DOI:
10.1039/C0FO00077A
(Paper)
Food Funct., 2010,
1, 124-129
Dealcoholized red wine reverse vascular remodeling in an experimental model of metabolic syndrome: role of NAD(P)H oxidase and eNOS activity
Received
15th July 2010
, Accepted 13th August 2010
First published on
22nd September 2010
Abstract
The present study examines the effect of chronic administration of dealcoholized red wine Malbec (DRW) on vascular remodeling and NAD(P)H oxidase and endothelial nitric oxide synthase activity (eNOS) in an experimental model of metabolic syndrome induced by fructose administration. Thirty-day old male Wistar rats were fed a normal rat diet (control) or the same diet plus 10% fructose in drinking water (FFR). During the last 4 weeks of a 10-week period of the corresponding diet, a subgroup of control and FFR (n = 8 each) received DRW in their drinking water. Systolic blood pressure (SBP), a homeostasis model assessment of insulin resistance (HOMA-IR), aortic NAD(P)H oxidase and eNOS activity in the heart and vascular tissue were evaluated. Vascular remodeling was evaluated in the left carotid artery (CA) and interlobar, arcuate and interlobular renal arteries (RA) through lumen to media (L/M) ratio determination. At the end of the study FFR increased the SBP (p < 0.001), HOMA-IR (p < 0.001), and aortic NAD(P)H oxidase activity (p < 0,05) but reduced cardiac and vascular eNOS activity (p < 0.01), L/M ratio in CA (p < 0.001) and RA (p < 0.01) compared with the C group. DRW reduced SBP (p < 0.05), aortic NAD(P)H oxidase (p < 0.05), and recovered eNOS activity (p < 0.001) and L/M in CA (p < 0.001) and RA (p < 0.001) compared with FFR. This study provides new data about the beneficial effect of DRW on oxidative stress and vascular remodeling in the experimental model of metabolic syndrome. Data suggest the participation of mechanisms involving oxidative stress in FFR alterations and the usefulness of natural antioxidant substances present in red wine in the reversion of these changes.
Introduction
Metabolic syndrome (MS), characterized by insulin resistance, dyslipidemia and hypertension, is an important risk factor for cardiovascular diseases.1 Rats chronically receiving fructose (FFR) provide a useful experimental model for the study of the interaction between factors clustered in MS.2 Endothelial dysfunction is associated with this experimental model.3 We previously reported a decrease of the endothelial isoform of nitric oxide synthase activity (eNOS) at cardiovascular level and an increase in vascular smooth muscle cell proliferation in primary culture, showing also evidence involving the renin angiotensin system (RAS) in the pathophysiology of these injuries.4,5 Furthermore, it has been demonstrated that angiotensin, acting on AT1 receptors, could induce oxidative stress, through activation of nicotinamide adenine dinucleotide phosphate (NAD(P)H) oxidase, the most important source of intracellular reactive oxygen species (ROS) in vascular cells.6
ROS play a physiological role in the vessel wall and participate as second messengers in endothelium dependent function, in smooth muscle cell and endothelial cell growth and survival, and in remodeling of the vessel wall.7,8 The major vascular ROS is superoxide anion (·O2−), which inactivates nitric oxide (NO˙), the main vascular relaxing factor.9 The relationship between oxidative stress and vascular remodeling had been previously reported in human and animal experimental studies,7 including fructose-fed rats.10,11
The study of the beneficial effect on human health of consumption of natural antioxidants, present in vegetables, fruits and beverages such as red wine, has recently increased in significance. Epidemiological studies suggest that moderate red wine consumption could decrease the risk of cardiovascular mortality,12 mainly attributable to the polyphenol content but also attributable to the alcohol content.13 Polyphenols could favor endothelium-dependent vasodilatation in aorta and human coronary arteries, inhibit vascular smooth muscle cell proliferation.14–16 We previously reported that resveratrol was able to increase the eNOS activity and reduce the systolic blood pressure (SBP) in this model of MS.17 In order to establish the beneficial effects of non-alcoholic constituents of red wine on vascular remodeling, the aim of this study was to determine the effect of chronic administration of dealcoholized red wine (DRW) in fructose-fed rats upon the possible participation of changes in ROS and NO˙ generation in the development of structural and functional alterations at cardiovascular and metabolic levels. Specifically, ROS production by the NAD(P)H oxidase system, and NO˙ generation by eNOS were examined in order to establish whether these systems are involved as pathogenic mechanisms in metabolic and structural cardiovascular changes associated with this experimental model.
