Molecular insights on the evolution of the lateral and head lantern luciferases and bioluminescence colors in Mastinocerini railroad-worms (Coleoptera: Phengodidae)
Received 11th August 2009, Accepted 28th October 2009
First published on 1st December 2009
Abstract
Among bioluminescent beetles of Elateroidea superfamily, railroad-worms (Phengodidae) produce the widest range of colors, from green to red, using the same luciferin–luciferase system. Members of the Mastinocerini tribe display additional unique cephalic organs that emit red-shifted light, with Phrixothrix railroad-worms being the most dramatic cases with head lanterns emitting red light. Although the luciferases from the head lanterns of Phrixothrix hirtus and from the lateral lanterns of P. vivianii were previously cloned, the luciferases from both lanterns of the same species were not cloned yet. Therefore the origin and evolution of head and lateral lanterns luciferases in Phengodidae remains unknown. In the present work, we cloned by PCR the cDNA for lateral lantern luciferases of three Mastinocerini species: Phrixothrix hirtus, Brasilocerus sp3. and Taximastioncerus sp. The results suggest that the head and lateral lanterns luciferases in Mastinocerini are coded by paralogous genes, and that the ancestral luciferase in the Phengodinae subfamily produced green bioluminescence. The evolutionary history of bioluminescence colors within Phengodinae is discussed.
Introduction
One of the most intriguing questions about beetle bioluminescence is the evolution of bioluminescence colors. In beetles, bioluminescence is found mainly in Elateroidea superfamily, which includes fireflies (Lampyridae), railroad-worms (Phengodidae) and click beetles (Elateridae). Despite sharing the same luciferin–luciferase system, with homologous luciferases catalyzing the ATP-dependent oxidation of a benzothiazolic luciferin,1,2 it is still unclear whether bioluminescence evolved once from a common ancestor, or if it arose independently many times in different families.1–6 Several beetle luciferases have already been cloned and sequenced1,2,7 and the three-dimensional structure of firefly luciferases was solved in the absence and presence of substrate analogs,8,9 showing important information about the active site structure. Recent theoretical and experimental studies suggest that the polarity of the active site and specific interactions with the excited oxyluciferin phenolate may play a major role in bioluminescence color determination.10–14Among bioluminescent beetles, railroad-worms are certainly the most spectacular cases, displaying the widest range of bioluminescence colors, from green to red, with the most dramatic case found in Phrixothrix species which display green and red bioluminescence through lateral and head lanterns, respectively.15
The family of Phengodidae is divided in two subfamilies: the American Phengodinae and the Asiatic Rhagophthalminae, although the taxonomical position of the latter as an independent family is still discussed.4,16,17,19,20 Although all members of these subfamilies have larvae and females with dorsal lateral lanterns usually emitting green and yellow-green light, only the Neotropical tribe Mastinocerini within the Phengodinae subfamily, which include the true railroad-worms, have cephalic lanterns displaying red-shifted light.
Despite the cloning of luciferases from the genus Phengodes,21 the green emitting from the lateral lanterns of Phrixothrix vivianii and the red emitting from the head lanterns of Phrixothrix hirtus,22 the luciferases from both lanterns of the same species of Mastinocerini have not been cloned yet. Therefore, it is unclear whether the head and lateral lantern luciferases in Mastinocerini railroad-worms are more similar between themselves, despite the different colors and lanterns, or to the respective lantern luciferases in different species. Furthermore, the evolution of bioluminescence in Phengodidae remains enigmatic.
Thus, in order to investigate the origin and evolution of the luciferases from these lanterns in Phengodidae, we cloned by PCR three new luciferases from the lateral lanterns of Phrixothrix hirtus, Brasilocerus sp3. and Taximastinocerus sp. (Fig. 1) and performed their phylogenetic analysis.
