DOI:
10.1039/B511584A
(Paper)
CrystEngComm, 2006,
8, 84-93
Adjusting the frameworks of polymeric silver(I) complexes with 2-aminopyrimidyl ligands by changing the counterions
Received
15th August 2005
, Accepted 6th January 2006
First published on 13th January 2006
Abstract
The complexes [Ag2(L)2(SO4)]∞, (L = 2-aminopyrimidine) 1, [Ag(L)1.5(ClO4)]∞, 2, and [Ag(L)(OAc)·2H2O]∞, 3, were prepared by layering the aqueous solution of the corresponding Ag(I) salt with L in ethanol, while [Ag(L′)(NO3)]∞, 4, and [Ag(L′)(ClO4)·H2O]∞, 5, were prepared by refluxing AgNO3 and AgClO4, respectively, with 2-amino-4,6-dimethylpyrimidine (L′) in H2O. Reaction of Ag2SO4 with L′ in methanol afforded the complex [Ag4(L′)2(SO4)2(H2O)4]∞, 6. In complex 1, the L ligands are coordinated to the Ag(I) metal centers in new tridentate fashions, forming 2-D pleated molecular sheets which are interlinked by the SO42− anions to form a 3-D layer structure. Complex 2 forms a pleated molecular sheet consisting of twenty four-membered metallocycles, involving six Ag ions which are bridged by six L ligands through the pyrimidyl nitrogen atoms. Complex 3 forms linear chains with sinusoidal geometry, which are interlinked through extensive Ag⋯Ag interactions (Ag⋯Ag = 3.102 Å) to form 2-D wavy rectangular grids. Complex 4 forms single-strained helical coordination polymers, which are interlinked through a series of Ag⋯O interactions (2.667 and 2.699 Å) to form 2-D chair like grids. The silver atoms of 5 are bound to the nitrogen atoms of two symmetry-related L′ ligands in a distinctly non-linear geometry, forming zigzag chains. The structure of 6 consists of clusters of the formula [Ag8(L′)4(SO4)4(H2O)8], which are interlinked through L′ ligands to form 2-D sheets. The μ1-O, μ2-O′- bonding mode of the SO42− anion in complex 6 is unique for Ag(I) complexes. Their thermal properties have been investigated by using differential scanning calorimetry (DSC) and thermogravimetric analyses (TGA)
Introduction
In recent years the study on the self-assembly of organic and inorganic molecules in the solid state has become one of intense research activity, because it extends the range of new solids which can be designed to have particular physical and chemical properties.1,2 In addition to the nature of the metal atoms and the ligands, counterion-based interaction is one of the common factors that can be modulated to determine the structural type. Many topologically promising Ag(I)-containing architectures have been constructed with nitrogen-containing bidentate building blocks.3 The various types of polymeric structure include 1-D, 2-D and 3-D network structure.3b Despite some recent progress, the ability to predict and control the supramolecular assembly of molecules remains an elusive goal, and much more work is required to understand the inter- and intramolecular forces that determine the patterns of molecular structure and crystal packing in the solid state.
During our study on the reactions of Ag(I) ion with 2-aminopyrimidine (L) and 2-amino-4,6-dimethylpyrimidine (L′), we have found that L and L′ can coordinate to the metal centers through various bonding modes and a new tridentate bonding mode was also observed. It was also found that changing the counterion of Ag(I) metal had a drastic effect on the structures of the Ag(I) coordination polymers, which were obtained as 1-D polymeric chains, 2-D molecular sheets and 3-D supramolecular frameworks. The syntheses, structures and thermal properties of {[Ag2(L)2(SO4)]}∞, 1, [Ag(L)1.5(ClO4)]∞, 2 and [Ag(L)(OAc)·2H2O]∞, 3, [Ag(L′)(NO3)]∞, 4, [Ag(L′)(ClO4)·H2O]∞, 5, and [Ag4(L′)2(SO4)2(H2O)4]∞, 6, form the subject of this report.
Experimental
General procedures
IR spectra were obtained from a Jasco FT/IR-460 plus spectrometer. Elemental analyses were obtained from a PE 2400 series II CHNS/O analyzer or a HERAEUS VaruoEL analyzer. Thermogravimetric analyses (TGA) were obtained on a DuPont TGA 2050. Differential scanning calorimetry (DSC) measurements were carried out on a DuPont DSC Q-10.
Materials
The reagents AgPF6, Ag2SO4, AgClO4, AgOAc were purchased from Aldrich Chemical Co., and 2-aminopyrimidine and 2-amino-4,6-dimethylpyrimidine were purchased from Lancaster Co.
Synthesis
[Ag2(L)2(SO4)]∞, 1.
An ethanol solution of 2-aminopyrimidine (0.095 g, 1 mmol) was layered on top of an aqueous solution of Ag2SO4 (0.312 g, 1 mmol). After one month, needle like crystals were found at the interface. The crystal were collected, washed by ether and then dried under vacuum. Yield: 0.45g (90%). Anal Calcd for C8H10Ag2N6O4S: C, 19.14; H, 2.01; N, 16.74%. Found: C, 19.14; H, 2.02; N, 16.86%. IR (KBr disk): 2298(m), 1650(m), 1625(s), 1572(s), 1460(m), 1352(m), 1200(m), 808(m), 595(m), 535(m).
[Ag(L)1.5(ClO4)]∞, 2.
