DOI:
10.1039/B609522D
(Paper)
J. Environ. Monit., 2006,
8, 1000-1005
Temporal trend studies on polybrominated diphenyl ethers (PBDEs) and polychlorinated biphenyls (PCBs) in ringed seals from East Greenland
Received
4th July 2006
, Accepted 3rd August 2006
First published on 18th August 2006
Abstract
The concentrations of polybrominated diphenyl ethers (PBDEs) 17, 28, 47, 49, 66, 85, 99, 100, 153, 154, and 183 were determined in ringed seal blubber from central East Greenland collected in 1986, 1994, 1999 and during the period 2001 to 2004. The trend of PBDEs was compared with the trends of polychlorinated biphenyls (PCBs) 28, 31, 52, 101, 105, 118, 138, 153, 156, and 180 during the same period. The levels of ΣPBDE in East Greenland ringed seals ranged from 21.8 ng g−1 lipid weight (lw) in 1986 to 39.3 ng g−1 lw in 2001 and are among the highest observed in ringed seal from the Arctic. The dominating congeners were BDE-47 (75.4%) and BDE-99 (9.7%). The concentrations of PBDEs and PCBs increased with the age of the seals, and therefore only young seals (≤4 years old) were included in the temporal trend analyses. No significant trend (p > 0.14) was observed in ΣPBDE or the congeners BDE 28, 47 and, 99 during the period while ΣPCB decreased significantly (p = 0.004) over the period from 1986 to 2004 with an estimated annual rate of 4.3%.
Introduction
Polybrominated diphenyl ethers (PBDEs) are one of the flame retardants widely used as additive in plastics, textiles, electronic equipment and other materials to prevent fires. PBDEs have similar physical and chemical properties and use patterns to those of PCBs, and therefore PBDEs have the potential of long-range transport comparable to that of PCBs.1 PBDEs containing two to seven bromines are also found in Arctic biotic and abiotic samples from zooplankton to polar bears (Ursus maritimus) and humans, and in air, soil and sediments.2 PBDEs are currently not included in the UN ECE Convention on Long-Range Transboundary Air Pollution or the Stockholm Convention on Persistent Organic Pollutants. However, the European Union (EU) decided to ban the penta- and octa-BDE products in late 20043 and similar bans are expected to become effective in several states of the United States in 2006.2
The knowledge of PBDEs in marine biota from Greenland has increased markedly in recent years. Johansen et al.4,5 have studied contaminants in the traditional Greenland diet and they reported content of PBDEs in three marine fish species, one sea bird species, three seal species, walrus (Odobenus rosmarus) and two whale species. The levels of PBDEs based on pooled samples of four fish species and blue mussels (Mytilus edulis) from southern Greenland were reported by Christensen et al.6 The levels of PBDEs in shorthorn sculpin (Myoxocephalus scorpius), Arctic char (Salvelinus alpinus), black guillemot (Cepphus grylle) and ringed seal (Phoca hispida) (data included in this study) all sampled in the same year in the same area in East Greenland have been reported by Vorkamp et al.7 The levels of PBDEs in liver and eggs of black guillemot from East and West Greenland showed higher concentrations of PBDEs as well as other organochlorine (PCBs, DDTs, chlordanes, HCHs, HCB and toxaphene) in East Greenland compared to West Greenland.8 Dietz et al.9 performed a comprehensive study on PBDE concentrations in 92 polar bears from East Greenland including the seasonal pattern. A subsample of these were compared to polar bears of the rest of the Arctic, showing that ΣPBDE as well as the individual congeners BDE-47, BDE-99, BDE-100, BDE-153, were highest in East Greenland.10 In general these Greenlandic studies have supported the existing evidence of PBDE biomagnifications in the Arctic marine food webs and the spatial trend of increasing PBDEs levels from the eastern Canadian Arctic over West and East Greenland to Svalbard. In these respects PBDEs seem to follow the same trends as previously established for PCBs.