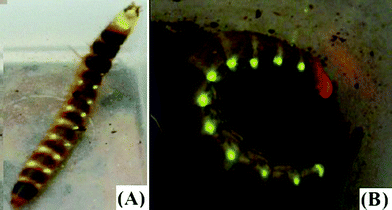 |
| Fig. 1 Mastinocerini railroad-worms: (A) Brasilocerus sp3. (Phengodinae: Mastinocerini); (B) Phrixothrix hirtus (Phengodinae: Mastinocerini). | |
Materials and methods
Insects
Larvae were collected during spring and summer nights. Brasilocerus sp3. larvae were collected at the Biological Station of Boracéia (São Paulo University), an Atlantic rain forest remnant located in Salesópolis (São Paulo, Brazil). Larvae of P. hirtus were previously collected in “cerradão” formation at Fazenda Sta Cruz (Costa Rica prefecture, MS, Brazil), and a cDNA library was previously constructed.22 One larva from the genus Taximastioncerus was also collected in “cerradão” formation in Corumbataí, SP. Larvae were identified according to Viviani and Bechara.15cDNA cloning
The cDNA libraries were constructed from the lateral lanterns of Phrixothrix hirtus (previous work),22Brasilocerus sp. and Taximastioncerus sp. The cDNA for luciferases were amplified and re-amplified using specific primers (P34F: 5′GATCAAAATGGAAGAAGAAAAC 3′; P1734R: 5′ AATGAATGTATTAGGTTCTAAAA 3′) and PCR Master Mix (Promega), in accordance with the manufacturer’s protocol and under the following conditions: 2′ at 94 °C; 25 cycles of 45′′ at 94 °C, 45′′ at 50 °C, 2′ at 72 °C; and a final 15′ at 72 °C. Two independent reactions of each library were carried out in order to avoid PCR artifacts on sequences. The PCR products were purified with Wizard SV Gel and PCR Clean-Up System (Promega), and cloned into the p-GEM T Easy Vector (Promega). At least one positive clone of each reaction was then cultured and had its plasmid purified using Wizard Plus SV Minipreps DNA Purification Systems (Promega). Just one single positive clone was isolated from Taximastioncerus sp. The cDNAs were then sequenced using M13 universal and specific primers.Luciferase expression and purification
The open reading frames (ORFs) of cloned cDNAs from P. hirtus and Brasilocerus sp. lateral lanterns were ligated in frame into the pProEx HTa expression vector (Invitrogen)—at Nco I and Not I sites—and transformed into competent cells of E. coli strain BL21 DE3. The new constructs were confirmed by sequencing. The transformants were grown in 300 mL of LB medium under shaking at 37 °C up to OD600 = 0.3, and then induced at 20 °C with 0.5 mM IPTG overnight. Cells were harvested by centrifugation at 2500 g for 15 min and resuspended in urea buffer A (8 M urea, 0.1 M sodium phosphate, 10 mM Tris, 20 mM imidazole, EDTA free protease inhibitor cocktail (Roche Applied Science), pH 7.5) or phosphate buffer A (0.1 mM phosphate, 250 mM NaCl, protease inhibitor, pH 7.5), freeze–thawed three times in dry ice and centrifuged at 15
000 g for 15 min at 4 °C. The supernatant was mixed with Ni-NTA resin (Qiagen) and incubated at 4 °C for 45 min with gentle shaking. The protein bound to the resin was packed in a column and washed with 10 column volumes of buffer A supplemented with 20 mM 2-mercaptoethanol, 5 mM ATP and 10% glycerol. Then, the recombinant luciferases were eluted using two buffers: (A) 1 volume of buffer A at pH 5.9 and (B) 1 volume of the same buffer at pH 4.5. Fractions were collected and analyzed by SDS-PAGE and Western blotting.Western blotting