An ethanol solution of 2-aminopyrimidine (0.095 g, 1 mmol) was layered on top of an aqueous solution of AgClO4 (0.207 g, 1 mmol). After one month, needle like crystals were found at the interface. The crystals were collected, washed with ether and then dried under vacuum. Yield: 0.28g (88%). Anal. Calcd for C6H7.5AgClN4.5O4: C, 20.60; H, 2.16; N, 18.01%. Found: C, 20.66; H, 2.19; N, 18.08%. IR (KBr disk): 3492(m), 3398(m), 3352(m), 3170(s), 2349(m), 1626(m), 1478(m), 1445(m), 1357(s), 1224(s), 1062(m), 803(s), 644(m), 466(m).
[Ag(L)(OAc)·2H2O]∞, 3.
An ethanol solution of 2-aminopyrimidine (0.095 g, 1 mmol) was layered on top of an aqueous solution of AgOAc (0.168 g, 1 mmol). After one month, needle like crystals were found at the interface. The crystals were collected, washed with ether and then dried under vacuum. Yield: 0.27g (87%). Anal. Calcd for C6H9AgN3O4: C, 24.43; H, 3.07; N, 14.24%. Found: C, 24.42; H, 3.26; N, 14.52%. IR (KBr disk): 3397(s), 3349(m), 3302(m), 3188(m), 1763(s), 1685(m), 1581(m), 1570(m), 1477(m), 1412(m), 1351(s), 1047(m), 973(m), 788(s), 658(s), 589(m), 515(s).
[Ag(L′)(NO3)]∞, 4.
2-amino-4,6-dimethylpyrimidine (0.049 g, 0.40 mmol) was placed in a flask containing 10 ml of H2O and AgNO3 (0.034 g, 0.20 mmol) was added. The mixture was then refluxed for 20 min to yield a white solid. The precipitate was filtered and washed with H2O and then dried under reduced pressure to give the white product. Yield: 0.048 g (82%). IR (KBr disk): 3383 (m), 3315 (m), 3177 (br), 1644 (m), 1598 (m), 1555 (m), 1479 (m), 1407 (m), 1367 (s), 1298 (m), 1033 (m), 958 (s), 843 (s), 811 (s), 782 (m), 710 (m), 580 (m), 527 (m). Calcd. For C6H9AgN4O3 (MW = 293.04): C, 24.59; H, 3.09; N, 19.12 %; Found: C, 24.39; H, 2.79; N, 19.10 %.
[Ag(L′)(ClO4)·H2O]∞, 5.
2-amino-4,6-dimethylpyrimidine (0.049 g, 0.40 mmol) was placed in a flask containing 10 ml of H2O and AgClO4 (0.041 g, 0.40 mmol) was added. The mixture was then refluxed for 20 min to yield a white solid. The precipitate was filtered and washed with H2O and then dried under reduced pressure to give the white product. Yield: 0.059 g (85%). IR (KBr disk): 3423 (m), 3310 (m), 3192 (m), 2359 (m), 1625 (m), 1600 (m), 1466 (m), 1392 (m), 1223 (s), 1093 (m), 792 (s), 626 (m), 476 (m). Calcd. For C6H11AgClN3O5 (MW = 348.15): C, 21.80; H, 2.74; N, 12.71 %; Found: C, 21.20; H, 2.71; N, 12.66%.
{[Ag4(L′)2(SO4)2(H2O)4]}∞, 6.
2-Amino-4,6-dimethylpyrimidine (0.049 g, 0.40 mmol) was placed in a flask containing 10 ml of CH3OH and Ag2SO4 (0.062 g, 0.20 mmol) was added. The mixture was then refluxed for 20 min to yield a white solid. The precipitate was filtered and washed with CH3OH and then dried under reduced pressure to give the white product. Yield: 0.096 g (78%). IR (KBr disk): 2360 (m), 1637 (m), 1571 (m), 1402 (m), 1285 (m), 1209 (m), 802 (m), 589 (m), 547 (s), 419 (s). Calcd. For C12H26AgN6O12S2 (MW = 941.99): C, 23.30; H, 4.23; N, 13.59 %; Found: C, 23.42; H, 4.18; N, 13.72 %.
X-ray crystallography
The diffraction data of 1 was collected on a Bruker AXS P4 diffractometer, which was equipped with graphite-monochromated Mo Kα (Kα = 0.71073 Å) radiation. Data reduction was carried out using standard methods with use of well-established computational procedures.4 Basic information pertaining to crystal parameters and structure refinement is summarized in Table 1. The structure factors were obtained after Lorentz and polarization corrections. The positions of the heavy atoms, including the silver atoms, were located by the direct method. The remaining atoms were found in a series of alternating difference Fourier maps and least-squares refinements.5 The final residuals of the first refinement were R1 = 0.0381, wR2 = 0.918. The crystallographic procedures for 2–6 were similar to those for 1. Basic information pertaining to crystal parameters and structure refinement is summarized in Table 1.
Complex |
1
|
2
|
3
|
4
|
5
|
6
|
R
1 = Σ||Fo| − |Fc||/Σ|Fo|
wR
2 = [Σw(Fo2
−
Fc2)2/Σw(Fo2)2]1/2. w = 1/[σ2(Fo2) + (ap)2
+ (bp)], p = [max(Fo2 or 0) + 2(Fc2)]/3. a = 0.0166, b = 6.6321, 1; a = 0.0000, b = 2.8489, 2; a = 0.0218, b = 11.2470, 3; a = 0.0215, b = 3.5078, 4; a = 0.0206, b = 3.9005, 5; a = 0.0243, b = 49.8382, 6.