Results
No differences were observed in food and drink intake between groups throughout the experimental period. Table 1 shows body weight, metabolic variables and SBP. The body weight did not vary among groups. At the end of the study fructose-fed rats developed insulin resistance, increased significantly the triglyceride levels and reduced the HDL cholesterol compared with control groups. Chronic administration of DRW significantly reduced the insulin resistance state and increased the HDL cholesterol. Systolic blood pressure was gradually increasing throughout the experimental period in FFR and reached a significant difference compared to controls. DRW administration to FFR during the last four weeks was able to reduce SBP in a slight but significantly way, without effect on control rats.
Table 1 Body weight, SBP, and metabolic parameters from C, C + DRW, FFR, and F + DRW rats.
|
Groups |
C |
C + DRW |
FFR |
FFR + DRW |
Values are expressed as mean ± SEM, n = 8; means without a common letter differ, P < 0.05. |
Body weight/g |
340 ± 7 |
324 ± 9 |
349 ± 7 |
325 ± 7 |
Plasma glycemia/mmol L−1 |
4.0 ± 0.2b |
4.0 ± 0.2b |
6.3 ± 0.4a |
5.6 ± 0.2a |
Plasma insulin (pmol/L) |
72 ± 6c |
75 ± 8c |
152 ± 7a |
118 ± 6b |
HOMA-IR |
1.8 ± 0.4c |
1.9 ± 0.5c |
6.1 ± 0.8a |
4.2 ± 0.6b |
Plasma triglycerides/mmol L−1 |
0.81 ± 0.02b |
0.72 ± 0.07b |
1.23 ± 0.08a |
1.08 ± 0.08a |
Plasma HDL/mmol L−1 |
0.92 ± 0.04a |
0.95 ± 0.04a |
0.80 ± 0.02b |
0.92 ± 0.01a |
Plasma total cholesterol/mmol L−1 |
1.48 ± 0.04 |
1.40 ± 0.06 |
1.46 ± 0.08 |
1.41 ± 0.08 |
SBP (mmHg)1 |
Baseline |
100 ± 1 |
102 ± 1 |
103 ± 1 |
100 ± 1 |
Week 6 |
106 ± 1b |
107 ± 1b |
130 ± 1a |
129 ± 1a |
Week 10 |
115 ± 1c |
116 ± 1c |
136 ± 1a |
125 ± 1b |
The NAD(P)H oxidase activity in aortic tissue was higher in FFR, compared with the C group. Administration of DRW significantly reduced the NAD(P)H oxidase activity (Fig. 1).
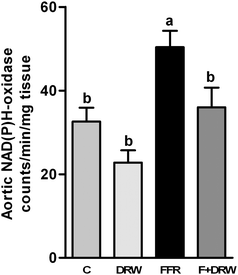 |
| Fig. 1 NAD(P)H oxidase aorta activity from C, C + DRW, FFR, and F + DRW rats. Values are mean ± SEM (n = 8). Bars without a common letter differ, P < 0.05. | |
Fig. 2 shows eNOS activity levels, measured in a mesenteric vascular bed (panel A) and heart tissue from left ventricle homogenates (panel B). The eNOS activity was significantly diminished in the FFR group, compared to control rats. DRW chronic administration to FFR was able to return NO˙ production to control levels in both mesenteric vascular and heart tissue, while DRW to control rats increased significantly the eNOS activity in mesenteric vascular tissue.
