DOI:
10.1039/B9NR00323A
(Paper)
Nanoscale, 2010,
2, 542-547
A novel method for preparing quantum dot nanospheres with narrow size distribution
Received
(in Hong Kong, China)
27th October 2009
, Accepted 21st November 2009
First published on
14th January 2010
Abstract
Differently colored quantum dot (QD) nanoparticles are incorporated into bovine serum albumin (BSA) nanospheres by spray-drying followed by thermal denaturization, which is a rapid, highly efficient, large scale, and low cost method. Because the spray-dryer is equipped with an ultrasonic atomizer, most of the nanospheres are no more than 550 nm in diameter and a have narrow size distribution. Ultrathin sections (70 nm) of nanospheres are first prepared using a technique which is normally applied to cell sectioning. The section images show that the QD–BSA nanospheres are solid, and that the QDs are successfully dispersed inside the BSA nanospheres. The nanospheres emit bright fluorescence, and their fluorescence stabilities are not obviously changed compared with that of the QDs. This work provides a novel and simple method for preparing nanoscale spheres encapsulating differently colored QDs. We also present an ultrathin sectioning method for investigating the interior details of nanomaterials.
1. Introduction
In the past few years, fluorescent microspheres containing semiconductor quantum dots (QDs) have attracted significant attention from researchers. These QD microspheres are ideal bioprobes for massively parallel, high-throughput, and high-sensitivity analysis in biomedical research. This is due to the many advantages QDs have over organic fluorescent dyes. For instance,1–3 a single wavelength can be used for simultaneous excitation of differently colored QDs, the fluorescent wavelength of QDs can easily be changed by changing their size and composition, and QDs exhibit narrow and symmetric emission spectra, broad and continuous excitation spectra, high quantum yield, and excellent photostability. One microsphere can accommodate numerous QDs with a variety of colors, so that the microsphere's fluorescent intensity and color can be tailored arbitrarily. Nie and co-workers showed that it is possible in theory to code one million nucleic acid or protein sequences using microspheres (0.5–1 μm) with 10 intensity levels and 6 colors.4 After incorporating them into microspheres, it is possible to reduce the toxicity of QDs by reducing the release of toxic ions such as Cd2+. At the same time, the photostability of QDs is enhanced since the QDs are protected by their microsphere. These QD microspheres have larger surface areas compared to nanometre-sized QDs, and the number of large biomolecules such as antibodies (∼14 nm) conjugated to microspheres is therefore more easily controlled than large biomolecules conjugated to small QDs. We know that if QD bioconjugates are used for labeling cells, the small QD nanoparticles are usually internalized by cells within several hours, and that the internalization of large numbers of QDs will damage the cells. Larger QD microspheres, however, are not easily internalized by cells.
Preparing high-quality QD microspheres is a first important step towards their biomedical application. The main preparation methods are summarized here. A first method involves allowing QDs to penetrate or diffuse into microspheres swelled in solvent.4–6 For example, Nie and co-workers obtained CdSe/ZnS QD polystyrene microspheres by swelling the porous microspheres in a solvent mixture containing 5% (vol/vol) chloroform and 95% (vol/vol) propanol or butanol, and by adding a controlled amount of QDs to the microsphere suspension.4 Another method involves incorporating QDs into polymer microspheres through polymerization,7–14 or into silica microspheres through tetraethyl orthosilicate (TEOS) hydrolyzation.15,16 A third method involves attaching QDs onto the microsphere surface using a layer-by-layer self-assembly method.17–19 Finally, a fourth method involves incorporating QDs into bovine serum albumin (BSA) microspheres using a spray-drying and thermal-denaturizing method, which was presented by our research group for the first time.20 For the first method, the preparation procedures are complex because the empty microspheres should be prepared first. In addition, the number of QDs wrapped in microspheres can not be accurately controlled if not all of the QDs are absorbed by the polymer spheres. For the second and third methods, the procedures are also complex, and the formation of QD–polymer (or SiO2) spheres is a chemosynthetic process, and the fluorescence wavelength and intensity of the QDs are usually changed after the chemosynthesis. In contrast to the first three methods, the formation of the microspheres and incorporation of QDs into microspheres using the fourth method is carried out synchronously in a very short time (several seconds or fewer). This is a simple physical method. Because the precursor for spray-drying is an aqueous solution, the preparation procedure is safe and no organic solvent remains in the products. Furthermore, QD–BSA microspheres can be produced rapidly on a large scale in a highly efficient and low-cost manner. All of the QDs mixed with the BSA molecules in aqueous solution can be incorporated into the BSA microspheres, so that the number of the QDs in the BSA microspheres can be accurately controlled. Importantly, QDs are firmly incorporated into the BSA microspheres so that essentially no QDs are released from the microspheres.
