DOI:
10.1039/C0AY00076K
(Paper)
Anal. Methods, 2010,
2, 837-843
Received
30th January 2010
, Accepted 27th April 2010
First published on
27th May 2010
Abstract
A disposable electrode, modified with multiwalled carbon nanotubes (MWCNTs) and gold nanoparticles (AuNPs), was developed to serve as a sensor for the simultaneous determination of dihydroxybenzene isomers (hydroquinone, catechol and resorcinol). The modified electrode was fabricated by electrodepositing AuNPs on a MWCNTs decorated screen-printed electrode (SPE), then it was characterized in morphology and electrochemical properties and utilized to determine the isomers of dihydroxybenzene in water samples. Compared with bare SPE, AuNPs-modified SPE, and MWCNTs-modified SPE, the fabricated SPE has much stronger electrocatalytic activity for the oxidation of dihydroxybenzenes with the increase of the peak current and the decrease of the potential difference (△Ep) between the anodic and cathodic peaks. By using differential pulse voltammetry (DPV), the three isomers can be determined simultaneously and sensitively at the modified SPE. For hydroquinone, catechol and resorcinol, the oxidation peak currents are linear to the concentrations at the range of 2.0 × 10−6∼7.3 × 10−4 M, 2.0 × 10−6∼7.3 × 10−4 M and 3.0 × 10−6∼9.6 × 10−4 M with the detection limits of 3.9 × 10−7 M, 2.6 × 10−7 M and 7.2 × 10−7 M, respectively. Moreover, a rapid on-filed determination is convenient to be performed at this disposable modified SPE incorporated in a portable electrochemical system. These results reveal that the presented sensor can be used for the simultaneous and sensitive on-field determination of dihydroxybenzene isomers.
1 Introduction
Hydroquinone (HQ), catechol (CC) and resorcinol (RC) are three isomers of dihydroxybenzenes which are important environmental pollutants because they are toxic to humans and difficult to be degraded in the ecological system.1 These isomers are widely used as industrial raw and synthetic intermediates in many fields such as cosmetic, pharmaceutical, tanning, dye and pesticide industries. During the normal operation of these industries, environmental accidents by dihydroxybenzene isomers happen sometimes due to inevitable releases, and these dihydroxybenzene isomers usually coexist in environmental samples because of their similar structures and properties.2 In order to know their pollution level in the relevant accident rapidly, it is very necessary to develop the fast and convenient methods which can determine dihydroxybenzene isomers simultaneously. So far, various determination methods have been developed like chromatography,2 spectrophotometry,3 and electrochemical method.4 Among them, the electrochemical method, for its rapid response, high sensitivity and easy operation, is one of the most favorable techniques. Nevertheless, the oxidation-reduction peak potentials of the isomers cannot be separated obviously enough to simultaneously determine the isomers using conventional electrodes.5
In recent years, much effort has been devoted to the electrochemical separation and determination of dihydroxybenzene isomers at new electrode materials. The early investigation concerning the simultaneous electrochemical determination of the three isomers was conducted at a glass carbon electrode (GCE) modified with multiwalled carbon nanotubes (MWCNTs), which yield promising results.6 In that study, using the MWCNTs-modified electrode and the first order derivative technique, the authors succeeded in simultaneously determining the dihydroxybenzene isomers in artificial wastewater. Further research on the simultaneous determination of dihydroxybenzenes was performed at the new complex-modified electrodes, which were fabricated by electropolymerizing acidic chrome blue K or bromophenol blue on MWCNTs-modified GCE.7,8 The results showed that the composite electrodes could be used to simultaneously determine the isomers of dihydroxybenzene through first order derivative voltammetry with good reproducibility and stability. While, the simultaneous dihydroxybenzenes determination was also achieved at a single-walled carbon nanotubes modified GCE with a 2.5 order derivative technique and a satisfying detection limit was obtained.9 In addition, the simultaneous electrochemical determination of dihydroxybenzene isomers was explored at other electrode materials, including, graphite-epoxy composite, surfactant, ordered mesoporous carbons, etc.10–16
Metal nanoparticles are favorable for use in electrode modification due to their conspicuous physical and chemical properties, which can improve electrochemical properties of an electrode.17 As a matter of fact, metal nanoparticles, especially gold nanoparticles (AuNPs) supported on conducting nanomaterials, can be used to remarkably enhance the sensitivity of the electrochemical sensor in virtue of their high surface areas and densely populated unsaturated surface coordination sites for catalysis.18 In addition, the screen-printed electrode (SPE) has enabled the production of modern sensors which can be incorporated in portable systems, an important requirement of detecting methods for the direct on-field analysis of a sample without alteration of the natural environmental conditions.19–21 However, their application to simultaneous electrochemical determination of dihydroxybenzene isomers has received little attention.