Goodness-of-fit = [Σw(|Fo2| − |Fc2|)2/Nobserved
−
Nparameters)]1/2.
|
Formula |
C8H10Ag2N6O4S |
C6H7.5AgClN4.5O4 |
C6H9AgN3O4 |
C6H9AgN4O3 |
C6H11AgClN3O5 |
C12H26Ag4N6O12S2 |
M
w
|
502.02 |
349.98 |
295.03 |
293.04 |
348.50 |
941.99 |
Crystal system |
Monoclinic |
Orthorhombic |
Orthorhombic |
Monoclinic |
Monoclinic |
Monoclinic |
Space group |
C2/c |
P21212 |
Ibam
|
P21/c |
P21/n |
P21/n |
a/Å |
13.689(3) |
9.9740(18) |
25.249(2) |
7.910(1) |
7.6708(6) |
10.727(2) |
b/Å |
8.3977(14) |
11.6687(14) |
6.2859(9) |
19.287(2) |
14.3064(17) |
17.216 (2) |
c/Å |
11.3004(14) |
9.2700(18) |
12.6954(13) |
12.2767(7) |
11.1349(7) |
13.462(3) |
β/° |
107.446(13) |
90 |
90 |
94.902(8) |
102.022(6) |
91.64(2) |
V/Å3 |
1239.3(4) |
1078.9(3) |
2014.9(4) |
1886.2(3) |
1195.16(19) |
2485.1(8) |
Z
|
4 |
4 |
8 |
8 |
4 |
4 |
D
calc/g cm−3 |
2.691 |
2.155 |
1.945 |
2.086 |
1.937 |
2.518 |
μ(Mo Kα)/mm−1 |
3.356 |
2.124 |
1.994 |
2.148 |
1.919 |
3.343 |
Range(2θ) for data collection/° |
5.76 ≤ 2θ
≤ 49.98 |
5.38 ≤ 2θ
≤ 50.04 |
6.42 ≤ 2θ
≤ 49.96 |
3.94 ≤ 2θ
≤ 49.98 |
4.70 ≤ 2θ
≤ 50.00 |
3.80 ≤ 2θ
≤ 50.04 |
Reflections collected |
1420 |
1525 |
1255 |
4290 |
2815 |
5420 |
Independent reflections |
1090 [R(int) = 0.0373] |
1389 [R(int) = 0.0281] |
932 [R(int) = 0.0185] |
3272 [R(int) = 0.0168] |
2091 [R(int) = 0.0148] |
4327 [R(int) = 0.0339] |
Data/restraints/parameters |
1090/0/117 |
1389/0/155 |
932/0/109 |
3272/0/265 |
2091/6/179 |
4327/0/333 |
Goodness-of-fit indicatorc |
1.046 |
1.018 |
1.030 |
1.048 |
1.035 |
1.066 |
final R indices [I > 2σ(I)]a,b |
R1 = 0.0223, wR2 = 0.0540 |
R1 = 0.0256, wR2 = 0.0524 |
R1 = 0.0258, wR2 = 0.0588 |
R1 = 0.0304, wR2 = 0.0680 |
R1 = 0.0375, wR2 = 0.0820 |
R1 = 0.0549, wR2 = 0.1203 |
R indices (all data) |
R1 = 0.0238, wR2 = 0.0553 |
R1 = 0.0259, wR2 = 0.0525 |
R1 = 0.0280, wR2 = 0.0603 |
R1 = 0.0381, wR2 = 0.0718 |
R1 = 0.0432, wR2 = 0.0852 |
R1 = 0.0740, wR2 = 0.1291 |
CCDC reference numbers 281177–281182. For crystallographic data in CIF or other electronic format see DOI: 10.1039/b511584a
Results and discussions
Syntheses
By layering the aqueous solution of the corresponding Ag(I) salt with L in ethanol, the complexes {[Ag2(L)2(SO4)]}∞, (L = 2-aminopyrimidine) 1, [Ag(L)1.5(ClO4)]∞, 2 and [Ag(L)(OAc)·2H2O]∞, 3, were prepared, while the complexes [Ag(L′)(NO3)]∞, 4, and [Ag(L′)(ClO4)·H2O]∞, 5, were prepared by refluxing AgNO3 and AgClO4, respectively, with 2-amino-4,6-dimethylpyrimidine (L′) in H2O. Reaction of Ag2SO4 with L′ in methanol afforded the complex [Ag4(L′)2(SO4)2(H2O)4]∞, 6. All the complexes have been structurally characterized by X-ray crystallography. Selected bond lengths and angles are listed in Table 2. Complexes 1–6 were insoluble in most of the organic solvents.
