DOI:
10.1039/C1FO10042D
(Paper)
Food Funct., 2011,
2, 438-444
Effect of preparation method on the glycaemic index of novel potato clones†‡§
Received
16th March 2011
, Accepted 23rd June 2011
First published on 15th July 2011
Abstract
The purpose of this study was to investigate whether the effects of cooling and reheating on the glycaemic index (GI) of novel potato clones (selections) differed depending on selection and whether cooling altered starch absorption in vivo. We conducted 3 experiments using 4 novel potato clones in healthy subjects. Experiment 1: the GI of 4 selections each prepared in 3 ways (freshly boiled, cooled, or cooled and reheated) was measured in 2 groups of 10 subjects (each group tested 2 selections). Experiment 2 (n = 10): two selections from Experiment 1 were re-tested one year later, by a different subject group. Experiment 3 (n = 10): two selections from Experiment 1 were tested by subjects from Experiment 2 to assess the rate and extent of starch absorption using the second-meal effect and the breath hydrogen method, respectively. Experiment 1 demonstrated a selection×treatment interaction for GI (p = 0.024); cooling reduced the GI of two selections by 40–50% (p < 0.05) but reduced GI of the other 2 by only 8–10% (ns). Experiment 2 confirmed the selection×treatment interaction (p = 0.018) seen in Experiment 1. Experiment 3: cooling reduced the GI by an average of 37% (p < 0.05) but only increased starch malabsorption in vivo from 3% to 5% (p = 0.021); there was no significant second-meal effect. It is concluded that the effect of cooling on the GI of potatoes may vary from 0–50% depending on selection. However, the mechanism for the effect is not clear: the 2% increase in starch malabsorption seen upon cooling potatoes was not nearly enough to account for the 37% reduction in GI.
Introduction
When prepared and consumed with a low amount of fat, potatoes are considered a good food choice because they are low in energy, are good sources of the short-fall nutrients vitamin C, potassium and dietary fibre, and contain very little sodium and fat.1,2 Potatoes contain approximately 80% water and 20% dry-matter, with 60–80% of dry-matter as carbohydrate, predominantly starch. Raw potato starch is resistant to digestive enzyme activity3 but when cooked it gelatinizes and becomes easily digestible. Foods high in rapidly digested starch have a high glycaemic index (GI) and elicit high insulin demand.4 Since high GI diets have been associated with increased risk of diabetes and cardiovascular disease, potatoes have been perceived as bad for health due to their purported high GI.5–8 However, reported GI values for 39 types of baked- or boiled-potatoes vary from 23 to 118, and although the majority of potatoes are high-GI (≥70), 36% are low- or intermediate-GI.9 This variation is too large to be explained by random error10 and, thus, is likely due to methodological errors or to variations in starch structure among potatoes related to differences in variety, processing methods and growing time.3,11–16
This study was part of a larger project whose overall aim was to develop new varieties of potato with a low-GI. We selected 4 novel potato clones for initial testing based on differences in their resistant starch and fibre contents, factors believed to be determinants of GI.17,18 Since cooling reduced the GI of red potatoes, and the effect of reheating tended to differ for russet and white potatoes,19 we hypothesized that the effect of cooling and reheating on the GI of potatoes would vary in different clones. This paper reports the results of 3 experiments performed to explore this hypothesis.
Materials, subjects and methods
All experiments were performed by healthy volunteers after 10–12 h overnight fasts using protocols approved by the Research Ethics Board at the University of Toronto. All subjects gave informed consent to participate. The potatoes tested were advanced clones from the Agriculture and Agri-Food Canada (AAFC) potato breeding program not available commercially during the study and grown in New Brunswick (AAFC Benton Ridge Potato Breeding Sub-station, 46°N), Alberta (AAFC Vauxhall Sub-station, 50°N), or Ontario (University of Guelph, 43°N). They are identified here as selections 1 (S-1, clone CV92056-4), 2 (S-2, clone CV1102-1), 3 (S-3, clone F03031) and 4 (S-4, clone F03028).
Experiment 1
Two groups of subjects (Group A: 5 female, 5 male; age, 20.1 ± 0.5 year; BMI, 24.6 ± 1.1kg m−2; Group B: 6 female, 4 male; age, 20.5 ± 0.5 year; BMI, 23.7 ± 0.7kg m−2) were studied on 10 mornings from 2 February to 16 April, 2009. S-1 and S-2, tested by Group A, were grown in Alberta and S-3 and S-4, tested by Group B, were grown in New Brunswick. Potatoes were grown to maturity, harvested in October 2008, treated with chlorpropham to inhibit sprouting and stored in a darkened growth chamber at 10–12 °C until mid-January 2009 at the University of Guelph prior to GI testing in Toronto. Each selection was prepared in 3 different ways: fresh-boiled (FB, 2.5–3 cm cubes boiled in salted water with skin intact for 15 min and served immediately); cold (C, boiled potatoes kept refrigerated at 4 °C for 24–28 h and served cold); and reheated (R, cold potatoes heated in a microwave oven for 2–3 min then served). All subjects tested baked-potato-chips (Baked Lays® Original Potato Crisps) as a positive control and also white-bread, baked as previously described,20 3 times as the reference food. All test-meals contained 50 g available carbohydrate (defined as total carbohydrate minus dietary fibre) based on proximate analysis of bread and potatoes (Agri-Food Laboratories, Guelph, Ontario and Maxxam Analytics Mississauga, Ontario) and the nutrition label for baked-potato-chips (Table 1).