Temporal trend information of contaminant concentrations is crucial for understanding the effect and spreading of emissions to the environment, and to evaluate the effectiveness of regulations on the production and use of chemicals of concern. However, only few studies have reported on temporal trends of PBDEs in the marine biota from the Arctic. Most of these studies report increasing trends. In the Canadian Arctic increasing trends of PBDEs from the mid-1970s have been reported in seabird eggs (northern fulmars, Fulmarus glacialis and thick billed murres, Uria lomvia) from Prince Leopold Island in Lancaster Sound,11 in ringed seal from Holman, Canada since the early 1980s12 and in beluga (Delphinapterus leucas) from southeast Baffin Island since the early 1980s.13 The only temporal trend study of PBDEs from Greenland was carried out on peregrine falcon (Falco peregrinus) eggs,14 which also showed increasing levels since the mid-1980s. However, the migratory behaviour of this species makes the evaluation difficult, since PBDE exposure and uptake is not limited to the Greenland environment. In Scandinavia and Europe a few temporal trend studies of PBDEs in guillemot (Uria algae) eggs from the Baltic Sea,15 in pike (Esox lucius) from Lake Bohmen in the southern part of Sweden,16 and in blue mussels (Mytilus edulis) from the Seine estuary, France17 have shown levelling off or decreasing trends of PBDEs in recent years after a period with an increasing trend.
The main objective of this study was to examine the temporal trend of PBDEs in the East Greenland area. We selected ringed seal as our monitoring species. The ringed seal is one of the top predators in the marine food chain and has a circumpolar distribution, which makes it a suitable monitoring species for both spatial and temporal trends. Furthermore, the ringed seal is regarded as a rather stationary marine mammal species although some long distance migrations (more than 100 km) may occur.18 A second objective of this study was to compare the temporal trend of PBDEs with that of PCBs. An earlier study on the temporal trend of PCBs in ringed seals from East Greenland indicated a decreasing trend.19 Since then, the time-series of PCBs has been extended allowing more firm conclusions. We have analysed both archived blubber tissues stored in our specimen bank and recently collected samples.
Materials and methods
Samples
Samples of ringed seals were available from Ittoqqortoormiit in central East Greenland (70°28′ N, 21°95′ E) collected in the years 1986, 1994 and 1999–2004 (Fig. 1). Ringed seal samples were obtained from local hunters mainly during May and June. The animals were either shot or caught in nets. Date, body length and circumference, weight and sex were routinely recorded. Blubber samples were taken for organochlorine analysis. Samples were frozen and shipped to Denmark, where they were kept at −20 °C. In the laboratory, blubber samples were lightly thawed and the outer exposed tissue layer was removed prior to analysis to minimize possible contamination due to handling and storage. The age of the seals were estimated by counting annual layering in the cementum of the canine tooth after decalcification, thin sectioning (14 μm) and staining in toluidine blue.20
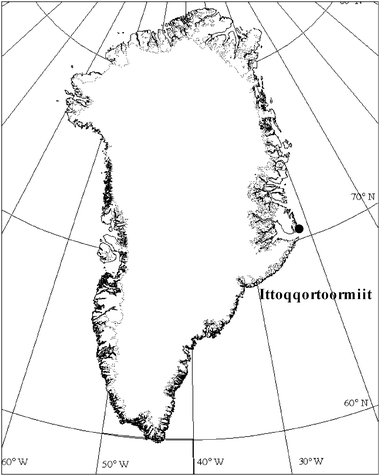 |
| Fig. 1 Map of Greenland showing the sampling location of ringed seals collected between 1986 and 2004 used for PBDE and PCB analysis. | |
Organohalogen residue analyses
Most of the chemical analyses were carried out soon after the collection, however in some cases (PBDE: 1986, 1994, 1999, 2000; PCB: 1986) the analyses were carried out based on archived material stored at −20 °C since sample collection. The analytical methods for PBDEs and PCBs were as described in detail by Vorkamp et al.7,8. In brief, the samples were homogenised, dried with anhydrous Na2SO4, spiked with the recovery standard BDE-77, followed by soxhlet extraction using n-hexane and acetone. The extracts were cleaned using a multilayered glass column packed with deactivated alumina, activated silica and activated silica impregnated with concentrated sulfuric acid and anhydrous Na2SO4. The samples were loaded on the column and the target compounds (BDEs and PCBs) were eluted in n-hexane. The eluates were spiked with quantification standards BDE- 71 and PCB-53 and concentrated to a final volume of 1 ml. BDEs in concentrated extracts were determined by gas chromatography–mass spectrometry in the negative chemical ionisation mode,7 and the CBs were determined by gas chromatography with electron capture detection, employing two capillary columns of different polarity.21 For quality assurance of the analyses, the samples were analysed in batches of 12, which included a blank, two samples of an internal reference material (sand launce (Ammodytes tobianus) oil) and a sample in duplicate. The industrial sand launce oil was received from Triple Nine Fish Protein, Esbjerg, Denmark. Accuracy was monitored by the control charts of internal reference material with warning and action limits. The overall quality of the analyses was monitored by participation in BSEF/QUASIMEME exercise.