In this work, a novel electrochemical sensor based on AuNPs and MWCNTs modified SPE (AuNPs/MWCNTs/SPE) was developed for a simultaneous and sensitive way to determine dihydroxybenzenes on field. AuNPs/MWCNTs/SPE was fabricated by electrodepositing AuNPs on MWCNTs-decorated SPEs and was characterized in morphology and electrochemical properties. Then, with differential pulse voltammetry (DPV), simultaneous determination of the dihydroxybenzene isomers was investigated at the developed AuNPs/MWCNTs/SPE. Furthermore, on-field determination of the water samples was carried out at this developed electrochemical sensor, which showed a promising performance on the analysis of dihydroxybenzene isomers.
2 Experimental
2.1. Reagents
Hydroquinone, catechol, resorcinol and HAuCl4 were purchased from Aladdin Reagent Co. Ltd. (Shanghai, China). All reagents were analytical reagent grade and all solutions were prepared with deionized water obtained with a Mili-Q System (Millipore, USA). Specially, the solutions of hydroquinone, catechol and resorcinol were prepared freshly before use. MWCNTs were supplied by Shenzhen Nanotech Port Co. Ltd. (Shenzhen, China) with a typical diameter of 10∼30 nm and length of 5∼15 μm, and their purity was 95∼98%. Besides, silver ink, carbon ink, silver/silver chloride ink (Camnano Technology Ltd., Xuzhou, China), and insulating ink (Jujo Chemical Co., Ltd., Japan) were used to fabricate SPE.
2.2. Apparatus
A disposable SPE, with a standard three-electrode system and a 3.1 mm2 working area, was manufactured onto a glass fiber plate with an AT-25P screen printing machine (ATMA CHAMP ENT. Corp., Taiwan). Before use, this SPE was pretreated in pH 7.0 phosphate buffer solution (PBS) by applying an anodic potential of 2.0 V for 200 s. The scanning electron microscopy (SEM) images of the AuNPs/MWCNTs/SPE were obtained by using a JSM-6360LV SEM instrument (JEOL, Japan). All the electrochemical experiments were carried out with a portable detecting system composed of a CHI-1211A electrochemical workstation (Chenhua Instrument Company, Shanghai, China) a laptop, and a SPE (Fig. 1).
2.3. Fabrication of AuNPs/MWCNTs/SPE
MWCNTs were purified by refluxing in concentrated nitric acid for 12 h at a constant temperature according to a previously described procedure which caused segmentation and carboxylation of the MWCNTs at their terminus.22 Then, 2.0 mg purified and functionalized MWCNTs were dispersed into 1.0 ml 1.0% HAc (acetic acid) with ultrasonic agitation, and a black colloidal solution was found. To prepare MWCNTs-decorated SPE (MWCNTs/SPE), 3 μl of MWCNTs colloid was dropped onto the working area of the manufactured bare SPE. After dried in air, MWCNTs/SPE was rinsed with water three times to remove the impurities. The clean MWCNTs/SPE was immersed into 0.5 M H2SO4 containing 1.0 mM HAuCl4, and electrochemical deposition of gold nanoparticles was conducted for 200 s at −0.2 V (vs. Ag/AgCl) referring to the reported literature.16 Thus, the AuNPs/MWCNTs/SPE was obtained and stored at ambient temperature for use following rinsing with water. Under the same electrodepositing condition, AuNPs-modified SPE (AuNPs/SPE) was obtained as well.
