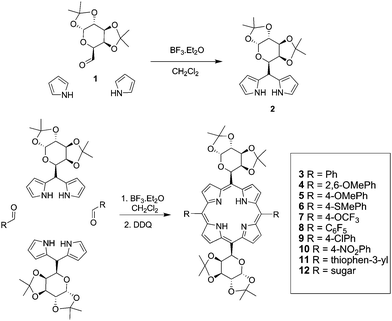 |
| Scheme 1 Expedited synthesis of glycofused porphyrin conjugates. | |
Characterization and spectral properties
The 1H and 13C NMR spectra of 1,2:3,4-di-O-isopropylidene-5,5-dipyrryl-6-deoxy-α-D-galactopyranose (2) displayed distinct peaks for the pyrrole methyne proton and proton an carbon owing to the diastereotopicity of the two pyrrole units attached at the homochiral sugar fragment. The porphyrin conjugates 3–12 were subjected to various spectral analyses, including lH NMR, 13C NMR, mass spectrometry, UV-Vis, and IR spectroscopy. All the compounds are homogeneous and have reliable spectral values. From the 1H NMR spectra of all the porphyrin compounds, it was observed that the ring system is highly conjugated and aromatic. In general, the protons at the following positions are responsible for the signals in the indicated regions of the spectra (Fig. 1S): (a) pyrroleββ′-protons (10.5 and 8.5 ppm), (b) mesophenyls and other aromatic protons (8.5 to 7.0 ppm), (c) sugar C-5′ protons (7.7 to 6.8 ppm), (d) sugar C-1′ anomeric proton (6.5 to 6 ppm), (e) other protons on the sugar legs (5.5 to 4.5 ppm), (e) four different methyl groups present in the isopropylidene groups (2.0 to 1.0 ppm), (f) characteristic NH proton (−2 to −3 ppm). The data suggest the slight changes in the chemical shift towards more deshielded region for the sugar methylenic proton as compared to the expected shift because it is directly linked with highly conjugated aromatic system. The diaryl sugar porphyrin (3–11) displayed two types of β,β′-pyrrole protons and five signals effect from sugar methylenic protons, thereby proving the presence of C2 symmetry. Also, the integral value of the methylenic proton sugar unit illustrates a highly C2 symmetric nature of the compounds. In contrast, compound 12 showed eight pyrroleβ,β′-protons as a singlet and all the methylene protons of four sugars displayed only five signals which emphasizes the presence of D2 symmetry. High resolution mass spectra (HRMS) gave molecular weights which are those expected for the corresponding (M + H)+ formula and it is in good agreement (within 0.5 ppm) with the theoretical values (Table 1). In the UV-Vis spectra, the Soret bands at 403–419 nm and four Q bands at 500–620 nm showed the characteristic of a porphyrin ring (Table 1). For most of the electron donating substituents, the Soret bands were significantly red-shifted compared to the reference porphyrin compound 3. In the Q-band region, similar spectral variations were obtained for all electron withdrawing substituents compared to the reference porphyrin compound 3.
