DOI:
10.1039/B714600K
(Emerging Area)
Org. Biomol. Chem., 2008,
6, 19-25
Received
21st September 2007
, Accepted 24th September 2007
First published on 5th November 2007
Abstract
Impregnation of nickel(II) onto graphite, upon reduction in situ, leads to a reagent that catalyzes both C–H and C–C bond formations between aryl halides, or aryl pseudo-halides, and various organometallics. Cross-couplings, most notably with tosylates, can lead to either reduced aromatics, biaryls, or styrenes as products under the influence of Ni/Cg. The catalyst is inexpensive, non-pyrophoric, and reusable.
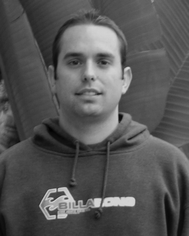 Tom Butler | Tom Butler received his B.S. degree at Cal State, Chico under the direction of Prof. David B. Ball. He arrived at UCSB in late 2004 and joined the research group of Prof. Bruce Lipshutz, where early work on nickel-on-graphite had begun. After completing this study on its use for heterogeneous reductions of aryl sulfonates, he spent considerable time and effort developing new technology for controlling regiochemistry in Negishi carboaluminations, and a process for applying this chemistry towards the industrial production of coenzyme Q10. Most recently, he has completed his studies on Ni/Cg as a catalyst for carbon–carbon bond formations and is employed at Gilead. |
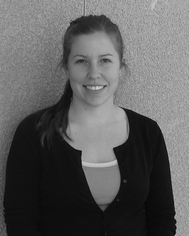 Elizabeth Swift | Liz Swift is in her senior year at UCSB, working towards her bachelor's degree in organic chemistry. As a member of the Lipshutz group since the summer of 2006, she has been involved with various projects in organometallic chemistry looking forward to graduate school in organic chemistry. |
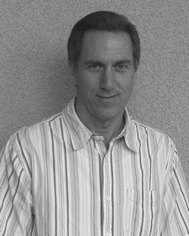 Bruce Lipshutz | Bruce Lipshutz has been at UC Santa Barbara since beginning his professorial career in 1979, after extensive training by three phenomenal instructors: Howard Alper (B.A.; SUNY Binghamton); Harry Wasserman (Ph.D.; Yale), and postdoctoral mentor E. J. Corey (Harvard). Much of his career has been devoted to developing reagents of general use for the synthetic community (e.g., SEM-Cl, “Higher Order Cuprates”, “Cuprate-in-a-Bottle”, DCAD, “Copper Hydride-in-a-Bottle”, Ni/C, etc.). The group’s efforts have now turned to a mix of heterogeneous catalysis, including development of metal-supported cross-coupling reagents, and homogeneous catalysis, including several ‘name’ reactions in water. A number of projects in total synthesis involving axially chiral biaryls and chemistry associated with coenzyme Q10 are also well underway. |
Introduction
“Make it cheaper, faster, and better.” We hear it all the time. What industrial process chemist doesn't look for ways to get all three in the lab? Even a single improvement in a process could make a huge difference in the lifetime of a compound making its way to market. One approach that may have merit, given the extraordinary popularity of organopalladium chemistry, involves finding suitable alternatives to this precious group 10 metal for highly valued cross-couplings. The ‘base’ metal among the group 10 trio (Ni, Pd, Pt) is nickel, and while most organonickel-catalyzed cross-couplings (as with Pd) are done in solution, there are other options. Initially considered was activated charcoal. The reagent derived from the combination of inexpensive nickel and charcoal (Aldrich; new catalog # 675326), namely nickel-in-charcoal (Ni/C), is easily prepared and has been shown to mediate several common ‘name’ reactions.1 Oftentimes, these heterogeneous events can be greatly accelerated by the convenient use of microwave heating.2 Although the extent of impregnation of metals (such as Ni) within a charcoal matrix benefits from ultrasonication of Ni(NO3)2 admixed with 100 mesh charcoal in water,3 the loading phenomenon can be dependent upon the intricacies of charcoal itself. That is, there are many “flavors” of charcoal; indeed, books with titles such as Active Carbon contain an enormous reservoir of science on this allotrope .4 The variety of natural sources from which activated charcoal is prepared is interesting, including as examples wood, coconut, coal, nutshells, lignite, lignin, sawdust, fruit stones, and peat. Processing (or “carbonization”) can determine pore structure and surface area, and hence catalytic activity. Although an empirically-derived “formulation” leads reproducibly to useful heterogeneous catalysts such as Ni/C5 and more recently Cu/C,6 switching to graphite (from graphein, Greek, to write) was expected to offer some advantages: (1) it is less costly than activated charcoals; (2) its thermal conductivity is far greater than that of charcoal, potentially important when used under microwave conditions; (3) it is free-flowing and thus easier to handle and control; and (4) its structural differences (Scheme 1) relative to charcoal might lead to different reactivity patterns in their corresponding metal-impregnated catalysts. While charcoal has its irregular, random pore structure brought about by cracking of carbon sheets during carbonization,7 graphite retains its ordered state in which the distance between sheets is constant (ca. 3.3 Å). Thus, charcoal can have micropores (diameters, d < 2 nm), mesopores (d = 2–50 nm), and macropores (d > 50 nm) in which cross-couplings can take place. On the other hand, graphite can accommodate metals between the sheets but the desired catalysis must occur on the surface (and hence, nickel-on-graphite).