3 Results and discussion
3.1. Characterization of AuNPs/MWCNTs/SPE
The morphologies of the prepared AuNPs/MWCNTs/SPE and bare SPE were observed with SEM and the typical SEM images are shown in Fig. 2. As can be seen from Fig. 2, the surface of the bare SPE is almost smooth while the multiwalled carbon nanotubes, coated on the working area of the bare SPE, are twisted together to form a three-dimensional structure with porous interspaces. On and among the MWCNTs coating, gold nanoparticles are built and congregated in a flower-like configuration, whose average size comes within several hundreds of nanometres. This stereo netlike modification coating may bring some amazing electrochemical activities on the modified SPE because of the conspicuous physicochemical properties and possible synergy effects of the well-distributed MWCNTs and AuNPs.17,23
By cyclic voltammetry, the electrochemical properties of the AuNPs/MWCNTs/SPE was characterized in a 0.1 mM [Fe(CN)6]3−/4− solution and compared with that of other electrodes. Fig. 3 shows the cyclic voltammetric responses of [Fe(CN)6]3−/4− at AuNPs/MWCNTs/SPE, AuNPs/SPE, MWCNTs/SPE and bare SPE. The potential difference (△Ep) between the anodic and cathodic peaks at AuNPs/MWCNTs/SPE is 74 mV, whereas at bare SPE, this difference is 165 mV. The smaller △Ep indicates that the electrochemical reversibility of [Fe(CN)6]3−/4− at AuNPs/MWCNTs/SPE can be improved strongly. Further more, the response current at AuNPs/MWCNTs/SPE is 6.4-fold, 1.9-fold and 2.7-fold larger than that at bare SPE, AuNPs/SPE and MWCNTs/SPE, respectively. This implies that the AuNPs/MWCNTs/SPE has higher electroactive surface area, which agrees with the above mentioned supposition. Therefore, these results demonstrate that the AuNPs/MWCNTs/SPE has relatively better electrochemical reaction ability and that the enhanced electrochemical properties may be due to the high surface areas and densely populated unsaturated surface coordination sites for catalysis coming from AuNPs and MWCNTs.
3.2. Electrochemical behaviors of dihydroxybenzene isomers
The electrochemical behaviors of the dihydroxybenzenes in a mixed solution at different electrodes were investigated by cyclic voltammetry. Fig. 4 displays the cyclic voltammograms of 1.6 × 10−4 M HQ, CC and RC in pH 7.0 PBS respectively. At the bare SPE, there are two broad oxidation peaks at potentials about 0.4 V and 0.6 V, which indicates the oxidation peaks of HQ and CC overlap to form a wide peak and cannot be divided. The similar results are found from the AuNPs/SPE. Whereas, at the MWCNTs/SPE, three well-defined oxidation peaks are present at potentials of 0.05, 0.15, and 0.54 V corresponding to HQ, CC, and RC respectively. At the same time, the oxidation peaks are all heightened. Though it is revealed that the MWCNTs/SPE has better selectivity for dihydroxybenzenes oxidation peaks, the peak currents are still comparatively lower. On the contrary, at the AuNPs/MWCNTs/SPE, the oxidation peak potentials of three isomers are far away from each other and the oxidation peak currents are much greater than those at the other electrodes. It may be owing to the unique properties of AuNPs/MWCNTs complex. On one hand, the three-dimensional porous structure of the AuNPs/MWCNTs has different space resistances to the dihydroxybenzene isomers, which affects the rates of dihydroxybenzenes diffusing to the electrode surface.24 Thus, the three isomers have different peak potentials to be separated. On the other hand, many favorable sites for electron transferring to molecules may be provided by lots of functional groups and surface defects on the AuNPs/MWCNTs coating, which results in a large and increasing peak current.18,23 Consequently, these results show that the AuNPs/MWCNTs/SPE combined the catalysis and the selectivity of the AuNPs/MWCNTs complex, and that this modified electrode may be used in the simultaneous analysis of the dihydroxybenzene isomers.
From Fig. 4, it can also be seen that there is no reduction peak of RC in the cyclic voltammograms, which means its electrochemistry irreversibility. According to the literature,25 phenoxy-type radical may be generated in the first stage of the electro-oxidation of RC. This radical is not stable and can be further oxidized to the quinone form or can couple with other radicals or dihydroxybenzenes to form polymers, which cannot be reduced to RC again. Thus, no reduction peak is observed in the cyclic voltammograms. These formed compounds may deactivate the electrode. However, this will not influence the analytical reproducibility in this work due to the disposable and low-cost properties of the proposed modified SPE, which has been proven to be excellent in coincidence (section 3.5). A new modified SPE can be used for a new determination of dihydroxybenzene sample to avoid the problem caused by electrode deactivation and ensure the analytical reproducibility.
3.3. Effects of scan rate and pH
The scan rate dependence of cyclic voltammetry for the AuNPs/MWCNTs/SPE was studied in the pH 7.0 PBS containing 1.6 × 10−4 M HQ, CC and RC. It can be found from the result in Fig. 5 that the oxidation peak potentials shift slightly to a more positive value with the increase of scan rate. In the mean time, the oxidation peak currents of dihydroxybenzenes at the AuNPs/MWCNTs/SPE increase linearly with the square root of scan rate in the range from 20 mV s−1 to 160 mV s−1. This means that the electrochemical oxidation of the dihydroxybenzene isomers is a diffusion-controlled process.