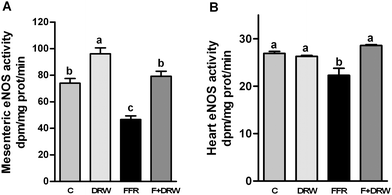 |
| Fig. 2 eNOS activity in mesenteric vascular bed homogenates (A) and heart tissue homogenates from the left ventricle (B), from C, C + DRW, FFR, and F + DRW rats. Values are mean ± SEM (n = 4). Bars without a common letter differ, P < 0.05. | |
Arterial wall modifications were detected by structural analysis performed by histological methods, which allow us to observe changes in arteries from different localizations and calibers. Fig. 3 shows lumen : media (L/M) ratios and representative microphotographs observed in arteries from different localizations: left carotid (A), renal interlobar (B), renal arcuate (C), and renal interlobular (D) arteries in each group. The carotid lumen to media ratio in the FFR group was significantly reduced, compared to control rats. Chronic administration of DRW to FFR increased the L/M ratio to control levels. A similar structural pattern was found in interlobar renal arteries (caliber between 120 to 180 μm), in smaller caliber arteries (50 a 120 μm) such as arcuate renal arteries and in very small arteries (10 a 50 μm) such as interlobular renal arteries.
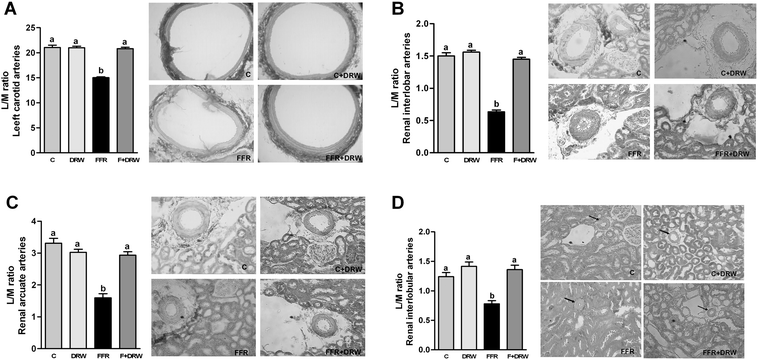 |
| Fig. 3 Lumen to media ratio observed in arteries from different localizations: left carotid (A), renal interlobar (B), renal arcuate (C), renal interlobular (D) arteries described by representative microphotographs and analyzed in the bar graph from C, C + DRW, FFR, and F + DRW rats. Values are mean ± SEM (n = 8). Bars without a common letter differ, P < 0.05. Arrows indicate the location of the arteries. | |
Discussion
In the present study we demonstrate that DRW was able to reverse vascular remodeling in fructose-fed rats, an experimental model of MS, associated with an increased eNOS activity and a reduced aortic NAD(P)H oxidase activity. These results suggest that non-alcoholic constituents of red wine reverse the structural and functional changes by mechanisms related to oxidative stress enhanced in this model.
We have previously demonstrated the development of endothelial dysfunction in this experimental model, supported by a diminished NO˙ generation capability and changes in vascular smooth muscle cell proliferative behavior in primary culture.4 These changes could be attributed to a significant increase in ROS production, evaluated through an increased activity of NAD(P)H oxidase, the most quantitatively important source of superoxide at vascular level.10,11,18 ROS produced by vascular wall cells can directly inactivate other biologically active free radicals, thereby disturbing vascular homeostasis. One of the main targets of ROS, particularly superoxide anion is NO˙, decreasing its bioavailability and favoring the formation of peroxynitrite, a potent vasoconstrictor.19 In this study, we found a decreased eNOS activity in both heart and mesenteric vascular tissue in FFR. DRW administered to FFR was able to restore the activity of this enzyme, suggesting that red wine polyphenols could be responsible for these beneficial effects. The effects of some polyphenols in these variables had been achieved. Quercetin increased the activity of eNOS and downregulates the activity and expression of NAD(P)H oxidase in an experimental model of hypertension.20 Others studies have shown that metabolites of flavonoids inhibit the activity of NAD(P)H oxidase.21,22
In this study, DRW administered to FFR induced a slight but significant decrease in systolic blood pressure, reduced the index of insulin resistance and increased HDL cholesterol. Furthermore, chronic administration of DRW was able to revert the vascular remodeling in FFR in both distribution and resistance arteries. The structural changes in FFR could be associated to vascular smooth muscle cell proliferative behavior previously observed in vitro in this model.4 Red wine administration could protect the NO˙ inactivation process by ROS and also increased the NO˙ generating system activity. The final result could be the inhibition of vascular remodeling associated with this experimental model.
