DOI:
10.1039/B9AY00293F
(Paper)
Anal. Methods, 2010,
2, 558-563
Determination of trace quantity of aluminium in dialysate concentrates using solid phase and cloud point extraction methods
Received
11th December 2009
, Accepted 14th January 2010
First published on
16th February 2010
Abstract
A new method is reported for the separation of aluminium ions (Al3+) from interfering cations in dialysis concentrate solutions (DS) through solid phase extraction (SPE) using 2-methyl-8-hydroxyquinoline (2-MHQ). While separated Al3+ was preconcentrated by cloud point extraction (CPE) using 8-hydroxyquinoline (8-HQ) as complexing reagent, the resulting complex was entrapped in non-ionic surfactant (Triton X-114). Then fluorescence intensity of the Al3+-8-HQ complex was measured by spectrofluorimetry and the results were compared with those obtained by flame atomic absorption spectrometry. The chemical variables affecting the analytical performance of the CPE were studied and optimized. The validity of methodology was checked by the standard addition method, under optimum experimental conditions >98% recovery of Al3+ was obtained. The enrichment factor and detection limit of Al3+ for the preconcentration of 50 ml of DS were found to be 200 and 0.384 μg L−1, respectively. The relative standard deviation for six replicate determinations of Al3+ at a concentration level of 50 μg L−1 for both techniques was <6%. The effect of different interferent species was also studied. The proposed method has been applied for the determination of trace amount of Al3+ in DS samples with satisfactory results. The concentration of Al3+ in DS was observed in the range of 15.2–43.8 μg L−1, which is above the permissible limit.
Introduction
Aluminium (Al3+) is a nonessential, toxic metal to which humans are frequently exposed.1 The potentially deleterious health effects of Al3+ have been linked to the pathogenesis of a number of neurodegenerative disorders such as Parkinson's disease2 and Alzheimer's disease.3 The serious toxic effects of Al3+ was observed on chronic renal failure patients, maintained on regular hemodialysis and in those patients receiving long-term parenteral nutrition.4 It is reported in literature that Al3+ can enhance the iron-mediated lipid per-oxidation process through which Alzheimer's disease is initiated5 both by interacting with lipid membrane conformation6 and by competing with Fe3+ for citrate in the cerebrospinal fluid.7 The dialysis fluids are prepared from dialysis concentrates that are mixed with pure water. If Al3+ is present as a contaminant in these fluids, it is able to diffuse through the dialysis membranes and penetrate into the blood stream of the patient. The contamination levels in these cases depend strongly on the quality of water and the dialysis concentrates used in the dialysis fluid preparation. The official pharmacopeias8 require an accurate control of the trace levels of Al3+ in commercial dialysis solutions, which must be lower than 10 μg L−1. Many research publications are dealing with the determination of unwanted elements like Al3+9,10 and heavy metals11 in different pharmaceutical formulations. The complex matrix with high levels of salts makes dialysis concentrates difficult to analyzed.12 Hence, a simple and sensitive method is a primary importance for the effective monitoring of Al3+ present as an contaminant in dialysis concentrates.13
There are a variety of analytical techniques such as, atomic absorption spectrometry,14 inductively coupled plasma-atomic emission spectrometry and spectrofluorometry, which are used for the determination of Al3+ in environmental and biological samples.15 However, all of the techniques require enrichment methods for the determination of trace amounts of Al3+.16 Due to the presence of interfering cations such as iron, chromium, copper and zinc in dialysis concentrate solutions (DS) or the presence of Al3+ below the detection limit, it becomes difficult for accurate determination of Al3+ by spectroscopic techniques.17,18 So, the separation and preconcentration steps for Al3+ are necessary for applying simple and less expensive techniques such as spectrofluorometry and flame atomic absorption spectrometry.19 The widely used techniques for the separation and preconcentration of Al3+ include liquid–liquid extraction,10 ion exchange,20 solid-phase extraction21 and cloud point extraction.22 The cloud point extraction (CPE) methodology has been used for the extraction and preconcentration of metal ions, after the formation of sparingly water-soluble complexes, as an initial step for their determination by FAAS.20,21,23–26 CPE is offered as the most frequently used extraction methodology that is simple, cheap, efficient and less toxic than other extraction methods.27 In addition, the cloud point strategies can enlarge the FAAS applications, because depending on preconcentration factors obtained; its sensitivity can significantly be increased, thus making the method more advantageous when compared with those based on direct determination using ET AAS or ICP techniques.28 The complexing reagent 8-hydroxyquinoline (8-HQ) has been acknowledged as one of the most sensitive organic ligands used for the determination of Al3+.29–31
The aim of the present study was to establish a solid phase extraction of interfering cations for the separation of trace quantities of Al3+ in DS by using 2-methyl-8-hydroxyquinoline (2-MHQ). While for enrichment of separated trace quantity of Al3+ in DS, a cloud point extraction was used to make a hydrophobic complex of Al3+ with 8-HQ, with the resulting complex entrapped by Triton X-114 prior to its determination by spectrofluorimetry (SPF) and flame atomic absorption spectrometry (FAAS) as a comparative technique. Several experimental variables affecting the method sensitivity and stability were investigated in detail. The proposed simple and cost effective methods have been applied for the determination of trace amount of Al3+ in dialysate concentrates with satisfactory results.