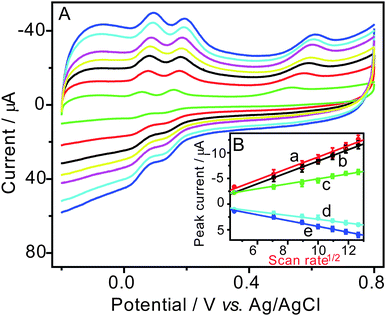 |
| Fig. 5 A. Cyclic voltammograms of hydroquinone, catechol and resorcinol (1.6 × 10−4 M for each) in pH 7.0 PBS at a scan rate of 20, 50, 80, 100, 120, 140 and 160 mV s−1. B. The relationship between the peak currents and the square root of scan rates. a: oxidation peak current of catechol, b: oxidation peak current of hydroquinone, c: oxidation peak current of resorcinol, d: reduction peak current of catechol, e: reduction peak current of hydroquinone. | |
Since the proton takes part in the electrochemical reaction, the acidity of electrolyte is considered to significantly affect the electrochemical behavior of dihydroxybenzenes.6 Accordingly, the relationship between the pH of solution and the oxidation peak potentials of HQ, CC and RC was investigated by cyclic voltammetry, and the result is demonstrated in Fig. 6. It can be observed that the oxidation peak potentials of the three isomers shift to more negative values with the increase of pH values and that these peak potentials increase linearly with the rising pH values in the range of pH 4.0∼9.0 (Fig. 6B). The regression equations and correlation coefficients for these lines are Ep = pH 0.488–0.057 (R = −0.995) for HQ, Ep = pH 0.591–0.057 (R = −0.994) for CC, and Ep = pH 1.010– 0.062 (R = −0.991) for RC, respectively. The slope values of the three equations are −57, −57 and −62 mV/pH, which are close to the theoretical value of −58 mV (20 °C). This indicates that a loss proton transfer for each of isomers accompanies the electrochemical reduction. Besides, the three lines are almost parallel, which implies that the difference of the peak potentials between HQ, CC and RC is constant and that the pH change in this range may not improve the resolution remarkably. In addition, with increase of pH value, the peak currents of HQ, CC, and RC increase and then decrease after pH exceeding 7.0 (Fig. 6C), which is similar with the reported results.12,26 This may be due to that dihydroxybenzenes become more ready to be deprotonated to form anions when the pH exceeds 7.0.26 With the increase of pH, those oxygen-containing functional groups at the modified electrode may become deprotonated and possessed negative charges as well. Electrostatic repulsion between the analytes and the electrode might be one of the reasons to cause the adsorptions of analytes on the electrode inefficient at higher pH.27 Thus, the responding oxidation peak currents in cyclic voltammograms decrease in a certain degree. Consequently, pH 7.0 was chosen to perform the test in present work.
3.4. Simultaneous determination of dihydtroxybenzene isomers
To test the feasibility of AuNPs/MWCNTs/SPE in the simultaneous and sensitive determination of dihydroxybenzene isomers, differential pulse voltammetry (DPV) was performed for different concentrations of one isomer at the AuNPs/MWCNTs/SPE in the presence of a constant concentration of the other two isomers (8.2 × 10−5 M for each) under the condition of 50 mV s−1 scan rate and pH 7.0 PBS. From the results in Fig. 7–9, we can see the monotonic increase of the peak current corresponding to the oxidation of one isomer with the increase of its concentration. For other two isomers with constant concentrations, the oxidation peak currents change slightly, which does not influence the determination of the two isomers. It means that the oxidation of the three isomers at the presented electrode may take place independently.
Moreover, it can be found from Fig. 10 that the oxidation peak current of each component is linearly proportional to its concentration in the range of 2.0 × 10−6∼7.3 × 10−4 M for HQ, 2.0 × 10−6∼7.3 × 10−4 M for CC and 3.0 × 10−6∼9.6 × 10−4 for RC, respectively. The correlation factors of the straight lines are more than 0.99 for all the isomers. Correspondingly, the limits of detection are 3.9 × 10−7 M for HQ, 2.6 × 10−7 M for CC and 7.2 × 10−7 M for RC (at a signal-to-noise ratio of 3), respectively. This may imply an excellent response effectiveness of the AuNPs/MWCNTs/SPE. Therefore, for the high resolving ability and sensitivity, the developed modified electrode can be used for the simultaneous and sensitive determination of dihydroxybenzenes with DPV technique.