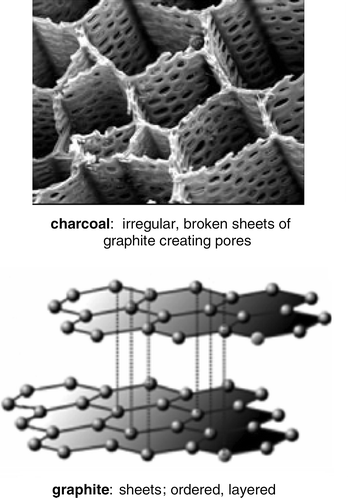 |
| Scheme 1 Comparison: charcoal vs. graphite. | |
Catalyst preparation: Ni/Cg
It's easy
…
at least in hindsight.8 Grams of graphite (Aldrich; catalog # 28,286–3) from a 1 kilo jug are mixed in water at room temperature with hydrated nickel nitrate [Ni(NO3)2·6H2O], also sold in multi-gram bottles (Aldrich; catalog # 24,407–4). Impregnation of nickel is best accomplished by ultrasonication of the heterogeneous mixture for ca. 1 hour in a standard laboratory ultrasonic bath (Scheme 2). Subsequent distillation of water presumably serves to convert the nickel present, by removal of (NO)x, to nickel(II) oxide, in which state the reagent is stored. Transmission electron microscopy (TEM ) shows both the sheets of graphite and the distribution of nickel nanoparticles (dark blobs; Scheme 3). The procedure below, carried out on a convenient scale, produces Ni/Cg in sufficient quantities for several trials as a group 10 metal catalyst. Activation by reduction to Ni(0)/Cg is done either immediately before use, or in situ, depending upon the intended chemistry (vide infra).
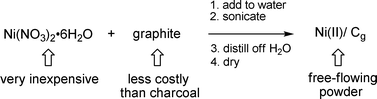 |
| Scheme 2 Impregnation of nickel(II) onto graphite. | |
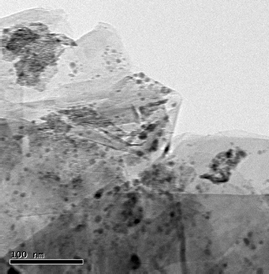 |
| Scheme 3 Bright field TEM of Ni/Cg nm size blobs. | |
The Ni(II)/Cg as prepared above is best stored under argon in a dessicator or glove box. This precaution is mainly to extend lifetime, which is on the order of months, rather than due to any safety issues that, in all likelihood, would have been observed by now after years of use in our group. Thus, unlike other metals impregnated onto graphite (e.g., K/Cg,9Cu/Cg10), Ni/Cg prepared in this fashion appears to have no pyrophoric nature. The same is true for the activated catalyst, Ni(0)/Cg, which is conveniently generated in dry THF at room temperature using n-BuLi (2 equiv. vs. Ni) for ca. 15 minutes (Scheme 4).