The beneficial effects of moderate red wine consumption have been demonstrated in several studies. Some mechanisms involved in those effects have pointed to the action of antioxidant properties of different polyphenols present in red wine.23 These substances could induce endothelium-dependent vasodilatation in human aorta and coronary arteries, inhibit vascular smooth muscle cell proliferation and protect ischemic myocardium.15,16,24,25In vivo, flavonoids such as quercetin prevent endothelial dysfunction and reduce blood pressure, oxidative stress and end-organ damage in hypertensive animals.26 Quercetin and theaflavin significantly attenuated the atherosclerotic lesion size in aorta arteries in ApoE deficient mice by alleviating inflammation, improving NO˙ bioavailability, and inducing heme oxygenase-1.27 The prevention of angiotensin II-induced hypertension and endothelial dysfunction by red wine polyphenol extract administration, with a normalization of vascular superoxide anion production and NAD(P)H oxidase expression, has also been described.28 It is important to note that the administration of DRW to the control rats had no positive effects on almost of all variables studied, suggesting that under normal conditions DRW adds no further benefit.
Our results are in agreement with epidemiological and experimental evidence demonstrating the beneficial effects of moderated red wine consumption on cardiovascular pathology and contribute to support the hypothesis that the non-alcoholic fraction of wine, represented mainly by phenolic compounds with antioxidant properties, may be the primary factor responsible for this protective effect. Further studies are needed to clarify the molecular mechanism of DRW on vascular alterations.
Experimental
Animals and experimental design
All procedures were performed according to institutional guidelines for animal experimentation and were approved by the Technical and Science Secretary from the School of Medicine of National University of Cuyo, Mendoza, Argentina. Thirty-day-old male Wistar rats, weighting 90–130 g were housed during the experimental period of 10 weeks in a room under conditions of controlled temperature (21 ± 2 °C), humidity and a 12 h light/dark cycle. At the beginning of the study, 32 rats were randomly distributed into two groups: one control group (C) (n = 16) and one experimental group (FFR) (n = 16). After six weeks of treatment, the half of C and experimental groups were assigned to receive 10 mL/Kg daily of DRW for four more weeks. The names of each group were assigned as follows: Control (C); C + DRW; FFR: 10% (w/v) fructose solution administration in the drinking water during all the experimental protocol; and F + DRW. All groups were fed the same standard rat diet (Gepsa-Feeds, Buenos Aires, Argentina) and tap water ad libitum. Administration of 10% fructose (Saporiti Labs., Buenos Aires, Argentina) solution in drinking water was used to achieve the pathological model.
The red wine (Malbec grape variety) was provided by the School of Agricultural Sciences, National University of Cuyo. The phenolic characterization of RW Malbec was evaluated by high performance liquid chromatography as previously described.29 One litre of red wine contained 2.9 g of total phenols expressed as gallic acid. The main phenolic content was (expressed as mg L−1): non-flavonoids: 18.2 gallic acid; 2 caffeic acid; 4.2 cis-caftaric acid, trans-resveratrol: 1.1, flavonoids: 24.1 catechin; 14.2 epicatechin; procyanidin (11.3 B1; 3.1 B3), flavonols: 4.9 quercetin, and anthocyanins (344 malvidin-3-glucoside; 16.2 peonidin-3-glucoside; 60.3 delphinidin-3-glucoside).
Red wine was dealcoholized by rotary evaporation at low pressure and temperature, and then the volume of alcohol evaporated was reconstituted with water, in order to conserve phenolic composition. DRW (10 mL/Kg) was administered in drinking water.
The weight of each animal was measured weekly and the energy intake was recorded twice per week during the experimental period in all groups.