Table 2 Selected bond distances (Å) and angles (°) for complexes 1–6
Symmetry transformations used to generate equivalent atoms: (A) x, −y, z
− 1/2; (B) −x
− 1/2, y
− 1/2, −z
− 1/2, for 1. (A) −x
+ 3/2, y
− 1/2, −z
+ 2, for 2. (A) x, −y, −z
+ 1/2, for 3. (A) −x
+ 1, y
− 1/2, −z
+ 3/2, for 4. (A) x
− 1/2, −y
+ 3/2, z
− 1/2, for 5. (A) −x
+ 2, y
+ 1/2, −z
+ 5/2; (B) −x
+ 2, −y, −z
+ 2, for 6.
|
Complex 1 |
Distances
|
Ag–N(1) |
2.257(3) |
Ag–N(2B) |
2.506(3) |
Ag–N(3A) |
2.356(3) |
Ag–O(1) |
2.406(3) |
Angles
|
N(1)–Ag–N(3A) |
108.83(10) |
N(1)–Ag–O(1) |
138.46(10) |
N(1)–Ag–N(2B) |
119.26(10) |
O(1)–Ag–N(2B) |
86.43(9) |
N(3A)–Ag–N(2B) |
95.22(10) |
N(3A)–Ag–O(1) |
99.71(10) |
Complex 2 |
Distances
|
Ag–N(1) |
2.216(4) |
Ag–N(3A) |
2.302(4) |
Ag–N(4) |
2.372(4) |
|
|
Angles
|
N(1)–Ag–N(3A) |
142.9(2) |
N(3A)–Ag–N(4) |
95.9(1) |
N(1)–Ag–N(4) |
121.1(2) |
|
|
Complex 3 |
Distances
|
Ag–N(1) |
2.254(2) |
Ag–O(1) |
2.239(5) |
Angles
|
N(1)–Ag–N(1A) |
127.95(12) |
O(1)–Ag–N(1A) |
134.65(14) |
O(1)–Ag–N(1) |
96.43(14) |
O(1A)–Ag–O(1) |
43.6(2) |
Complex 4 |
Distances
|
Ag(1)–N(4) |
2.276(3) |
Ag(1)–N(1) |
2.280(3) |
Ag(1)–O(5) |
2.709(6) |
Ag(1)–O(6) |
2.666(4) |
Ag(2)–N(6) |
2.182(3) |
Ag(2)–N(3A) |
2.190(3) |
Ag(2)–O(3) |
2.590(3) |
|
|
Angles
|
O(5)–Ag(1)–N(1) |
133.88(12) |
O(5)–Ag(1)–N(4) |
94.67(12) |
O(6)–Ag(1)–N(1) |
98.85(11) |
O(6)–Ag(1)–N(4) |
133.40(11) |
O(6)–Ag(1)–O(5) |
46.51(10) |
N(4)–Ag(1)–N(1) |
127.33(10) |
N(3A)–Ag(2)–O(3) |
95.84(10) |
N(6)–Ag(2)–N(3A) |
156.40(10) |
N(6)–Ag(2)–O(3) |
101.26(10) |
|
|
Complex 5 |
Distances
|
Ag–N(1) |
2.179(3) |
Ag–N(3A) |
2.175(3) |
Angles
|
N(3A)–Ag–N(1) |
156.81(14) |
|
|
Complex 6 |
Distances
|
Ag(1)–N(1) |
2.202(7) |
Ag(3)–O(6B) |
2.448(8) |
Ag(1)–O(1) |
2.476(7) |
Ag(3)–O(7) |
2.265(7) |
Ag(1)–O(5) |
2.264(7) |
Ag(3)–O(1B) |
2.506(7) |
Ag(2)–O(2) |
2.353(8) |
Ag(4)–O(12) |
2.292(8) |
Ag(2)–O(9) |
2.425(7) |
Ag(4)–O(10) |
2.525(7) |
Ag(2)–N(3A) |
2.233(7) |
Ag(4)–N(6) |
2.242(8) |
Ag(2)–O(7B) |
2.381(7) |
Ag(4)–O(11) |
2.441(8) |
Ag(3)–N(4) |
2.252(8) |
|
|
Angles
|
N(1)–Ag(1)–O(5) |
155.0(3) |
O(7)–Ag(3)–O(1B) |
96.1(2) |
O(5)–Ag(1)–O(1) |
90.7(3) |
N(4)–Ag(3)–O(6B) |
99.7(3) |
N(1)–Ag(1)–O(1) |
103.7(3) |
N(4)–Ag(3)–O(1B) |
100.1(3) |
N(3A)–Ag(2)–O(7B) |
139.4(3) |
O(6B)–Ag(3)–O(1B) |
93.0(3) |
N(3A)–Ag(2)–O(9) |
105.4(2) |
N(4)–Ag(3)–O(7) |
151.7(3) |
O(7B)–Ag(2)–O(9) |
98.5(2) |
O(7)–Ag(3)–O(6B) |
102.4(3) |
N(3A)–Ag(2)–O(2) |
111.2(3) |
N(6)–Ag(4)–O(11) |
112.3(3) |
O(2)–Ag(2)–O(7B) |
86.0(3) |
N(6)–Ag(4)–O(10) |
103.5(3) |
O(2)–Ag(2)–O(9) |
115.9(3) |
O(11)–Ag(4)–O(10) |
116.6(3) |
O(12)–Ag(4)–O(11) |
88.8(3) |
N(6)–Ag(4)–O(12) |
147.0(3) |
O(12)–Ag(4)–O(10) |
87.8(2) |
|
|
Structure of 1
The crystals of complex 1 conform to the space group C2/c. The S-atom of the anion lies on the C2 axis and, hence, each asymmetric unit contains one Ag(I) cation, one L ligand and a half SO42− anion. Fig. 1a shows a molecular diagram about the Ag(I) metal center, which is coordinated by two pyrimidine nitrogen atoms, one amino nitrogen atom and one sulfate oxygen atom to form a distorted tetrahedral geometry. The largest N(1)–Ag–O(1) angle opened up to 138.46 (10)° from the ideal tetrahedral angle while the remaining angles are in the range from 86.43 (9) to 119.26 (10)°. Noticeably, the Ag–N distances to amino nitrogen atoms [Ag–N(2B) = 2.506 (3) Å] are significantly longer than those to the pyrimidyl nitrogen atoms [Ag–N(1) = 2.257 (3) and Ag–N(3A) = 2.356 (3) Å]. The L ligands are coordinated to the Ag(I) metal centers in new tridentate fashions to form 2-D pleated molecular sheets, Fig. 1b, which are interlinked by the SO42− anions [Ag–O(1) = 2.406 (3) Å] to form a 3-D structure, Fig. 1c. The SO42− anions are coordinated to the metal centers in a bidentate μ1-O, μ1-O′- bonding mode. It is seen from Fig. 1b that in each molecular sheets, the Ag ions and L ligands are interlinked to form eight-membered and sixteen-membered metallocycles. Inside the sixteen-membered metallocycles, the amino group of the L forms the hydrogen bonds with the oxygen atoms on the coordinated sulfate anion. Distances and angles for these N–H⋯O hydrogen bonds are O1A⋯H22 = 2.205 and O2⋯H21 = 2.033 Å; N2⋯O1A = 2.994 and N2⋯O2 = 2.824 Å, ∠N2–H22⋯O1A = 178.0 and ∠N2–H21⋯O2 = 170.5° (symmetry for A: x, −y, 0.5 +
z).