 |
| Scheme 4 Reduction of Ni(II)/Cg to active Ni(0)/Cg. | |
Ni/Cg-catalyzed reductions of aryl sulfonates…
…No, not triflates, or even nonaflates; the cheaper ones: tosylates and mesylates, compliments of nickel. In hindsight, it was not obvious that such reductions represented the ideal starting point for developing the chemistry of this reagent. Nonetheless, the methodology for effecting such transformations at the time was quite limited11 and this observation provided the incentive. More recently, several new procedures have appeared that offer both homogeneous alternatives, such as cat. Pd–HCOOH–Et3N–cat. PPF-P(t-Bu)212 and cat. Ni–NaBH4–Cy3P,13 and heterogeneous options, including Pd/C as catalyst.14 A heterogeneous method applicable to both tosylates and mesylates, however, based on inexpensive nickel should be competitive. Preformed Ni(0)/Cg (prepared in THF as above) and then used in dry(!) DMF under conventional conditions of heating at 120 °C (Scheme 5), catalyzes the reduction of aryl tosylates in the presence of the potassium salt of commercially available dimethylamine-borane, Me2NH·BH3 (Acros; catalog # 177310250). The combination of K2CO3 with this Lewis acid –Lewis base complex, forming a white solid of somewhat limited shelf-life, provides a highly chemoselective source of hydride for the presumed Ni(II) intermediate following oxidative addition. The caesium salt, generated using Cs2CO3 in place of K2CO3, provides a more quickly formed, more soluble (but far more expensive) alternative that works equally well. Usually, ca. 5% Ni/Cg is used relative to substrate, not to be confused with the percent loading of Ni on the solid support (which can be far different). The amount of catalyst is actually not crucial, since the metal is being used on the support, although too much catalyst may prevent stirring and hence slow the rate. Thus, there is a balance that needs be achieved, and at the loading indicated in the procedure provided (ca. 3–4%), a 5% level of catalyst allows for essentially complete conversion. Noteworthy is the overall tolerance to several functional groups, including aryl esters , amides , and ketones . Moreover, since the amide–borane is pre-formed prior to introduction of the substrate, the reaction conditions are not highly basic. Thus, peptidic educts (e.g., 1, Scheme 6) survive the time needed for full conversion, which is on the order of a day (although most substrates took 3–12 hours).
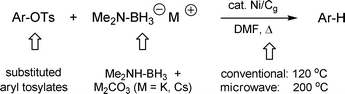 |
| Scheme 5
Ni/Cg-catalyzed reduction of aryl tosylates. | |
To add potential value to reductions with Ni/Cg, two additional features needed attention: (1) reaction times should be shortened, and (2) the catalyst should be recyclable. Thus, while conventional heating led to clean reactions, reducing the timeframe from several hours to minutes seemed like a worthwhile goal. By carrying out these reductions in a microwave instrument at elevated temperatures (ca. 200 °C) under otherwise identical conditions, reaction times were dramatically lowered (e.g., 1 to 2: conventional 29 h, µW 45 min; Scheme 6). A similar pattern of susceptibility to Ni/Cg-mediated reduction was observed for mesylates. Using tosylated quinoline 3 to arrive at product 4 as a test case for recycling of the catalyst, virtually identical results were obtained after a second use (Scheme 7). Also, particularly intriguing was the unexpected result with substrate 3 using Ni/C, realized under otherwise identical conventional conditions (DMF, 120 °C) but run overnight: no reaction. A hint that Ni/C ≠ Ni/Cg (vide infra).
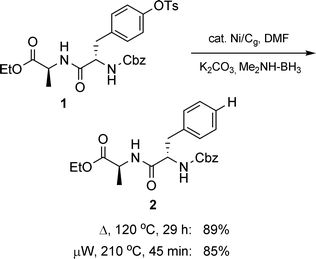 |
| Scheme 6 Microwave-assisted reduction of a tyrosine tosylate. | |
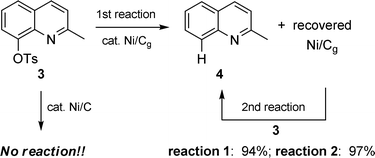 |
| Scheme 7 Recycling of Ni/Cg; comparison with Ni/C. | |
Cross-couplings catalyzed by Ni/Cg
Suzuki couplings
Prior success using Ni/C15 suggested that Ni/Cg should also mediate biaryl bond formation between ArB(OH)2 and aryl halides (including chlorides). In light of the reductions of tosylates and mesylates catalyzed by Ni/Cg (vide supra),8 perhaps these pseudo-halides are viable alternatives? If both halides (especially chlorides) and tosylates are useful in such couplings, then several aspects of the chemistry look economically attractive: substrates (Ar–Cl/OTs), catalyst (heterogeneous Ni), ligand (Ph3P), solvent (dioxane), reaction times (shortened by microwave assistance?).