Denatured samples from heterologous expression were applied to 10% acrylamide SDS gels and subsequently electro-blotted onto nitrocellulose membranes. The membranes were first incubated with polyclonal antibodies raised against Phrixothrix hirtus head luciferase and then with anti-rabbit secondary antibody. The immunodetection was then visualized by chemiluminescence using an ECL Western Blotting kit (GE HealthCare) and a CCD camera system LightCapture I (Atto, Tokyo, Japan), in accordance with the manufacturer's protocol.Luminescence assay
Luminescence activity was measured using a luminometer model TDIII (Tokyo, Japan) and a reaction mixture consisting of 100 μL of 1 mM D-luciferin, 2 mM ATP, 5 mM MgSO4 and 10 μL of purified luciferase sample in 100 mM Tris-HCl buffer pH 8.0.Alignment and general sequence analysis
Multiple sequence alignments were performed using Clustal W 1.823 using default parameters and BLOSUM. General sequence analyses were performed using MPAlign editor 2.24 Protein molecular weight and isoeletric point of luciferases were predicted using Biopython.25Phylogenetic analyses
Phylogenetic analyses were performed with PAUP* 4.0b1026 using parsimony and neighbor-joining27 with the mean character difference as the distance measure. Two phylogenetic approaches were used to test the influence of different criteria on the data set, and, consequently, the reliability of its result. For parsimony analysis, the branch and bound method was used in order to find the best tree. In each analysis, all characters had equal weight.In order to analyze branch support and repeatability, we used bootstrap values performed with PAUP* default parameters and 5000 replications, which are enough to achieve around 1% of accuracy in a 95% confidence interval.28
Results and discussion
Previously, Viviani et al.22 cloned and sequenced the green emitting luciferase from the lateral lanterns of P. vivianii and the red emitting from the head lanterns of P. hirtus. The primary sequences of these luciferases displayed 71% identity. However, the luciferases for both lanterns of any of these species were not cloned because no bioluminescent clone could be isolated by functional screening at that time. Therefore, it was unclear if the luciferase isozymes from different lanterns of the same species were closer to each other, despite different colors, or if the luciferases from the corresponding lanterns from different species were closer to each other.In this work we cloned and sequenced the luciferase cDNAs from the lateral lanterns of three other Mastinocerini railroad-worms species: Brasilocerus sp3. (Genbank FJ545728), Taximastinocerus sp. (GU062703) and Phrixothrix hirtus (FJ545729) (Fig. 2). As expected, these luciferases displayed high identity with those previously cloned from Phrixothrix spp. The luciferase cDNA from Brasilocerus sp3. lateral lanterns has an ORF of 545 residues and displayed the highest similarity with the luciferases from the same lanterns of P. hirtus and P. vivianii (86%). The luciferase from the lateral lanterns of Taximastinocerus sp. displayed an ORF with 547 residues and 81–86% identity with the luciferases from the same lanterns of other Mastinocerini. The luciferase cDNA from P. hirtus lateral lanterns also has 545 residues and displayed the highest similarity (99%) with P. vivianii luciferase from the same lanterns, and just 72% with that from the head lanterns of the own species (Table 1). Therefore, for the first time, luciferases from both lanterns of the same species of Phrixothrix genus are available.