At the end of the experimental period, and after an overnight fast, the rats were weighed, anesthetized with ketamine (50 mg kg−1) and acepromazine (1 mg kg−1). Blood was collected from the abdominal aorta into heparinized tubes. Plasma obtained after centrifugation was frozen at −70 °C until assayed. Arteries and organs were excised aseptically for the measurement of various parameters described below.
Systolic blood pressure (SBP).
Systolic blood pressure was monitored indirectly in conscious, pre-warmed (32 °C) slightly restrained rats by the tail-cuff method and recorded on a Grass Model 7 polygraph (Grass Instruments Co., Quincy, MA, USA).
Biochemical determinations
Plasma glucose, triglycerides, HDL-cholesterol and total cholesterol concentrations were determined using commercial kits by enzymatic colorimetric methods (Wiener Lab, Rosario, Argentina). Insulin was measured by RIA (Coat-A-Count, Siemens, CA, USA), and insulin resistance was assessed using the homeostasis model assessment (HOMA-IR) described by Mathew et al.30 HOMA-IR was calculated using the following formula: HOMA-IR (mmol L−1 × μU/mL) = fasting glucose (mmol L−1) × fasting insulin (μU/mL)/22.5.
Measurements of eNOS activity.
Ca2+/calmodulin-dependent nitric oxide synthase activity was measured in homogenates from mesenteric resistance arteries and left ventricular cardiac tissue by the conversion of L-[3H]arginine to L-[3H]citrulline, as previously described.4 Mesenteric vessels were homogenized on ice for four 15 s intervals with a Politron homogenizer and then sonicated in a buffer (pH 7.4, 37 °C) containing 50 mmol L−1 Tris, 20 mmol l−1 HEPES, 250 mmol L−1 sucrose, 1 mmol L−1 dithiothreitol, 10 μg mL−1 leupeptin, 10 μg mL−1 soybean trypsin inhibitor, 5 μg mL−1 aprotinin and 0.1 mmol L−1 phenyl methyl sulfonyl fluoride. Heart tissue from left ventricle myocardium was also homogenized on ice for four 15 s intervals with a polytron homogenizer and then sonicated in the same buffer. After centrifugation of the homogenates (100 g, 5 min, 4 °C) and determination of the protein content (Bradford method), aliquots of 50 μL were added to 100 μL of a cocktail reaction buffer containing 50 mmol L−1 Tris, 20 mmol L−1 HEPES, 1 mmol L−1 dithiothreitol, 1 mmol L−1 NADPH, 0.1 mmol L−1 tetrahydrobiopterin, 50 μmol L−1 FAD, 50 μmol L−1 FMN, and 10 μCi/ml L-[2,3-3H]-arginine (New England Nuclear, Boston MA), and incubated for 30 min at 37 °C in a shaking bath in the presence of 10 μg ml−1 calmodulin and 3 mmol l−1 CaCl2 or with 3 mmol L−1 EGTA in the absence of Ca2+/calmodulin. The reaction was stopped by adding 1 mL cold distilled water and the mixture applied to an anion-exchange chromatography column containing Dowex AG 50W-X8 (200–400 Mesh) resin previously saturated with 50 μL of 100 mmol L−1 L-citrullin and 2 mL of 50 mmol L−1 Tris, 20 mmol L−1 HEPES buffer (pH 7.4) and eluted with 2 mL of distilled water. Specifically eluted L-[3H]citrulline concentration was determined by liquid scintillation counting. The calcium-dependent NOS activity was calculated as the difference between activity in the presence and absence of Ca2+/calmodulin. Values were corrected to the amount of protein present in the homogenates and the incubation time (dpm/mg protein/min). Each rat mesenteric vascular bed and heart tissue was processed and eNOS activity measured independently.
Measurement of vascular NAD(P)H oxidase activity.
