DOI:
10.1039/C1FO10003C
(Paper)
Food Funct., 2011,
2, 273-278
Lesser in vitro anaerobic cecal isoflavone disappearance was associated with greater apparent absorption of daidzein and genistein in Golden Syrian hamsters
Received
14th January 2011
, Accepted 7th April 2011
First published on 26th April 2011
Abstract
Our hypothesis in this study was that in vitro disappearance of isoflavones from fecal or cecal contents of Golden Syrian hamsters paralleled the apparent absorption of these compounds, comparable with previous findings from in vitro human fecal incubations. Two studies were conducted to test this idea: one on in vitro fecal (study 1, n = 20/sex) and the other on in vitro cecal contents (study 2, n = 10/sex) ability to degrade isoflavones. According to HPLC analysis, urinary isoflavone excretion was significantly less by 2–4 fold in males compared with females in both studies. Fecal isoflavone excretion was not significantly different between sexes or isoflavones (study 1) and was <0.5% of ingested dose. In vitro anaerobic fecal isoflavone degradation rate constants from study 1 were minimal with no significant correlation between urinary and fecal isoflavone excretion. However, in vitro anaerobic cecal isoflavone degradation rate constants (study 2) were greater and significantly correlated with urinary excretion of daidzein (R = 0.90; p = 0.01) and genistein (R = 0.93; p = 0.004), but not glycitein (R = 0.50; p = 0.3). Both male and female hamsters showed a pattern of urinary isoflavone excretion similar to that found in humans (daidzein > genistein). Hamster in vitro cecal isoflavone degradation rate constants seemed to be analogous to human in vitro fecal isoflavone degradation rate constants for genistein and daidzein. The sex difference in isoflavone excretion in hamsters and the instability in glycitein excretion across studies coupled with the paucity of human data on this isoflavone deserve further investigation.
Introduction
Dietary isoflavones are found mainly in soybeans and soy products.1 Their chemical structure is estrogen-like.2 They prevent mammary carcinogenesis in rodents,3 but the health effects of these compounds are highly variable among individuals and their bioavailability is not well understood. In humans, 1–50% of the ingested dose of isoflavones is excreted in the urine, mostly as glucuronide conjugates, whereas less than 1% is recovered in the feces. The great proportion of isoflavones not recovered or disappearing has been linked with microbial metabolism and degradation of these compounds.4,5 When isoflavones were incubated in vitro with human feces, they disappeared within 48 h. Human fecal isoflavone degradation rate constants sorted into statistically significant clusters: high, moderate or low.6 Asian women who were low isoflavone degraders had threefold greater urinary genistein excretion than did Asian high isoflavone degraders or Caucasian low or high isoflavone degraders and more rapid gut transit time (GTT6). Thus in vitro fecal isoflavone degradation and GTT interacted in predicting apparent isoflavone absorption in women. Also, in vitro human fecal isoflavone degradation rate constant predicted urinary excretion of isoflavones from ingestion of soy milk in 10 human subjects.7
Animal models need to be established to facilitate long term study of the role of isoflavone bioavailability in dietary modulation of chronic diseases. Golden Syrian hamsters deserve attention because of the hypocholesterolemic action of isoflavones in this species.8 Bravo et al.9 showed that human and hamster cholesterol metabolism is similar. Hypocholesterolemic effects of a diet containing 25% soy protein fed to 40 hamsters for 4 weeks was only observed in animals clustering statistically as high isoflavone excreters.10 This finding highlights the importance of more detailed studies of isoflavone bioavailability in hamsters, with a focus on gut microbial metabolism as a determinant of isoflavone bioavailability. Our hypothesis in this study was that disappearance of isoflavones from in vitro incubations of fecal or cecal contents in hamsters predicted isoflavone bioavailability as seen previously for in vitro human fecal incubations. To test this hypothesis, two studies were conducted on in vitro fecal or cecal contents and their ability to degrade isoflavones.
Materials and methods
Chemicals and diets
Novasoy® (ADM, Decatur, IL) was fed to hamsters as an isoflavone source; isoflavone composition was determined by HPLC.1 Total isoflavone concentration was 963 μmol kg−1 diet and daidzein, genistein and glycitein were 36%, 52% and 12% of total isoflavones respectively. The casein-based isoflavone-containing diet was formulated to contain 0.1% cholesterol and saturated fat from coconut oil (10% by weight of diet).8Isoflavones used for fecal and cecal in vitro incubations were synthesized chemically according to Chang et al.11 and Lang'at-Thoruwa et al.12 Other chemicals used for isoflavone extraction and analysis were purchased from Fisher Scientific (Pittsburg, PA) and Sigma Chem. Co. (St. Louis, MO).