Halides were tested first to gauge the relative catalytic properties of nickel-in-charcoal versusnickel-on-graphite. Ni(0)/Cg was formed in the usual way, by prior reduction of Ni(II)/Cg with n-BuLi. Under conventional heating conditions, the trend was clear: in all cases Ni/Cg gave either similar or better results (meaning cleaner, higher yielding reactions) relative to nickel-in-charcoal. Thus, for deactivated p-chloroanisole5, coupling with boronic acid 6 gave rise to 7 in roughly the same yields and reaction times, although Ni/Cg was slightly favored with respect to both parameters (Scheme 8). The o-chloro-analog 8, however, revealed a greater difference, with none of the corresponding biaryl being observed in the Ni/C-catalyzed reaction with phenylboronic acid9, while Ni/Cg led to a good yield of product 10.
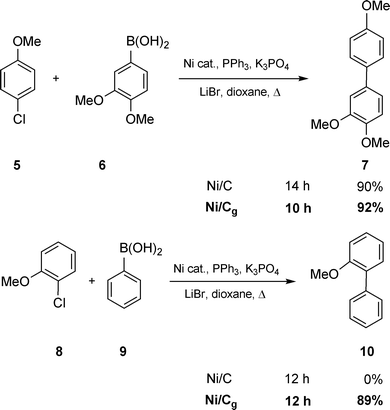 |
| Scheme 8
Ni/Cgvs.Ni/C as catalysts in Suzuki couplings. | |
Aryl tosylates were studied next. Apparently, biaryl bond formations involving tosylates that rely on heterogeneous nickel of any form are unprecedented. Nonetheless, a variety of this substrate type along with boronic acid partners (2 equivalents vs. sulfonate) could be coupled in good yields. Reactions under conventional conditions of heating were run in refluxing dioxane at ca. 0.2 M in tosylate using excess K3PO4 as the base, with the Ni/Cg pre-reduced with n-BuLi. Done in this fashion, however, reactions can take several hours or even days to reach completion. On the other hand, increasing the temperature, conveniently done in a microwave instrument at 180–200 °C, greatly accelerates the process leading to completion in 1.5–5 hours. Under microwave irradiation, lower molecular weight bases/additives (e.g., LiOH and/or KF) can be used, allowing for more efficient stirring. Employing these modified conditions, the graphite-based catalyst led to a better yield, e.g., of biaryl 12 from precursors 6 and 11 (Scheme 9), although only ca. half the time was needed for reaction relative to that using Ni/C. An additional representative example involving tosylate 13 is illustrated in Scheme 10, highlighting the deactivated nature of the boronic acid 14 en route to 15.
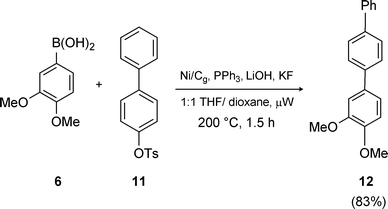 |
| Scheme 9 Suzuki couplings using µW assistance: effect of temperature. | |
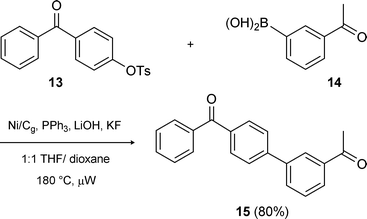 |
| Scheme 10 Another example of Ni/Cg-catalyzed coupling of an aryl tosylate. | |
Noteworthy is the finding that homocoupling is minimal in these reactions. Curiously, these couplings are also considerably less sensitive to hydrolysis of the sulfonate back to phenols by adventitious water (perhaps more readily retained within the charcoal matrix). Although roughly twice the more commonly used amount of Ni/Cg (ca. 8% relative to substrate) is required for net tosylate displacement, the virtues of this heterogeneous catalyst should far outweigh the usual goal of achieving high turnover numbers . Thus, given a recyclable, heterogeneous nickel catalyst, this is a case where “more is better than less”: who cares whether a reaction takes 8% Ni or 4% Ni under such circumstances?