Table 1 Predicted features of Phengodidae luciferases
Species | Lantern position | Residues | Isoelectric point | Molecular weight/kDa | Genbank |
---|
P. hirtus | Head | 546 | 6.94 | 60.95 | AAD34543.1 |
| Lateral | 545 | 6.37 | 59.71 | FJ545729 |
P. vivianii | Lateral | 545 | 6.37 | 59.73 | AAD34542.1 |
Brasilocerus sp3. | Lateral | 545 | 6.52 | 59.95 | FJ545728 |
Taximastinocerus sp. | Lateral | 547 | 7.27 | 60.29 | GU062703 |
R. ohbai | Lateral | 543 | 8.16 | 60.13 | BAF34360.1 |
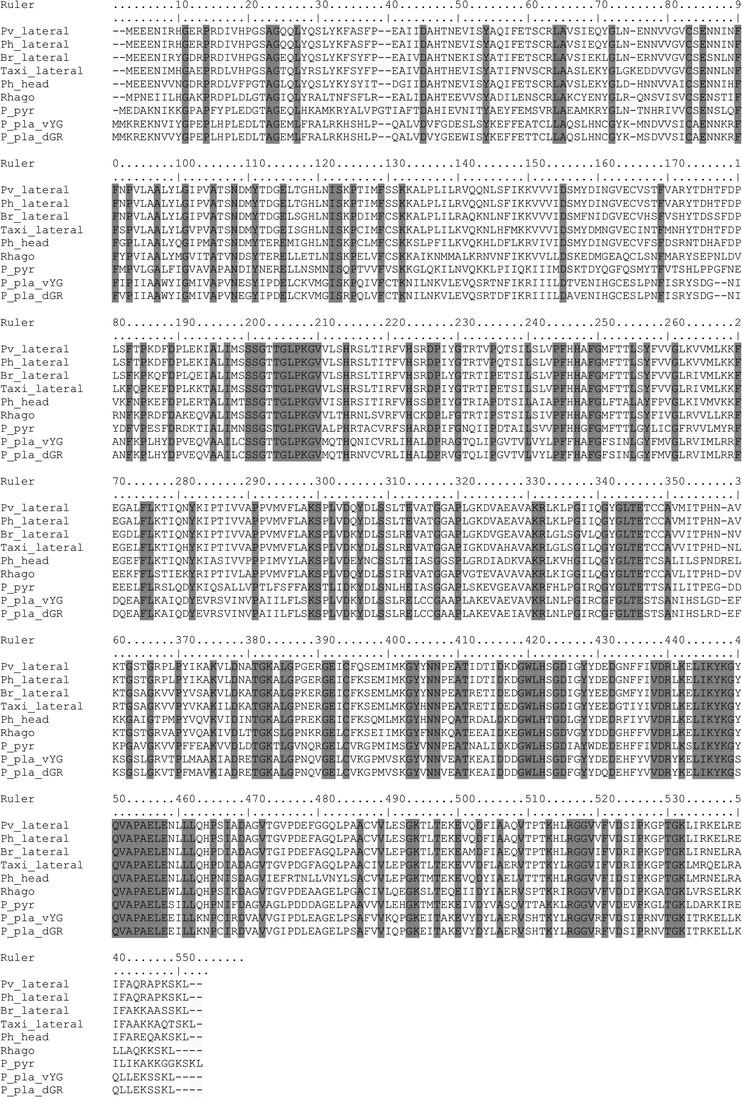 |
| Fig. 2 Alignment of the new railroad-worm luciferases. Pv_lateral: P. vivianii lateral lanterns (AAD34542.1); Ph_lateral: P. hirtus lateral lanterns (FJ545729); Br_lateral: Brasilocerus sp. lateral lanterns (FJ545728); Taxi_lateral: Taximastinocerus sp. lateral lanterns (GU062703); Ph_head: P. hirtus head lanterns (AAD34543.1), Rhago: R. ohbai (BAF34360.1); P_pyr: Photinus pyralis (M15077.1); P_pla_vYG: Pyrophorus plagiophthalmus yellow-green ventral lantern (AAQ11688.1); P_pla_dGR: Pyrophorus plagiophthalmus green dorsal lantern (AAQ11732.1). | |
Unexpectedly, these luciferases were inactive when expressed in bacteria. The lack of activity could be related to the inability of these enzymes to fold properly in bacteria. This possibility is supported by two lines of evidence: (I) no functional bioluminescent clones could ever be isolated from the original cDNA libraries of these lanterns, despite intensive screening, and (II) the inability to purify them under native conditions indeed suggests that these enzymes do not fold properly in this expression system. Although the absence of bioluminescence activity could indicate that these enzymes could not be true luciferases, but luciferase-like enzymes, the high identity observed (above 80% identity) and their phylogenetic analysis leaves little doubt that they are indeed luciferases. Luciferase-like enzymes found in luminescent and non-luminescent beetles have less than 50% identity with true luciferases and, phylogenetically, are not closely related to them.
As expected, the phylogenetic analysis of the luciferase sequences from the three major families of luminescent Coleoptera, including those shown here, is in accordance with the literature, in which the luciferases are grouped in three distinct clades, corresponding to Lampyridae, Phengodidae and Elateridae3–6,16 (Fig. 3). In the resulting phylogeny, the luciferases from the lateral lanterns of different Mastinocerini species (Phrixothrix vivianii, P. hirtus, Taximastinocerus sp. and Brasilocerus sp3.) are clustered together in the same clade, whereas the luciferase from the head lanterns of P. hirtus remains alone in a neighbor branch, indicating that head and lateral lantern luciferases are coded by paralogous genes.
