DOI:
10.1039/C0SC00250J
(Edge Article)
Chem. Sci., 2010,
1, 499-501
Catalytic asymmetric synthesis of cyclic amino acids and alkaloid derivatives: application to (+)-dihydropinidine and Selfotel synthesis†
Received 2nd April 2010, Accepted 4th June 2010
First published on 2nd July 2010
Organocatalysis is a highly promising tool for the rapid assembly of natural products and biologically active compounds.1 In this area, chiral quaternary ammonium salts are frequently utilized as phase-transfer catalysts for the asymmetric synthesis of non-proteinogenic amino acids.2 Recently, we have developed an organocatalytic approach for the asymmetric one-pot synthesis of pyrrolidine derivatives through phase-transfer catalyzed asymmetric 1,4-addition and subsequent reductive amination.3 With this method, however, other cyclic amino acid derivatives with a larger ring size (e.g. pipecolic acid) could not be accessed in spite of their synthetic and pharmacological importance.4 Accordingly, we have been interested in applying the powerful phase-transfer catalyzed asymmetric alkylation as the initial C–C bond forming reaction to prepare cyclic amino acids with several different ring sizes (Scheme 1). Here we wish to report our study on this subject, targeting the catalytic asymmetric synthesis of a wide variety of cyclic amino acid and alkaloid derivatives starting from readily available glycine ester and alanine ester with a combination of phase-transfer catalyzed asymmetric alkylation and subsequent diastereoselective reductive amination.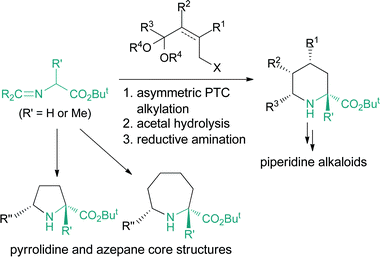 |
| Scheme 1 Asymmetric synthesis of cyclic amino acids. | |
We first examined the asymmetric alkylation of N-(diphenylmethylene)glycine ester 1 and alkyl halide 3a (n = 1) by using a chiral phase transfer catalyst of type (S)-25 (Table 1). Attempted reaction of glycine derivative 1 and alkyl bromide 3a with CsOH in the presence of 1 mol% of catalyst (S)-2a in toluene at −20 °C gave alkylation product 4a (n = 1) in 46% yield with 88% ee (entry 1).6 While use of (S)-2b improved the yield (entry 2), a sterically more hindered catalyst (S)-2c was not as effective as (S)-2a (entry 3). Lowering the reaction temperature improved the enantioselectivity (entry 4). Using a decreased amount of CsOH and an increased amount of 3a, the desired 4a was obtained in a satisfactory yield with virtually complete enantioselectivity (entries 6 and 7). With the optimal reaction conditions for asymmetric alkylation in hand, we carried out the acetal hydrolysis of 4a with CF3CO2H (3 equiv.) in aqueous EtOH at room temperature and subsequent intramolecular reductive amination with Pd on carbon under a H2 atmosphere at 40 °C to furnish 2,6-disubstituted cis-piperidine 5a stereospecifically in 88% yield (Scheme 2).7
Table 1 Asymmetric alkylation of glycine ester 1 with (S)-2a–c under phase transfer conditionsa
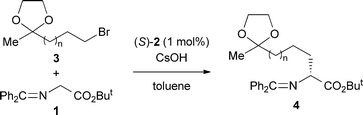
|
---|
Entry | n | Catalyst | Conditions (°C, h) | Yield (%)b | ee (%)c |
---|
Unless otherwise specified, the reaction was carried out with glycine derivative 1 and 5 equiv. of alkyl bromide 3 in the presence of 1 mol% of (S)-2a–c, and 5 equiv. of CsOH under the given reaction conditions. Isolated yield. Determined by HPLC analysis using a chiral column (Chiralpak AD-H, Daicel Chemical Industries, Ltd). 2.5 equiv. of CsOH. 10 equiv. of 3. 2 mol% of (S)-2a. |
---|
1 | 1 | (S)-2a | −20, 6 | 46 | 88 |
2 | 1 | (S)-2b | −20, 6 | 58 | 85 |
3 | 1 | (S)-2c | −20, 6 | 36 | 39 |
4 | 1 | (S)-2a | −40, 18 | 31 | 98 |
5d | 1 | (S)-2a | −40, 20 | 50 | 97 |
6d,e | 1 | (S)-2a | −40, 20 | 79 | 99 |
7d,e,f | 1 | (S)-2a | −40, 16 | 85 | 99 |
8d,e,f | 2 | (S)-2a | −40, 20 | 81 | 98 |
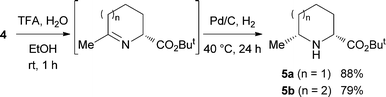 |
| Scheme 2 Synthesis of disubstituted cyclic amino acids 5. | |
Under similar conditions, the reaction of alkyl bromide 3b (n = 2) with a longer alkyl chain was examined. The reaction of 3b proceeded smoothly to afford the corresponding alkylated product in good yield with excellent enantioselectivity (Table 1, entry 8), and the following cyclization gave 2,7-disubstituted cis-azepane 5b. In both cases in Scheme 2, their minor diastereomers were not detected. When N-(4-chlorophenylmethylene)alanine ester 6 was used instead of glycine ester 1, 2,2,6-trisubstituted piperidine 7 was obtained with excellent diastereo- and enantioselectivity (Scheme 3).