Experimental
Instrumentation
The pH was measured by a 720-pH meter. A WIROWKA Laboratoryjna type WE-1, nr-6933 centrifuge (Mechanika Phecyzyjna, Poland) was used for centrifugation. Fluorescence measurement of the Al-8-HQ complex was made on a Shimadzu RF-5301 PC spectrofluorophotometer equipped with a 150 W Xenon lamp and using 1.00 cm quartz cells. A double beam Perkin-Elmer model A Analyst 700 atomic absorption spectrometer (Norwalk, CT, USA), equipped with burner was also used for comparative purposes. The single element hollow cathode lamp (Perkin Elmer) was used as energy source at wavelength (nm) 309.3, slit width (nm) 1.3, lamp current (10 mA), burner height (12.5 mm), fuel (acetylene 0.45 kg cm−1) and oxidant (nitrous oxide as oxidant = 1.6 kg cm−2).
Reagents
Ultrapure water obtained from ELGA labwater system (Bucks, UK), was used throughout the work. The non-ionic surfactant Triton X-114 was obtained from Sigma (St. Louis, MO, USA) and was used without further purification. Certified stock standard solution of Al3+ at a concentration of 1000 μg L−1 was obtained from Fluka (Bush, Switzerland). Working standard solutions were obtained by appropriate dilution of the stock standard solutions before analysis. Concentrated nitric acid, hydrochloric acid were of analytical reagent grade obtained from Merck (Darmstadt, Germany) and were checked for possible trace Al3+ contamination by preparing blanks for each procedure. The 2-methyl-8-hydroxyquinoline (2-MHQ) and 8-hydroxyquinoline (8-HQ) (Merck) were prepared (0.1 M L−1) by dissolving appropriate amount of these reagents in 10 ml ethanol (Merck) and diluting to 100 ml with 0.01 mol L−1 acetic acid and was kept in a refrigerator (4 °C) for one week. The chelating solutions were further diluted according to required concentrations. Acetate buffer solutions (CH3COO−/CH3COOH) were prepared by mixing appropriate volumes of 1 mol L−1 acetic acid and 1 mol L−1 sodium acetate solutions. The pH of the samples was adjusted to the desired pH (4–8) by the addition of 0.1 mol L−1 HCl/NaOH solution in acetate buffer.
In low-level Al3+ analysis, contamination is a serious problem. Hence, to avoid contamination, only polyethylene materials were used. Calibrated flasks were immersed for 24 h in a 10% v/v HNO3 solution and then thoroughly rinsed with Milli-Q water before use. Pipette tips, tubes, and caps were used as supplied by the manufacturer and no contamination problems were found.
Sampling
Dialysate concentrate solutions were purchased from different pharmaceutical stores of four different batches packed on different dates (n = 5) in 2009. The same DS were also collected from urological department of civil hospital Hyderabad Pakistan. On arrival to laboratory, the DS were stored at −20 °C till further analysis.