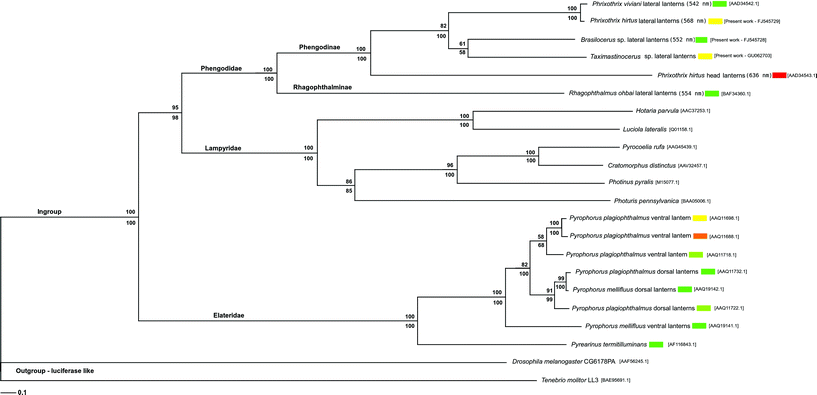 |
| Fig. 3 Phylogenetic analysis of Elateroidea luciferases. The values above and below branches display bootstrap support (5000 pseudo-replications) for parsimony and neighbor-joining respectively. Branch lengths are drawn according to neighbor-joining results. Numbers in brackets are Genbank acession numbers. | |
Considering that the head lanterns are found only Mastinocerini tribe members,15 but not in Rhagophthalminae subfamily and Phengodini tribe, these lanterns and their luciferases may have evolved later, constituting a synapomorphy of Mastinocerini. Since all cloned Mastinocerini luciferases, independently of their lanterns of origin, are closer to each other than to Rhagophthalmus ohbai luciferase, it is likely that the head and lateral lantern luciferases may have diverged after the splitting of Phengodinae and Rhagophthalminae subfamilies, probably after the splitting of Mastinocerini and Phengodini tribes.
Furthermore, based on the fact that only green-yellow bioluminescence is found in the lateral lanterns of members of Phengodinae and that members of Rhagophthalminae are known to just emit light in the green region (Table 1), the most parsimonious hypothesis suggest that the ancestor of Phengodinae had lateral lantern luciferases emitting green light.
Mastinocerini head lantern luciferases are, in general, red-shifted when compared with lateral lanterns (Table 1), suggesting that the ancestor of Mastinocerini had head lanterns with luciferase emitting a red-shifted color. The Phrixothrix genus is the most extreme case, displaying wavelengths longer than 600 nm,15 and its ancestor should represent a further step towards the red color. However, the longest reported wavelength for lateral lanterns is λmax = 586 nm for Mastinomorphus sp2.,15 while the shortest reported value for the head lanterns is λmax = 565 nm for Stenophrixothrix sp2.29 (Table 2). Altogether, it is likely that the most parsimonious model can explain the main steps in the evolution of bioluminescence colors in Phengodinae luciferases, however, minor fluctuations in bioluminescence colors in different taxa of Mastinocerini, probably generated by genetic drift, must also be considered.
Table 2 Maximum wavelengths for the in vivo bioluminescence spectra of different Phengodidae members (sorted by wavelengths)
Species | Lantern location | λmax | Reference |
---|
P. viviani | Lateral | 535 | 29 |
Phengodes laticollis | Larvae | 535 | 29 |
R. ohbai | Larvae (luciferase) | 538 | 18 |
Phrixothrix sp2. | Lateral | 540 | 29 |
Pseudophengodes brasilienses | Adult male | 542 | 15 |
Mastinocerus nigrocollis | Adult male | 549 | 29 |
Brasilocerus impressicollis | Lateral | 550 | 15 |
Stenophrixothrix sp2. | Lateral | 550 | 29 |
Stenophrixothrix sp3. | Lateral | 552 | 29 |
P. viviani | Adult male | 560 | 29 |
P. hirtus | Lateral | 563 | 29 |
Stenophrixothrix sp2. | Head | 565 | 29 |
Phrixothrix heydeni | Larval lateral | 568 | 15 |
Stenophrixothrix sp3. | Head | 570 | 29 |
Stenophrixothrix sp1. | Lateral | 577 | 29 |
Mastinocerus nigrocollis | Head | 578 | 29 |
Mastinomorphus sp1. | Female head | 578 | 15 |
Mastinomorphus sp1. | Adult male | 580 | 15 |
Mastinocerus nigrocollis | Lateral | 580 | 29 |
Mastinomorphus sp1. | Female lateral | 580 | 15 |
Mastinomorphus sp2. | Female lateral | 586 | 15 |
Brasilocerus impressicollis | Head | 595 | 15 |
Mastinomorphus sp2. | Female head | 597 | 15 |
Stenophrixothrix sp1. | Head | 597 | 29 |
Phrixothrix sp4. | Adult male | 600 | 29 |
Phrixothrix sp2. | Head | 600 | 29 |
P. hirtus | Head | 609 | 29 |
P. viviani | Head | 620 | 29 |
Phrixothrix heydeni | Larval head | 636 | 15 |
Although the evolutionary history of bioluminescence colors in Phengodinae is still incomplete, our data suggest a scenario like that in the Fig. 4, with the ancestral luciferase for the lateral lanterns in Phengodinae emitting green light, Mastinocerini head lantern luciferases appearing later, after the divergence of this tribe and Phengodini, followed by a red-shifting in the bioluminescence color, reaching its longest wavelength in Phrixothrix genus.