Compd |
UV-visible spectrum: λmax nm (log ε) |
HRMS (ESI): m/z (M + H) + (Calcd) |
3
|
406 (4.478), 516 (4.136), 549 (3.749), 589 (3.672), 644 (3.549) |
919.3948 (919.3918) |
4
|
419 (4.506), 518 (3.577), 550 (3.103), 590 (3.106), 645 (2.811). |
1009.3619 (1009.3620) |
5
|
416 (4.480), 516 (3.453), 548 (3.051), 590 (2.979), 644 (2.723) |
919.3138 (987.3139) |
6
|
414 (4.540), 518 (4.282), 550 (3.817), 591(3.796), 640 (3.30) |
1011.3665 (1011.3673) |
7
|
414 (4.494), 518 (3.514), 550 (3.412), 590 (3.160), 646 (2.890) |
979.4128 (979.4129) |
8
|
412 (4.533), 516 (4.205), 546 (3.607), 590 (3.768), 644 (3.526) |
1039.4347 (1039.4341) |
9
|
403 (4.538), 516 (4.211), 550 (3.827), 591(3.757), 645 (3.487) |
1087.3545 (1087.3564) |
10
|
405 (4.521), 513 (4.163), 543 (3.422), 586 (3.701), 640 (3.163) |
1099.2979 (1099.2976) |
11
|
412 (4.501), 518 (3.767), 550 (3.480), 591 (3.329), 646 (3.089). |
931.3044 (931.3047) |
12
|
406 (4.519), 519 (4.017), 552 (3.381), 591(3.591), 646(3.437) |
1223.5266 (1223.5288) |
Cellular phototoxicity
The light dose-dependent phototoxicity of the photosensitizers was investigated in two different human cancer cell lines, HeLa and HCT116 by MTS assay at a concentration of 1 μM. Among the ten compounds studied, four compounds (5, 6, 11, and 12) have shown the phototoxic effects in both cancer cell lines (Fig. 2. A and B) while the rest six compounds showed marginal phototoxicity (data not shown). In addition, those compounds exhibited a minor dark cytotoxicity in both cell lines, which maintained more than 90% of survival rate. On the other hand, the control cells irradiated in the absence of the photosensitizer were found to be negligible in cell death. These results suggest that the electron donating substituents present in the para position of the phenyl group, especially p-methoxy (5) and p-thiomethoxy(6), enhance phototoxicity, compared with the electron withdrawing groups (pentafluro, p-chloro and p-nitro, 8–10) and simple phenyl substituent (3). Similarly, 3-thiopheneyl group present in compound 11 showed good activity due to its electron donating nature. In contrast, the methoxy group present at ortho position of the phenyl ring of compound 4 and trifluromethoxy at para position of the phenyl ring of compound 7 did not show any activity. However, phenyl group replaced by sugar unit called tetra-sugar porphyrin (12) conjugates exhibited quite reasonable phototoxicity. These results further supported that the cancer cells are sensitive to the photosensitizers. The amount of the photosensitizers taken up by the cells was determined by fluorescence microscopy after 24 h treatment. As shown in Fig. 3, compounds 5 and 6 were poorly internalized by HeLa cells compared with compounds 11 and 12. Thus, the extent of uptake of the conjugates is dependent upon the nature of the sugar component and the electron donating nature of the substituent attached at meso position of the porphyrin ring. The cellular uptake of conjugates 12 was 3–8 times higher than that of COMPOUND LINKS
Read more about this on ChemSpider
Download mol file of compoundporphyrin 5, 6 & 11 at all time points studied under the same testing condition. It has been postulated that isopropyledine-protected sugar groups from the porphyrin residues play an important role in facilitating cellular uptake, probably through deprotection of solketal group and may contribute to the formation of free hydroxyl group due to the acidic environment of cancer cells.18
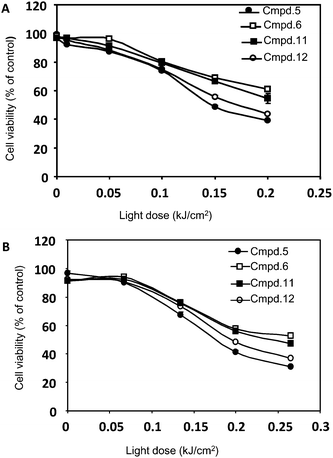 |
| Fig. 2 Dose-response curve obtained with compounds 5, 6, 11, and 12 in HeLa (A) and HCT116 (B) cells. Cells treated with 1 μM of each compound were incubated for 24 h and then irradiated using several light doses (0 to 0.25 kJ/cm2). After 24 h, viability was assessed by MTS assay. | |
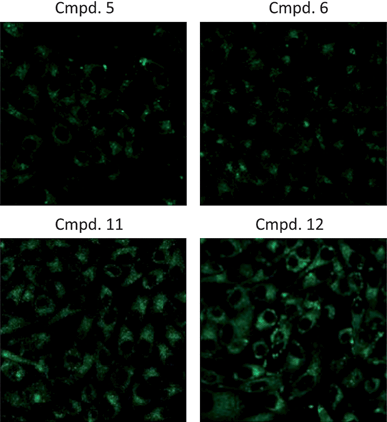 |
| Fig. 3 Cellular uptake. HeLa cells were treated with 1 μM of each compound for 24 h, rinsed, and fixed with 3.7% PFA. Fluorescence images were taken under identical conditions. Compound 12 is preferentially taken up by HeLa cells over compounds 5, 6, and 11. | |
Subcellular localization
The precise phototoxic effect of compound 12 was evaluated by examining its subcellular localization in Hela cells. To this end, its fluorescence pattern was monitored with the organelle-specific fluorescent probes LysoTracker-Red and MitoTracker-Deep Red by fluorescence microscopy, which target lysosomes and mitochondria, respectively. As shown in Fig. 4, compound 12 is primarily localized in lysosomes. In addition, the subcellular localization was also examined in HCT116 cells, showing the similar pattern as seen in Hela cells (data not shown). Turk and coworkers reported that apoptosis can be induced by selectively disrupting lysosome, through the cleavage by papain-like cathepsins independent of caspase activation.19 Several cathepsins were shown to cleave Bid and assist cytochrome c release from mitochondria in the presence of Bid in vitro, indicating their redundant roles. However, we cannot exclude the possibility that lysosomal proteases can also activate apoptosis other than Bid-mediated apoptotic pathways, prompting us to check the underlying molecular mechanism of the novel PDT compound.