Methods
Separation/preconcentration experimental conditions and optimum values of each parameter are shown in Table 1. For separation of Al3+ ions from different interfering cations present in matrices of DS, a glass column with an inner diameter of 20 mm and a length of 25 cm was filled up to a height of about 20 cm with activated silica gel (heated in an electric oven at 100 °C prior to use), the column was preconditioned with acetate buffer solution at pH 6, and passed 10 ml of 0.1 mol L−1 solution of 2-MHQ (prepared in 0.1 M acetic acid–ethyl alcohol). For Al3+ preconcentration, three replicate of each of standard solutions (25 mL) containing Al3+ in the range of 10–50 μg L−1), while triplicate samples of 100 ml of each DS were passed through the column to separate Al3+ from other interfering cations at a flow rate of 1.0 ml min−1. The eluents was divided into two sub-samples, taken in graduated centrifuge tubes (100 ml of each) and subjected to CPE for preconcentration of Al3+, and then subjected to FAAS and SPF, separately. The same triplicate sub-samples of DS, without removing interfering cations were taken in graduated centrifuge tubes and subjected to CPE directly for comparison purposes. After several separation experiments (n = 10), the silica column was regenerated by washing with 10 ml of 0.1 mol L−1 of HNO3, 10% ethanol in 0.1 mol L−1 of CH3COOH and deionized water and then reused for further experiments.
Table 1 Experimental conditions for SPE/CPE in the determination of aluminium in dialysate concentrate
Parameters |
Reagents |
Range |
Optimum value |
Complexing reagent for SPE |
2-Methyl-8-hydroxyquinoline |
0.1 mol L−1 |
|
Complexing reagent for CPE |
8-Hydroxyquinoline (8-HQ) |
1.37 × 10−5 to 10.9 × 10−5 (0.1–0.8 ml of 1.37 × 10−3 mol L−1) |
6.85 × 10−5 mol L−1 |
Non-ionic solvent |
Triton X-114 |
0.05–0.2% (v/v) |
0.1% (v/v) |
pH |
Acetate buffer (0.01 mol L−1) with HNO3 |
4–8 |
5.5 |
Exposure time |
Ultrasonic bath |
10–30 min |
20 min |
Temperature |
— |
30–80 °C |
40 °C |
For Al3+ preconcentration, six replicate samples of each standard and DS samples were treated with quinaldine to remove interfering species were simultaneously preconcentrated by CPE. The same sub-samples of standards and DS without removing interfering cations were taken in graduated centrifuge tubes and subjected to preconcentration for comparison purposes, treated with 0.2–1.0 ml 8-HQ solution (6.90 × 10−3 mol L−1), 2 ml of Triton X-114 (0.05–0.2% v/v), 2 ml of acetate buffer, and adjusted to a pH range of 4–8 with 0.1 mol L−1 of each HCl/NaOH. The tubes were kept in an ultrasonic water bath at 40–80 °C for 10–30 min. Thus, after different time intervals, the phase separation was accelerated by centrifuging for 5 min at 3500 rpm. After cooling in an ice bath, the surfactant-rich phase became viscous and the upper aqueous phase was decanted.
To decrease the viscosity of one of the duplicate extracts 0.5 ml of ethanol–water mixture was added and the fluorescence intensity of the Al3+-8-HQ complex in the micellar phase was measured by SPF against a corresponding reagent blank at λexitation 370 ± 3 nm and λemmision 510 ± 3 nm. While the viscosity of the other subsample was decreased by adding acidic ethyl alcohol (0.1 mol L−1 HNO3) and introduced into flame by conventional aspiration. Calibration was performed against aqueous standards submitted to the same CPE procedure as described above. A blank submitted to the same procedure was measured parallel to the calibration solutions of standards and real samples.