Test-meals |
Weight (g) |
Energy (kcal) |
Fat (g) |
Protein (g) |
Total CHO (g) |
Fibre (g) |
Available CHO (g) |
Abbreviation: CHO, carbohydrate.
Potato serving size roughly equivalent to 2 medium potatoes.
|
Experiment 1
|
Selection 1 |
345b |
252 |
0 |
7.2 |
55.8 |
5.8 |
50.0 |
Selection 2 |
360 |
256 |
0 |
6.5 |
57.2 |
7.2 |
50.0 |
Selection 3 |
321 |
244 |
0 |
6.1 |
55.6 |
5.4 |
50.0 |
Selection 4 |
394 |
256 |
0 |
7.1 |
56.3 |
6.3 |
50.0 |
Baked Potato Chips |
66 |
264 |
3.3 |
5.3 |
54.1 |
4.1 |
50.0 |
White Bread |
104 |
245 |
1.7 |
7.5 |
52.8 |
2.8 |
50.0 |
|
Experiment 2
|
Selection 2 |
291 |
242 |
0 |
5.5 |
54.9 |
4.9 |
50.0 |
Selection 3 |
250 |
235 |
0 |
4.8 |
54.0 |
4.0 |
50.0 |
|
Experiment 3
|
Selection 1 |
235 |
237 |
0.24 |
5.4 |
53.5 |
3.5 |
50.0 |
Selection 3 |
301 |
250 |
0.30 |
6.3 |
55.4 |
5.4 |
50.0 |
Test-meals were served in randomized order and consumed within 15 min. After 2 fasting finger-prick blood samples at 5 min intervals, subjects consumed a test-meal plus 250ml of water, tea, or coffee with 30 ml 2% milk if desired (drink chosen was consistent for all tests) and further blood samples were taken at 15, 30, 45, 60, 90 and 120 min after starting to eat.
Experiment 2
The results of Experiment 1 suggested that cooling reduced GI in S-3 and S-4, but not S-1 and S-2. The purpose of experiment 2 was to confirm these effects in S-2 and S-3 harvested in October, 2009. Healthy subjects (5 female, 5 male; age, 42.3 ± 3.3 year; BMI, 26.9 ± 0.4 kg m−2), none of whom participated in Experiment 1, attended on 6 mornings from 25 November, 2009 to 22 January, 2010. S2, grown in Alberta and harvested prior to maturity, and S3, grown to maturity in Ontario, were stored in Guelph prior to GI testing. The procedures were the same as those for Experiment 1 except that each potato selection was served in only 2 ways (FB and C) and subjects tested white-bread 2 times.
Experiment 3
The purpose of Experiment 3 was to determine if the effects of cooling on the GI of S-1 and S-3 were associated with differences in the rate or extent of starch absorption in vivo. Starch malabsorption was estimated using the breath hydrogen (H2) method.21 The rate of starch absorption was assessed using the second-meal effect,22 whereby a reduction in glycaemic response after lunch suggests that the carbohydrate consumed at breakfast was slowly absorbed.23 Ten subjects (4 female, 6 male; age, 43.8 ± 2.7 year; BMI, 26.8 ± 0.4 kg m−2), 9 of whom participated in Experiment 2, attended on 6 mornings between 26 February and 8 April, 2010. After fasting blood samples they ate one of 6 test-meals containing 50g available-carbohydrate in randomized order as in Experiment 1. However, after the 120 min blood sample, subjects remained seated quietly until 240 min, when they took another finger-prick blood sample and consumed, within 15 min, a lunch consisting of a turkey, cheese, tomato and lettuce sandwich with juice and 2 cookies (2530 kJ (606 kcal), 25.4 g protein, 19.5 g fat, 82.5 g carbohydrate and 2.1 g dietary fibre). Additional blood samples were taken at 255, 270, 285, 300, 330 and 360 min. Samples of expired air were collected fasting and hourly for 8 h into 10 ml evacuated glass tubes using an EasySampler device (Quintron Instruments, Milwaukee, WI).
The potatoes in Experiment 3 were grown in Ontario (S-1) or New Brunswick (S-3), harvested in October 2009, treated with sprout suppressant and stored in Guelph prior to proximate analysis (Table 1) and GI testing. Both potato selections were served freshly-boiled and cold (same as Experiment 2). All subjects consumed white-bread on two separate occasions to one of which was added 10 g lactulose (Ratio-Lactulose 667 mg ml−1) in 100 ml water.
Analytical methods
Finger-prick blood (2–3 drops) was collected into fluoro-oxalate tubes and stored at −20 °C for <48 h prior to analysis of whole-blood glucose (Model 2300 STAT analyzer, Yellow Springs Instruments, Yellow Springs, OH). Fresh-boiled (but not cold) potatoes from Experiment 1 (but not Experiments 2 and 3) were analysed for amylose using a colourimetric method24 and for rapidly digested-, slowly digested-, resistant- and total-starch (RDS, SDS, RS and TS, respectively; Table 2) in cooked potato dry-matter using an in vitro method.25 Breath carbon dioxide, H2 and methane were measured by gas chromatography (Microlyzer Gas Analyzer, model SC; Quintron Insturments).