However, in our previous work,20 we obtained only large QD–BSA spheres (microscale) with a very broad size distribution using the fourth method described above, because we used a spray-dryer equipped with a rotary atomizer. In general, the diameter of aqueous droplets produced by the ultrasonic atomizer ranges mainly from submicrometre to several micrometres (<5 μm), which is significantly smaller than the droplets produced by the rotary atomizer (five to several hundred micrometres). In order to obtain small (nanoscale) QD spheres with narrow size distribution, a spray-dryer equipped with an ultrasonic atomizer was used in the current work. This is a novel method for preparing nanoscale QD spheres. This method has the same advantages as that described above (the fourth method). More importantly, small spheres with a narrow size distribution (most of them are no more than 600 nm) can be easily obtained. We used this method for preparing QD–BSA fluorescent nanospheres. We found that dry QD–BSA nanosphere powders of quite considerable weight can be produced within 24 h. The nanospheres retain their bright fluorescence, and these colored nanospheres may be used for multiplexed bioassays and high-throughput detection in the future.
2. Experimental section
2.1 Materials
CdCl2·2.5 H2O, Te powder, NaBH4, Na2S, OsO4, resin (Epon 812), uranyl acetate, lead citrate, glutaraldehyde, BSA (Mw = 66
000, purity = 99%) and acetone were purchased from Shanghai Chemical Regent Co. 3-Mercaptopropionic acid (MPA) was purchased from Aldrich Chemical Co. Fresh plasma was obtained from the Shanghai Dongfang Hospital.
2.2 Preparation of CdTe/CdS core/shell QDs
MPA-capped CdTe/CdS QDs with 6 different sizes (and hence six fluorescence colors) were used in this work. Where the CdTe cores were synthesized in aqueous solution (pH 9.0) using CdCl2, fresh NaHTe (NaHTe was prepared by dissolving Te powder into an NaBH4 solution under an N2 atmosphere) and MPA as precursors. For obtaining differently colored CdTe, the solutions were reacted at 95 °C for 1 h to several days. For overcoating with a CdS shell, Na2S solutions were introduced into the CdTe solutions and reacted at 60 °C for 1 h. The molar ratio of Cd to Te to S to MPA in the reaction solution was about 1
:
0.4
:
0.012
:
4.16. All the reactions were performed under an N2 atmosphere. The QDs obtained were then stored at 4 °C.
2.3 Preparation of BSA nanospheres containing QD nanoparticles
The nanospheres were prepared using a spray-dryer equipped with an ultrasonic atomizer (Fig. 1). This spray-dryer was designed by Mr. Qingyong Wu, Yong Zhang, Hongqing Yang, and the first author of this paper, and manufactured by Hongqing Yang and his colleagues. The spray-drying conditions are given in Table 1.