Negishi couplings of vinylzirconocenes17
Another comparison between Ni/Cg and Ni/C came in the form of cross-couplings between vinylic zirconocenes , derived from terminal alkynes, and aryl halides. Previously, it had been shown that such intermediates, in fact, are unexpectedly robust upon heating to 200 °C under microwave irradiation in the presence of an aryl halide and Ni/C, leading to stereodefined styrenes in good yields.15 Perhaps not unexpectedly, pre-activated Ni(0)/Cg, likewise, produced clean E-β-substituted styrenes, typified by the conversion of octyne16 to product 19, R = n-C6H13 upon exposure to bromide 18 (83%; Scheme 11). By way of comparison, related acetylene 17 led to 19 (R = (CH2)4OTIPS) in comparable yield under identical conditions using Ni/C. In these sequential hydrozirconation–cross-couplings, THF can be used for both steps and the catalysis is complete within 15 minutes at 200 °C. As is oftentimes the case in hydrozirconation chemistry, the quality of the Schwartz reagent and handling of the derived intermediate zirconocenes can be crucial in subsequent group 10 metal-catalyzed C–C bond formation. Care should be taken to exclude moisture, not only given the sensitivity of C–Zr bonds but also to avoid hydrolysis of the starting tosylates back to the corresponding phenols , which has been observed if the solvent (THF) is wet. The conclusion here is that there is no obvious benefit to graphite over charcoal, other than the fundamental differences noted earlier.
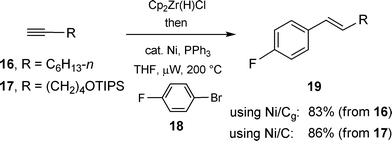 |
| Scheme 11 Hydrozirconation–Ni-catalyzed couplings. | |
Carboalumination-coupling
Vinylalanes are viable coupling partners with various sp2-based substrates, using Pd(0) catalysis. On the other hand, use of catalytic Ni(0) to effect the equivalent process is not common and, to the best of our knowledge, cross-couplings of vinylalanes with aryl tosylates have not been described, let alone with a heterogeneous source of Ni(0). While admittedly only a single example has been demonstrated to date, a modified carboalumination of 1-octyne16 was initially carried out in toluene (Scheme 12).18 Then, in the presence of a mixed Al–Zr catalyst generated from excess Me3Al and 5% Cp2ZrCl2, addition of commercial isobutylaluminoxane (IBAO), exchange of isobutyl residues into a mixed metal complex presumably occurs. This approach takes advantage of our recently discovered method for controlling the regiochemistry in Negishi carboaluminations18 using the inexpensive zirconocene Cp2ZrCl2, as opposed to an earlier solution using the bridged Brintzinger zirconocene (ebi)ZrCl2 (ebi = ethylene bis-indenyl).19 Transfer of the vinylalane intermediate 20, upon consumption of the acetylene, to a microwave vial containing Ni/Cg and an aryl tosylate (e.g., 11) was followed by heating at 200 °C for one hour. Filtration of the mixture through a Buchner funnel and workup led to an 83% isolated yield of the desired E-trisubstituted styrene 21.
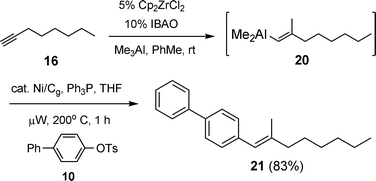 |
| Scheme 12 Carboalumination–cross-coupling: vinylalane + aryl tosylate. | |
Reaction variables & processing
There are several other reaction parameters associated with the use of Ni/Cg not addressed as yet in these latest cross-coupling reactions, including: (1) ligand used; (2) reaction work-up; and (3) recycling of Ni/Cg, all of which can be considered in terms of the potential impact insofar as the overall economics of the chemistry are concerned. Fortunately, all three work to the advantage of this heterogeneous catalysis . That is, screening of ligands (e.g., PCy3, S-Phos) led to the conclusion that Ph3P is the most effective, and least expensive. In cases where biaryl products were close in polarity to Ph3P, addition of CuCl to the crude worked-up reaction mixtures cleanly and quickly removed the phosphine and allowed for the smooth isolation of products (Scheme 13).20 Recycling of Ni/Cg, as with Ni/C, is possible, and here the greater ease of handling of graphite over charcoal allows for more facile recovery and reuse. As illustrated in Scheme 14, an initial Suzuki coupling under microwave conditions between boronic acid 6 and halide 18 led to biaryl 22 (84%). The Ni/Cg could be easily recovered by simple filtration in air, and then reused following activation in the standard fashion (i.e., using n-BuLi in THF; vide supra). Re-exposure of the catalyst to the same reaction conditions, albeit involving completely different coupling partners (13 and 14), afforded product 15 in similar yield (83%; cf. Scheme 10).