Table 2
Amylose, RDS, SDS, RS, TS content of potato dry matter of fresh-boiled potatoes used in Experiment 1a,b
Potato
|
Amylose (%) |
RDS (%)c |
SDS (%)c |
RS (%)d |
TS% (w/w) |
Abbreviations: RDS, rapidly digestible starch, SDS, slowly digestible starch, RS, resistant starch, TS, total starch.
Values are means ±SEM. For amylose and TS values were obtained from the mean of two raw dry matter samples and RDS and SDS were obtained from the mean of two cooked dry matter samples from potatoes harvested in 2008.
RDS and SDS is based on rate of starch hydrolysis, RDS classified as starch digested within 20 min, SDS classified as starch digested between 20–120 min.
RS determined by; (%TS-(%RDS + % SDS)) and is classified as undigested starch after 120 min.
|
Selection 1 |
27.2 ± 0.2 |
55.4 ± 1.5 |
4.4 ± 1.0 |
9.8 |
69.6 ± 3.3 |
Selection 2 |
24.3 ± 0.2 |
52.0 ± 1.2 |
5.1 ± 0.5 |
12.6 |
69.7 ± 3.6 |
Selection 3 |
22.7 ± 0.2 |
41.8 ± 0.3 |
5.2 ± 0.6 |
16.0 |
62.9 ± 2.4 |
Selection 4 |
22.8 ± 0.1 |
40.4 ± 0.5 |
4.6 ± 1.1 |
19.5 |
64.6 ± 0.9 |
Calculations and statistical analysis
Incremental areas under the glucose curve (AUC), excluding area below fasting, were calculated using the trapezoid rule.26 To calculate GI, the AUC for each potato test meal was expressed as a percentage of the mean AUC for white-bread taken by the same subject and multiplied by 0.71 to convert to the glucose standard (i.e. the GI of glucose = 100).26
In Experiment 3, glucose AUCs were calculated separately for 0–120 min (breakfast) and 240–360 min (lunch) periods. Total H2 was the sum of H2 concentrations from the lowest value within the first 3 h to 8 h.21 Total H2/g lactulose, L = (BL − B)/10g where BL and B are total H2 after white-bread-plus-lactulose and white-bread alone, respectively. The amount of starch malabsorbed was calculated as P/L, where P is total H2 after the potato test meal.
Results are expressed as means ± SEM. Data from each experiment were subjected to repeated measures ANOVA examining for the effects of potato selection and treatment (preparation method) and the selection × treatment interaction; year-of-harvest was brought into the model for Experiments 2 and 3. GI values >2SD from the mean were excluded as outliers.26 Values for breath H2 and carbohydrate malabsorbed were normalized by taking the logarithm prior to statistical anlaysis. Glucose and H2 profiles were subjected to ANOVA to investigate time × selection × treatment interactions. The significance of differences among means was assessed using Tukey's test to adjust for multiple comparisons with two-tailed p ≤ 0.05. Analysis was done using SPSS Statistics version 17.0.
Results
Experiment 1
S-2 spoiled during the study and 5 tests involving it were not done (2 fresh-boiled, 1 cold, 2 reheated). Missing data were interpolated.27 One outlying GI value (S-1-FB) was excluded. Glycemic responses are shown in the ESI (ESI Fig. 1).† The overall AUC after cold potatoes (123 ± 22 mmol min L−1) was significantly less than fresh-boiled (183 ± 28 mmol min L−1) and reheated potatoes (182 ± 27 mmol min L−1) (p < 0.001, ESI Table 1†).
The GI of baked-potato-chips were similar in Groups A and B, 72.3 ± 6.1 and 71.2 ± 8.2 respectively. Cooling significantly reduced GI in S-3 and S-4 by 49% and 38% respectively, but in S-1 and S-2 by only 8% and 10% (ns; selection × treatment interaction p = 0.024; Table 3, ESI Fig. 2). Compared to fresh-boiling, reheating tended to increase the GI of S-1, reduce the GI of S-4, and have no effect in S-2 and S-3 (ns).
Table 3 Glycaemic index values of potato selections treated in different ways prior to conusmptiona,b
|
Treatment |
Mean for Selection
|
Fresh boiled |
Reheated |
Cold |
Values are means ± SEM for n = 10 healthy subjects.
Experiment 1: potatoes harvested in 2008; Experiments 2 and 3: potatoes harvested in 2009.
Values within rows not sharing the same letter superscript differ significantly (P < 0.05).
Values within columns not sharing the same letter superscript differ significantly (P < 0.05).