Results and discussion
The developed procedure was easy and rapid and did not require any particular technical skill. Compared to other publications in the literature, most of the examined cations interfere in Al3+ determination by SPF.23 While in the present work, interfering cations were separated with a complexing reagent (2-MHQ), then the separated Al3+ was complexed with 8-HQ to form a hydrophobic complex (Al3+-8-HQ), which was subsequently trapped in the non-ionic surfactant and separated from the aqueous phase. The separation of matrix cations reduces the interference in determination of fluorescence intensity of Al3+-8-HQ complex by SPF. After removal of different interfering metal ions (Zn, Cu, Fe, Ni) the high concentration of alkali metals salts may not influence the speed of the Al3+-8-HQ chelation reaction and the fluorescence intensity obtained from known amount of added Al standards at the three concentration levels. In ethanolic–aqueous solution the fluorescent intensity of Al3+-8-HQ remained unchanged for 48 h. To check the accuracy of the SPE and CPE, the results obtained by SPF were compared with those obtained by FAAS. It was reported in the literature that determination of trace quantity of Al3+ in biological fluids is generally performed by GF-AAS, probably due to the complicated matrix of these samples, making it difficult to determined Al by a fluorimetric method.32
Optimization of SPE
The interference of different cations was eliminated by using 2-MHQ, which forms chelates with most of the metal ions except Al3+, which was not complexed with 2-MHQ due to stearic hindrance of the methyl group at the 2-position of the aromatic ring.33 The 2-MHQ solution sorbed on activated silica used for SPE of interfering cations, at pH range 4–8 were adjusted by buffer solution with either 0.1 mol L−1 nitric acid or NaOH of standards and real samples solutions. It was found that the maximum retention of interfering cations were achieved at a pH range of 5.0–6.0 and begins to decrease when the solution is over pH 6.5. Therefore, pH 5.5 was used for separation of Al3+ from interfering cations. The separation of Al was >95% at an optimum flow rate of the sample solution up to 1.0 ml min−1. However, at a flow rate above 2 ml min−1, there was a decrease in the sorption percentage of interfering cations, because ligand formation between interfering cations and 2-MHQ is slow at room temperature.33
Optimization of CPE
Effects of pH.
Acetate buffer was preferred as pH stabilizer because it showed fairly low levels of Al3+ contamination. Extraction yield depends on the pH at which complex formation is carried out. The CPE of Al3+ was performed at different pH. The six replicate standard solutions of Al3+ (50 μg L−1) were adjusted to the different pH with the acetate buffer solutions with the help of 0.1 mol L−1 HNO3/NaOH, covering the pH range of (pH 4–8). Fig. 1 shows the effect of pH on the extraction recovery of Al3+, which was calculated based on the amounts of analyte in the surfactant rich solution after extraction at the starting pH 4. It can be seen that quantitative extraction was obtained for Al3+ in the pH range of 4.5–6 and starts to decrease after pH 7.0. Hence, pH 5.5 was chosen for CPE of Al3+ in DS.
 |
| Fig. 1 Effect of pH on the % recovery of Al3+: 50 μg L−1 Al, 6.85 × 10−5 mol L−1 8-HQ, 0.1% (w/v) Triton X-114, Temp. 40 °C, stirring time 20 min. | |
Effect of 8-HQ concentration.
The effect of concentration of the chelating agent on the analytical responses was examined and the results are shown in Fig. 2. The standards, real samples with and without standard additions were treated with 8-HQ solutions in the range of 1.37 × 10−5 to 10.9 × 10−5 (0.1–0.8 ml of 1.37 × 10−3 mol L−1) and appropriate buffer solution was added to keep pH 5.5. The complex was entrapped in 0.1% (w/v) Triton X-114 to preconcentrate traces of Al3+ in understudy samples. Major improvement in the fluorescence intensity was obtained as the chelating agent concentration increased up to 6.85 × 10−5 mol L−1 of 8-HQ, which is sufficient for total complexation and extraction. The concentrations above this value have no significant effect on the fluorescence intensity. The stoichiometry of the Al3+-8-HQ ternary complex, as reported in the literature is 1
:
3 ratios.34
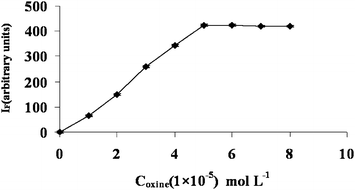 |
| Fig. 2 Effect of oxine concentration on the spectrofluorimetric response: 50 μg L−1 Al, 0.1% (w/v) Triton X-114, pH 5.5, Temp 40 °C, stirring time 20 min. | |
Effect of Triton X-114 concentration.