All samples from 1986 and the period 2001 to 2004 were analysed for both PBDEs and PCBs, while samples from 1994 included 7 samples analysed for PBDEs and 24 samples analysed for PCBs (Table 1). Samples from 1999 were only analysed for PBDEs, and samples from 2000 only for PCBs. As the number of individual BDE and PCB congeners analysed at NERI have changed over the years only congeners common for all years were included in this temporal trend (i.e. BDE-17, BDE-28, BDE-47, BDE-49, BDE-66, BDE-85, BDE-99, BDE-100, BDE-153, BDE-154, BDE-183). The detection limit was generally <0.1 ng g−1 wet weight (ww) for all congeners except for BDE 183, where it increased to <0.6 ng g−1 ww for some samples due the small sample amount analysed. For calculating the sum of the congeners determined (ΣPBDE), a value of zero was used for congeners which were below the detection limits. The following individual PCB congeners were analysed in all samples: PCB-28, PCB-31, PCB-52, PCB-101, PCB-105, PCB-118, PCB-138, PCB-153, PCB-156, PCB-180. No congener concentrations were below the detection limit of generally 1.5 ng g−1 ww.
Table 1 Mean concentrations (ng g−1 lipid weight) of ΣPBDE and ΣPCB-10 in ringed seals from central East Greenland and characteristics of the samples collected in each years between 1986 and 2004
|
1986 |
1994 |
1999 |
2000 |
2001 |
2002 |
2003 |
2004 |
M/F = 13/11, Age range = 0–11, Mean age = 4.5.
One seal without sex determination.
|
Mean (SD) ΣPCB-10 |
1243 (373) |
967 (420) |
— |
680 (234) |
680 (296) |
680 (212) |
596 (196) |
605 (240) |
N (ΣPCB-10) |
20 |
24a |
0 |
17 |
19 |
20 |
20 |
20 |
Mean (SD) ΣPBDE |
21.8 (4.9) |
32.4 (6.0) |
39.3 (18.0) |
— |
37.5 (14.9) |
34.0 (8.8) |
30.9 (9.8) |
32.5 (13.8) |
N (ΣPBDE) |
20 |
7 |
19 |
0 |
19 |
20 |
20 |
20 |
Male/female |
12/8 |
5/2 |
11/8 |
8/8 |
11/8 |
11/8b |
13/7 |
13/7 |
Age range |
2–4 |
3–6 |
0–10 |
1–9 |
0–24 |
1–11 |
2–14 |
0–7 |
Mean age |
3.0 |
4.7 |
4.7 |
4.5 |
5.2 |
4.6 |
5.9 |
3.4 |
Statistical treatment
The statistical analyses of temporal trends follow the procedures used in assessments at ICES (International Council for the Exploitation of the Sea).22 Log-linear regression analyses using annual median values were performed. To describe possible non-linear trend component in the time-series a three-point running average smoother was fitted to the annual median concentrations. The smoother was tested by means of analyses of variance to see whether it provided a significantly better description of the trend than the log-linear trend model or a “no change” in concentrations over time.