Animals and study designs
The animal experimental protocols for both studies were approved by the Iowa State University Institutional Animal Care and Use Committee.
Study 1.
20 male and 20 female Golden Syrian hamsters (∼4 weeks of age), were obtained from Harlan-Teklad (Madison, WI), housed individually in a temperature-controlled room (23 °C) with a 12 h light
:
dark cycle and fed rodent chow for 2 weeks. Hamsters were then fed the isoflavone, casein-based diet ad libitum for 2 weeks and put in metabolic cages for 24 h to collect urine and feces. Body weight and food intake were monitored daily. Isoflavone analysis was performed within 48 h after urine collection and animals euthanized under CO2 less than 48 h after isoflavone extraction and HPLC analysis of all urine samples. During that time, animals were fed the same isoflavone/casein-based diet, which was withdrawn 12 h before euthanizing them under CO2. After ranking animals from lowest to highest isoflavone excretion and selecting only animals that were high or low excreters of all 3 isoflavones, then performing statistical cluster analysis to confirm groupings, contents from the large intestine of the 4 highest and 4 lowest urinary excreters of isoflavones were collected for in vitro fecal incubations. Urine could not be obtained from one male and one female over the 24 h in metabolic cages. Those hamsters were withdrawn from the experiment.
Study 2.
10 male and 10 female Golden Syrian hamsters (∼4 weeks of age), were obtained as above. Protocols and design of this study was similar to study 1, except that cecal instead of fecal contents from the three highest and lowest urinary excreters were collected for in vitro incubations, again, after selecting animals either high or low in excretion of all 3 isoflavones and verifying grouping by statistical cluster analysis. In addition, only urinary, but not fecal isoflavone analysis was performed in this study. Urine was not collectable from one male over 24 h in the metabolic cage. That hamster was withdrawn from the experiment.
Isoflavone
extraction from urine samples was done following a modified protocol established by Zhang et al.13 Five mL urine were incubated with 50 μL β-glucuronidase-sulfatase (Sigma, St. Louis, MO) at 37 °C for 20 h to obtain isoflavone aglucons. Fifty μL 2,4,4′-trihydroxydeoxybenzoin (THB) was added as internal standard. Incubation mixtures were loaded on Extrelut (TM) QE columns (EM Science, Gibbstown, NJ) and extracted with ethyl acetate. The eluent was collected, dried using a rotary evaporator (Buchï, Flawil, Switzerland) and dissolved in 9.8 mL of 20% ethanol and 0.2 mL of 1 N HCL. Five mL of this solution was loaded onto a pre-wetted Sep-pak C18 cartridge (Waters, Rainin, Woburn, MA), washed twice with 2 mL of distilled deionized water and eluted with 2 mL of 80% methanol. Samples were stored at 4 °C until HPLC analysis (12). Urinary isoflavone excretion was expressed as a percentage of the dose ingested over 24 h, to normalize data across variations in food intake and body weight.
Fecal excretion of isoflavone was measured using 2 g of ground fecal sample mixed with 10 mL acetonitrile and 2 mL of 0.1 mol L−1HCl. The mixture was stirred for 3 h, then filtered through No. 1 Whatman filter paper. The eluent was collected in a round bottom flask and evaporated using a roto-evaporator (Buchï, Flawil, Switzerland). The residue was dissolved in 2 mL ethanol, 7.8 mL ultrapure water and 200 μL of 1 mol L−1HCl. Five mL were loaded on a pre-wetted Sep-Pak C18 cartridge (Waters, Rainin, Woburn, MA), washed with 2 mL of distilled deionized water and eluted with 2 mL of 80% methanol. Samples were stored at 4 °C until HPLC analysis.