Table 1 The concentrations of the components in the precursor solutions for spray-drying
Components |
Concentration/μmol l−1 |
Precursor 1 (QDs:BSA ≈ 1 : 4 molar ratio) |
Precursor 2 (QDs:BSA ≈ 1 : 15 molar ratio) |
Precursor 3 (QDs:BSA ≈ 1 : 30 molar ratio) |
Precursor 4 (QDs:BSA ≈ 1 : 75 molar ratio) |
Precursor 5 |
QDs |
∼2 |
∼2 |
∼1 |
∼0.4 |
0 |
BSA |
8 |
30 |
30 |
30 |
30 |
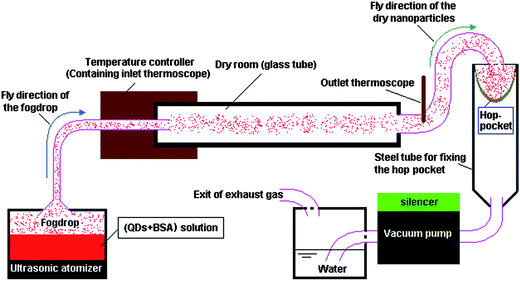 |
| Fig. 1 The schematic illustration of the spray-dryer equipped with an ultrasonic atomizer. | |
For all experiments involving spray-drying, the inlet and outlet temperatures of the spray-dryer were 85 °C and 60 °C, respectively, and the feed flow rate was about 20 ml h−1. After spray-drying, the spray-dryer was continually run for 30 min to further dry the powder accumulated in the collector (hop-pocket). The spray-dried powders were then heated at 160 °C for 3 h using a thermostatic drying oven to denature the BSA. Water-insoluble QD–BSA nanospheres were thus obtained.
2.4 SEM observation and size measurements
The morphologies of the QD–BSA nanospheres were observed using scanning electron microscopy (SEM, JEOL, JSM-6360LV, Japan). The size distributions were measured by SEM for over 200 particles for each sample.
2.5 Preparation of ultrathin sections of the nanospheres and TEM observation
For clearly observing how the nanoparticles were dispersed inside the BSA spheres, ultrathin sections of the QD–BSA nanospheres were prepared and stained using a method that is typically employed for the preparation of cell sections. This procedure involves the following steps. First, 10 mg of QD–BSA nanospheres were dispersed in 60 μl of fresh blood plasma and centrifuged at 5000 rpm for 5 min to remove the excess plasma. 0.5 ml of glutaraldehyde (1%) was then added to the nanosphere–plasma precipitate, and the mixture stored overnight at 4 °C. The second step was cutting the nanosphere–plasma mixture into small pieces (about 1 mm3), followed by post-fixing with 1% OsO4 solution for 1 h and washing three times with PBS (pH 7.3) (30 min for each wash). Third, the fixed pieces were dehydrated in 30, 50, 70, and 90% acetone for 45 min, and dehydrated in acetone three times (30 min each time). For the next step the dehydrated samples were infiltrated for 2 h with a 7
:
3 mixture of resin:acetone, infiltrated overnight with 3
:
7 mixture of resin:acetone; and then dispersed into pure resin for 2 h. The resin was polymerized overnight at 45 °C followed by 48 h at 60 °C. Following this, the ultrathin (70 nm) sections of the embedded specimens were cut with an ultramicrotome (Lecia EM uc6); and the thin sections were placed onto glass slides and stained with uranyl acetate and lead citrate for 30 and 10 min, respectively. The sections were then washed with distilled water three times (5 min for each wash) for imaging with a transmission electron microscope (TEM, JEOL-1230, Japan). As a control, ultrathin sections of pure BSA nanospheres were also prepared and stained using the same method as described above.
2.6 Fluorescence measurements
The true-color florescence images of the QD solutions and the nanosphere powders were recorded using a digital color camera (Coolpix4300, Nikon, Japan) attached to an ultraviolet detector (ZF, Kanghua, Shanghai, China). The room-temperature photoluminescence (PL) spectra of the QD solutions and water-dispersed nanosphere powders were measured using a fluorescence spectrometer (F-2500 from Hitachi, Japan) with a xenon lamp source. The quantum yields (QYs) of the QDs and QD–BSA nanospheres were determined by comparing the integrated emission to that of rhodamine 6G in ethanol, according to the procedure described in previous work.21 To detect the fluorescence stability of the QD nanospheres, the QD nanosphere powders were dispersed in aqueous solution and were continuously irradiated at 365 nm over a period of 1 h using the ultraviolet detector. During the irradiation process, the fluorescence spectra of the samples were measured. As a control, the QD solutions were continuously irradiated and their fluorescence spectra were measured using the same method as described above. All the fluorescence measurements were taken at room temperature.