Results and discussion
Concentration levels and geographical trend
The annual mean ΣPBDE concentrations ranged from 21.8 (1986) to 39.3 (1999) ng g−1 lipid weight (lw) and the annual mean ΣPCB-10 concentrations from 596 (2003) to 1243 (1986) ng g−1 lw (Table 1). The levels of ΣPBDE and ΣPCB-10 found in ringed seal blubber were lower than found in adipose from polar bears9,23 and in eggs from black guillemot7,8 from the same area. The PBDEs levels in ringed seals from different Arctic locations was recently reviewed by de Wit24 and de Wit et al.2 Ringed seals from East Greenland have the highest level of PBDEs found in the Arctic, including data from six locations in the Canadian Arctic, three locations in the Russian Arctic, and one location from Svalbard and West Greenland.2 The ΣPBDE levels of East Greenland ringed seals found in 2004 were approximately eight times higher than reported for ringed seals from West Greenland,4 approximately six times higher than ringed seals from the western Canadian Arctic12 and approximately 1.8 times higher than ringed seals from Svalbard.25 Although the numbers of congeners included in these figures vary among the studies, they all include the most dominating congeners such as BDE-47, -99, and -100. A similar geographical trend, with the highest levels of PBDEs in East Greenland and Svalbard, has been observed in polar bears,10 which could be expected as ringed seals are the main food item of polar bear. East Greenland is therefore one of the hot spots of PBDEs in the Arctic. However, compared to ringed seals from the Scandinavian part of the Baltic Sea the levels in East Greenland ringed seals were about ten times lower.24
Age accumulation
The ΣPBDE and ΣPCB-10 concentrations increase with age in both males and females (Fig. 2) indicating PBDE and PCB accumulation. In males the ΣPBDE and ΣPCB-10 levels increased up to an age of about 10 years old, after which no further accumulation was found. In females the accumulation with age was not as obvious as the males, however only few females above 10 years old existed. One reason is that adult females transfer lipophilic compounds to their offspring during gestation and lactation. Lower levels of persistent organic pollutants in adult vs. younger females are therefore often seen in cetaceans and pinnipeds.26,27 Ringed seals generally become sexually mature when 3–5 years old.28
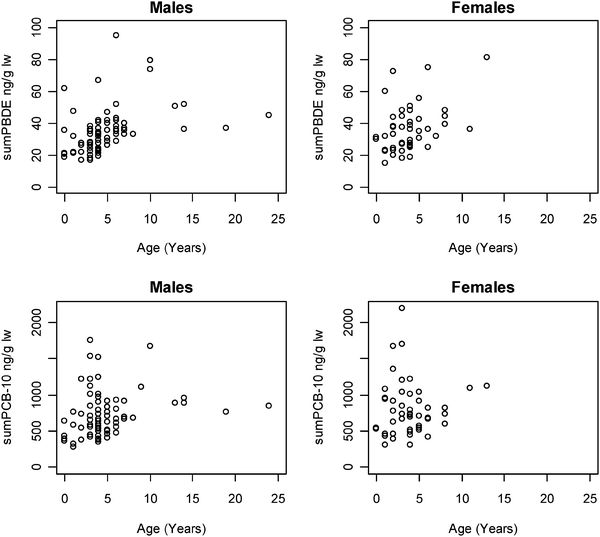 |
| Fig. 2 Plot of ΣPBDE (top) and ΣPCB-10 (bottom) versus age of male (left) and female (right) ringed seals. | |
Congener composition
BDE-47 was the dominating congener constituting 75.4% of ΣPBDE, followed by BDE-99 constituting 9.7% (Table 2). BDE-47 was also the by far the most dominating congener, followed by BDE-99, in different marine fish, seabird and marine mammal species.4,7 In polar bears from East Greenland BDE-47 was also the dominating congener (72.2%), followed by BDE-153 (19.5%).9 In ringed seal, BDE-153 constituted only 1.7% of the total PBDEs in this study. Muir et al.10 reported biomagnification factors (BMF) for PBDE congeners in polar bears assuming ringed seal as prey species from five Arctic locations. The BMF for BDE-153 was considerably higher than for other PBDE congeners (BDE-47, -99, -100, -154). Possible explanations may be the chemical structure making BDE-153 resistant to biodegradation and/or result in low efficiency of transfer via lactation,10 as well as metabolic debromination of higher brominated BDEs.2
Table 2 Average percent distributions of the individual PBDE and PCB congeners in ringed seal from central East Greenland collected between 1986 and 2004
PBDE congener |
Average percentage |
PCB congener |
Average percentage |
BDE-17 |
0.3% |
PCB-28 |
1.4% |
BDE-28 |
4.8% |
PCB-31 |
1.1% |
BDE-47 |
75.4% |
PCB-52 |
4.1% |
BDE-49 |
0.7% |
PCB-101 |
11.6% |
BDE-66 |
0.3% |
PCB-105 |
5.4% |
BDE-85 |
0.9% |
PCB-118 |
11.7% |
BDE-99 |
9.7% |
PCB-138 |
21.8% |
BDE-100 |
4.3% |
PCB-153 |
32.2% |
BDE-153 |
1.7% |
PCB-156 |
1.4% |
BDE-154 |
2.0% |
PCB-180 |
9.2% |
BDE-183 |
<0.1% |
|
|
Temporal trends
PBDE time trends.