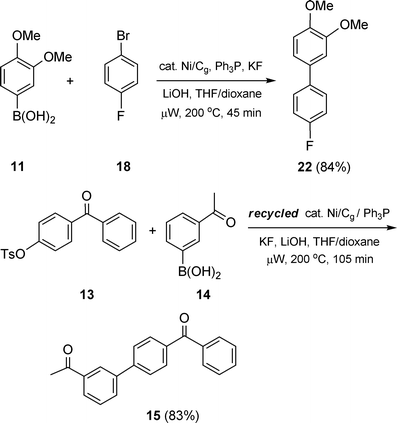 |
| Scheme 14 Recycling the Ni/Cg catalyst. | |
Outlook
Nickel-on-graphite has some attractive features, including elements of “green” chemistry,21 and if these are highly competitive with those of the catalyst impregnated into charcoal, it could find broader use. Nonetheless, it's the unexpected differences between reagents that may offer the biggest ‘bonus’; in particular, with respect to chemoselectivities that lead to options otherwise not possible under more commonly used homogeneous conditions. For example, Ni/C catalyzes aminations of aryl chlorides15 easily under microwave irradiation conditions22 especially when using an activated case such as chlorobenzonitrile (23, Scheme 15). Remarkably, all attempts to effect this standard coupling with morpholine and 23 under identical conditions with nickel-on-graphite have met with total failure.23 But in this case, failure might be a good thing! When considered in light of the above discussion on selected Suzuki couplings (8 + 9 → 10), where Ni/Cg catalyzes the intended chemistry while Ni/C does not (cf. Scheme 8), it's clear that there is much to be learned about the properties of these materials. With progress, there are likely to be other surprises ahead with Ni, as well as other inexpensive metals (e.g., Fe), impregnated into graphite. Perhaps these contributions will find utility within the synthetic community; after all, when starting with base metal salts and graphite, two trivial ingredients approaching earth, wind, and fire, the eventual catalysis is almost for free.
Acknowledgements
We warmly thank the NIH (GM 40287) for its support of our program in biaryl synthesis involving Ni-catalyzed cross-coupling chemistry, and Dr Louise Weaver (University of New Brunswick) for TEM analyses.
References
- B. H. Lipshutz, B. A. Freiman, T. Butler, B. R. Taft and C.-T. Lee, Synthesis, 2005, 17, 2989–2993 CrossRef.
- B. H. Lipshutz, T. Butler, B. A. Frieman, V. Kogan, C.-T. Lee, A. Lower, D. M. Nihan, B. R. Taft and A. E. Tomaso, Jr., Pure Appl. Chem., 2006, 78, 377–384 CrossRef CAS.
- B. H. Lipshutz and S. Tasler, Adv. Synth. Catal., 2001, 343, 327–329 CrossRef CAS.
-
H. Jankowska, A. Swiatkowski and J. Choma, Active Carbon (Ellis Horwood Series in Physical Chemistry), Ellis Horwood Ltd., West Sussex, 1992 Search PubMed.
-
(a) B. H. Lipshutz, Adv. Synth. Catal., 2001, 343, 313–326 CrossRef CAS;
(b) B. H. Lipshutz, S. Tasler, W. Chrisman, B. Spliethoff and B. Tesche, J. Org. Chem., 2003, 68, 1177–1189 CrossRef CAS;
(c) S. Tasler and B. H. Lipshutz, J. Org. Chem., 2003, 68, 1190–1199 CrossRef CAS.