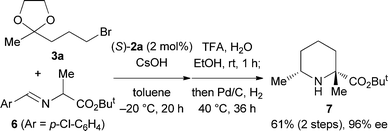 |
| Scheme 3 Synthesis of 2,2,6-trisubstituted piperidine 7. | |
Our approach was highlighted by the short synthesis of the naturally occurring alkaloid (+)-dihydropinidine8 in a highly stereoselective manner as shown in Scheme 4. Most of the previous asymmetric syntheses of dihydropinidine have generally involved the use of auxiliaries derived from the chiral pool or chiral starting compounds, and our approach via a catalytic asymmetric transformation represents a rare example.
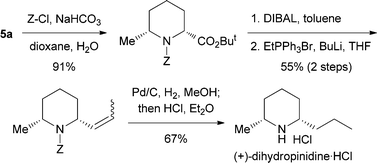 |
| Scheme 4 Asymmetric synthesis of (+)-dihydropinidine. | |
We then turned our attention to the stereoselective synthesis of 2,5,6-trisubstituted piperidines 12 using racemic alkyl bromides 8 which have an alkyl branch (R2) (Scheme 5). It is known that compounds of type 10 can be epimerized via the enamine tautomer 11 and the stereochemistry at the 5-position of 2,5,6-trisubstituted piperidines 12, in addition to the 2- and 6-positions, is controllable in the reductive amination.9 Indeed, treatment of the alkylation products with CF3CO2H in aqueous EtOH and then the catalytic hydrogenation with Pd on carbon under a H2 atmosphere produced 2,5,6-trisubstituted piperidines 12 stereoselectively as expected. In addition, stereoselective synthesis of 2,4-disubstituted piperidines 1510 has also been realized by using 2-substituted allyl bromides 13 (Scheme 6).
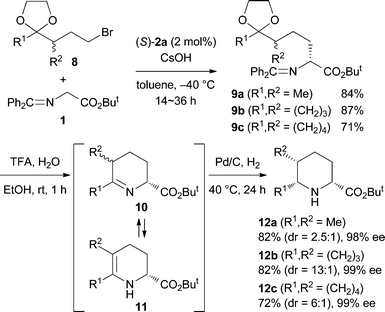 |
| Scheme 5 Stereoselective synthesis of 2,5,6-trisubstituted piperidines 12. | |
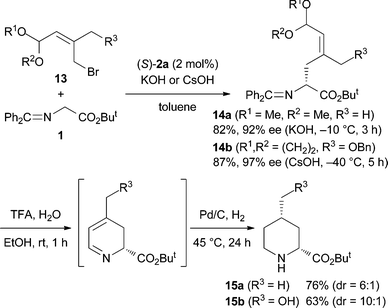 |
| Scheme 6 Synthesis of 2,4-disubstituted piperidines 15. | |
The synthetic utility of this method was demonstrated in the asymmetric synthesis of Selfotel (CGS-19755),11 which is a potent NMDA receptor antagonist,12 as shown in Scheme 7. To the best of our knowledge, this approach represents the first synthesis of Selfotel involving a catalytic asymmetric transformation.
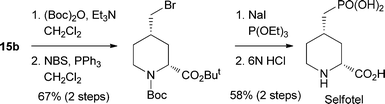 |
| Scheme 7 Asymmetric synthesis of Selfotel. | |
In summary, we were successfully able to develop an asymmetric synthesis of piperidine and azepane core structures starting from a readily available glycine ester by the combination of a phase-transfer catalyzed asymmetric alkylation and a subsequent diastereoselective reductive amination. This approach allows the facile synthesis of (+)-dihydropinidine and Selfotel.