Concluding remarks
Our analysis suggest that Mastinocerini lateral lantern luciferases are coded by orthologous genes to the Rhagophthalminae and Phengodini luciferases, while those from the head lanterns are paralogous. The head lantern luciferases may have evolved by a gene duplication event departing from a green ancestral luciferase before the Mastinocerini tribe members have diverged. After gene duplication, and before the divergence of Mastinocerini members, the head lanterns luciferase accumulated mutations towards the yellow. After divergence, in different taxa of Mastinocerini, different mutations have changed the color of emitted light, notably in Phrixothrix genus, where a unique natural red bioluminescence in Coleoptera evolved in the head lanterns. The cloning and sequencing of new Mastinocerini railroad-worm luciferases, mainly from the head lanterns, is underway in our laboratory in order to give more details about the evolution of these interesting luciferases.
Acknowledgements
We would like to thank Fundação de Amparo à Pesquisa do Estado de São Paulo (FAPESP, Brazil) and Conselho Nacional de Pesquisa (CNPq, Brazil) for the financial support and Laboratório Nacional de Luz Síncrotron (LNLS, Campinas, Brazil) for providing sequencing facilities.References
- V. R. Viviani, The origin, diversity and structure function relationships of insect luciferases, Cell. Mol. Life Sci., 2002, 59, 1833–1850 CrossRef CAS.
- K. V. Wood, Luc genes: introduction of colour into bioluminescence assays, J. Biolumin. Chemilumin., 1990, 5, 107–114 CrossRef CAS.
- F. G. C. Arnoldi, K. Ogoh, Y. Ohmiya and V. R. Viviani, Mitochondrial genome sequence of the Brazilian luminescent click beetle Pyrophorus divergens (Coleoptera: Elateridae): Mitochondrial genes utility to investigate the evolutionary history of Coleoptera and its bioluminescence, Gene, 2007, 405, 1–9 CrossRef CAS.
- M. Bocakova, L. Bocak, T. Hunt, M. Teraväinen and A. P. Vogler, Molecular phylogenetics of Elateriformia (Coleoptera): evolution of bioluminescence and neoteny, Cladistics, 2007, 23, 477–496 CrossRef.
- R. Sagegami-Oba, N. Takahashi and Y. Oba, The evolutionary process of bioluminescence and aposematism in cantharoid beetles (Coleoptera: Elateridae) inferred by the analysis of 18 S ribosomal DNA, Gene, 2007, 400, 104–113 CrossRef CAS.
- J. C. Day, T. I. Goodall and M. J. Bailey, The evolution of the adenylate-forming protein family in beetles: Multiple luciferase gene paralogues in fireflies and glow-worms, Mol. Phylogenet. Evol., 2009, 50, 93–101 CrossRef CAS.
- H. Fraga, Firefly luminescence: A historical perspective and recent developments, Photochem. Photobiol. Sci., 2008, 7, 146–158 RSC.
- E. Conti, N. P. Franks and P. Brick, Crystal structure of firefly luciferase throws light on a superfamily of adenylate-forming enzymes, Structure, 1996, 4, 287–298 CrossRef CAS.
- T. Nakatsu, S. Ichiyama, J. Hiratake, A. Saldanha, N. Kobashi, K. Sakata and H. Kato, Structural basis for the spectral difference in luciferase bioluminescence, Nature, 2006, 440, 372–376 CrossRef CAS.
- M. DeLuca, Hydrophobic nature of the active site of firefly luciferase, Biochemistry, 1969, 8, 160–166 CrossRef CAS.
- N. N. Ugarova, L. G. Maloshenok, I. V. Uporov and M. I. Koksharov, Bioluminescence spectra of native and mutant firefly luciferases as a function of pH, Biochemistry (Moscow), 2005, 70, 1262–1267 CrossRef CAS.
- B. R. Branchini, T. L. Southworth, M. H. Murtishaw, R. A. Magyar, S. A. Gonzales, M. C. Ruggiero and J. G. Stroh, An alternative mechanism of bioluminescence color determination in firefly luciferase, Biochemistry, 2004, 43, 7255–7262 CrossRef CAS.