The analyses of temporal trends of PBDEs and ΣPCB-10 concentrations included only young ringed seals (≤4 years old) to minimise the influence of differences between sex caused by female transfer and possible differences in the age-contaminant relationship from year to year. Table 3 shows the annual median concentrations of each PBDE congener and of ΣPBDE. Temporal trend analyses were performed only for congeners with median values above the detection limit in all years (BDE-28, -47, and -99). The temporal trend of ΣPBDE concentrations from 1986 to 2004 is shown in Fig. 3. No significant time trend in ΣPBDE concentrations was detected by a linear regression analysis of logarithmic transformed median values (slope = 0.012, p = 0.28). A three-year running smoother applied to represent any systematic trend did not result in a significantly better description than no trend at all (p = 0.57). Fig. 4 shows the temporal developments of BDE-28, -47, and -99 together with ΣPBDE. No single congener showed a significant trend whether tested by log-linear regression (p > 0.14) or by a three-year running smoother (p > 0.24). The lack of trend in our study is not in agreement with the only other time trend study of PBDEs in Greenland biota. The temporal trend of PBDEs in peregrine falcon eggs from South Greenland covering approximately the same period from 1986 to 2003 was studied by Vorkamp et al.14 They found a significant increase of approximately 5–10% per year for BDE-99, -100, -153 and -209 but for ΣPBDE the significance level was just above 5%. However, the peregrine falcon in Greenland migrates to Central and South America in winter and therefore the observed increase of PBDEs may not solely reflect contamination of the Greenland environment.14
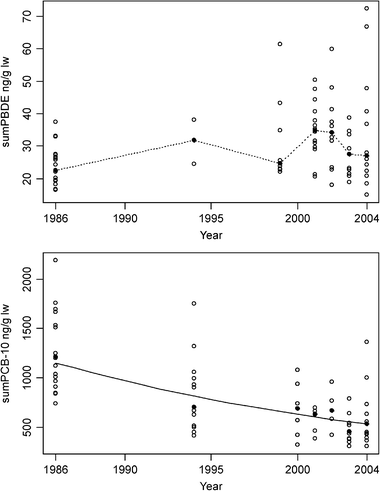 |
| Fig. 3 Temporal trend in ΣPBDE concentrations (top) and ΣPCB-10 (bottom) in blubber of young (≤4 years old) ringed seals from central East Greenland between 1986 and 2004. The filled circles represent the median concentrations. The solid line represents an exponential curve determined by log-linear regression analysis, only shown when the regression was significant. A broken line connecting median concentrations was shown when the log-linear regression analysis was not significant. | |
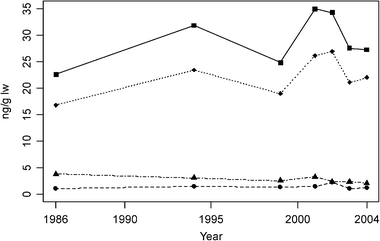 |
| Fig. 4 Median concentrations of ΣPBDE (squares), BDE-47 (diamonds), BDE-99 (triangle) and BDE-28 (octagons) in blubber of young (≤4 years old) ringed seals from central East Greenland. | |
Table 3 Median concentrations (ng/g lipid weight) of BDE-17, 28, 47, 49, 66, 85, 99, 100, 153, 154, 183 and ΣPBDE in (≤4 years old) ringed seal from central East Greenland
|
1986 |
1994 |
1999 |
2001 |
2002 |
2003 |
2004 |
BDE-17 |
<0.1 |
<0.1 |
<0.1 |
0.3 |
0.8 |
<0.1 |
<0.1 |
BDE-28 |
1.1 |
1.5 |
1.3 |
1.5 |
2.3 |
1.1 |
1.3 |
BDE-47 |
16.8 |
23.4 |
19.0 |
26.1 |
26.9 |
21.1 |
22.0 |
BDE-49 |
<0.1 |
0.5 |
0.4 |
0.4 |
<0.1 |
0.3 |
0.3 |
BDE-66 |
<0.1 |
0.3 |
<0.1 |
0.3 |
<0.1 |
<0.1 |
<0.1 |
BDE-85 |
<0.1 |
<0.1 |
<0.1 |
1.6 |
<0.1 |
<0.1 |
<0.1 |
BDE-99 |
3.8 |
3.1 |
2.6 |
3.3 |
2.4 |
2.4 |
2.1 |
BDE-100 |
1.4 |
1.7 |
1.4 |
<0.1 |
1.7 |
1.3 |
1.4 |
BDE-153 |
<0.1 |
0.8 |
<0.1 |
0.5 |
<0.1 |
0.7 |
0.7 |
BDE-154 |
0.9 |
0.6 |
0.5 |
0.8 |
<0.1 |
0.4 |
<0.1 |