Significance of selection × treatment interaction.
|
Experiment 1
|
Selection 1 |
59.8 ± 5.2 |
75.3 ± 5.3 |
55.3 ± 6.9 |
63.5 ± 5.8
|
Selection 2 |
61.2 ± 3.9 |
69.1 ± 5.3 |
55.0 ± 6.5 |
61.8 ± 5.2
|
Selection 3 |
79.1 ± 8.8c |
78.5 ± 4.3c |
40.3 ± 5.3d |
66.0 ± 6.1
|
Selection 4 |
71.6 ± 7.8c |
57.8 ± 5.3cd |
44.1 ± 6.7d |
57.8 ± 6.6
|
Mean for Treatment
|
67.9 ± 6.4
c
|
70.2 ± 5.1
c
|
48.7 ± 6.4
d
|
P = 0.024g |
|
Experiment 2
|
Selection 2 |
37.8 ± 5.5 |
|
40.6 ± 5.0 |
39.2 ± 5.3
e
|
Selection 3 |
72.5 ± 6.1c |
|
48.6 ± 6.9d |
60.5 ± 6.5
f
|
Mean for Treatment
|
55.2 ± 5.8
|
|
44.6 + 6.0
|
P = 0.018g |
|
Experiment 3
|
Selection 1 |
82.2 ± 6.8c |
|
48.3 ± 5.5d |
65.3 ± 6.2
|
Selection 3 |
74.2 ± 9.0c |
|
50.3 ± 5.9d |
62.3 ± 7.5
|
Mean for Treatment
|
78.2 ± 7.9c |
|
49.3 ± 5.7d |
nsg |
Fresh-boiled potato GIs were inversely related to amylose (p = 0.031) and RDS (p = 0.028), cold potato GIs were positively related to amylose (p = 0.062) and RDS (p = 0.045) and reheated potato GIs were inversely related to RS (p = 0.048) (SI Figure 3). The regression slopes for fresh-boiled differed from cold potatoes for amylose (p = 0.004), RDS (p = 0.003) and RS (p = 0.026) and from reheated potatoes for RDS (p = 0.028) and RS (p = 0.013) (ESI Fig. 3†).
Experiment 2
Glycaemic responses are shown in ESI Fig. 4.† Cooling significantly reduced AUC and GI in S-3 but tended to increase AUC and GI in S-2 (selection × treatment interaction p = 0.008 for AUC and p = 0.018 for GI; Table 3). The GI of S-2 was lower in 2009 than 2008, while S-3 had similar GIs in both years (year-of-harvest × selection interaction, p = 0.027; ESI Fig. 5A†). Combining data from 2008 and 2009 showed that cooling reduced the GI of S-3, but not S-2 (selection × treatment interaction, p = 0.001; ESI Fig. 5B†).
Experiment 3
Glycaemic responses and second-meal effect.
Blood glucose was significantly higher after fresh-boiled than cold potatoes for S-3 at 15 min and for both S-1 and S-3 at 30, 45 and 60 min (Fig. 1). Blood glucose after S-3-FB was less than S-3-C at 240 min S-1-FB was less than S-2-C at 255 min (p < 0.05; Fig. 1). Cold potatoes elicited a 40% lower 0–120 min AUC than fresh-boiled potato (main effect of treatment, p < 0.001; selection × treatment interaction, p = 0.168; ESI Table 1†). After lunch there was no difference in AUC among treatments or selections and no selection × treatment interaction (p = 0.970).
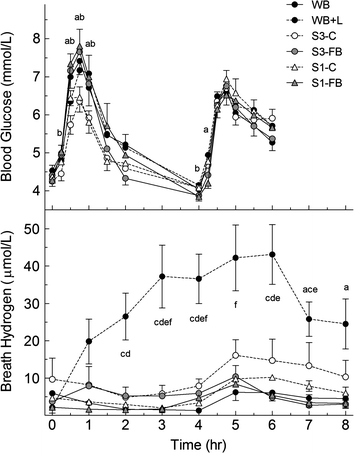 |
| Fig. 1 Results for Experiment 3: blood glucose and breath H2 responses elicited by 2 selections of potatoes (Selection 1 = S1 and Selection 3 = S3) served either fresh boiled (FB) or cold (C) taken at breakfast (0 h) followed by a standard lunch taken at 4 h. Values are means ± SEM (n = 10). Significant differences (p < 0.05): a, S1-FBvs.S1-C; b, S3-FBvs.S3-C; c, S1-FBvs. WB + L; d, S1-Cvs. WB + L; e, S3-FBvs. WB + L; f, S3-Cvs. WB + L. | |
Glycaemic index.
One outlying GI value (S-1-FB) was excluded. GI was higher for fresh-boiled than cold potatoes (p < 0.001) regardless of selection (Table 3). The GI of S-3-C was significantly less than S-3-FB in both Experiments 1 and 3, but S-1-C was less than S-1-FB only in Experiment 3 (year-of-harvest × selection × treatment interaction, p = 0.025; Table 3).
Breath H2 and malabsorbed carbohydrate.
Mean breath H2 was higher after lactulose than the other meals at 6 of the 9 time points (P < 0.05) and higher after S-1-C than S-1-FB at 420 and 480 min (p < 0.05) (Fig. 1). Total H2 after white-bread-plus-lactulose, 258 ± 40 μmol L−1, was greater than bread alone, 24 ± 7 μmol L−1, and all the potato test meals (p < 0.05). Cold potatoes produced significantly more total H2 (61 ± 19 vs. 34 ± 7 μmol L−1) (P = 0.021) than fresh-boiled potatoes, regardless of selection. More carbohydrate was malabsorbed after cold than fresh-boiled potatoes, 2.5 ± 0.4gvs. 1.6 ± 0.3g (p = 0.021; no selection × treatment interaction, p = 0.982, ESI Fig. 6†).