Notes and references
- For reviews, see:
(a) P. I. Dalko and L. Moisan, Angew. Chem., Int. Ed., 2001, 40, 3726 CrossRef CAS;
(b) P. I. Dalko and L. Moisan, Angew. Chem., Int. Ed., 2004, 43, 5138 CrossRef CAS;
(c) A. Berkessel and H. Gröger, Asymmetric Organocatalysis, Wiley-VCH, Weinheim, 2005 Search PubMed;
(d) Enantioselective Organocatalysis, ed. P. I. Dalko, Wiley-VCH, Weinheim, 2007 Search PubMed;
(e) H. Pellissier, Tetrahedron, 2007, 63, 9267 CrossRef CAS.
- For reviews, see:
(a) T. Shioiri, in Handbook of Phase Transfer Catalysis, ed. Y. Sasson and R. Neumann, Blackie Academic & Professional, London, 1997, ch. 14 Search PubMed;
(b) S. Ebrahim and M. Wills, Tetrahedron: Asymmetry, 1997, 8, 3163 CrossRef CAS;
(c) I. A. Esikova, T. S. Nahreini, and M. J. O'Donnell, in Phase-Transfer Catalysis, ed. H. E. Halpern, ACS Symposium Series 659, American Chemical Society, Washington, DC, 1997, ch. 7 Search PubMed;
(d) T. Shioiri, A. Ando, M. Masui, T. Miura, T. Tatematsu, A. Bohsako, M. Higashiyama and C. Asakura, in Phase-Transfer Catalysis, ed. M. E. Halpern, ACS Symposium Series 659, American Chemical Society, Washington, DC, 1997, ch. 11 Search PubMed;
(e) M. J. O'Donnell, in Catalytic Asymmetric Synthesis, 2nd edn, ed. I. Ojima, Wiley-VCH, New York, 2000, ch. 10 Search PubMed;
(f) M. J. O'Donnell, Aldrichimica Acta, 2001, 34, 3 CAS;
(g) K. Maruoka and T. Ooi, Chem. Rev., 2003, 103, 3013 CrossRef CAS;
(h) T. Ooi and K. Maruoka, Angew. Chem., Int. Ed., 2007, 46, 4222 CrossRef CAS.
- Y.-G. Wang, T. Kumano, T. Kano and K. Maruoka, Org. Lett., 2009, 11, 2027 CrossRef CAS.
- For reviews, see:
(a) P. D. Bailey, P. A. Millwood and P. D. Smith, Chem. Commun., 1998, 633 RSC;
(b) F. Couty, Amino Acids, 1999, 16, 297 CrossRef CAS;
(c) M. G. P. Buffat, Tetrahedron, 2004, 60, 1701 CrossRef CAS;
(d) C. Kadouri-Puchot and S. Comesse, Amino Acids, 2005, 29, 101 CrossRef CAS.
-
(a) K. Kitamura, S. Shirakawa and K. Maruoka, Angew. Chem., Int. Ed., 2005, 44, 1549 CrossRef CAS;
(b) M. Kitamura, S. Shirakawa, Y. Arimura, X. Wang and K. Maruoka, Chem.–Asian J., 2008, 3, 1702 CrossRef CAS.
- Under similar conditions, the reaction between 1 and the corresponding alkyl iodide gave 4a with slightly lower enantioselectivity (74%, 85% ee).
-
(a) M. E. Swarbrick, F. Gosselin and W. D. Lubell, J. Org. Chem., 1999, 64, 1993 CrossRef CAS;
(b) F. A. Davis, H. Zhang and S. H. Lee, Org. Lett., 2001, 3, 759 CrossRef CAS;
(c) H. Takahata and M. Shimizu, Amino Acids, 2003, 24, 267 CrossRef CAS and references therein.