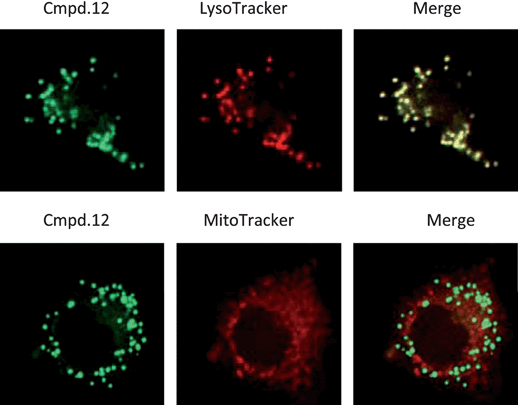 |
| Fig. 4
Intracellular localization of compounds. Subcellular localization of compound 12 determined by confocal laser scanning microscopy. HeLa cells treated with compound 12 were loaded with specific probes for lysosomes and mitochondria. Compound 12 (green) is shown in the left panels, LysoTracker or MitoTracker (Red) is shown in the middle panels, and an overlay of compound 12 with LysoTracker or MitoTracker (yellow) is shown in the right panels. | |
Studies on PDT induced apoptotic cell death
In order to delineate cell death mechanism20 in response to the treatment of compound 12, standard apoptotic assays were performed. As shown in Fig. 5, a majority of the non-illuminated cells appeared annexin V-negative section, whereas 80% of the illuminated HeLa cell population was annexin V-positive.
Studies about nuclear condensation and fragmentation
Similar results were obtained from the nuclear condensation and fragmentation analysis. The significant nuclear fragmentation occurred 24 h after light exposure with compound 12 in HeLa cells (Fig. 6). In contrast, cells treated with compound 12 without illumination did not exhibit significant changes in nuclear fragmentation analyses (Fig. 6).
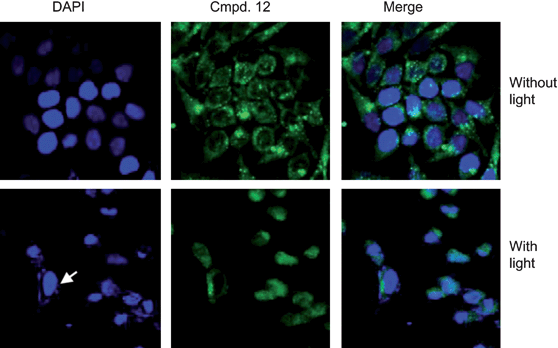 |
| Fig. 6 Compound 12 with PDT induces apoptotic cell death in HeLa cells. Nuclear condensation or fragmentation, one of typical apoptotic features, was assessed by nuclei staining with DAPI after cells were treated with or without 1 μM compound 12 with PDT. Images were visualized using a fluorescent microscope and captured with a CCD camera. Arrow indicates normal nuclei. | |
Studies on PARP cleavage
Based on our literature and research experience,21 next, we also checked poly (ADP-ribose) polymerase (PARP) cleavage after compound treatment. PARP is a DNA repair enzyme whose expression is triggered by DNA strand breaks, and one of caspase-3 targets. If cells undergo apoptosis, PARP with 113 kD peptide is cleaved into 24 and 89 kD polypeptides by active caspase-3. We found that the treatment with compound 12 resulted in a cleavage of 113 kD PARP to 85 kD in HeLa cells, which was most dramatic in cells at 24 h after treatment with PDT (Fig. 7). The results were consistent with the phototoxicity effect of compound 12. Taken together, our data suggest that apoptosis induced by the treatment of compound 12 was via caspase-dependent apoptotic pathway in cancer cells.