In vitro fecal and cecal incubations: isoflavone analysis
At the end of each study, animals were euthanized under CO2 and the content of the colon (study 1) or cecum (study 2) of the 3 or 4 highest and lowest urinary isoflavone excreters placed in 15 mL of anaerobic brain heart infusion media (BHI, Fisher, Chicago, IL). BHI was prepared with 50 mL 8% sodium bicarbonate/L as a buffer and 20 mL 1.25% cysteine sulfide (Sigma, St Louis, MO)/L as an oxygen indicator. The BHI containing fecal or cecal contents was vortexed for ∼15 s and 5 mL mixed with 20 mL BHI media containing a final concentration of 50 μmol each of daidzein, genistein and glycitein/L. Duplicate tubes were incubated for each fecal or cecal sample obtained as well as a negative control. After adding isoflavone, each tube was then vortexed for 10 s and duplicate samples taken immediately afterwards and frozen at −60 °C to measure isoflavone concentration at time 0. Tubes were placed at 37 °C and isoflavone concentrations over time were measured in duplicate samples from each tube at 3, 6, 9 and 12 h. All the samples were stored at −60 °C until isoflavone extraction and HPLC analysis. Incubation tubes were maintained anaerobically at all times to preserve bacterial quality.
Statistical analysis
Statistical analysis was performed using SAS (SAS Institute, version 6.12; 1998, Cary, NC). Urinary isoflavone excretion phenotypes were established using a statistical cluster analysis. Fecal and urinary isoflavone excretion was compared between clusters by one-way ANOVA and correlations between urinary and fecal isoflavone excretion and between the different isoflavones for their excretion were determined by regression analysis. All results were considered significant at p < 0.05.
Results
Body weight and food intake
Study 1.
Initial mean body weights were similar (p = 0.1) between males (122 ± 9 g) and females (117 ± 10 g). Despite similar food intakes of males and females (7.1 ± 0.7 g/d and 7.3 ± 0.5 g/d, respectively; p = 0.3), females gained significantly more weight (1.0 ± 0.3 g/d) than did males (0.7 ± 0.2 g/d; p = 0.01).
Study 2.
Mean initial body weight was similar (p = 0.09) between males (114 ± 4 g) and females (119 ± 7 g). Food intakes of males (7.6 ± 0.5 g/d) and females (7.7 ± 0.4 g/d) were similar (p = 0.4). Mean daily body weight gain was significantly different (p = 0.001) between males (0.8 ± 0.2 g/d) and females (1.1 ± 0.1 g/d).
Study 1.
Males and females differed greatly in urinary excretion of isoflavones over 24 h (Fig. 1A). In males, daidzein and glycitein excretion were similar (18 ± 8% vs. 16 ± 6% ingested dose; p = 0.3), whereas urinary excretion of genistein was significantly less (8 ± 6% ingested dose) than that of daidzein (p < 0.001) or glycitein (p < 0.001). In females, mean urinary glycitein and genistein excretion was similar (31 ± 11% vs. 27 ± 12% ingested dose; p = 0.2), whereas mean urinary excretion of daidzein was significantly greater (44 ± 14% ingested dose) than that of glycitein (p = 0.003) and genistein (p < 0.001). Overall, males had less urinary isoflavone excretion of daidzein (p < 0.001), glycitein (p < 0.001) and genistein (p < 0.001) than did females. Clustering of animals as high or low excreters based on urinary isoflavone excretion showed that females were mostly high excreters whereas males were mostly low excreters (Fig. 2; data not shown for daidzein or glycitein). Regardless of sex, urinary daidzein correlated with both glycitein and genistein (r = 0.97 and r = 0.96; p < 0.001). Urinary glycitein and genistein were also correlated (r = 0.97; p < 0.001).
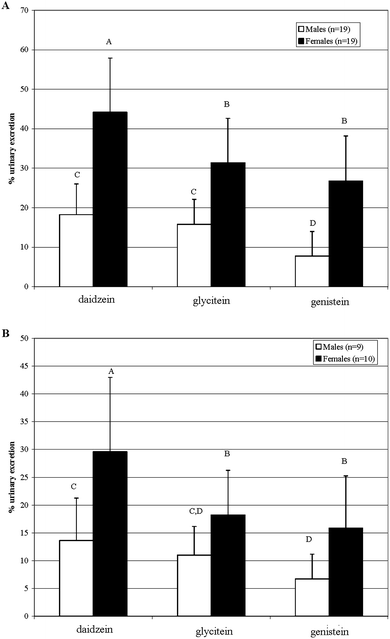 |
| Fig. 1 Comparison of urinary excretion levels between in male and female Golden Syrian hamsters; A) Study 1, B) Study 2, male □, female ■). Different letters indicate significant differences, p < 0.05. | |
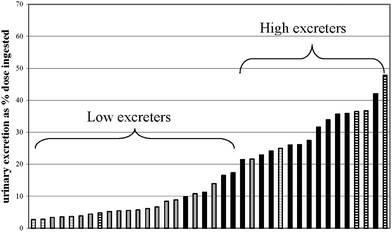 |
| Fig. 2 Ranking and cluster analysis of individual genistein urinary excretion values in male and female Golden Syrian hamsters (study 1). Grey bars indicate male hamsters; black bars indicate female hamsters; horizontal stripes indicate animals selected for fecal in vitro incubations. | |
Study 2.