BDE-183 |
<0.6 |
<0.6 |
<0.6 |
<0.6 |
<0.6 |
<0.6 |
<0.6 |
ΣPBDE |
22.6 |
31.8 |
24.8 |
34.9 |
34.2 |
27.5 |
27.2 |
N
|
19 |
3 |
8 |
14 |
11 |
11 |
13 |
Temporal trends of PBDEs in biota from the Canadian Arctic were recently reviewed by Braune et al.11 and de Wit et al.2 Mean concentrations of ΣPBDE in eggs of northern fulmar (Fulmarus glacialis) and thick-billed murres (Uria lomvia) from Prince Leopold Island, central Canadian Arctic archipelago increased 9.1- and 4.4-fold, respectively, between 1975 and 1998. In male ringed seal aged 0–15 years from Holman Island, Western Canadian Arctic a 9-fold increase in ΣPBDE was reported12 over the period 1981 to 2000. However, more recent results from this group have shown a levelling off or decline from 2000 to 2003.29
ΣPBDE also increased significantly in beluga from southeast Baffin Island over the period 1982 to 1997.13 In landlocked Arctic char (Salvelinus alpinus), representing the freshwater environment in Canada, BDE-47 and BDE-99 doubled from 1997 to 2002.30 The increase in Canadian biota is likely reflecting the North American (>95% of the world total) use of penta-mix formulation, which according to Law et al.31 is likely to continue to increase for some time.
The temporal development of PBDEs during the last two decades in East Greenland seals does not follow the increasing trend found in biota from the Canadian Arctic. At the same time the levels of PBDEs in East Greenland seals are markedly higher and have been so since 1986, than the levels in seals from the high Arctic Canadian locations. The sources and pathway of PBDEs to the Canadian Arctic and to East Greenland may be quite different and therefore result in different temporal trends in ringed seals from the two regions. An explanation may be that the main increasing phase of PBDEs in East Greenland seals occurred before 1994, and is therefore covered only by the first two data points (1986 and 1994), and that PBDEs in the most recent years are in a phase of levelling off or decreasing. The most recent data points in our time trend study show a slight decrease (Fig. 3).
Several studies of PBDE concentrations in biota outside the Arctic have shown decreasing trends in the recent years. In eggs of guillemot from the Baltic Sea, a retrospective study including BDE-47, -99, -100 covering the period 1969 to 2001 showed increasing concentrations from the 1970s to the 1980s, peaking around late 1980s and then followed by a rapid decrease.15 A somewhat similar picture was found in pike (Esox lucius) from Lake Bohmen in the southern part of Sweden, where all BDE congeners show increasing trends from late 1960s up to the mid-1980s and then decrease or level off.16 In blue mussels (Mytilus edulis) from the Seine estuary, France the temporal trend picture of PBDEs showed a marked increase during the period 1981 to 1991–95 followed by a levelling off and a possible beginning of decrease until 2003.17 In northern fur seals (Callorhinus ursinus) from northern Japan ΣPBDE peaked in the early 1990s.32 These studies are all closer to the highly industrialized areas than the Arctic and the observed decreasing trend in recent years is likely to be the result of phasing out and restricted use of PBDEs by several Baltic, European countries and Japan.