3. Results and discussion
MPA-capped CdTe/CdS core/shell QDs(528), QDs(552), QDs(570), QDs(595), QDs(609) and QDs(650) (the maximum emission wavelength is given in parentheses) were selected for the experiments reported here. The true-color fluorescence images and emission spectra of these QDs are shown in Fig. 2. After being incorporated into BSA, the QD–BSA composites are spherical in shape and most of the resulting spheres are no more than 550 nm in diameter (Fig. 3A). When the precursor for spray-drying contains 2 μmol l−1 of QDs and low concentrations of BSA (8 μmol l−1), the average diameter of the resulting nanospheres is 350.59 ± 81.78 nm. Increasing the concentration of BSA in the precursor increases the diameter of the resulting dry nanospheres. For example, the average diameter of nanospheres prepared from the precursor for spray-drying containing 2 μmol l−1 of QDs and 30 μmol l−1 of BSA is 395.68 ± 116.93 nm. Because the number of QDs is less than that of BSA in the precursor, and the QD diameter is very small (<5 nm), the size distribution of the QD–BSA nanospheres is essentially controlled by the amount of BSA (Fig. 3B). It is known that the diameter of the droplets produced by the ultrasonic atomizer is very small (from sub-micrometre to several micrometres). After the water in the spherical droplet evaporates, the solute in the droplet will separate out and aggregate into spherical solid particles whose diameter is much smaller than the original droplet. The lower the concentration of the precursor, the smaller the solid particles obtained. Therefore, the smaller nanospheres are obtained with a low BSA-concentration solution. However, most of the nanospheres prepared from the low-BSA-concentration solution were shrunken or hollow in shape (Fig. 3Aa), which may be due to the low volume fraction of the solute in the droplets prior to drying. However, the viscosity of the precursor for spray-drying increases with increasing concentration of BSA, more BSA in the precursor leads to more difficult ultrasonic atomization. In this work, the concentration of BSA in the precursor is no more than 30 μmol l−1.
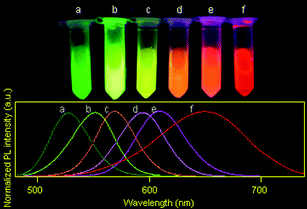 |
| Fig. 2 The true-color fluorescence images (excitation wavelength: 365 nm) and emission spectra (excitation wavelength: 400 nm) of (a) QDs(528), (b) QDs(552), (c) QDs(570), (d) QDs(595), (e) QDs(609) and (f) QDs(650) aqueous solutions prepared and used in this work. | |
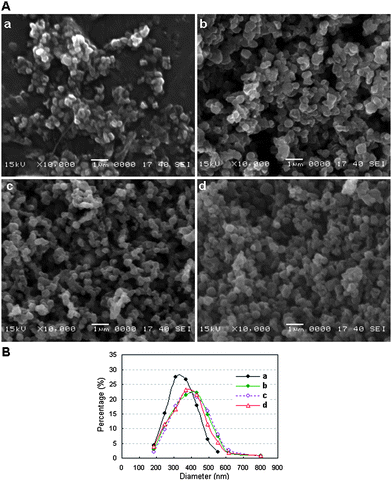 |
| Fig. 3 A: SEM images and B: size distributions of the QD–BSA nanospheres prepared with the following precursors: (a) 2 μmol l−1 QDs(650) and 8 μmol l−1 BSA; (b) 2 μmol l−1 QDs(650) and 30 μmol l−1 BSA; (c) 1 μmol l−1 QDs(650) and 30 μmol l−1 BSA and (d) 0.4 μmol l−1 QDs(650) and 30 μmol l−1 BSA. | |
In order to clearly observe the state of the inorganic nanoparticles dispersed inside the BSA nanospheres, QD–BSA nanospheres were sliced using an ultrathin sectioning technique that is often applied in cell biology. Typical TEM images of QD–BSA nanosphere ultrathin sections are shown in Fig. 4. When the precursor for spray-drying contains 1 μmol l−1 QDs and 30 μmol l−1 BSA, each resulting nanosphere has large numbers of nanometre-sized dark dots (Fig. 4a and 4a′). These dark dots are considered to be the QDs since the spheres prepared with only BSA have no such dark dots (Fig. 4b and 4b′). This result indicates that the QDs are successfully encapsulated by the BSA nanospheres, and the QD encapsulation process may be carried out simultaneously with the formation of QD–BSA nanospheres using the spray drying technique. In addition, no obvious large holes are observed inside most of the nanospheres, which is in contrast to the hollow fluorescent microspheres reported in our previous work [20]. It should be noted that because the nanosphere sections are at random radial positions, the size distribution of the nanosphere cross sections is very broad, and most of the nanosphere section diameters are smaller than those of the spheres observed by SEM (see Fig. 3).