In males, urinary daidzein and glycitein excretion were similar over 24 h (14 ± 8% vs. 11 ± 5% ingested dose; p = 0.4), whereas urinary genistein excretion was significantly less (7 ± 5% ingested dose) than that of daidzein (p = 0.03) but not glycitein (p = 0.07). In females, mean urinary excretion of glycitein and genistein was similar (18 ± 8% vs. 16 ± 9% ingested dose; p = 0.54), whereas mean urinary excretion of daidzein was significantly greater (30 ± 13% ingested dose) than glycitein (p = 0.03) or genistein (p = 0.01). Males had less urinary isoflavone excretion than did females for each isoflavone (Fig. 1B). As observed in study 1, clustering of animals as high and low urinary isoflavone excreters showed that females were mostly high excreters whereas males were mostly low excreters (Fig. 3; data shown only for genistein).
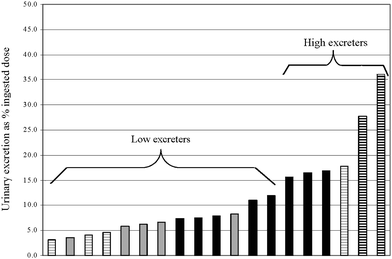 |
| Fig. 3 Ranking and cluster analysis of individual genistein urinary excretion values in male and female Golden Syrian hamsters (study 2). Grey bars indicate male hamsters; black bars indicate female hamsters; horizontal stripes indicate animals selected for fecal in vitro incubations. | |
In study 1, fecal isoflavone excretion was analyzed for each animal over 24 h with less than 0.5% of ingested isoflavones present in feces. There was no significant difference between fecal isoflavones within sex or for the same isoflavone between sexes (data not shown). Fecal isoflavone excretion was not significantly correlated with urinary isoflavone excretion (regardless of sex) for daidzein (r = 0.35; p = 0.24), glycitein (r = 0.16; p = 0.6) and genistein (r = 0.29; p = 0.34). But among the three isoflavones, fecal daidzein excretion correlated significantly with that of glycitein (r = 0.92; p < 0.001) and genistein (r = 0.91; p < 0.001); fecal glycitein correlated with genistein (r = 0.86; p < 0.001).
Correlation between in vitro anaerobic fecal degradation and urinary excretion of isoflavones
From study 1, feces from 4 high urinary excreters (M1, F7, F9, F14) and 4 low urinary excreters (M20, M16, M18, F4) were assessed for in vitro isoflavone degradation rate. Animals were selected based on their combined urinary excretion of the three isoflavones. Mean in vitro fecal isoflavone degradation rate constants did not differ between high and low urinary excreters of daidzein, glycitein or genistein (Table 1). Across urinary isoflavone excretion phenotypes, mean fecal in vitrodegradation rate of genistein (0.28 ± 0.14 h−1; n = 8) was significantly greater than that of daidzein (0.012 ± 0.006 h−1; p < 0.001) or glycitein (0.03 ± 0.016 h−1; p < 0.001). In addition, overall mean fecal degradation rate of daidzein was significantly less than that of glycitein (p = 0.008). Correlations between in vitro fecal degradation rate constants and urinary excretion were not statistically significant for daidzein (r = 0.49; p = 0.21), glycitein (r = 0.31; p = 0.44) or genistein (r = 0.33; p = 0.41). Correlations between in vitro fecal degradation rate constants and fecal isoflavone excretion were not significant for daidzein (r = 0.5; p = 0.19), glycitein (r = 0.28; p = 0.49) or genistein (r = 0.16; p = 0.69).