Discussion
The results supported the hypothesis that the effects of cooling on the GI of potatoes differs amongst different selections, however the glycaemic effects we observed could not be explained by the intakes of amylose, RDS, SDS or RS measured in vitro, nor by a reduced rate or extent of starch absorption assessed in vivo.
In Experiment 1 we used 2 rather than 1 subject group to try to complete the study before the potatoes began to spoil. Potential disadvantages of this were that, without repeated measures across all treatments, statistical power might be reduced; also, differences in glycaemic response between subject groups might bias the results. However, expressing results as GI reduces between-subject variation,28,29 as shown here by the similar GI for baked-potato-chips in the 2 groups in the face of a 20% higher AUC after WB in Group B than Group A.
The GI values obtained for S-3 were similar in Experiments 1, 2 and 3, respectively for both fresh-boiled (79, 73 and 74) and cooled (40, 49 and 50). The slightly different GI values in Experiments 2 and 3 than Experiment 1 might reflect differences in starch structure due to diverse growing conditions,11 but the differences in GI are within experimental error. However, the GI for S-2 in 2009, 39, was significantly less than in 2008, 62 (Table 3). We believe this is because the potatoes were harvested before maturity in 2009 but at maturity in 2008. New (immature) potatoes contain more amylose and resistant starch than mature potatoes12 and elicit lower glycaemic responses.13 It is of interest that, compared to fresh-boiled, cooling had no effect on the GI in S-2 regardless of whether they were mature (2008) or immature (2009).
We tested S-1 and S-3 in Experiment 3 to see if measures of starch digestibility in vivo differed in a potato in which, based on the results of Experiment 1, cooling reduced GI (S-3) compared to a potato in which it did not (S-1). However, in Experiment 3, the GI of both potatoes was reduced by cooling. The GI of S-1-C was similar in Experiments 1 and 3, but S-1-FB had a significantly lower GI in Experiment 1 than 3, 60 vs. 82. Although it has been argued that the GI method is imprecise,30,31 inconsistent32 and unreliable,33 we believe these opinions to be mistaken.26,34 The precision and reliability of the GI method is supported in the present study by the similar GI values for S-3 in all 3 experiments and the similar values for baked-potato-chips in 2 different subject groups. Thus, while experimental error cannot be ruled out, it seems more likely that the difference in GI of S-1-FB between Experiments 1 and 3 is due to differences in starch structure related to variation in growing conditions.11
The breath H2 method is used to estimate the amount of starch malabsorbed in vivo35 because of its simplicity and low cost. The results may be imprecise or inaccurate because of high day-to-day variation within- and between-subjects,36 differences in the stoichiometry of H2 production from the fermentation of different carbohydrates, or other confounding variables.37,38 However, we believe the method to be accurate for starchy foods because, in our hands, the amounts of carbohydrate malabsorbed after white-bread, whole-wheat-bread and lentils estimated using the breath H2 method with lactulose as the reference carbohydrate agreed closely with those obtained by direct measurement in subjects with an ileostomy.21 The mean values for carbohydrate malabsorption estimated here for cooked and cooled potatoes, 3% and 5%, respectively, are similar to the amounts of starch and free sugars recovered from subjects with an ileostomy, 2% and 9%.39 If the results are accurate, the amount of resistant starch created upon cooling the potatoes in Experiment 3 does not explain the 37% reduction in GI (78 vs. 49; Table 3). Because of the non-linear dose-response curve for glucose AUC on carbohydrate intake,40 a 48% reduction in carbohydrate absorption (50 to 26g) would be required to reduce AUC by 37%.
The second-meal phenomenon refers to how the administration of oral or intravenous carbohydrate modifies the utilization of subsequent closely spaced carbohydrate loads.41 Reducing available carbohydrate intake at breakfast increases the glycaemic response after lunch,22,42,43 while slowing the rate of carbohydrate consumption44 or consuming low GI foods at breakfast reduces the glycaemic response after lunch.22,43,45,46 Resistant starch intake at breakfast does not affect the lunch response.23 In Experiment 3 there was no significant second-meal effect and, therefore, no direct evidence that cooling potatoes reduced the rate of carbohydrate absorption. Nevertheless, the blood glucose concentration 4h after S-3-C was significantly higher than S-3-FB suggests some degree of delayed absorption which was not large enough to elicit a second-meal effect.
There were several significant relationships between GI and starch characteristics in Experiment 1. The negative correlation between amylose and GI of fresh-boiled potatoes was expected because high-amylose starches are digested more slowly and elicit lower glycemic responses than those low in amylose.47,48 However, the positive relationship between amylose and GI of cold potatoes is inconsistent with the finding that amylose retrogrades more readily than amylopectin.49 The negative relation between GI of fresh-boiled potatoes and RDS was also unexpected. RDS is the major source of rapidly available glucose (RAG) in potatoes, and the RAG content of foods is directly related to GI and insulinaemic index (II).17,18,50 However, the reported correlations between RAG and GI50 and II18 are not close enough (r = 0.45 to 0.50) to reliably distinguish GI or II for foods varying in RAG by only 10 g, approximately the range of RDS intakes in this study; 27 to 37 g (ESI Fig. 3†). A weakness of the present study is that RDS, SDS and RS were not measured in cooled potatoes.