- G. Orlova, J. D. Goddard and L. Y. Brovko, Theoretical study of the amazing firefly bioluminescence: the formation and structure of the light emitters, J. Am. Chem. Soc., 2003, 125, 6962–6971 CrossRef CAS.
- T. Hirano, Y. Hasumi, K. Ohtsuka, S. Maki, H. Niwa, M. Yamaji and D. Hashizume, Spectroscopic studies of the light-color modulation mechanism of firefly (beetle) bioluminescence, J. Am. Chem. Soc., 2009, 131, 2385–2396 CrossRef CAS.
- V. R. Viviani and E. J. H. Bechara, Bioluminescence and Biological Aspects of Brazilian Railroad-Worms (Coleoptera: Phengodidae), Ann. Entomol. Soc. Am., 1997, 90, 389–398.
- R. A. Crowson, A review of the classification of Cantharoidea (Coleoptera), with the definition of two new families, Cneoglossidae and Omethidae, Rev. Univ. Madrid, 1972, 21, 35–77 Search PubMed.
- W. Wittmer and N. Ohba, Neue Rhagophthalmidae (Coleoptera) aus China und benachbarten Ländern, Jpn. J. Entomol., 1994, 62, 341–355 Search PubMed.
- Y. Ohmiya, S. Mina, V. R. Viviani and N. Ohba, Comparative aspects of a luciferase molecule from the Japanese luminous beetle Rhagophthalmus ohbai, Sci. Rep. Yokosuka City Mus., 2000, 47, 31–38 Search PubMed.
- W. Wittmer, Arbeiten zu einer Revision der Familie Phengodidae, Entomol. Arb. Mus. G. Frey, 1976, 27, 415–524 Search PubMed.
- D. L. Tiemann, Observations on the natural history of the western banded glowworm Zarhipis integripennis (LeConte) (Coleoptera: Phengodidae), Proc. Calif. Acad. Sci., 1967, 35, 235–264 Search PubMed.
- M. G. Gruber, G. D. Kutuzova, K. V. Wood, Cloning and expression of a Phengodes luciferase, Bioluminescence and Chemiluminescence: Molecular Reporting with Photons, in Proceedings of the 9th International Symposium, 1996, pp. 244–247 Search PubMed.
- V. R. Viviani, E. J. Bechara and Y. Ohmiya, Cloning, sequence analysis, and expression of active Phrixothrix railroad-worms luciferases: relationship between bioluminescence spectra and primary structures, Biochemistry, 1999, 38, 8271–8279 CrossRef CAS.
- J. D. Thompson, D. G. Higgins and T. J. Gibson, CLUSTAL W: improving the sensitivity of progressive multiple sequence alignment through sequence weighting, positions-specific gap penalties and weight matrix choice, Nucleic Acids Res., 1994, 22, 4673–4680 CrossRef CAS.
- F. G. C. Arnoldi, MPAlign editor: uma ferramenta gráfica e intuitiva para alinhamentos moleculares, Anais do II Workshop TIDIA–FAPESP, 2005 Search PubMed.
- P. J. Cock, T. Antao, J. T. Chang, B. A. Chapman, C. J. Cox, A. Dalke, I. Friedberg, T. Hamelryck, F. Kauff, B. Wilczynski and M. J. de Hoon, Biopython: freely available Python tools for computational molecular biology and bioinformatics, Bioinformatics, 2009, 25, 1422–1423 CrossRef CAS.
- D. L. Swofford, PAUP*. Phylogenetic Analysis Using Parsimony (*and Other Methods), Version 4, Sinauer Associates, Sunderland, Massachusetts, 1999 Search PubMed.
- N. Saitou and M. Nei, The neighbor-joining method: a new method for reconstruction of phylogenetic trees, Mol. Biol. Evol., 1987, 4, 406–425 CAS.
- K. F. Müller, The efficiency of different search strategies in estimating parsimony jackknife, bootstrap, and Bremer support, BMC Evol. Biol., 2005, 5, 58 CrossRef.
- V. R. Viviani and E. J. H. Bechara, Biophysical and biochemical aspects of Phengodid (Railroad-worm) bioluminescence, Photochem. Photobiol., 1993, 58, 615–622 CrossRef CAS.
|
This journal is © The Royal Society of Chemistry and Owner Societies 2010 |