Table 1 Relation between urinary isoflavone excretion and in vitro fecal or cecal isoflavone degradation rate constantsa
Correlation between in vitro cecal degradation and urinary excretion of isoflavone
In study 2, cecal contents of 3 high (F1, F9, M5) and 3 low urinary isoflavone excreters (M2, M8, M9) were assessed for in vitro isoflavone degradation. Animals were selected based on their combined urinary excretion of the three isoflavones. Mean cecal degradation rate constants were significantly different between high and low urinary excreters of daidzein and genistein, whereas mean cecal glycitein degradation rate constants of high and low urinary isoflavone excreters did not differ (Table 1). For both high and low urinary isoflavone excreters, genistein was significantly more degraded in vitro than daidzein (p = 0.003 (high), p < 0.001 (low)) or glycitein (p = 0.01 (high), p = 0.001 (low)) whereas cecal degradation of glycitein and daidzein did not differ (p = 0.07 (high), p =0.10 (low)). Correlations between in vitro cecal degradation rate constants and urinary excretion were statistically significant for daidzein (r = 0.90; p = 0.01) and genistein (r = 0.93; p = 0.004), but not for glycitein (r = 0.50; p = 0.3, Fig. 4, data shown only for genistein).
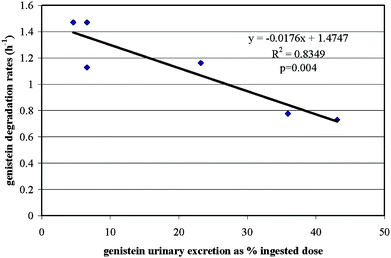 |
| Fig. 4 Correlation between genisteinin vitro cecal degradation rate constants and in vivo urinary excretion data between high (n = 3) and low (n = 3) urinary excreters (study 2). | |
Discussion
This study examined the relevance to humans of Golden Syrian hamsters as a model for gut microbial influences on the apparent absorption of isoflavones. Despite initial body weight and similar daily food intake, females gained significantly more weight on a daily basis compared with males. This unusual sexual dimorphism is documented but not well explained, and could be an obstacle to the use of hamsters as models for human metabolic conditions.
Urinary excretion of isoflavones showed surprising differences within and between studies. Whereas a similar pattern was observed between studies in females (daidzein > glycitein = genistein), urinary isoflavone excretion was less in study 2 compared to study 1 for all isoflavones. Study 1 may have more relevance than study 2 due to the greater numbers of individuals. But, 50% of females in study 2 were low urinary isoflavone excreters (Fig. 3) compared with 25% of females in study 1 (Fig. 2), which may have explained the lesser overall isoflavone excretion observed in study 2 (Fig. 3). Although gut microbes were not studied in these hamster studies, human studies6 suggest that the greater proportion of low urinary isoflavone excreters in study 2 may be due to differences in gut microbes between studies. Although urinary excretion of glycitein in males shifted from being similar to daidzein and greater than genistein in study 1 to not differing from the other isoflavones in study 2, urinary daidzein excretion was greater than that of genistein in both sexes and both studies. A similar pattern of greater urinary daidzein than genistein was observed in humans across several bioavailability studies.12–14
Sex differences in isoflavone excretion were observed in both studies, with males being lesser urinary excreters of all three isoflavones compared with females (Fig. 1). Zhang et al.13 showed 20% less urinary excretion of isoflavones from single doses of soymilk or soygerm in 7 men than in 7 women. During a one month feeding study, 6 women excreted 24 and 66% of the ingested doses of genistein and daidzein, respectively, whereas 6 men excreted 15 and 47% of ingested dose of genistein and daidzein, respectively.14 After one month, women excreted 14% less daidzein and 45% less genistein in urine than at time 0, whereas in men, urinary isoflavone excretion was stable. Urinary isoflavones in women were within the same range observed in female hamsters (Fig. 1) for daidzein (∼60 vs. 40% of ingested dose) and genistein (∼20 vs. ∼20% ingested dose).15 Urinary isoflavones for men15 and male hamsters seemed different for daidzein (∼47 vs. ∼15% ingested dose) but were fairly similar for genistein (∼15 vs. ∼8% ingested dose). Thus, urinary excretion was more similar to humans for female than for male hamsters. But Wiseman et al.16 found no sex differences in daidzein and genistein excretion in 19 men and 19 women after 10 weeks of soy intake. Overall, human sex differences in isoflavone excretion seem less pronounced than that observed in hamsters.