In addition, other characteristics of potato starch which may affect its glycemic impact were not assessed. For example the degree of gelatinization of starch in foods has generally been found to correlate positively with starch digestibility in vitro51–53 although the correlation between degree of starch gelatinization and GI is less consistent.51–53 A potentially important parameter which may influence starch gelatinization54 is the phosphate content of potatoes which is known to vary by variety11 and maturity.12 It is assumed that the GI of potatoes is reduced upon cooling due to starch retrogradation, but since we measured neither gelatinization nor retrogradation it is unknown if differences in these parameters in the different potato clones account for the different effects of cooling on GI. Therefore, future research on the glycemic impact of potatoes should include a wider variety of measures of starch physicochemical properties than was assessed in this study.
We conclude that our results demonstrate that the effect of cooling on the GI of potatoes may vary from 0–50% depending on selection. However, the mechanism for the effect is not clear: differences in GI were not explained by the amylose, RDS, SDS or RS content of the potatoes, and the 2% increase in starch malabsorption in vivo seen upon cooling potatoes was not nearly enough to account for the 37% reduction in GI.
Acknowledgements
Supported by the Agricultural Bioproducts Innovation Program of Agriculture and Agri-Food Canada.
References
-
Dietary Guidelines Advisory Committee ( 2011), Report of the Dietary Guidelines Advisory Committee on the Dietary Guidelines for Americans, 2010, http://www.cnpp.usda.gov/Publications/DietaryGuidelines/2010/DGAC/Report/2010DGACReport-camera-ready-Jan11-11.pdf, accessed 2 March, 2011 Search PubMed.
-
Health Canada ( 2009), Nutrient value of some common foods, http://www.hc-sc.gc.ca/fn-an/nutrition/fiche-nutri-data/nutrient_value-valeurs_nutritives-eng.php, accessed: July 12, 2010 Search PubMed.
- M. Camire, S. Kubow and D. J. Donnelly, Potatoes and human health, Crit. Rev. Food Sci. Nutr., 2009, 49, 823–840 CrossRef CAS.
- L. S. Augustin, S. Franceschi, D. J. A. Jenkins, C. W. Kendall and C. La Vecchia, Glycemic index in chronic disease: a review, Eur. J. Clin. Nutr., 2002, 56, 1049–1171 CrossRef CAS.
- W. C. Willet, J. Manson and S. Liu, Glycemic index, glycemic load and risk of type 2 diabetes, Am. J. Clin. Nutr., 2002, 76, S274–S280.
- F. Golden, And now the bad news: Potatoes, Time Magazine, 21 January, 2002, 55–56 Search PubMed.
- S. Liu and W. C. Willett, Dietary glycemic load and atherothrombotic risk, Curr. Atheroscler. Rep., 2002, 4, 454–461 Search PubMed.
- M. B. Schultz, T. T. Fung, J. Manson, W. C. Willett and F. B. Hu, Dietary patterns and changes in body weight in women, Obesity, 2006, 14, 1444–1453 CrossRef.
- F. S. Atkinson, K. Foster-Powell and J. C. Brand-Miller, International tables of glycemic index and glycemic load values, Diabetes Care, 2008, 31, 2281–2283 CrossRef.
-
T. M. S. Wolever, The Glycaemic Index: A Physiological Classification of Dietary Carbohydrate, CABI Publishing, Wallingford, UK, 2006, pp. 120–123 Search PubMed.
- Q. Liu, R. Tarn, D. Lynch and N. M. Skjodt, Physicochemical properties of dry matter and starch from potatoes grown in Canada, Food Chem., 2007, 105, 897–907 CrossRef CAS.
- Q. Liu, E. Weber, V. Currie and R. Yada, Physicochemical properties of starches during potato growth, Carbohydr. Polym., 2003, 51, 213–221 CrossRef CAS.
- N. L. Soh and J. C. Brand-Miller, The glycemic index of potatoes: The effect of selection, cooking method and maturity, Eur. J. Clin. Nutr., 1999, 53, 249–254 CrossRef CAS.
- G. Zhang and B. R. Hamaker, Slowly digestible starch: Concept, mechanism, and proposed extended glycemic index, Crit. Rev. Food Sci. Nutr., 2009, 49, 852–867 CrossRef CAS.
- C. J. K. Henry, H. J. Lightowler, C. M. Strik and M. Storey, Glycaemic index values for commercially available potatoes in Great Britain, Br. J. Nutr., 2007, 94, 917–921.
- M. Leeman, E. Östman and I. Björck, Glycaemic and satiating properties of potato products, Eur. J. Clin. Nutr., 2007, 62, 87–95.
- K. N. Englyst, H. N. Englyst, G. J. Hudson, T. J. Cole and J. H. Cummings, Rapidly available glucose in foods: An in vitro measurement that reflects the glycemic response, Am. J. Clin. Nutr., 1999, 69, 448–454 CAS.
- K. N. Englyst, S. Vinoy, H. N. Englyst and V. Lang, Glycaemic index of cereal products explained by their content of rapidly and slowly available glucose, Br. J. Nutr., 2007, 89, 329–339.