In the present work Triton X-114 (surfactant) was chosen because of its higher extraction efficiency as well as its lower cloud point temperature as compared with other reported surfactants.20 The low cloud point temperature avoids back extraction during centrifugation. Fig. 3 shows the variation in fluorescence intensity of Al-8-HQ complex within the concentration range of Triton X-114 (0.05–0.20%). 60–70% recovery was observed at 0.05% of Triton X-114, while the fluorescence intensity reaches a maximum at the concentration of 0.10%. So, a concentration of 0.10% was chosen as the optimum surfactant concentration in order to achieve the highest possible extraction recovery of Al3+ from standards, and real samples, while <0.10% (w/v), the extraction efficiency of the complexes is low probably because of the inadequacy of the assemblies to entrap the hydrophobic complex quantitatively. At concentrations >0.10% the signals decrease because of increment in the volumes and viscosity of the surfactant phase (ethanol–water for SPF and ethanol–0.1 mol L−1 nitric acid for FAAS) to reducing the viscosity of the surfactant.
 |
| Fig. 3 Effect of Triton X-114 on the spectrofluorimetric response: 50 μg L−1 Al, 6.85 × 10−5 mol L−1 8-HQ, pH 5.5, Temp. 40 °C, stirring time 20 min. | |
Effect of temperature and time.
A sufficiently long reaction time was allowed for the Al3+-8-HQ complex formation to precede up to 300 min at room temperature.20 While subjecting to heating in ultrasonic water bath in the range of 10–60 min, the formation of complex was optimum at 20 min. We preferred to stop the reaction at 60 min, as a longer reaction time did not increase the fluorescence intensity and % recovery of Al3+.
The effects of temperature on the analytical signal were studied in the range of 30–60 °C. It was observed that at 40 °C, maximum extraction efficiency was obtained, while at higher temperature the fluorescent intensity of Al3+-8-HQ complex was lowered.
Interferences
The interference are those relating to the preconcentration step, i.e. cations which may react with 8-HQ or species that may react with Al3+ and decrease the extraction efficiency as well as effecting the determination of fluorescence intensity of the Al3+-8-HQ complex, using the SPF technique.35 To perform this study, 25 ml solution containing 50 μg L−1 of Al3+ and different ions at three levels (interfering ions to analyte ratios), were subjected to CPE procedure. The determination of Al3+ in the presence of Zn2+ produce positive error and a broad band in emission spectrum was observed, while Fe3+, Cd2+, Pb2+ and Cu2+ can interfere with the determination of Al3+, to produce negative error effects. The interference from these ions can be eliminated by using 2 methyl 8-hydroxyquinoline (2-MHQ) that can form chelates with these ions. Our results are not consistent with other studies to determine simultaneously Al3+ and Zn2+ using oxine as chelator.21 It was reported earlier that Al3+ was not complexed with 2-MHQ due to stearic hindrance of the methyl group at the 2 -position of the aromatic ring.33 The silica gel is an active adsorbent and also plays a role as the solid material coated with complexing reagent 2-MHQ solution in ethanolic acetic acid. In addition, other advantages of silica gel are local availability, high surface area and high thermal resistance.36,37
Characteristics of the method
The calibration graph using the preconcentration system for Al3+ was linear at 10–50 μg L−1 with a correlation coefficient (r) of 0.9997. Due to unavailability of certified reference material of the same matrix in our laboratory, the validity and accuracy of the proposed methods were checked by the standard addition method by adding certified standards at three concentration levels in replicate sub samples of a DS. The Student's t-test showed that the % recovery of Al3+ obtained with and without pre-separation of interference cations using SPF was significantly different at 95% confidence level. While the differences were not significant in those results obtained by FAAS. The relative standard deviation for six replicates containing 50 μg L−1 of Al3+ without pre-separations of interference ions were observed in the range of (7.3–9.2%) and (4.2–6.0%) for SPF and FAAS, respectively. While after pre-separations of Al+3 from interference ions, the % relative errors were found to be <6% for SPF but no significant difference was observed for FAAS (Table 2). The method's precision (expressed as coefficient of variation, CV %), determined by analysis of nine aliquots of a DS (DS3), was <6% for both techniques (Table 2). The preconcentration factor (PCF), which is defined as the concentration ratio of analyte in the final diluted surfactant-rich extract ready for its determination and in the initial solution. After optimization of experimental conditions, PCF of 200 was achieved with % recoveries of >98% for Al3+ in DS. The PCF achieved in this work was higher than those obtained in other CPE methods used for extraction and preconcentration of Al3+.21,23
Table 2 Validation of cloud point extraction of Al3+ in dialysate concentrate after (AS) and before (BS) pre-separation of interference ionsd
Added Al3+/μg L−1 |
FAAS |
SPF |
AS |
BS |
Paired t-testctExperimental |
AS |
BS |
Paired t-testctExperimental |
Average value ± confidence interval (P = 0.05).