The present data in male hamsters were similar to that of another rodent model. King17 compared the bioavailability of daidzein and genistein in male Wistar rats fed a soy extract providing 74 μmol genistein and 77 μmol daidzein/kg body weight. Urinary excretion over 48 h was 17 ± 1% (∼15% in the present study) and 12 ± 1% (∼8% here) of ingested daidzein and genistein, respectively, whereas fecal excretion was 2 ± 1% and 3 ± 1% of ingested dose of these isoflavones. Given the highly variable isoflavone excretion reported across human studies,4,13,14,16 the overall apparent absorption of isoflavones in hamsters seems generally consistent with that seen in humans.
Fecal isoflavone excretion in study 1 did not show sex differences, with very low levels of excretion (0.1 to 0.5% ingested dose). Up to 3.5% of ingested isoflavone dose was found in fecal sample from rats17 whereas women who excreted more urinary isoflavones also excreted greater fecal isoflavones (up to 6% of ingested dose) compared with lower urinary excreters.4 Thus, we expected greater fecal isoflavones in female than in male hamsters. But there was no significant sex difference in fecal isoflavones, nor was there a significant correlation between fecal and urinary excretion of individual isoflavones. Positive correlations were found between isoflavone excretion in human urine and feces.4,13 Because human fecal18 and hamster cecal isoflavone degradation rate constants were similar, but limited hamster fecal isoflavone degradation also occurs, the seemingly lesser fecal recovery of isoflavones in hamsters than in humans probably results from combined cecal and fecal isoflavone disappearance.
In vitro anaerobic fecal degradation of isoflavones may predict apparent absorption of isoflavones in humans.4,6,13,19,20 Subjects who had high in vitro isoflavone degradation were low urinary excreters whereas those who degraded lesser amounts of isoflavonein vitro excreted more urinary isoflavones. Our hypothesis that in vitro fecal isoflavone degradation in hamsters would correlate with urinary excretion pattern was incorrect. Fresh hamster feces collected from the large intestine of high and low urinary isoflavone excreters showed low in vitro isoflavone degradation rate constants, not exceeding 0.015, 0.030 and 0.300 for daidzein, glycitein and genistein respectively, whereas in humans, in vitro fecal isoflavone degradation rate constants were 0.299 and 0.400 for daidzein and genistein, respectively.19 Only genistein degradation seemed to reach levels that indicated significant hamster fecal bacterial isoflavone degradation, which may have partly explained the lesser apparent absorption of genistein, but the absence of correlation with urinary isoflavone excretion and very low in vitro fecal degradation of daidzein and glycitein led us to the conclusion that in vitro fecal degradation assay was of limited use to predict isoflavone bioavailability in hamsters. Cecal isoflavone degradation in study 2 was apparently greater than was fecal isoflavone degradation in study 1, and the significantly lesser cecal degradation rates of daidzein and genistein in 3 low degraders compared with 3 high degraders paralleled the significantly greater urinary isoflavone excretion in the low degraders compared with the high degraders (Table 1). Significant correlations were found between urinary isoflavone excretion and in vitro cecal degradation rates of daidzein and genistein, similar to that seen for human urinary isoflavone excretion and in vitro fecal isoflavone degradation. Therefore, in vitro cecal isoflavone degradation rather than fecal degradation in hamsters is more metabolically equivalent to human in vitro fecal isoflavone degradation.
In vitro cecal degradation of glycitein was not predictive of urinary glycitein excretion. The cecal glycitein degradation rate constants were more variable than the other cecal isoflavone degradation rate constants and the difference between the two excretion phenotypes in urinary excretion of glycitein was less than that seen for the other two isoflavones. This suggests different hamster gut microbial dynamics for glycitein degradation than for the degradation of the other isoflavones. In one human subject, we found that fecal glycitein degradation rate but not degradation rate constants of other isoflavones changed markedly over one month (data not shown). The limited human bioavailability data on glycitein and these findings in hamsters support the need for further investigation of this isoflavone in both species.