3.5. Reproducibility and interferences
The coincidence of electrochemical property among different individual SPEs (or modified SPE) is crucial issue to perform its disposable advantage and to evaluate its perfect reproducibility of the determination results. To verify this coincidence and reproducibility, six different bare SPEs were chosen randomly from 100 pieces of store SPEs and were modified with AuNPs and MWCNTs in the same procedure. Then, using the modified SPEs, repetitive measurements were performed for the mixture of hydroquinone, catechol and resorcinol (1.6 × 10−4 M for each). The results showed that the relative standard deviations (RSD) of peak currents for dihydroxybenzene isomers in a day are no more than 2.3, 2.1 and 3.4% (n = 6). This indicates that the modified electrode has excellent coincidence so using it to determinate the concentration of dihydroxybenzene isomers simultaneously will have a good reproducibility and accuracy.
The possible interferences of some inorganic ions and organic compounds were also investigated with a mixed dihydroxybenzenes solution (1.6 × 10−4 M of HQ, CC and RC for each). In the investigation, if the presence of an inorganic ion or organic compound changed the average current signals of dihydroxybenzenes only with deviations below 5%, it was considered that no interference was caused. The results (Fig. 11) display that a 1000-fold of K+, Na+, Cl−, HPO42−, H2PO4−, Ac−, NO3−, Cu2+ and 100-fold of ethanol, acetone have no influences on the signals of the dihydroxybenzenes. Besides, the results also indicate that 5-fold of phenol, nitrophenol or aniline almost have no effect on the determination of dihydroxybenzene isomers, while they interfere that determination, especially for RC, when their concentration increase to 10-fold.
 |
| Fig. 11 Interferences of some inorganic ions and organic compounds on the determination of dihydroxybenzene isomers. A: a mixture of K+, Na+,Ca2+, Mg2+, Cl−, HPO42−, H2PO4−, Ac−, NO3− Cu2+ (1000-fold excess for each); B: a mixture of ethanol and acetone (100-fold excess for each); C∼–E: 5-fold excess of phenol, nitrophenol and aniline; F–H: 10-fold excess of phenol, nitrophenol and aniline. | |
Associating with a portable electrochemical workstation and a laptop, the AuNPs/MWCNTs/SPE was applied to the on-field determination of tap water and the water samples of a local river (Qingchun River, Shanghai, China). The results demonstrated that no dihydroxybenzene was detected in the water samples with the modified electrode, which meant that dihydroxybenzenes contents were below the detection limits. In order to evaluate the validity of the proposed analysis method, different polluted samples were prepared by adding the standard solution of dihydroxybenzenes in the water samples and were tested with the AuNPs/MWCNTs/SPE. The results obtained are also summarized in Table 1. As can be seen, when known amounts of HQ, CC and RC are added into the water samples, quantitative recoveries of 96–105% are obtained. This may imply the proposed modified electrode could be used to portably determine the dihydroxybenzene isomers on the filed of some real water samples.
Sample |
Compounda |
Added/10−5 M |
Foundb/10−5 M |
Recovery (%) |
HQ: hydroquinone, CC: catechol, RC: resorcinol.
Average value of five determinations.
|
Tap water |
HQ |
8.1 |
8.2 |
101.2 |
CC |
8.1 |
8.0 |
98.8 |
RC |
8.1 |
8.4 |
103.7 |
River water |
HQ |
8.1 |
8.4 |
103.7 |
CC |
8.1 |
7.8 |
96.3 |
RC |
8.1 |
8.5 |
104.9 |
4 Conclusions
A novel modified electrode was successfully fabricated by electrodepositing AuNPs on MWCNTs-decorated SPE. The developed AuNPs/MWCNTs/SPE exhibits an excellent electrocatalytic activity and reversibility towards the oxidation of dihydroxybenzene isomers. Besides, in a mixture solution, the isomer oxidation peaks become well resolved at the modified electrode and are separated by more than 100 mV. This enables the simultaneous and sensitive determination of dihydroxybenzene isomers to be achieved by using DPV technique without any pre-treatment. On the other hand, benefitting from disposable and low-cost advantages of SPE, it is facile to perform rapid on-filed determination using the AuNPs/MWCNTs/SPE incorporated in portable systems. Thus, electrochemical sensor such as that presented herein provides attractive potential for the development of the methods to simultaneously and sensitively determine dihydroxybenzenes and its integration in convenient on-field use, which is significant to the emergency detection, especially in the pollution accident.