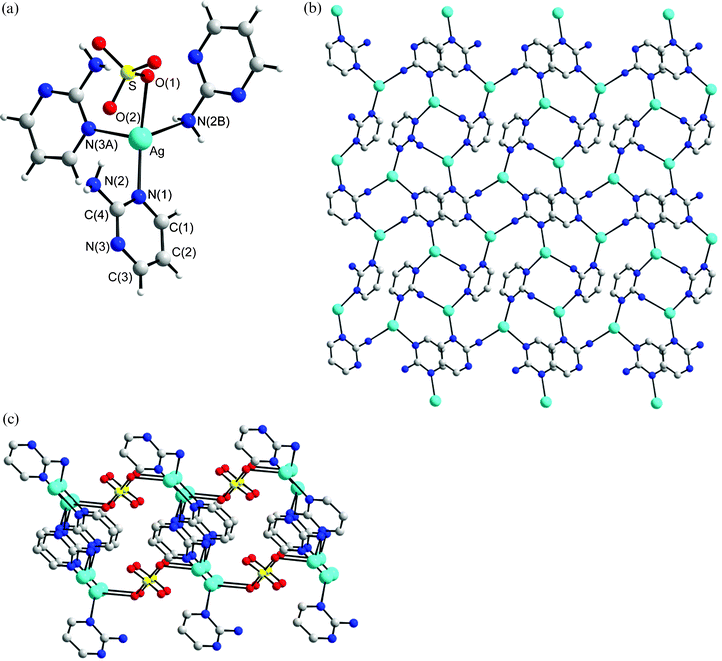 |
| Fig. 1 (a) A molecular diagram showing the geometry concerning the Ag(I) ion for 1. Symmetry for equivalent atoms: (A) x, −y, z
− 1/2; (B) −x
− 1/2, y
− 1/2, −z
− 1/2. (b) A packing diagram showing the 2-D molecular sheet. (c) The 2-D sheets are interlinked by the SO42− anions to form a 3-D structure. | |
Structure of 2
The crystals of complex 2 conform to the chiral space group P21212 (with the Flack parameter x = −0.03(6)). A crystallographic twofold axis passes through the C5, C7 and N5 atoms of one L ligand, and there is another independent L ligand in the lattice. Thus, each asymmetric unit contains one Ag(I) cation, one-and-a-half L ligands and one ClO4− anion. As illustrated in Fig. 2a, the Ag(I) ion, which is coordinated by three nitrogen atoms of three pyrimidine rings, has a distorted triangular planar geometry [∠N(1)–Ag–N(4) +
∠N(1)–Ag–N(3A) +
∠N(3A)–Ag–N(4) = 359.9°]. The single crystal X-ray structure shows that 2 is a pleated molecular sheet consisting of twenty four-membered metallocycles. The metallocycles involve six Ag ions which are bridged by six L ligands through the pyrimidyl nitrogen atoms, Fig. 2b. The ClO4− anions bridge adjacent sheets through series of C–H⋯O (O⋯H = 2.418 Å, C⋯O = 3.124 Å, ∠C–H⋯O = 131.8°) and N–H⋯O (O⋯H = 2.229 to 2.256 Å, N⋯O = 3.078 to 3.109 Å, ∠N–H⋯O = 159.7 to 166.8°) hydrogen bonds to form a 3-D structure.