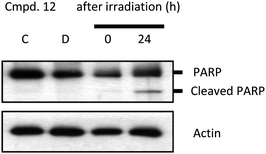 |
| Fig. 7 Compound 12 with PDT induces apoptotic cell death in HeLa cells. Cells were treated with or without 1 μM compound 12 for 24 h and illuminated, and 24 h after irradiation, cells were collected and lysed. The supernatant of the lysate was applied to immunoblotting to detect PARP cleavage. C, untreated cells; D, cells incubated with compound 12 without irradiation; 0 and 24, designate time points of cell harvest after irradiation (0.2 kJ cm−2). | |
Conclusions
A series of glycofused porphyrin derivatives with C2 and D2 symmetry, 3–12 have been designed and efficiently prepared. Their structures were fully confirmed by spectroscopic techniques, and their spectral properties were well characterized. The derivatives 5, 6, 11, and 12 showed significant cellular uptake and photocytotoxicity in HeLa cells and HCT116 cells, respectively at a concentration of 1 μM. In particular, the tetra-glycofused structure 12 exhibited the highest cellular uptake and photocytotoxicity. Unlike the reported sugar-porphyrin conjugates, which normally localize in mitochondria or endoplasmic reticulum, the unique glycofused porphyrins we designed in this study were dominantly localized in lysosomes. Sugar moieties in our molecules should take credit for the enhanced cellular uptake and also for the lysosome localization. The measurement of the dual flurorescence of annexin V-FITC/PI by flow cytometry revealed that the cell death was caused by apoptosis. Further PARP cleavage study suggested that apoptosis induced by the treatment of compound 12 was via caspase-dependent apoptotic pathway in cancer cells. The in vivoPDT efficacy of compound 12 is under investigation in our laboratory.
Acknowledgements
We thank Drs Malini Carolene Olivo and Patricia Thong for their kind advice for the experiments. Financial supports from Nanyang Technological University (RG50/08) and the Ministry of Health, Singapore (NMRC/H1N1R/001/2009 and NMRC/1177/2008) are gratefully acknowledged.
Notes and references
-
(a) J. F. Lovell, T. W. B. Liu, J. Chen and G. Zheng, Chem. Rev., 2010, 110, 2839 CrossRef CAS;
(b) J. P. Celli, B. Q. Spring, I. Rizvi, C. L. Evans, K. S. Samkoe, S. Verma, B. W. Pogue and T. Hasan, Chem. Rev., 2010, 110, 2795 CrossRef CAS;
(c) M. Ethirajan, Y. Chen, P. Joshi and R. K. Pandey, Chem. Soc. Rev., 2011, 40, 340, 10.1039/B915149B;
(d) A. P. Castano, P. Mroz and M. R. Hamblin, Nat. Rev. Cancer, 2006, 6, 535 CrossRef CAS;
(e) M. R. Detty, S. L. Gibson and S. J. Wagner, J. Med. Chem., 2004, 47, 3897 CrossRef CAS;
(f) W. M. Sharman, J. E. van Lier and C. M. Allen, Adv. Drug Delivery Rev., 2004, 56, 53 CrossRef CAS;
(g) D. E. J. G. J. Dolmans, D. Fukumura and R. K. Jain, Nat. Rev. Cancer, 2003, 3, 380 CrossRef CAS.
- P. Morlière, J.-C. Mazière, R. Santus, C. D. Smith, M. R. Prinsep, C. C. Stobbe, M. C. Fenning, J. L. Golberg and J. D. Chapman, Cancer Res., 1998, 58, 3571 CAS.
- L. V. Chekulayeva, V. A. Chekulayev and I. N. Shevchuk, J. Photochem. Photobiol., B, 2008, 93, 94 CrossRef CAS.