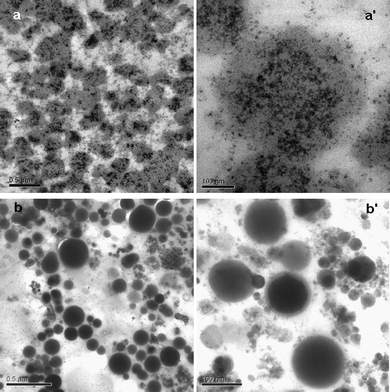 |
| Fig. 4 TEM images of ultrathin (70 nm) sections of the nanospheres. (a, a′): QD–BSA nanospheres prepared with 1 μmol l−1 QDs(650) and 30 μmol l−1 BSA; (b, b′): pure BSA nanospheres prepared with 30 μmol l−1 BSA. (a, b): low-magnification (bar = 0.5 μm); (a′ b′): high-magnification (bar = 100 nm). | |
The emission spectra and fluorescence color of the QD–BSA nanosphere powders are shown in Fig. 5. Compared with the fluorescence emission of the original QDs(650), the emission peak of the QD–BSA nanospheres is blue-shifted by 8 to 15 nm. The more BSA used, the larger the blue-shift of the emission peak (Fig. 5A). The true-color fluorescence of the QD–BSA nanospheres differs from that of the original QDs(650) when the precursor solution contains a low ratio of QD:BSA (1
:
75, 0.4 μmol l−1 QDs and 30 μmol l−1 BSA), as can be seen in Fig. 5B. This may be due to the larger blue-shift of the emission spectra and to the pure BSA emitting blue autofluorescence (Fig. 5C). The fluorescence color of the nanospheres can be changed easily by changing the size of the QDs. As shown in Fig. 5D, the dry powders emit bright green, green-yellow, yellow, orange, and red fluorescence when the QD–BSA nanospheres are prepared with QDs(528), QDs(552), QDs(570), QDs(595), QDs(609) and QDs(650), respectively (all precursors contained 1 μmol l−1 QDs and 30 μmol l−1 BSA), and their fluorescence spectra are also slightly shift to blue compared with those of the corresponding original QDs (Fig. 5E). After being incorporated into BSA, the QYs of all QD–BSA nanospheres obtained decreased compared with those of QDs. For example, the QY of QDs(650) solution is about 24.00%, whereas the QY of QDs(650)–BSA nanosphere suspensions prepared from the precursors containing 2 μmol l−1 QDs and 30 μmol l−1 BSA and prepared from the precursors containing 0.4 μmol l−1 QDs and 30 μmol l−1 BSA are about 5.03% and 3.60%, respectively. The reduced QY may be caused by the excess BSA coating and the thermal denaturization treatment.