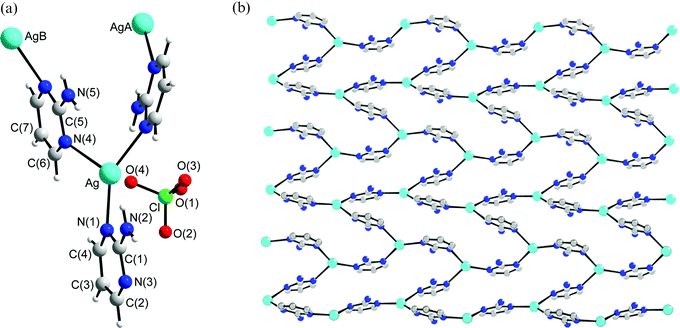 |
| Fig. 2 (a) A molecular diagram showing the geometry concerning the Ag(I) ion for 2. Symmetry for equivalent atoms: (A) −x
+ 3/2, y
− 1/2, −z
+ 2; (B) −x
+ 2, −1 +
y, z. (b) A packing diagram showing the 2-D molecular sheet. | |
Structure of 3
The crystals of complex 3 conform to the space group Ibam. Both Ag(I) cation and OAc− anion lie on the twofold axis that is parallel to the crystallographic a-axis and a crystallographic mirror plane bisects the L ligand. The OAc− anion is disordered due to the imposed symmetry. Each asymmetric unit, therefore, contains a half Ag(I) cation, a half L ligand, a half OAc− anion and one (in three different positions) uncoordinated H2O molecules. As illustrated in Fig. 3a, the Ag(I) ion, which is coordinated by two nitrogen atoms of two L ligands and one oxygen atom of the OAc− anion, has a distorted triangular planar geometry [∠N(1)–Ag–O(1) +
∠O(1)–Ag–N(1C) +
∠N(1)–Ag–N(1C) = 359.1°]. Fig. 3b shows that complex 3 forms linear chains with sinusoidal geometry, which are interlinked through extensive Ag⋯Ag interactions (Ag⋯Ag = 3.145 Å) to form 2-D wavy rectangular grids. The Ag⋯Ag distance is longer than that of the metallic Ag⋯Ag contact (2.89 Å), but shorter than the van der Waals contact distance (3.40 Å), indicating weak Ag⋯Ag interaction. The OAc− anions are coordinated to the Ag(I) ions in a monodentate fashion. In addition, the uncoordinated amino group on the L interacts with the OAc− anion through N–H⋯O hydrogen bonds (H⋯O = 1.907 and 2.111 Å, N⋯O = 2.769 and 2.951 Å, ∠N–H⋯O = 170.4 and 161.6°). The length and the width of the grid are 6.348 and 3.145 Å, respectively. The water molecules bridging adjacent grids also interact with the uncoordinated oxygen atoms of the OAc− anions through O–H⋯O (O⋯O = 2.793 Å) hydrogen bonds.
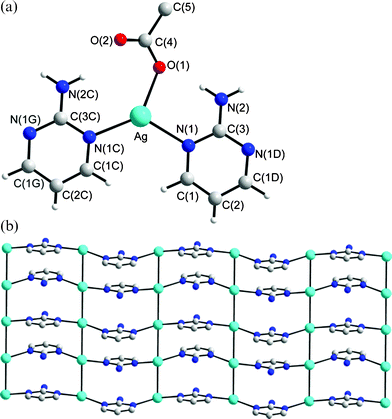 |
| Fig. 3 (a) A molecular diagram showing the geometry concerning the Ag(I) ion for 3. Symmetry for equivalent atoms: (C) x, −y, −z
+ 1/2; (D) x, y, −z; (G) x, −y, z
+ 1/2. (b) A packing diagram showing the wave-like 1-D chains, which are interlinked through extensive Ag⋯Ag interactions to form 2-D pleated rectangular grids. | |
Structure of 4
The crystals of complex 4 conform to the space group P21/c and each asymmetric unit contains two Ag(I) cation, two L′ ligands and two NO3− anions. While one anion is coordinated to the metal center in a monodentate fashion, the other is in a bidentate fashion. Fig. 4a shows the geometries concerning the Ag(I) atoms and Fig. 4b depicts the infinite helical structure of 4. The Ag metal centers possess two types of geometries. The Ag(1) atom adopts the distorted tetrahedral geometry in which two nitrogen atoms of two L′ ligands and two oxygen atoms of separate NO3− anions coordinate the metal center. The largest N(1)–Ag–O(5) angle opens up to 133.88 (12)° from the ideal tetrahedral angle while the remaining angles are in the range from 46.51 (10) to 133.40 (11)°. The O(6)–Ag(1)–O(5) angle of 46.51 (10)° is presumably due to the chelation of the NO3− anion. The Ag(2) atom adopts the distorted triangular geometry [∠N(6)–Ag(2)–O(3) +
∠N(6)–Ag(2)–N(3A) +
∠N(3A)–Ag(2)–O(3) = 353.51°], in which the metal is coordinated by the nitrogen atoms of two L ligands and one oxygen atom of the anion. Fig. 4b shows that 4 is a single-strained helical coordination polymer, which is interlinked through a series of Ag⋯O interactions [2.666(4) and 2.709(6) Å] to the oxygen atoms of the bidentate NO3− anions, forming 2-D molecular sheets. Fig. 4c shows the diagram of the Ag⋯O interactions where two Ag atoms are doubly bridged and chelated by two oxygen atoms of two separate NO3− anions. The NO3− anion coordinating to the Ag(1) atom thus adopts a μ1-O, μ2-O′ tridentate bonding mode, while the other coordinating the Ag(2) atom adopts a μ1-O monodentate mode. In this complex, the amino group of the L′ ligand interacts with the NO3− anions through N–H⋯O hydrogen bonds (H⋯O = 2.006 to 2.564 Å, N⋯O = 2.869 to 3.307 Å, ∠N–H⋯O = 143.6 to 164.7°).