-
(a) S. Banfi, E. Caruso, L. Buccafurni, R. Murano, E. Monti, M. Gariboldi, E. Papa and P. Gramatica, J. Med. Chem., 2006, 49, 3293 CrossRef CAS;
(b) Y. Ferrand, L. Bourré, G. Simonneaux, S. Thibaut, F. Odobel, Y. Lajat and T. Patrice, Bioorg. Med. Chem. Lett., 2003, 13, 833–835 CrossRef CAS.
- J. E. van Lier, H. Tian, H. Ali, N. Cauchon and H. M. Hasséssian, J. Med. Chem., 2009, 52, 4107 CrossRef CAS.
- F. Yukruk, A. L. Dogan, H. Canpinar, D. Guc and E. U. Akkaya, Org. Lett., 2005, 7, 2885 CrossRef CAS.
- Y. Chen, W. R. Potter, J. R. Missert, J. Morgan and R. K. Pandey, Bioconjugate Chem., 2007, 18, 1460 CrossRef CAS.
-
(a) S. Hirohara, M. Obata, H. Alitomo, K. Sharyo, T. Ando, S. Yano and M. Tanihara, Bioconjugate Chem., 2009, 20, 944 CrossRef CAS;
(b) X. Zheng and R. K. Pandey, Anti-Cancer Agents Med. Chem., 2008, 8, 241 CrossRef CAS;
(c) M. Amessou, D. I. Carrez, D. Patin, M. Sarr, D. S. Grierson, A. Croisy, A. C. Tedesco, P. Maillard and L. Johannes, Bioconjugate Chem., 2008, 19, 532 CrossRef CAS;
(d) D. Samaroo, M. Vinodu, X. Chen and C. M. Drain, J. Comb. Chem., 2007, 9, 998 CrossRef CAS;
(e) J. P. C. Tomé, E. M. P. Silva, A. M. V. M. Pereira, C. M. A. Alonso, M. A. F. Faustino, M. G. P. M. S. Neves, A. C. Tomé, J. A. S. Cavaleiro, S. A. P. Tavares, R. R. Duarte, M. F. Caeiro and M. L. Valdeira, Bioorg. Med. Chem., 2007, 15, 4705 CrossRef CAS;
(f) I. Laville, S. Pigaglio, J.-C. Blais, F. Doz, B. Loock, P. Maillard, D. S. Grierson and J. Blais, J. Med. Chem., 2006, 49, 2558 CrossRef CAS;
(g) S. Hirohara, M. Obata, S.-I. Ogata, C. Ohtsuki, S. Higashida, S.-I. Ogura, I. Okura, M. Takenaka, H. Ono, Y. Sugai, Y. Mikata, M. Tanihara and S. Yano, J. Photochem. Photobiol., B, 2005, 78, 7 CrossRef CAS;
(h) X. Chen and C. M. Drain, Drug Des. Rev.–Online, 2004, 1, 215 Search PubMed;
(i) K. Fujimoto, T. Miyata and Y. Aoyama, J. Am. Chem. Soc., 2000, 122, 3558 CrossRef CAS;
(j) C. Schell and H. K. Hombrecher, Bioorg. Med. Chem., 1999, 7, 1857 CrossRef CAS;
(k) M. Momentreau, P. Maillard, M. A. De Bélinary, D. Carrez and A. Croisy, J. Biomed. Opt., 1999, 4, 298 CrossRef CAS.
- X. Chen, L. Hui, D. A. Foster and C. M. Drain, Biochemistry, 2004, 43, 10918 CrossRef CAS.
- E. D. Sternberg and D. Dolphin, Tetrahedron, 1998, 54, 4151 CrossRef CAS.
- J.-W. Hofman, F. van Zeeland, S. Turker, H. Talsma, S. A. G. Lambrechts, D. V. Sakharov, W. E. Hennink and C. F. van Nostrum, J. Med. Chem., 2007, 50, 1485 CrossRef CAS.
- S. P. Zamora-León, D. W. Golde, I. I. Concha, C. I. Rivas, F. Delgado-López, J. Baselga, F. Nualart and J. C. Vera, Proc. Natl. Acad. Sci. U. S. A., 1996, 93, 1847 CrossRef CAS.