The lucigenin-derived chemiluminescence assay was used to determine NAD(P)H oxidase activity in the aorta as previously described.18 A 2 cm length segment of thoracic aorta was cut, cleaned, washed, transferred to a tube with 2 mL of Jude's Krebs buffer (JKB) containing (in mmol L−1) 2 HEPES, 11.9 NaCl, 0.46 KCl, 0.1 MgSO4·7H2O, 0.015 Na2HPO4, 0.04 KH2PO4, 0.5 NaHCO3, 1.2 CaCl2, 5.5 glucose; pH 7.40; and equilibrated at 37 °C during 30 min. Then the aortic segment was transferred to a tube containing 1 mL JKB and 5 μmol L−1 lucigenin and left in darkness at room temperature for 10 min. This concentration of lucigenin does not appear to be involved in redox cycling and specifically detects superoxide anion. To assess NAD(P)H oxidase activity, 500 μmol L−1 βNAD(P)H was added and chemiluminescence was immediately measured in a liquid scintillation counter (LKB Wallac Model 1219 Rack-Beta Scintillation Counter, Finland) set in the out-of coincidence mode. Time-adjusted and normalized to tissue weight scintillation counts were used for calculations. Measurements were repeated in the absence and presence of diphenylene iodinium (DPI) (10−6 mol L−1), which inhibits flavin-containing enzymes, including NAD(P)H oxidase.
Tissue preservation.
Tissue samples for histopathology were processed as previously reported.10 Samples from all rats were used in these observations. The kidneys were in vivo perfused with PBS (pH 7.40, 4 °C) through the renal artery over 5 min. For histological studies, left kidneys were then perfused with 4% paraformaldehyde solution for 10 min, then additionally fixed by immersion in the same solution for 48 h, introduced to a 30% sucrose solution and kept at −70 °C. Five μm thick tissue slices were transversely cut through the entire kidney on a cryostat (Microm HM 505E, Germany) at −26 °C and processed for histological studies. Common left carotid arteries were fixed and processed as described above for kidneys.
Histopathology and morphometry.
Lumen to media ratio in kidney arteries transversal slices from common left carotid artery and left kidney were placed on microscope slides and stained with Masson's trichrome solution and examined under a light microscope (Nikon Optiphot-2, Kanagawa, Japan). Images were digitalized with a digital camera (GP-KR222 color CCD, Panasonic, Osaka, Japan) and processed with an analysis system Scion Image 4.01 (Scion, Bethesda, MD, USA). To evaluate the renal arterial wall thickening, images from three different artery types were studied in each kidney: interlobar, arcuate and interlobular arteries. The lumen-to-wall media ratio (the internal diameter to the medial thickness) was then calculated. Forty slices from each kidney were processed and 5 to 10 arteries of each type in each slice were analyzed, in order to obtain an average value for each rat. The average values were then used for final analysis. Common left carotid arteries were sectioned transversely. L/M was then calculated in 10 slices from each artery, in order to obtain an average value for each rat and then used for final analysis.
Reagents
Unless otherwise noted, reagents were purchased from Sigma Chemical Co, MO USA. All other chemicals were of molecular biology or reagent grade.
Statistical and data analysis
Results were expressed as mean and their deviation errors. The statistical significance was assessed by one-way ANOVA followed by Student-Newman-Keuls post-test using GraphPad Prism version 5.00 for Windows, GraphPad Software, San Diego, California USA. Differences were considered significant at p < 0.05. In the figures and tables, data shown without a common letter differ at a p < 0.05 significance level.
Conclusions
The non-alcoholic constituents of red wine increased the eNOS activity, reduced the activity of the enzyme NAD(P)H oxidase and reversed vascular remodeling. The antioxidant properties of polyphenols could be responsible for the beneficial effects of DRW.
Acknowledgements
We thank Susana Gonzalez and Cristina Lama for their technical assistance. This work was supported by grants from Program 06/P01 SECTyP Universidad Nacional de Cuyo, and PIP-5192 CONICET.
References
- J. E. Tooke and M. M. Hannemann, Adverse endothelial function and the insulin resistance syndrome, J. Intern. Med., 2000, 247, 425–31 CrossRef CAS.
- I. S. Hwang, H. Ho, B. B. Hoffman and G. M. Reaven, Fructose-induced insulin resistance and hypertension in rats, Hypertension., 1987, 10, 512–516 CAS.