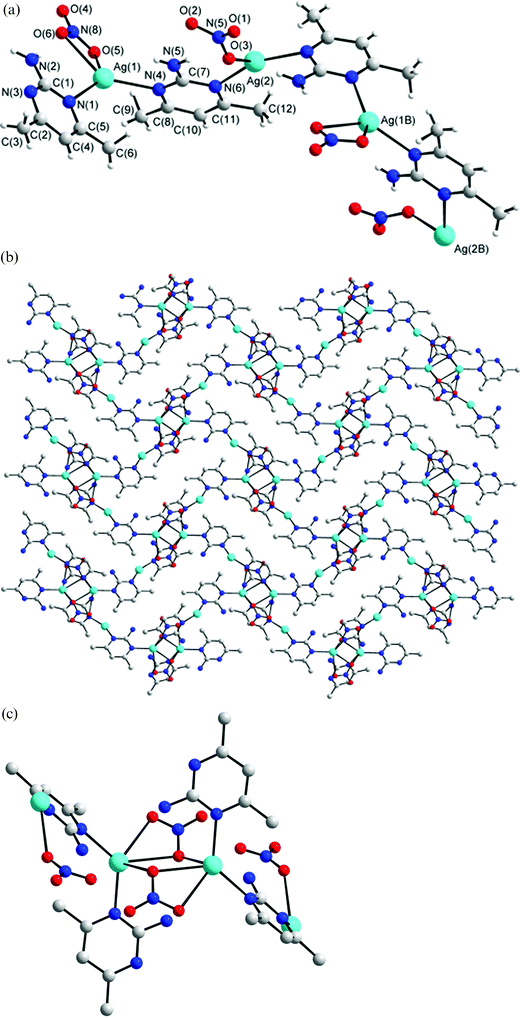 |
| Fig. 4 (a) Molecular diagram showing the geometry about the Ag(I) ions for 4. Symmetry for equivalent atoms: (B) 1 −
x, ½
−
y, 3/2 −
z. (b) Packing diagram showing the 1-D helical structure linked by a series of Ag⋯O interactions. (c) The Ag⋯O interactions that interlink the helical chains. | |
Structure of 5
The crystals of complex 5 conform to the space group P21/n and each asymmetric unit contains one Ag(I) cation, one L′ ligand, one ClO4− anion and one H2O molecule, Fig. 5a. The structure of complex 5 shows that the silver atom is bound to the nitrogen atoms of two symmetry-related L′ ligands [Ag–N (1) = 2.179 (3), Ag–N(3A) = 2.175 (3) Å] in a distinctly non-linear geometry [N(1)–Ag–N(3A) = 156.81 (14)°], forming zig-zag chains, Fig. 5b. The deviation from linear geometry is due to the weak interaction between Ag atom and O atoms on the solvent molecule (Ag–O5 = 2.623 (4) Å). The dihedral angle between two adjacent pyrimidyl rings is 105.6°. The ClO4− anions and solvent water molecules bridge an adjacent chain through series of N–H⋯O (O⋯H = 2.193 to 2.271 Å, N⋯O = 2.970 to 3.027 Å, ∠N–H⋯O = 171.9 to 172.7°) and O–H⋯O (O⋯H = 1.998 to 2.090 Å, O⋯O = 2.853 to 3.032 Å, ∠O–H⋯O = 170.0 to 177.2°) hydrogen bonds and Ag⋯O interactions (Ag⋯O = 2.623 Å for water solvent molecule and 2.836 Å for anion).
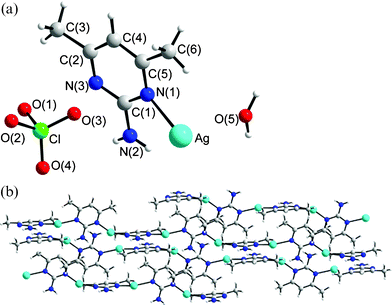 |
| Fig. 5 (a) The asymmetric unit for 5. (b) Packing diagram showing the packing of the zig-zag chains. | |
Structure of 6
Crystals of complex 6 conform to the space group P21/n. The molecular diagrams showing the asymmetric unit of complex 6 and its packing diagram are depicted in Fig. 6a–6c. The structure of 6 consists of clusters of the formula [Ag8(L′)4(SO4)4(H2O)8], Fig. 6b, which are interlinked through L′ ligands to form 2-D sheets, Fig. 6c. This sheet structure is further extended to 3-D network by series of N–H⋯O (O⋯H = 2.102 to 2.204 Å, N⋯O = 2.889 to 3.022 Å, ∠N–H⋯O = 132.3 to 163.0°) and O–H⋯O (O⋯O = 2.715 to 2.914 Å) hydrogen bonds. Two types of coordination geometries are observed for the four independent Ag metal centers. The Ag(1) ion which is coordinated by one nitrogen atom of the L′ ligand and two oxygen atoms is distorted triangular planar while the other three which are coordinated by one nitrogen atoms of the L′ ligand and three oxygen atoms are distorted tetrahedral. The oxygen atoms are either from the H2O molecule or SO42− anion. Two types of SO42− anions are found in the cluster. The first type adopts the μ1-O, μ2-O′- bonding mode to bridge three Ag(I) ions, while the other adopts the μ1-O, μ1-O′, μ2-O″- bonding mode to bridge four Ag(I) atoms. The four Ag(I) atoms bridged by the μ4-SO42− anions form a rectangular plane, with the length and width of 3.661 [Ag(2)⋯Ag(3A)]and 3.353 [Ag(2)⋯Ag(3)] Å, respectively. While the shorter distance shows weak Ag⋯Ag interaction, the Ag⋯Ag distance of 3.661 Å is too long for any metal–metal interaction. Coordination polymers with discrete silver clusters and organic spacers are very rare and only two related compounds, [Ag2{OOC–(CH2)2–COO}]∞6 and [Ag8(μ4-SO4)4(2,2′-bpe)5]·10 H2O [2,2′-bpe = 2,2′bis(2-pyridyl)ethane],7e were reported. Complex 6 makes interesting comparisons with [Ag8(μ4-SO4)4(2,2′-bpe)5]·10 H2O. While the SO42− groups in 6 adopt both of the μ1-O, μ2-O′- and μ1-O, μ1-O′, μ2-O″- bonding modes, those in [Ag8(μ4-SO4)4(2,2′-bpe)5]·10 H2O adopt the same μ1-O, μ1-O′, μ2-O″- bonding mode.