-
(a) C.-F. Choi, J.-D. Huang, P.-C. Lo, W.-P. Fong and D. K. P. Ng, Org. Biomol. Chem., 2008, 6, 2173 RSC;
(b) P.-C. Lo, C. M. H. Chan, J.-Y. Liu, W.-P. Fong and D. K. P. Ng, J. Med. Chem., 2007, 50, 2100 CrossRef CAS;
(c) P. P. S. Lee, P.-C. Lo, E. Y. M. Chan, W.-P. Fong, W.-H. Ko and D. K. P. Ng, Tetrahedron Lett., 2005, 46, 1551 CrossRef CAS.
- M. R. Prinsep, G. M. L. Patterson, L. K. Larsen and C. D. Smith, Tetrahedron, 1995, 51, 10523 CrossRef CAS.
-
(a) R. Lorpitthaya, Z. H. Xie, K. B. Sophy, J. L. Kuo and X.-W. Liu, Chem.–Eur. J., 2010, 16, 588 CrossRef CAS;
(b) R. Y. Yang, K. K. Pasunooti, F. Li, X.-W. Liu and C.-F. Liu, J. Am. Chem. Soc., 2009, 131, 13592 CrossRef CAS;
(c) H. G. Sudibya, J. Ma, X. Dong, S. Ng, L.-J. Li, X.-W. Liu and P. Chen, Angew. Chem., Int. Ed., 2009, 48, 2723 CrossRef CAS;
(d) B. K. Gorityala, R. Lorpitthaya, Y. Bai and X.-W. Liu, Tetrahedron, 2009, 65, 5844 CrossRef CAS;
(e) R. Lorpitthaya, K. B. Sophy, J. L. Kuo and X.-W. Liu, Org. Biomol. Chem., 2009, 7, 1284 RSC;
(f) B. K. Gorityala, S. Cai, R. Lorpitthaya, J. Ma, K. K. Pasunooti and X.-W. Liu, Tetrahedron Lett., 2009, 50, 676 CrossRef CAS;
(g) X.-W. Liu, T. N. Le, Y. P. Lu, Y. J. Xiao, J. M. Ma and X. Li, Org. Biomol. Chem., 2008, 6, 3997 RSC;
(h) R. Lorpitthaya, Z.-Z. Xie, J.-L. Kuo and X.-W. Liu, Chem.–Eur. J., 2008, 14, 1561 CrossRef CAS;
(i) X.-M. Cheng and X.-W. Liu, J. Comb. Chem., 2007, 9, 906–908 CrossRef CAS.
- S. R. Griegel, T. Ciesiolka and H. J. Gabius, Anticancer Res., 1989, 9, 723 CAS.
-
(a) G. Casiraghi, M. Cornia, F. Zanardi, G. Rassu, E. Ragg and R. Bortolini, J. Org. Chem., 1994, 59, 1801 CrossRef CAS;
(b) M. Cornia, G. Casiraghi, S. Binacchi, F. Zanardi and G. Rassu, J. Org. Chem., 1994, 59, 1226 CrossRef CAS.
-
(a) Y. Urano, D. Asanuma, Y. Hama, Y. Koyama, T. Barrett, M. Kamiya, T. Nagano, T. Watanabe, A. Hasegawa, P. L. Choyke and H. Kobayashi, Nat. Med., 2008, 15, 104.
- T. Cirman, K. Orešić, G. D. Mazovec, V. Turk, J. C. Reed, R. M. Myers, G. S. Salvesen and B. Turk, J. Biol. Chem., 2003, 279, 3578 CrossRef.
-
(a) Klara Stefflova, Juan Chen, Diane Marotta, Hui Li and Gang Zheng, J. Med. Chem., 2006, 49, 3850 CrossRef CAS;
(b) M. Lam, N. L. Oleinick and A.-L. Nieminen, J. Biol. Chem., 2001, 276, 47379 CrossRef CAS.
-
(a) S. M. Chiu, L. Y. Xue, K. Azizuddin and N. Oleinick, Apoptosis, 2005, 10, 1357–1368 Search PubMed;
(b) J. He, C. M. Whitacre, L.-Y. Xue, N. A. Berger and N. L. Oleinick, Cancer Research, 1998, 58, 940–946 CAS.
Footnote |
† Electronic supplementary information (ESI) available: Experimental procedures and compound characterization data; experimental procedures, materials and methods for bioassay. See DOI: 10.1039/c0md00175a |
|
This journal is © The Royal Society of Chemistry 2011 |
Click here to see how this site uses Cookies. View our privacy policy here.