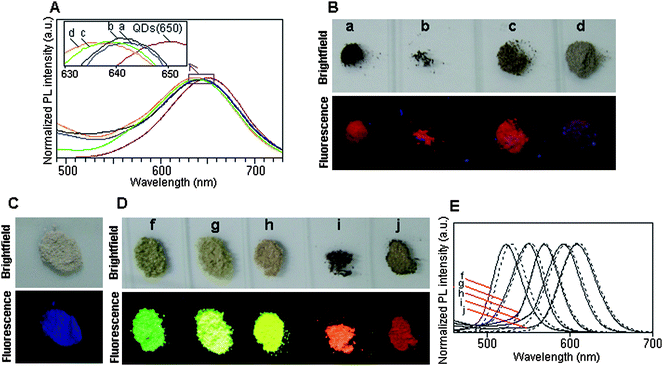 |
| Fig. 5 (A) Emission spectra (excitation wavelength: 400 nm) and (B) bright-field and true-color fluorescence images (excitation wavelength: 365 nm) of QDs(650)–BSA nanospheres prepared with different ratio of QD:BSA: (a) 1 : 4 (2 μmol l−1 QDs, 8 μmol l−1 BSA); (b) 1 : 15 (2 μmol l−1 QDs, 30 μmol l−1 BSA); (c) 1 : 30 (1 μmol l−1 QDs, 30 μmol l−1 BSA) and (d) 1 : 75 (0.4 μmol l−1 QDs, 30 μmol l−1 BSA). (C) Bright-field and true-color fluorescence images of pure BSA nanospheres. (D) Bright-field and true-color fluorescence images of the QD–BSA nanospheres prepared with differently colored QDs: (f) QDs(528), (g) QDs(552), (h) QDs(570), (i) QDs(595) and (j) QDs(609). (E) Emission spectra (excitation wavelength: 400 nm) of QD–BSA nanospheres prepared with differently colored QDs: (f) QDs(528), (g) QDs(552), (h) QDs(570), (i) QDs(595) and (j) QDs(609). (The dotted lines are the emission spectra of QDs(528), QDs(552), QDs(570), QDs(595) and QDs(609), respectively). | |
The typical fluorescence stabilities of the QD–BSA nanospheres are shown in Fig. 6. When the QDs(650)–BSA nanospheres contain a high ratio of QD:BSA (1
:
4, 2 μmol l−1 QDs and 8 μmol l−1 BSA), the fluorescence emission decreases with prolonging the irradiation time, but decreases by only 4.06% after being continuously irradiated (365 nm) for 1 h. When the QDs(650)–BSA nanospheres contain lower ratios of QDs to BSA, the fluorescence emission increases for the initial 15 min (or 30 min) and then decreases during any subsequent irradiation time, which is similar to that of the QD solution. During the 1 h irradiation, although the fluorescence emission of the nanospheres containing lower ratios of QDs to BSA are always higher than those of the nanospheres without irradiation, the maximal increment of the fluorescence emission is just 12.80%. This result indicates that the stability of the QD–BSA nanospheres has been not obviously changed compared with that of the QDs, and the fluorescence of the QD–BSA nanospheres could be used for long-term fluorescence observation in biomedical research areas.
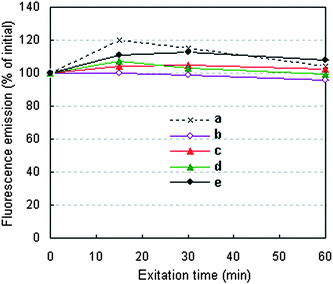 |
| Fig. 6 Fluorescence stability of the (a) QDs(650) and (b)–(e) QDs(650)–BSA nanospheres varied with excitation time. QDs(650)–BSA nanospheres prepared with different ratios of QDs to BSA: (b) 1 : 4 (2 μmol l−1 QDs, 8 μmol l−1 BSA); (c) 1 : 15 (2 μmol l−1 QDs, 30 μmol l−1 BSA); (d) 1 : 30 (1 μmol l−1 QDs, 30 μmol l−1 BSA) and (e) 1 : 75 (0.4 μmol l−1 QDs, 30 μmol l−1 BSA). | |
4. Conclusions
In summary, QD fluorescent nanospheres with narrow size distributions have been prepared using a highly efficient spray-drying and thermal-denaturizing method, in which the spray-dryer is equipped with an ultrasonic atomizer. TEM images of ultrathin nanosphere sections show that each sphere contains a large number of nanoparticles. The fluorescence of the BSA nanospheres can be controlled by changing the QD size, so that nanospheres exhibiting different fluorescence colors are easily obtained. Any other combined nanomaterial such as various-sized QDs may be synchronously incorporated into a single BSA nanosphere so that multi-fluorescent nanospheres may be obtained for biomedical applications in the future. This work opens the possibility of developing mass-produced QD multi-colored nanospheres with narrow size distributions for biomedical applications.