ΣPCB-10 time trends
The median ΣPCB-10 concentration showed a significantly (p = 0.004) decreasing trend with an estimated annual decrease of 4.3% (Fig. 3). A non-linear trend component caught by a three-year running smoother was not a significantly (p = 0.99) better trend description than the log-linear regression. A decreasing trend has also been found in polar bears from the same area,23 however this study was only based on samples from 1990 and from 1999–2001. During this period the ΣPCB had decreased to 22% of the concentration in 1990, which is equivalent of a yearly decrease of 14.0% per year; considerably higher than the 4.3% decrease found in the seals. Several of the Greenlandic time-series of organochlorine compounds in biota (ringed seals and shorthorn sculpin from West and East Greenland) indicate decreasing trends since the mid 1990s, however the power of the time-series to detect statistical trend were rather poor.19
Braune et al.11 recently reviewed temporal trends of persistent organic pollutants in marine biota of the Canadian Arctic. PCBs showed significant declines in seabird (black-legged kittiwake (Rissa tridactyla), northern fulmar, thick-billed murre) eggs since 1975 and in ringed seals from three different locations since the early 1970s. However, in beluga from southeast Baffin Island, narwhal (Monodon monoceros) from the Baffin Bay–Lancaster Sound region and polar bears from the Churchill area of western Hudson Bay did not show a consistent or major decline since the early 1980s, and in the case of polar bear from the late 1960s.11
Acknowledgements
The study was funded by the Danish Environmental Protection Agency within the Danish Cooperation for Environment in the Arctic (DANCEA). Hanne Tuborg, Birger Sandell and Sigga Joensen helped the authors in obtaining the samples from the local hunters in East Greenland. The following lab technicians helped with the sample handling in the freezer and conducted the PBDE and PCB analyses: Lene Bruun, Sigga Joensen, Annegrete Ljungqvist, Karin Rosengarrd Pedersen and Birgit Groth.
References
- F. Wania and C. B. Dugani, Environ. Toxicol. Chem., 2003, 22, 1252 CrossRef CAS.
- C. A. de Wit, M. Alaee and D. C. G. Muir, Chemosphere, 2006 Search PubMed , in press.
- P. Cox and P. Ethymiou, Official Journal of the European Union OJ, 2003, L 42, 45 Search PubMed.
-
P. Johansen, D. Muir, G. Asmund and F. Riget, NERI Technical Report No. 492, 2004, pp.74, http://technical-reports.dmu.dk Search PubMed.
-
P. Johansen, D. Muir, G. Asmund and F. Riget, NERI Technical Report No. 2006, in press http://technical-reports.dmu.dk Search PubMed.
- J. H. Christensen, M. Glasius, M. Pécseli, J. Platz and G. Pritzl, Chemosphere, 2002, 47, 631 CrossRef CAS.
- K. Vorkamp, J. H. Christensen and F. Rigét, Sci. Total Environ., 2004, 331, 143 CrossRef CAS.
- K. Vorkamp, J. H. Christensen, M. Glasius and F. F. Rigét, Mar. Pollut. Bull., 2004, 48, 111 CrossRef CAS.
- R. Dietz, F. F. Rigét, C. Sonne, D. C. G. Muir, S. Backus, E. W. Born, M. Kirkegaard and R. J. Letcher, Environ. Pollut. (Amsterdam, Neth.) Search PubMed , in press.
- D. C. G. Muir, S. Backus, A. E. Derocher, R. Dietz, T. J. Evans, G. W. Gabrielsen, J. Nagy, R. J. Norstrom, C. Sonne, I. Stirling and R. J. Letcher, Environ. Sci. Technol., 2006, 40, 449 CrossRef CAS.
- B. M. Braune, P. M. Outridge, A. T. Fisk, D. C. G. Muir, P. A. Helm, K. Hobbs, P. F. Hoekstra, Z. A. Kuzuk, M. Kwan, R. J. Letcher, W. L. Lockhart, R. J. Norstrom, G. A. Stern and I. Stirling, Sci. Total Environ., 2005, 351–352, 4 CrossRef CAS.