- X. Wang, Y. Hattori, H. Satoh, C. Iwata, N. Banba, T. Monden, K. Uchida, Y. Kamikawa and K. Kasai, Tetrahydrobiopterin prevents endothelial dysfunction and restores adiponectin levels in rats, Eur. J. Pharmacol., 2007, 555, 48–53 CrossRef CAS.
- R. M. Miatello, N. R. Risler, C. M. Castro, E. S. González, M. E. Rüttler and M. C. Cruzado, Aortic smooth muscle cell proliferation and endothelial nitric oxide synthase activity in fructose-fed rats, Am. J. Hypertens., 2001, 14, 1135–1141 CrossRef CAS.
- R. M. Miatello, N. R. Risler, C. M. Castro, M. E. Rüttler and M. C. Cruzado, Effects of Enalapril on the Vascular Wall in an Experimental Model of Syndrome X, Am. J. Hypertens., 2002, 15, 872–878 CrossRef CAS.
- R. M. Touyz and E. L. Schiffrin, Signal transduction mechanisms mediating the physiological and pathophysiological actions of angiotensin II in vascular smooth muscle cells, Pharmacol. Rev., 2000, 52, 639–672 CAS.
- A. Fortuño, G. San José, M. U. Moreno, J. Díez and G. Zalba, Oxidative stress and vascular remodelling, Exp. Physiol., 2005, 90, 457–62 Search PubMed.
- X. Chen, R. Touyz, J. B. Park and E. Schiffrin, Antioxidant effects of Vitamin C and E are associated with altered activation of vascular NADPH oxidase and superoxide dismutase in stroke-prone SHR, Hypertension, 2001, 38, 606–611 CrossRef CAS.
- G. Kojda and D. Harrison, Interactions between NO and reactive oxygen species: pathophysiological importance in atherosclerosis, hypertension, diabetes and heart failure, Cardiovasc. Res., 1999, 43, 562–571 CrossRef CAS.
- N. F. Renna, M. A. Vazquez, M. C. Lama, S. Gonzalez and R. Miatello, Effect of chronic aspirin administration on an experimental model of metabolic syndrome, Clin. Exp. Pharmacol. Physiol., 2009, 36, 162–168 CrossRef CAS.
- M. A. Vazquez-Prieto, R. E. Gonzalez, N. F. Renna, C. R. Galmarini and R. M. Miatello, Aqueous Garlic Extracts Prevents Oxidative Stress and Vascular Remodeling in an Experimental Model of Metabolic Syndrome, J. Agric. Food Chem., 2010, 58, 6630–6635 CrossRef CAS.
- S. Renaud and M. de Lorgeril, Wine, alcohol, platelets and the French paradox for coronary artery disease, Lancet, 1992, 339, 1523–6 CrossRef CAS.
- J. Belleville, The French Paradox: Possible involvement of ethanol in the protective effect against cardiovascular diseases, Nutrition, 2002, 18, 173–177 CrossRef CAS.
- J. C. Stoclet, T. Chataigneau, M. Ndiaye, M. H. Oak, J. El Bedoui, M. Chataigneau and V. B. Schini-Kerth, Vascular protection by dietary polyphenols, Eur. J. Pharmacol., 2004, 500, 299–313 CrossRef CAS.
- F. Leighton, A. Cuevas and V. Guasch, Plasma polyphenols and antioxidants oxidative DNA damage and endothelial function in a diet and wine intervention study in humans, Drugs. Exp. Clin. Res., 1999, 25, 133–141 Search PubMed.
- K. Iijima, M. Yoshizumi, M. Hashimoto, S. Kim, M. Eto, J. Ako, Y. Q. Liang, N. Sudoh, K. Hosoda, K. Nakahara, K. Toba and Y. Ouchi, Red wine polyphenols inhibit proliferation of vascular smooth muscle cells and downregulate expression of cyclin A gene, Circulation, 2000, 101, 805–811 CAS.
- R. M. Miatello, M. A. Vazquez, N. F. Renna, M. C. Cruzado, A. Z. Ponce Zumino and N. R. Risler, Chronic administration of resveratrol prevents biochemical cardiovascular changes in fructose-fed rats, Am. J. Hypertens., 2005, 18, 864–870 CrossRef CAS.