Conclusions
The present study indirectly investigated the concept that gut microbial degradation of isoflavones predicts isoflavone bioavailability by associating in vitro fecal and cecal degradation rate constants of isoflavones with urinary isoflavone excretion in hamsters. This approach showed similar patterns of apparent absorption of the two major isoflavones, daidzein and genistein, as found in humans, in whom vitro fecal degradation of isoflavones was compared with urinary isoflavone excretion,6,7,19,20 insofar as hamster cecal isoflavone degradation was similar to human fecal isoflavone degradation in predicting apparent isoflavone absorption. The implication of this finding is that prescreening hamsters for predictors of apparent isoflavone absorption (e.g., gut microbial species yet to be determined) may facilitate exploration of the long term health effects of interindividual variation in isoflavone uptake,10 and related compounds, with a strong likelihood of developing health concepts transferrable to human circumstances.
References
- P. Murphy, T. Song, G. Buseman and K. Barua, J. Agric. Food Chem., 1997, 45, 4635–4638 CrossRef CAS.
-
K. D. R. Setchell and H. Adlercreutz, in Role of the gut flora in toxicity and cancer, I. Rowland, ed., Academic Press, London, UK, 1988, pp. 315–345 Search PubMed.
- C. A. Lamartiniere, M. S. Cotroneo, W. A. Fritz, J. Wang, R. Mentor-Marcel and A. Elgavish, J. Nutr., 2002, 132, 552S–558S.
- X. Xu, K. S. Harris, H. J. Wang, P. A. Murphy and S. Hendrich, J. Nutr., 1995, 125, 2307–2315 CAS.
- H. G. Hur, J. O. Lay, R. D. Beger, J. P. Freeman and F. Rafii, Arch. Microbiol., 2000, 174, 422–428 CrossRef CAS.
- Y. Zheng, J. Hu, P. A. Murphy, D. L. Alekel, W. D. Franke and S. Hendrich, J. Nutr., 2003, 133, 3110–3116 CAS.
- A. L. Simons, M. Renouf, P. A. Murphy and S. Hendrich, J. Agric. Food Chem., 2009, 58, 141–147.
- T. Song, S. O. Lee, P. A. Murphy and S. Hendrich, Exp. Biol. Med. (Maywood, NJ, U. S.), 2003, 228, 1063–1068 Search PubMed.
- E. Bravo, A. Cantafora, A. Calcabrini and G. Ortu, Comp. Biochem. Physiol., Part B: Biochem. Mol. Biol., 1994, 107, 347–355 CrossRef.
- Z. Ye, M. Renouf, S. O. Lee, C. C. Hauck, P. A. Murphy and S. Hendrich, J. Nutr., 2006, 136, 2773–2778 CAS.
- Y.-C. Chang, M. G. Nair, R. C. Santell and W. G. Helferich, J. Agric. Food Chem., 1994, 42, 1869–1871 CrossRef CAS.
- C. Lang'at-Thoruwa, T. T. Song, J. Hu, A. L. Simons and P. A. Murphy, J. Nat. Prod., 2002, 66, 149–151.
- Y. Zhang, G. J. Wang, T. T. Song, P. A. Murphy and S. Hendrich, J. Nutr., 1999, 129, 957–962 CAS.
- L. J. Lu, J. J. Grady, M. V. Marshall, V. M. Ramanujam and K. E. Anderson, Nutr. Cancer, 1995, 24, 311–323 Search PubMed.
- L. J. Lu and K. E. Anderson, Am. J. Clin. Nutr., 1998, 68, 1500S–1504S CAS.
- H. Wiseman, K. Casey, E. A. Bowey, R. Duffy, M. Davies, I. R. Rowland, A. S. Lloyd, A. Murray, R. Thompson and D. B. Clarke, Am. J. Clin. Nutr., 2004, 80, 692–699 CAS.
- R. A. King, Am. J. Clin. Nutr., 1998, 68, 1496S–1499S CAS.
- A. L. Simons, M. Renouf, S. Hendrich and P. A. Murphy, J. Agric. Food Chem., 2005, 53, 4258–4263 CrossRef CAS.
-
S. Hendrich, G.-J. Wang, X. Xu, B. Y. Tew, H.-J. Wang and P. A. Murphy, in Functional Foods. ACS Monograph, ed. T. Shibamoto, ACS Books, Washington, DC, 1998, pp. 150–155 Search PubMed.
- Y. Zheng, S. O. Lee, M. A. Verbruggen, P. A. Murphy and S. Hendrich, J. Nutr., 2004, 134, 2534–2539 CAS.
Footnote |
† Supported by a Bailey Career Research Development Award, Iowa State University. |
|
This journal is © The Royal Society of Chemistry 2011 |