() Values in parenthesis % recovery.
Paired t-test between MD vs. CD, degree of freedom (n − 1) = 5.
t
Critical at 95% confidence limit = 2.57.
|
0.0 |
14.7 ± 0.876a |
14.0 ± 1.02 (97.2) |
0.224 |
15.2 ± 0.892 |
14.2 ± 1.05 (93.4) |
0.143 |
10.0 |
24.4 ± 1.12 (98.8)b |
23.7 ± 1.43 (97.1) |
0.312 |
24.9 ± 1.47 (98.8) |
23.1 ± 2.13 (92.7) |
0.156 |
25.0 |
39.3 ± 1.45 (99) |
38.3 ± 1.74 (97.4) |
0.382 |
39.3 ± 2.1 (98.7) |
36.3 ± 3.12 (92.4) |
0.121 |
50.0 |
64.0 ± 2.84 (98.9) |
62.4 ± 3.12 (97.5) |
0.387 |
64.2 ± 3.14 (98.4) |
59.0 ± 4.51(91.9) |
0.132 |
The limit of detection (LOD) calculated (0.384 μg L−1) as three times the standard deviation of the blank signals. The LODs were in same range (0.4 μg L−1), to that obtained with the fluorimetric extractive procedure with mordant dyestuff,38 but better than for the fluorimetric extractive procedure using the same chelating reagent, 8-HQ.31 A flow injection spectrophotometric determination based on the reaction of Al3+ with eriochrome cyanine R associated with cetyltrimethyl-ammonium bromide obtained LOD (3.24 μg L−1),39 and application of electrothermal atomic absorption spectrometry (ETAAS) after preconcentration on micro columns filled with Chelex 100 resin (0.5 μg L−1).7 The obtained LOD was sufficiently low as to be valuable for detecting low levels of Al3+ in different DS.
Application
The optimized separation/preconcentration steps were carried out on DS samples without any previous treatment. Separation of Al3+ from other interference cations by 2-MHQ, while chelating of separated Al3+ with 8-HQ, which was entrapped in non-ionic surfactant Triton X-114 and analysed by two analytical techniques SPF and FAAS. The concentration of Al3+ in different dialysates were found in the range of 15.2–43.8 μg L−1 (Table 3), which were above the maximal acceptable levels according to the official legislative recommendations (<10 μg L−1). In understudy samples, the commonly encountered matrix components such as alkali and alkaline earth elements generally do not form stable complexes with understudy reagents and are not extracted into the surfactant rich phase. Even though iron normally present in DS at trace levels, was removed by complexation with 2-MHQ. Its accessibility, ease of use, and low costs make this technique quite attractive, as it provides a procedure that is simple, robust, and readily reproducible in clinical and pharmaceutical laboratories. Chronic kidney disease is an important cause of morbidity and mortality all over the world. Renal replacement therapy or dialysis in an individual with renal failure is an important step in medical science.40 Control of the Al3+ in dialysis concentrates is one of the most important factors in prevention of diseases related to elevated levels of Al3+ in patients with kidney disorders that are being cured by dialysis processes.41
Dialysate samples |
FAAS |
SPF |
Na+ 145 meq L−1; K+ 1.3 meq L−1; Ca2+ 2.7 meq L−1; Cl− 149.5 meq L−1.
Na+ 145 meq L−1; K+ 1.6 meq L−1; Ca2+ 3 meq L−1; Cl− 125 meq L−1.
Na+ 140 meq L−1; K+ 2.6 meq L−1; Ca2+ 5 meq L−1; Mg2+ 3 meq L−1, Cl− 103 meq L−1.