- M. Cruzado, N. Risler, R. Miatello, G. Yao, E. Schiffrin and R. Touyz, Vascular smooth muscle cell NAD(P)H oxidase activity during the development of hypertension: effect of angiotensin II and role of insulin-like growth factor-1 receptor transactivation, Am. J. Hypertens., 2005, 18, 81–7 CrossRef CAS.
- J. S. Beckman and W. H. Koppenol, Nitric oxide, superoxide, and peroxynitrite: the good, the bad, and ugly, Am. J. Physiol., 1996, 271, 1424–1437.
- M. Sanchez, M. Galisteo, R. Vera, I. C. Villar, A. Zarzuelo, J. Tamargo, F. Perez-Vizcaino and J. Duarte, Quercetin downregulates NAPDH oxidase, increases eNOS activity and prevents endotelial dysfunction in spontaneously hypertensive rats, J. Hypertens., 2006, 24, 75–84 CrossRef CAS.
- Y. Steffen, C. Gruber, T. Schewe and H. Sies, Mono-O-methylated flavonols and other flavonoids as inhibitors of endothelial NADPH oxidase, Arch. Biochem. Biophys., 2008, 469, 209–219 CrossRef CAS.
- Y. Steffen, T. Schewe and H. Sies, (-)-Epicatechin elevates nitric oxide in endothelial cells via inhibition of NADPH oxidase, Biochem. Biophys. Res. Commun., 2007, 359, 828–833 CrossRef CAS.
- C. G. Fraga, Plant polyphenols: how to translate their in vitro antioxidant actions to in vivo conditions, IUBMB Life, 2007, 59, 308–315 Search PubMed.
- S. M. Mosca and H. E. Cingolani, Cardioprotection from ischemia/reperfusion induced by red wine extract is mediated by K(ATP) channels, J. Cardiovasc. Pharmacol., 2002, 40, 429–437 CrossRef CAS.
- J. C. Fantinelli, G. Schinella, H. E. Cingolani and S. M. Mosca, Effects of different fractions of a red wine non-alcoholic extract on ischemia-reperfusion injury, Life Sci., 2005, 76, 2721–2733 CrossRef CAS.
- F. Perez-Vizcaino, J. Duarte and R. Andriantsitohaina, Endothelial function and cardiovascular disease: effects of quercetin and wine polyphenols, Free Radical Res., 2006, 40, 1054–1065 CrossRef CAS.
- W. M. Loke, J. M. Proudfoot, J. M. Hodgson, A. J. McKinley, N. Hime, M. Magat, R. Stocker and K. D. Croft, Specific dietary polyphenols attenuate atherosclerosis in apolipoprotein E-knockout mice by alleviating inflammation and endothelial dysfunction, Arterioscler., Thromb., Vasc. Biol., 2010, 30, 749–757 Search PubMed.
- M. Sarr, M. Chataigneau, S. Martins, C. Schott, J. El Bedoui, M. H. Oak, B. Muller, T. Chataigneau and V. B. Schini-Kerth, Red wine polyphenols prevent angiotensin II induced hypertension and endothelial dysfunction in rats: role of NADPH oxidase, Cardiovasc. Res., 2006, 71, 794–802 CrossRef CAS.
- M. Fanzone, A. Peña-Neira, V. Jofré, M. Assof and F. Zamora, Phenolic characterization of malbec wines from mendoza province (Argentina), J. Agric. Food Chem., 2010, 58, 2388–2397 CrossRef CAS.
- D. R. Matthews, J. P. Hosker, A. S. Rudenski, B. A. Naylor, D. F. Treacher and R. C. Turner, Homeostasis model assessment:insulin resistance and beta-cell function from fasting plasma glucose and insulin concentrations in man, Diabetologia, 1985, 28, 412–419 CrossRef CAS.
|
This journal is © The Royal Society of Chemistry 2010 |
Click here to see how this site uses Cookies. View our privacy policy here.