- M. G. Ikonomou, S. Rayne and R. F. Addison, Environ. Sci. Technol., 2002, 36, 1886 CrossRef CAS.
- G. A. Stern and M. G. Ikonomou, Organohalogen Compd., 2000, 47, 81 CAS.
- K. Vorkamp, M. Thomsen, K. Falk, H. Leslie, S. Møller and P. B. Sørensen, Environ. Sci. Technol., 2005, 39, 8199 CrossRef CAS.
- U. Sellström, A. Bignert, A. Kierkegaard, L. Häggberg, C. de Wit, M. Olsson and B. Jansson, Environ. Sci. Technol., 2003, 37, 5496 CrossRef.
- A. Kierkegaard, A. Bignert, U. Sellström, M. Olsson, L. Asplund, B. Jansson and C. A. de Wit, Environmental Pollution., 2004, 130, 187 Search PubMed.
- I. Johansson, K. H. Moisan, N. Guiot, C. Munschy and J. Tronczyński, Chemosphere, 2006, 64, 296 CrossRef CAS.
- F. O. Kapel, J. Christensen, M.-P. Heide-Jørgensen, T. Härkönen, E. W. Born, L. Ø. Knutsen, F. Riget and J. Teilmann, NAMMCO Sci. Contrib., 1998, 1, 211 Search PubMed.
- F. Riget, R. Dietz, K. Vorkamp, P. Johansen and D. Muir, Sci. Total Environ., 2004, 331, 29 CrossRef CAS.
- R. Dietz, M.-P. Heide-Jørgensen, J. Teilmann, N. Valentin and T. Härkönen, Sarsia., 1991, 76, 17 Search PubMed.
- M. Cleemann, G. B. Paulsen, E. Storr-Hansen and A. Fromberg, J. AOAC Int., 1999, 82(5), 1175 CAS.
-
M. D. Nicholson, R. Fryer and J. R. Larsen, Techniques in Marine Environmental Science, ICES, Copenhagen, 1995 Search PubMed.
- R. Dietz, F. F. Rigét, C. Sonne, R. Letcher, E. W. Born and D. C. G. Muir, Sci. Total Environ., 2004, 331, 107 CrossRef CAS.
- C. A. de Wit, Chemosphere, 2002, 46, 583 CrossRef.
- H. Wolkers, B. van Bavel, A. E. Derocher, Ø. Wiig, K. M. Kovacs, C. Lydersen and G. Lindström, Environ. Sci. Technol., 2004, 38, 1667 CrossRef CAS.
- R. Dietz, F. Rigét, K. A. Hobson, M.-P. Heide-Jørgensen, P. Møller, M. Cleemann, J. de Boer and M. Glasius, Sci. Total Environ., 2004, 331, 83 CrossRef CAS.
- G. A. Stern, D. C. G. Muir, M. D. Segstro, R. Dietz and M.-P. Heide-Jørgensen, Medd. Grønland Biosci., 1994, 39, 245 Search PubMed.
- T. G. Smith, Bull. – Fish. Res. Board Can., 1973, 181, 55 Search PubMed.
-
M. G. Ikonomou, B. C. Kelly and G. A. Stern, Presented at the 25th International Symposium on Halogenated Organic Pollutants and POPs, 2005, in press Search PubMed.
-
D. C. G. Muir and G. Köck, Northern Contaminants Program, Indian and Northern Affairs Canada, Ottawa, ON, Canada, 2003, p. 318 Search PubMed.
- R. J. Law, C. R. Allchin, J. de Boer, A. Covaci, D. Herzke, P. Lepom, S. Morris, J. Tronczynski and C. de Wit, Chemosphere, 2006, 64, 187 CrossRef CAS.
- N. Kajiwara, D. Ueno, A. Takahashi, N. Baba and S. Tanabe, Environ. Sci. Technol., 2004, 38, 3804 CrossRef CAS.
|
This journal is © The Royal Society of Chemistry 2006 |
Click here to see how this site uses Cookies. View our privacy policy here.