-
(a) R. K. Hill and T. Yuri, Tetrahedron, 1977, 33, 1569 CrossRef CAS;
(b) L. Guerrier, J. Royer, D. S. Grierson and H.-P. Husson, J. Am. Chem. Soc., 1983, 105, 7754 CrossRef;
(c) E. Theodorakis, J. Royer and H.-P. Husson, Synth. Commun., 1991, 21, 521 CAS;
(d) H. Takahata, H. Bandoh, M. Hanayama and T. Momose, Tetrahedron: Asymmetry, 1992, 3, 607 CrossRef CAS;
(e) Z.-H. Lu and W.-S. Zhou, J. Chem. Soc., Perkin Trans. 1, 1993, 593 RSC;
(f) E. Lee, T. S. Kang, B. J. Joo, J. S. Tae, K. S. Li and C. K. Chung, Tetrahedron Lett., 1995, 36, 417 CrossRef CAS;
(g) R. Chênevert and M. Dickman, J. Org. Chem., 1996, 61, 3332 CrossRef CAS;
(h) T. Momose, M. Toshima, N. Toyooka, Y. Hirai and C. H. Eugster, J. Chem. Soc., Perkin Trans. 1, 1997, 1307 RSC;
(i) O. Muraoka, B.-Z. Zheng, K. Okumura, E. Tabata, G. Tanabe and M. Kubo, J. Chem. Soc., Perkin Trans. 1, 1997, 113 RSC;
(j) A. R. Katritzky, G. Qiu, B. Yang and P. J. Steel, J. Org. Chem., 1998, 63, 6699 CrossRef CAS;
(k) F. A. Davis and J. M. Szewczyk, Tetrahedron Lett., 1998, 39, 5951 CrossRef CAS;
(l) T. Yamauchi, H. Fujikura, K. Higashiyama, H. Takahashi and S. Ohmiya, J. Chem. Soc., Perkin Trans. 1, 1999, 2791 RSC;
(m) T. J. Wilkinson, N. W. Stehle and P. Beak, Org. Lett., 2000, 2, 155 CrossRef CAS;
(n) S. Ciblat, P. Besse, V. Papastergiou, H. Veschambre, J.-L. Canet and Y. Troin, Tetrahedron: Asymmetry, 2000, 11, 2221 CrossRef CAS;
(o) S. Fréville, P. Delbecq, V. M. Thuy, H. Petit, J. P. Célérier and G. Lhommet, Tetrahedron Lett., 2001, 42, 4609 CrossRef CAS;
(p) M. Amat, N. Llor, J. Hidalgo, C. Escolano and J. Bosch, J. Org. Chem., 2003, 68, 1919 CrossRef CAS;
(q) C. Shu and L. S. Liebeskind, J. Am. Chem. Soc., 2003, 125, 2878 CrossRef CAS;
(r) B. Kranke, D. Hebrault, M. Schultz-Kukula and H. Kunz, Synlett, 2004, 671 CAS;
(s) L. F. Roa, D. Gnecco, A. Galindo and J. L. Terán, Tetrahedron: Asymmetry, 2004, 15, 3393 CrossRef CAS;
(t) S. Yamauchi, S. Mori, Y. Hirai and Y. Kinoshita, Biosci., Biotechnol., Biochem., 2004, 68, 676 CrossRef CAS;
(u) X. Wang, Y. Dong, J. Sun, X. Xu, R. Li and Y. Hu, J. Org. Chem., 2005, 70, 1897 CrossRef CAS;
(v) L. C. Pattenden, R. A. J. Wybrow, S. A. Smith and J. P. A. Harrity, Org. Lett., 2006, 8, 3089 CrossRef CAS;
(w) J. C. González-Gómez, F. Foubelo and M. Yus, Synlett, 2008, 2777 CAS.
- M. E. Swarbrick and W. D. Lubell, Chirality, 2000, 12, 366 CrossRef CAS.
-
(a) G. R. Proctor and F. J. Smith, J. Chem. Soc., Perkin Trans. 1, 1981, 1754 RSC;
(b) P. D. Bailey, R. D. Wilson and G. R. Brown, J. Chem. Soc., Perkin Trans. 1, 1991, 1337 RSC;
(c) P. D. Bailey, G. R. Brown, F. Korber, A. Reed and R. D. Wilson, Tetrahedron: Asymmetry, 1991, 2, 1263 CrossRef CAS;
(d) C. Alegret, F. Santacana and A. Riera, J. Org. Chem., 2007, 72, 7688 CrossRef CAS.
-
(a) J. W. Skiles, P. P. Giannousis and K. R. Fales, Bioorg. Med. Chem. Lett., 1996, 6, 963 CrossRef CAS;
(b) P. Etayo, R. Badorrey, M. D. Díaz-de-Villegas and J. A. Gálvez, Synlett, 2006, 2799 CAS.
- W. E. Childers Jr. and R. B. Baudy, J. Med. Chem., 2007, 50, 2557 CrossRef.
Footnote |
† Electronic supplementary information (ESI) available: Experimental details. See DOI: 10.1039/c0sc00250j |
|
This journal is © The Royal Society of Chemistry 2010 |