- B. H. Lipshutz, B. A. Frieman and A. E. Tomaso, Angew. Chem., Int. Ed., 2006, 45, 1259–1264 CrossRef CAS.
-
R. C. Bansal, J.-B. Donnet and F. Stoeckli, in Active Carbon, Marcel Dekker Inc., New York, 1988 Search PubMed.
- B. H. Lipshutz, B. A. Freiman, T. Butler and V. Kogan, Angew. Chem., Int. Ed., 2006, 45, 800–803 CrossRef CAS.
- W. Uhlig, J. Organomet. Chem., 1997, 545–546, 281–289 CrossRef CAS.
-
(a) Z. Schay and L. Guczi, Carbon, 1990, 28, 35–42 CrossRef;
(b) A. G. Ruiz, I. R. Ramos and J. L. G. Fierro, Appl. Catal., 1991, 72, 119–137 CrossRef.
-
(a) K. Sasaki, T. Kubo, M. Sakai and Y. Kuroda, Chem. Lett., 1997, 617–618 CrossRef CAS;
(b) W. Cabri, S. De Bernadinis, F. Francalanci and S. Penco, J. Org. Chem., 1990, 55, 350–353 CrossRef CAS;
(c) A. J. Bartlett, J. S. E. Holker, E. O'Brien and T. J. Simpson, J. Chem. Soc., Perkin Trans. 1, 1983, 667–670 RSC.
- H. Tsukamoto, R. Suzuki and Y. Kondo, J. Comb. Chem., 2006, 8, 289–292 CrossRef CAS.
- V. Kogan, Tetrahedron Lett., 2006, 47, 7515–7518 CrossRef CAS.
-
(a) A. Mori, T. Mizusaki, T. Ikawa, T. Maegawa, Y. Monguchi and H. Sajiki, Chem.–Eur. J., 2007, 13, 1432–1441 CrossRef CAS;
(b) A. Mori, T. Mizusaki, T. Ikawa, T. Maegawa, Y. Monguchi and H. Sajiki, Tetrahedron, 2007, 63, 1270–1280 CrossRef CAS;
(c) H. Sajiki, A. Mori, T. Mizusaka, T. Ikawa, T. Maegawa and K. Hirota, Org. Lett., 2006, 8, 987–990 CrossRef CAS.
- B. H. Lipshutz, B. A. Freiman, D. M. Nihan, B. R. Taft, C.-T. Lee and A. Lower, Chem.–Asian J., 2006, 1, 417–429 CrossRef CAS.
-
B. H. Lipshutz, T. Butler and E. Swift, unpublished work.
-
(a) E. Negishi and D. E. Van Horn, J. Am. Chem. Soc., 1977, 99, 3168–3170 CrossRef CAS;
(b) N. Okukado, D. E. Van Horn, W. L. Klima and E. Negishi, Tetrahedron Lett., 1978, 19, 1027–1030 CrossRef.
- B. H. Lipshutz, T. Butler, A. Lower and J. Servesko, Org. Lett., 9, 3737 Search PubMed.
- B. H. Lipshutz, T. Butler and A. Lower, J. Am. Chem. Soc., 2006, 128, 15396–15398 CrossRef CAS.
- B. H. Lipshutz and B. A. Freiman, Org. Lett., 2004, 6, 2305–2308 CrossRef CAS.
-
R. A. Sheldon, I. Arends and U. Hanefeld, in Green Chemistry and Catalysis, Wiley-VCH, New Jersey, 2007 Search PubMed.
-
(a)
Microwave Assisted Organic Synthesis, ed. J. P. Tierney and P. Lidstrom, Blackwell, Oxford, 2005 Search PubMed;
(b) A. K. Bose, M. S. Manhas, S. N. Ganguly, A. H. Sharma and B. K. Banik, Synthesis, 2002, 1578–1591 CrossRef CAS;
(c) C. O. Kappe, Angew. Chem., Int. Ed., 2004, 43, 6250–6284 CrossRef CAS;
(d) B. Desai and C. O. Kappe, Top. Curr. Chem., 2004, 242, 177–208 CAS.
-
B. H. Lipshutz, V. Kogan, B. A. Freiman and T. Butler, unpublished work.
|
This journal is © The Royal Society of Chemistry 2008 |
Click here to see how this site uses Cookies. View our privacy policy here.