Acknowledgements
This work was supported in part by the 863 National High Technology Research and Development Program of China (No. 2007AA03Z319), the National Natural Science Foundation of China (No. 30870711), the Program for New Century Excellent Talents in University (No. NCET-07-0618) and the Nanoscience Foundation of Shanghai (No. 0652nm007)
References
- A. P. Alivisatos, Science, 1996, 271, 933 CrossRef CAS.
- P. Mitchell, Nat. Biotechnol., 2001, 19, 1013 CrossRef CAS.
- W. C. W. Chan, D. J. Maxwell, X. Gao, R. E. Bailey, M. Y. Han and S. Nie, Curr. Opin. Biotechnol., 2002, 13, 40 CrossRef CAS.
- M. Han, X. Gao, J. Su and S. Nie, Nat. Biotechnol., 2001, 19, 631 CrossRef CAS.
- V. Stsiapura, A. Sukhanova, M. Artemyev, M. Pluot, J. H. M. Cohen, A. V. Baranov, V. Oleinikov and I. Nabiev, Anal. Biochem., 2004, 334, 257 CrossRef CAS.
- X. Gao and S. Nie, Anal. Chem., 2004, 76, 2406 CrossRef CAS.
- S. V. Vaidya, M. L. Gilchrist, C. Maldarelli and A. Couzis, Anal. Chem., 2007, 79, 8520 CrossRef CAS.
- W. Sheng, S. Kim, J. Lee, S.-W. Kim, K. Jensen and M. G. Bawendi, Langmuir, 2006, 22, 3782 CrossRef CAS.
- N. Joumaa, M. Lansalot, A. Theretz, A. Elaissari, A. Sukhanova, M. Artemyev, I. Nabiev and J. H. M. Cohen, Langmuir, 2006, 22, 1810 CrossRef CAS.
- Y. Yang, Z. Wen, Y. Dong and M. Gao, Small, 2006, 2, 898 CrossRef CAS.
- R. L. Sherman and W. T. Ford, Langmuir, 2005, 21, 5218 CrossRef CAS.
- Y. Li, E. C. Y. Liu, N. Pickett, P. J. Skabara, S. S. Cummins, S. Ryley, A. J. Sutherland and P. O’Brien, J. Mater. Chem., 2005, 15, 1238 RSC.
- F. Fleischhake and R. Zentel, Chem. Mater., 2005, 17, 1346 CrossRef CAS.
- P. O’Brien, S. S. Cummins, D. Darcy, A. Dearden, O. Masala, N. L. Pickett, S. Ryley and A. J. Sutherland, Chem. Commun., 2003, 2532 RSC.
- Y. Yang and M. Gao, Adv. Mater., 2005, 17, 2354 CrossRef CAS.
- M. Chu, Y. Sun and S. Xu, J. Nanopart. Res., 2008, 10, 613 CrossRef CAS.
- A. Sukhanova, A. S. Susha, A. Bek, S. Mayilo, A. L. Rogach, J. Feldmann, V. Oleinikov, B. Reveil, B. Donvito, J. H. M. Cohen and I. Nabiev, Nano Lett., 2007, 7, 2322 CrossRef CAS.
- D. Wang, A. L. Rogach and F. Caruso, Nano Lett., 2002, 2, 857 CrossRef CAS.
- Q. Ma, X. Wang, Y. Li, Y. Shi and X. Su, Talanta, 2007, 72, 1446 CrossRef CAS.
- M. Chu, L. Zhou, X. Song, M. Pan, L. Zhang, Y. Sun, J. Zhu and Z. Ding, Nanotechnology, 2006, 17, 1791 CrossRef CAS.
- M. Chu, X. Shen and G. Liu, Nanotechnology, 2006, 17, 444 CrossRef CAS.
|
This journal is © The Royal Society of Chemistry 2010 |
Click here to see how this site uses Cookies. View our privacy policy here.