- G. Fernandes, A. Velangi and T. M. S. Wolever, Glycemic index of potatoes commonly consumed in North America, J. Am. Diet. Assoc., 2005, 105, 557–562 CrossRef.
- T. M. S. Wolever and C. Bolognesi, Source and amount of carbohydrate affect postprandial glucose and insulin in normal subjects, J. Nutr., 1996, 126, 2798–2806 CAS.
- T. M. S. Wolever, Z. Cohen, L. U. Thompson, M. J. Thorne, M. J. A. Jenkins, E. J. Prokipchuk and D. J. A. Jenkins, Ileal loss of available carbohydrate in man: Comparison of a breath hydrogen method with direct measurement using a human ileostomy model, Am. J. Gastroenterol., 1986, 81, 115–122 CAS.
- D. J. A. Jenkins, T. M. S. Wolever, R. H. Taylor, C. Griffiths, K. Krzeminska, J. A. Lawrie, C. M. Bennett, D. V. Goff, D. L. Sarson and S. R. Bloom, Slow release carbohydrate improves second meal tolerance, Am. J. Clin. Nutr., 1982, 35, 1339–1346 CAS.
- H. G. M. Liljeberg, A. K. E. Åkerberg and I. M. E. Björck, Effect of the glycemic index and content of indigestible carbohydrates of cereal-based breakfast meals on glucose tolerance at lunch in healthy subjects, Am. J. Clin. Nutr., 1999, 69, 647–655 CAS.
- P. C. Williams, F. D. Kuzina and I. Hlynka, A rapid colorimetric procedure for estimating the amylose conent of starches and flours, Cereal Chem., 1970, 47, 411–420 CAS.
- H. N. Englyst, S. M. Kingman and J. H. Cummings, Classification and measurement of nutritionally important starch fractions, Eur. J. Clin. Nutr., 1992, 46(suppl.2), S33–S50.
- T. M. S. Wolever, J. C. Brand-Miller, J. Abernethy, A. Astrup, F. Atkinson, M. Axelsen, I. Björck, F. Brighenti, R. Brown, A. Brynes, M. C. Casiraghi, M. Cazaubiel, L. Dahlqvist, E. Delport, G. S. Denyer, D. Erba, G. Frost, Y. Granfeldt, S. Hampton, V. A. Hart, K. A. Hätönen, C. J. Henry, S. Hertzler, S. Hull, J. Jerling, K. L. Johnston, H. Lightowler, N. Mann, L. Morgan, L. N. Panlasigui, C. Pelkman, T. Perry, A. F. H. Pfeiffer, M. Pieters, D. D. Ramdath, R. T. Ramsingh, S. D. Robert, C. Robinson, E. Sarkkinen, F. Scazzina, D. C. D. Sison, B. Sloth, J. Staniforth, N. Tapola, L. M. Valsta, I. Verkooijen, M. O. Weickert, A. R. Weseler, P. Wilkie and J. Zhang, Measuring the glycemic index of foods: interlaboratory study, Am. J. Clin. Nutr., 2008, 87(suppl), S247–S57.
-
G. W. Snedecor and W. G. Cochran, Statistical Methods, Seventh Edition, Iowa State University Press, Ames, IA, 1980 Search PubMed.
- T. M. S. Wolever, Glycemic index vs. glycemic response: non-synonymous terms, Diabetes Care, 1992, 15, 1436–1437 CAS.
- T. M. S. Wolever, A. L. Jenkins, V. Vuksan and J. Campbell, The glycaemic index values of foods containing fructose are affected by metabolic differences between subjects, Eur. J. Clin. Nutr., 2009, 63, 1106–1114 CrossRef CAS.
- F. X. Pi-Sunyer, Glycemic index and disease, Am. J. Clin. Nutr., 2002, 76, 290S–298S CAS.
- J. W. DeVries, The glycemic index: the analytical perspective, Cereal Foods World, 2007, 52(2), 45–49 CAS.
- S. Vega-López, L. M. Ausman, J. L. Griffith and A. H. Lichtenstein, Interindividual variability and intra-individual reproducibility of glycemic index values for commercial white bread, Diabetes Care, 2007, 30, 1412–1417 CrossRef.
- S. M. Williams, B. J. Venn, T. Perry, R. Brown, A. Wallace, J. I. Mann and T. J. Green, Another approach to estimating the reliability of glycaemic index, Br. J. Nutr., 2008, 100, 364–372 CAS.
- T. M. S. Wolever, Another approach to estimating the reliability of the glycaemic index: a different interpretation, Br. J. Nutr., 2010, 103, 1695–1696 CrossRef CAS.
- A. Strocchi and M. D. Levitt, Measurement of starch absorption in humans, Can. J. Physiol. Pharmacol., 1991, 69, 108–110 CrossRef CAS.
- B. Braden, Methods and functions: Breath tests, Best Pract. Res. Clin. Gastroenterol., 2009, 23, 337–352 Search PubMed.
- G. Livesey, I. T. Johnson, J. M. Gee, T. Smith, W. E. Lee, K. A. Hillan, J. Meyer and S. C. Turner, ‘Determination’ of sugar alcohol and polydextrose® absorption in humans by the breath hydrogen (H2) technique: the stoichiometry of hydrogen production and the interaction between carbohydrates assessed in vivo and in vitro, Eur. J. Clin. Nutr., 1993, 47, 419–430 CAS.