Acknowledgements
This work was supported by National High Technology Research and Development Program of China (863 Project No. 2008AA06A406), the Shanghai Postdoctoral Sustentation Fund, China (No. 09R21411700) and the Ministry of Health of China (No. 2009ZX10004-301).
Notes and references
- H. E. Wise and P. D. Fahrenthold, Environ. Sci. Technol., 1981, 15, 1292 CrossRef.
- H. Cui, C. X. He and G. W. Zhao, J. Chromatogr., A, 1999, 855, 171 CrossRef CAS.
- G. N. Chen, J. S. Liu, J. P. Duan and H. Q. Chen, Talanta, 2000, 53, 651 CrossRef CAS.
- R. M. de Carvalho, C. Mello and L. T. Kubota, Anal. Chim. Acta, 2000, 420, 109 CrossRef CAS.
- I. C. Vieira and O. Fatibello-Filho, Talanta, 2000, 52, 681 CrossRef CAS.
- Y. P. Ding, W. L. Liu, Q. S. Wu and X. G. Wang, J. Electroanal. Chem., 2005, 575, 275 CrossRef CAS.
- P. H. Yang, W. Z. Wei and L. Yang, Microchim. Acta, 2007, 157, 229 CrossRef CAS.
- P. H. Yang, W. Z. Wei, C. Y. Tao and J. X. Zeng, Bull. Environ. Contam. Toxicol., 2007, 79, 5 CrossRef CAS.
- Z. H. Wang, S. J. Li and Q. Z. Lv, Sens. Actuators, B, 2007, 127, 420 CrossRef.
- K. H. Xue, W. Xu and S. Y. Yin, J. Electrochem. Soc., 2007, 154, F147 CrossRef CAS.
- L. L. Skvortsova, V. N. Kiryushov, T. P. Aleksandrova and O. V. Karunina, J. Anal. Chem., 2008, 63, 258 CAS.
- J. P. Dong, X. M. Qu, L. J. Wang, C. J. Zhao and J. Q. Xu, Electroanalysis, 2008, 20, 1981 CrossRef CAS.
- W. Z. Liu, X. G. Wang, Q. S. Wu and Y. P. Ding, J. Anal. Chem., 2009, 64, 54 CrossRef CAS.
- D. D. Zhang, Y. G. Peng, H. L. Qi and C. X. Zhang, Sens. Actuators, B, 2009, 136, 113 CrossRef.
- J. Bai, L. P. Guo, J. C. Ndamanisha and B. Qi, J. Appl. Electrochem., 2009, 39, 2497 CrossRef CAS.
- L. Han and X. Zhang, Electroanalysis, 2009, 21, 124 CrossRef CAS.
- S. Hrapovic, Y. L. Liu and J. H. T. Luong, Anal. Chem., 2007, 79, 500 CrossRef CAS.
- H. Xu, L. P. Zeng, S. J. Xing, G. Y. Shi, Y. Z. Xian and L. T. Jin, Electrochem. Commun., 2008, 10, 1839 CrossRef CAS.
- M. Tudorache and C. Bala, Anal. Bioanal. Chem., 2007, 388, 565 CrossRef CAS.
- D. K. Kampouris, R. O. Kadara, N. Jenkinson and C. E. Bands, Anal. Methods, 2009, 1, 25 RSC.
- N. A. Choudhry, D. K. Kampouris, R. O. Kadara, N. Jenkinson and C. E. Bands, Anal. Methods, 2009, 1, 183 RSC.
- H. Hiura, T. W. Ebbesen and K. Tanigaki, Adv. Mater., 1995, 7, 275 CrossRef CAS.
- Z. Yu and P. J. Burke, Nano Lett., 2005, 5, 1403 CrossRef CAS.
- M. A. Ghanem, Electrochem. Commun., 2007, 9, 2501 CrossRef CAS.
- B. Nasr, G. Abdellatif, P. Canizares, C. Saez, J. Lobato and M. A. Rodrigo, Environ. Sci. Technol., 2005, 39, 7234 CrossRef CAS.
- H. L. Qi and C. X. Zhang, Electroanalysis, 2005, 17, 832 CrossRef CAS.
- Y. Y. Sun, J. J. Fei, K. B. Wu and S. S. Hu, Anal. Bioanal. Chem., 2003, 375, 544 CAS.
|
This journal is © The Royal Society of Chemistry 2010 |
Click here to see how this site uses Cookies. View our privacy policy here.