Na+ 142 meq L−1; K+ 1.6 meq L−1; Ca2+ 4 meq L−1; Cl− 102 meq L−1; Mg2+ 1.5 meq L−1.
Na+ 145 meq L−1; K+ 1.45 meq L−1, acetate and citrate 55 meq L−1.
|
DS1 |
27.8 ± 2.75 |
28.2 ± 3.12 |
DS2 |
37.7 ± 3.12 |
38.2 ± 3.14 |
DS3 |
14.7 ± 1.85 |
15.2 ± 2.17 |
DS4 |
18.8 ± 2.35 |
19.2 ± 2.47 |
DS5 |
43.3 ± 3.32 |
43.8 ± 4.32 |
Conclusion
The proposed methods could be used for routine analysis in clinical laboratories for accurate determination of Al3+ in aqueous or biological fluids. In this work, the use of micelle systems as a separation and preconcentration for Al3+ offers several advantages including low cost, safety, preconcentration of Al3+ with high recoveries and very good extraction efficiency. The surfactant rich phase can be easily subjected to FAAS and SPF after dilution with ethanol–water and acidic ethanol (0.1 mol L−1 HNO3). The proposed method can be applied to the determination of trace amounts of Al3+ in various pharmaceutical formulations.
References
- G. L. Klein, Curr. Opin. Pharmacol., 2005, 5, 637–640 CrossRef CAS.
- M. J. D. Cassidy, Medicine, 2003, 31, 56–61 CrossRef.
- M. L. Hegde, P. Shanmugavelu, B. Vengamma, T. S. S. Rao, R. B. Menon, R. V. Rao and K. S. J. Rao, J. Trace Elem. Med. Biol., 2004, 18, 163–171 CrossRef CAS.
- V. Rondeau, D. Commenges, H. Jacquin-Gadda and J. F. Dartigues, Am. J. Epidemiol., 2000, 152, 59–66 CrossRef CAS.
- O. I. Egbuna and A. Bose, J. Nephrol., 2005, 2, 1–8 Search PubMed.
-
K. Berend, G. B. van der Voet and F. A. de Wolff, in Group 13 Chemistry II—Biological Aspects of Aluminium, ed. D. M. P. Mingos, Springer, Berlin, 2002, vol. 104, pp. 1–58 Search PubMed.
- G. Berthon and N. Dousset, Redox Report, 1996, 2, 412.
- N. Dousset, G. Ferretti, T. Galeazzi, M. Taus, V. Gouaz, G. Berthon and G. Curatola, Free Radical Res., 1997, 27, 291–302 CrossRef CAS.
-
European Pharmacopeia, Council of Europe, Strasbourg, 1997, pp. 1540–1542 Search PubMed.
- J. Komarek, R. C. Ervenka, T. Ruzicka and V. Kuban, J. Pharm. Biomed. Anal., 2007, 45, 504–509 CrossRef CAS.
- S. Beniz Guenduez, K. Semahat and A. Orhan, Spectrochim. Acta, Part A, 2005, 61, 913–921 CrossRef.
- P. C. Do Nascimento, D. Bohrer, L. De Carvalho, C. Caon, E. Pilau, Z. Vendrame and R. Stefanello, Talanta, 2005, 65, 954–959 CrossRef.
- S. Knezevic, R. Milacic and M. Veber, Fresenius J. Anal. Chem., 1998, 362, 162–166 CrossRef CAS.
- L. M. Carvalho, P. C. Nascimento, D. Bohrer, R. Stefanello and D. Bertagnolli, Anal. Chim. Acta, 2005, 546, 79–84 CrossRef CAS.
- L. Zhu, K. Li, S. Zhou, Q. Hu, G. Yang, Z. Huang and J. Chen, Asian J. Chem., 2008, 20, 4433–4442 CAS.
- B. Bocca, A. Alimonti, F. Petrucci, N. Violante, G. Sancesario, G. Forte and O. Senofonte, Spectrochim. Acta, Part B, 2004, 59, 559–566 CrossRef.
- S. Beniz Gunduz, S. Kucukkolbasy, O. Atakol and E. Kylyc, Spectrochim. Acta, Part A, 2005, 61, 913–921 CrossRef.