- P. M. Owira, G. I. Young and T. A. Winter, Comparison of inulin and lactulose as reference standards in the breath hydrogen test assessment of carbohydrate malabsorption in patients with chronic pancreatic exocrine insufficiency, Dig. Dis. Sci., 2005, 50, 1058–1063 CrossRef CAS.
- H. N. Englyst and J. H. Cummings, Digestion of polysaccharides of potato in the small intestine of man, Am. J. Clin. Nutr., 1987, 45, 423–431 CAS.
- T. M. S. Wolever, A. L. Gibbs, M. Spolar, E. V. Hitchner and C. Heimowitz, Equivalent glycemic load (EGL): a method for quantifying the glycemic responses elicited by low carbohydrate foods, Nutr. Metab., 2006, 3, 33 CrossRef.
- C. Abraira, B. Buchanan and L. Hodges, Modification of glycogen desposition by priming glucose loads: the second-meal phenomenon, Am. J. Clin. Nutr., 1987, 45, 952–957 CAS.
- G. R. Collier, T. M. S. Wolever and D. J. A. Jenkins, Concurrent ingestion of fat and reduction in starch content impairs carbohydrate tolerance to subsequent meals, Am. J. Clin. Nutr., 1987, 45, 963–969 CAS.
- T. M. S. Wolever, A. Bentum-Williams and D. J. A. Jenkins, Physiologic modulation of plasma FFA concentrations by diet: metabolic implications in non-diabetic subjects, Diabetes Care, 1995, 18, 962–970 CrossRef CAS.
- D. J. A. Jenkins, T. M. S. Wolever, V. Vuksan, F. Brighenti, S. C. Cunnane, A. V. Rao, A. Jenkins, G. Buckley, R. Patten, W. Singer, P. Corey and R. G. Josse, “Nibbling versus gorging”: Metabolic advantages of increased meal frequency, N. Engl. J. Med., 1989, 321, 929–934 CrossRef CAS.
- D. J. A. Jenkins, T. M. S. Wolever, R. Nineham, D. L. Sarson, S. R. Bloom, J. Ahern, K. G. M. M. Alberti and T. D. R. Hockaday, Improved glucose tolerance four hours after taking guar with glucose, Diabetologia, 1980, 19, 21–24 CrossRef CAS.
- H. Liljeberg and I. Björck, Effects of a low-glycaemic index spaghetti meal on glucose tolerance and lipaemia at a subsequent meal in healthy subjects, Eur. J. Clin. Nutr., 2000, 54, 24–28 CrossRef CAS.
- M. S. Goddard, G. Young and R. Marcus, The effect of amylose content on insulin and glucose responses to ingested rice, Am. J. Clin. Nutr., 1984, 39, 388–392 CAS.
- A. Åkerberg, H. Liljeberg and I. Björck, Effects of amylose/amylopectin ratio and baking conditions on resistant starch formation and glycaemic indices, J. Cereal Sci., 1998, 28, 71–80 CrossRef CAS.
- Z. Zhou, D. L. Topping, M. K. Morell and A. R. Bird, Changes in starch physical characteristics following digestion of foods in the human small intestine, Br. J. Nutr., 2010, 104, 573–581 CrossRef CAS.
- M. Garsetti, S. Vinoy, V. Lang, S. Holt, S. Loyer and J. C. Brand-Miller, The glycemic and insulinemic index of plain sweet biscuits: relationships to in Vitro starch digestibility, J. Am. Coll. Nutr., 2005, 24, 441–447.
- S. W. Ross, J. C. Brand, A. W. Thorburn and A. S. Truswell, Glycemic index of processed wheat products, Am. J. Clin. Nutr., 1987, 46, 631–635 CAS.
- L. N. Panlasigui, L. U. Thompson, B. O. Juliano, C. M. Perez, S. H. Yiu and G. R. Greenberg, Rice varieties with similar amylose content differ in starch digestibility and glycemic response in humans, Am. J. Clin. Nutr., 1991, 54, 871–877 CAS.
- Y. Granfeldt, B. Hagander and I. Björck, Metabolic responses to starch in oat and wheat products. On the importance of food structure, incomplete gelatinization or presence of viscous dietary fibre, Eur. J. Clin. Nutr., 1995, 49, 189–199 CAS.
- S. L. Slaughter, P. R. Ellis and P. J. Butterworth, An investigation of the action of porcine pancreatic α-amylase on native and gelatinized starches, Biochim. Biophys. Acta, 2001, 1525, 29–36 CrossRef CAS.
Footnotes |
† Electronic supplementary information (ESI) available. See DOI: 10.1039/c1fo10042d |
‡ Supported by a grant from the Agricultural Bioproducts Innovation Program of Agriculture and Agri-Food Canada. |
§ Conflict of interest: T. Wolever is President and part owner of Glycemic Index Laboratories, Inc., a contract-research organization, is co-author of a range of popular books on the glycemic index under the general title of The New Glucose Revolution, and receives compensation as a consultant for McCain Foods Ltd, Canada. None of the other authors has any conflict of interest to declare. |
|
This journal is © The Royal Society of Chemistry 2011 |
Click here to see how this site uses Cookies. View our privacy policy here.