DOI:
10.1039/B923816F
(Highlight)
Lab Chip, 2010,
10, 11-12
Research Highlights
First published on 18th November 2009
How pathogen bacteria colonise the intestine
Epithelial cells in the gastrointestinal tract of humans co-exist with non-pathogenic, so called commensal bacterial cells. Indeed, the interactions between the bacteria and the intestine host cells are important for normal intestine functioning. Infections by pathogenic bacterial cells can severely disturb the balance between host cells and commensal bacteria. Interestingly, few pathogen bacterial cells are often sufficient to out-compete huge numbers of non-pathogen bacterial cells, and manage to pass the commensal layer and infect the intestine epithelial cells. From former investigations on intestine infections it became evident that some signalling molecules produced by the commensal bacteria in high concentration play a significant role on pathogen infection. Arul Jayaraman and co-workers from Texas A&M University (College Station, TX, USA) could now investigate in more detail the interactions of host cells and pathogen bacteria and influences by signalling molecules by means of a microfluidic device.1 The device is designed to form localised cultures of epithelial cells (HeLa cells) and commensal bacteria. This is achieved by integration of a pneumatically controlled trap for the bacterial cells into a microchannel that contains the epithelial cells. After introduction of the cells in their respective compartment, epithelial cells reached confluence after 48 h, while the bacterial cells formed a biofilm during the same time. Subsequent introduction of pathogen bacterial cells (enterohemorrhagic E.coli, EHEC) into the bacteria cell trap resulted in uniform distribution of the pathogen cells within the commensal cell population. Next, 6 hours after introduction of EHEC cells, the cell trap is opened to allow the migration of pathogen cells into the epithelial cell layer, and the infection of the epithelial cells around the cell traps is determined using a live/dead cell assay. This system can be used to study influences of signalling molecules on infection. For this the authors repeated the experiment using a mutant of the commensal bacterial cells that do not produce the signalling molecule indole. After introduction of pathogen cells and opening the trap, it turns out that the number of dead epithelial cells significantly increased, i.e. the infectivity of the pathogen cells doubled. Pre-treatment of the epithelial cells with indole could only partially decrease the infectivity. In other words, high local concentration of the signalling molecules seems to support more efficient infection of epithelial cells than equal distribution of the signalling molecules. The method described in the paper could be extended to investigate the role of other signalling molecules. It might be useful as well for screening probiotic cell strains for modulating pathogen infectivity on the gastrointestinal tract.
Separation and detection of enantiomers
The separation and detection of tert-butoxycarbonyl-tryptophan (Boc-Trp) enantiomers (Boc-L-Trp and Boc-D-Trp, respectively) are of great interest in agriculture, food and pharmaceutical industries since chiral molecules have different physiological activity. Conventional techniques to separate the enantiomers are based on HPLC, but these techniques are time and sample consuming, and the columns have to be packed with Boc-L-Trp-imprinted particles. Researchers from Nanjing University (China) have recently developed a microchip approach, which uses the molecular imprinting technique to form in situ the imprinted polymer on the microchannel wall.2 The molecular imprinted polymer was polymerized directly on the surface of a microchannel made of poly(dimethylsiloxane) (PDMS) (Fig. 1). In this reaction, acrylamide was used as a functional monomer and ethylene glycol dimethacrylate (EDMA) as a cross-linker. The process was optimized, and the obtained molecular imprinted polymer was carefully analysed. The porous polymer film supports highly efficient interactions with the analytes. Indeed, the separation of Boc-D-Trp and Boc-L-Trp was demonstrated within 75 s instead of several (8–40) minutes required in former methods. Amperometric detection was accomplished by using carbon fibre microdisk electrodes, and the detection limit was in the range of micromolar concentration. The good reproducibility and stability of the separation underlines the potential of the device for high-throughput analysis of chiral compounds.
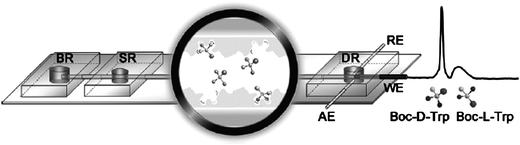 |
| Fig. 1 Separation of enantiomers by means of in situ molecular imprinting of a polymer on a microchannel wall. The performance of the device was demonstrated for enantioseparation and amperometric detection of tert-butoxycarbonyl-D-tryptophan (Boc-D-Trp) and Boc-L-Trp. (Reprinted from ref. 2 with permission. Copyright 2009 American Chemical Society). | |
Raman microspectroscopy is a label-free detection technique particularly useful for spatially resolved quantification of the concentration of compounds in a mixture. An advanced technique is coherent anti-Stokes Raman scattering (CARS) microscopy, which has much faster acquisition rates than conventional Raman microspectroscopy, and can be employed in combination with microfluidic platforms. However, one of the drawbacks of this technique is the inherent non-specific background signal originating from non-resonant excitation of the sample. An elegant way to account for the background signal has been recently presented by a research team from the Universities of Würzburg and Jena (both Germany) (Fig. 2).3 They used a microfluidic chip with two parallel channels, one of which contains a mixture of the perdeuterated sample (perdeuterated toluene, C7D8) and a reference compound (toluene), the other channel contains the reference compound only. Excitation of the sample at the resonant wavelength of the perdeuterated toluene (aromatic C–D stretching at around 2200 cm−1) results in emission of a resonant signal plus background. At the same time, non-resonant background signal is obtained in the other channel. Comparison of the signal intensities allows quantification of the mole fraction of perdeuterated toluene with a detection limit of about 470 nM. The authors expect to lower the detection limit in future work and hence, to be able to detect molecules in the micro- to nanomolar range, i.e. molecules in physiologically relevant concentrations.
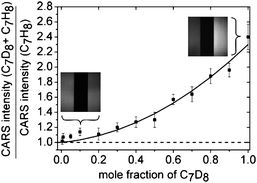 |
| Fig. 2 Label-free, quantitative CARS microspectroscopy. In this detection technique the signal originating from perdeuterated toluene is compared to a reference signal (toluene) that is measured simultaneously to account for the non-specific background signal. The simultaneous detection is achieved by supplying the sample and the reference compounds through two parallel microchannels. (Reprinted with permission from ref. 3. Copyright 2009, Wiley-VCH). | |
Petra S. Dittrich
ETH Zürich, Switzerland
dittrich@org.chem.ethz.ch
References
- J. Kim, M. Hegde and A. Jayaraman, Co-culture of epithelial cells and bacteria for investigating host-pathogen interactions, Lab Chip, 2009 10.1039/b911367c.
- P. Qu, J. Lei, R. Ouyang and H. Ju, Enantioseparation and amperometric detection of chiral compounds by in situ molecular imprinting on the microchannel wall, Anal. Chem., 2009 DOI:10.1021/ac902201a.
- G. Bergner, S. Chatzipapadopoulos, D. Akimow, B. Dietzek, D. Malsch, T. Henkel, S. Schlücker and J. Popp, Quantitative CARS microscopy detection of analytes and their isotopomers in a two-channel microfluidic chip, Small, 2009 DOI:10.1002/smll.200900807.
|
This journal is © The Royal Society of Chemistry 2010 |
Click here to see how this site uses Cookies. View our privacy policy here.