- C. Brach-Papa, B. Coulomb, C. Branger, A. Margaillan, F. Theraulaz, P. Van Loot and J. L. Boudenne, Anal. Bioanal. Chem., 2004, 378, 1652–1658 CrossRef CAS.
- E. K. Paleologos, C. D. Stalikas, S. M. Tzouwara-Karayanni, G. A. Pilidis and M. I. Karayannis, J. Anal. At. Spectrom., 2000, 15, 287–292 RSC.
- S. B. Erdemoglu, K. Pyrzyniska and S. Gucer, Anal. Chim. Acta, 2000, 411, 81–89 CrossRef CAS.
- M. B. Luo and S. P. Bi, J. Inorg. Biochem., 2003, 97, 173–178 CrossRef CAS.
- A. B. Tabrizi, Food Chem., 2007, 100, 1698–1703 CrossRef.
- S. Rubio and D. Perez-Bendito, TrAC, Trends Anal. Chem., 2003, 22, 470–485 CrossRef CAS.
- E. K. Paleologos, C. D. Stalikas and M. I. Karayannis, Analyst, 2001, 126, 389–393 RSC.
- Z. M. Sun, P. Liang, Q. Ding and J. Cao, J. Hazard. Mater., 2006, 137, 943–946 CrossRef CAS.
- M. Ghaedi, A. Shokrollahi, F. Ahmadi, H. R. Rajabi and M. Soylak, J. Hazard. Mater., 2008, 150, 533–540 CrossRef CAS.
- J. Li, P. Liang, T. Q. Shi and H. B. Lu, At. Spectrosc., 2003, 24, 69–172.
- J. L. Manzoori and A. B. Tabrizi, Anal. Chim. Acta, 2002, 470, 215–221 CrossRef CAS.
- F. Papadimitrakopoulos, X. M. Zhang and K. A. Higginson, IEEE J. Sel. Top. Quantum Electron., 1998, 4, 49–57 CrossRef CAS.
- R. H. Zhu and W. T. Kok, Anal. Chim. Acta, 1998, 371, 269–277 CrossRef CAS.
- A. Alonso, M. J. Almendral, M. J. Porras, Y. Curto and C. Garcia de Maria, Anal. Chim. Acta, 2001, 447, 211–217 CrossRef CAS.
- M. Buratti, C. Valla, O. Pellegrino, F. M. Rubino and A. Colombi, Anal. Biochem., 2006, 353, 63–68 CrossRef CAS.
- A. Ohashi, A. Tsuguchi, H. Imura and K. Ohashi, Synergistic cloud point extraction behaviour of Al (111) with 2-methyl-8-quinolinol and 3,5-Dichlorophenol, Anal. Sci., 2004, 20, 1091–1093 CAS.
- S. Bi, X. Yang, F. Zhang, X. Wang and G. Zou, Fresenius J. Anal. Chem., 2001, 370, 984–996 CrossRef CAS.
- A. Ohashi, A. Tsuguchi, H. Imura and K. Ohashi, Anal. Sci., 2004, 20, 1091–1093 CAS.
- H. Irving, E. J. Butler and M. F. Ring, J. Chem. Soc., 1949, 1489–1498 RSC.
- P. K. Jal, S. Patel and B. K. Mishra, Talanta, 2004, 62, 1005–1028 CrossRef CAS.
- K. Sharma, S. Mittal and M. Koel, Crit. Rev. Anal. Chem., 2003, 33, 183–198 CrossRef CAS.
- M. A. Raggi, C. Sabbioni and G. Cantelli Forti, J. Pharm. Biomed. Anal., 2000, 21, 1191–1196 CrossRef CAS.
- J. L. Rodrigues, C. S. Magalhaes and P. O. Luccas, J. Pharm. Biomed. Anal., 2005, 36, 1119–1123 CrossRef CAS.
- T. G. Kazi, N. Jalbani, N. Kazi, M. K. Jamali, M. B. Arain, H. I. Afridi, A. Kandhro and Z. Pirzado, Renal Failure, 2008, 30, 737–745 CrossRef CAS.
|
This journal is © The Royal Society of Chemistry 2010 |
Click here to see how this site uses Cookies. View our privacy policy here.