![(a) The asymmetric unit for 6. Symmetry for equivalent atoms: (A) 2 −
x, −y, 2 −
z. (b) A molecular diagram showing the cluster of the formula [Ag8(L′)4(SO4)4(H2O)8]. (c) The clusters are interlinked by the L′ ligands to for a 2-D pleated structure.](/image/article/2006/CE/b511584a/b511584a-f6.gif) |
| Fig. 6 (a) The asymmetric unit for 6. Symmetry for equivalent atoms: (A) 2 −
x, −y, 2 −
z. (b) A molecular diagram showing the cluster of the formula [Ag8(L′)4(SO4)4(H2O)8]. (c) The clusters are interlinked by the L′ ligands to for a 2-D pleated structure. | |
Ag(I) complexes containing SO42− anions are rare. In these reported complexes, the SO42− anions adopt the μ1-O,7a,b,f,g
μ1-O, μ1-O′7c,h and μ1-O, μ1-O′, μ2-O″7d,e- bonding modes, respectively. The μ1-O, μ2-O′- bonding mode in complex 6 is thus unique for Ag(I) complexes.
Bonding modes of L and L′
The neutral L and L′ ligands have been subjected to several studies of its coordination ability to metal centers. Monodentate8–10 and bidentate11–13 coordination modes (Scheme 1) were seen for these neutral ligands. In the monodentate mode of coordination, I, the nitrogen atom of the amino group is bonded to the metal. Such coordination was found, for example, in the mononuclear complex [Ru(L)(NH3)5](PF6)2.8 One of the pyrimidyl nitrogen atoms of the L ligand in the complex, Cu(L)(2,6-dapy)·3H2O (2,6-dapy = pyridine-2,6-dicarboxylato)9c and that of the L′ ligand in the dinuclear complex Rh2(O2CCH3)4(L′)2,10a is coordinated to the metal centers, II. The coordination mode, III, which features chelation through one amine nitrogen atom and one pyrimidyl nitrogen atom, was found in trans-Ni(SCN)2(L)211c and in the complex trans-ZnCl2(L′)2.12 In the dinuclear complex, Zn2(DPD)(L) (DPD = diporphyrin dibenzofuran),13c the L ligand is bridged to the metal centers through the two pyrimidyl nitrogen atoms, IV, while in the dinuclear complexes Pt2(NO3)4(L)2,14b the L ligand is bridged to the metal center through one amino nitrogen atom and one pyrimidyl nitrogen atom, V. Complex 1 is thus the first complex containing L ligand which is bridged to the metal centers in a tridentate fashion, VI. Different bonding modes for anionic L′15,16 and for π-bonded L17 have also been reported. Noticeably, only two Ag(I) complexes containing L and L′, [{Ag(pts)}(L)]n (pts = p-toluene sulfonate) and [{Ag(pts)}2(L′)]n, respectively, are known,18 where both L and L′ adopt the bonding mode IV.
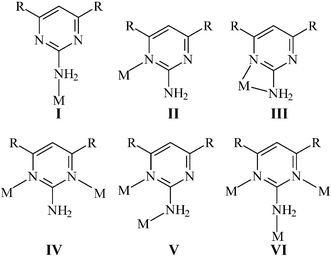 |
| Scheme 1 The bonding modes of the neutral L and L′ ligands and their anions. | |
Complexes 1–6 make interesting comparisons with the Ag(I) complexes containing the pyrimidine ligand which has no amino group.19 All the pyrimidine ligands in the complexes reported19 act as bidentate N,N′-donor ligands, similar to the bonding mode IV in Scheme 1. While tetracationic squares were found in the complexes [Ag(pyrimidine)][NO3]19a,b, [Ag(pyrimidine)][ReO4],19a,b [Ag2.5(pyrimidine)3][ClO4]2.519b and [Ag(pyrimidine)1.5][BF4],19b tetracationic rectangles were seen in complex 1, presumably due to the coordination of the amino group of the L ligand to the Ag(I) ion. The structural characteristics in complexes 1–6 may, in part, be ascribed to the N–H⋯O interactions between the amino groups of pyridyl ligands and the anions
Thermal properties
The Tm values of complexes 1–6 have been measured by using differential scanning calorimetry (DSC), which are 302.4, 267.4, 223.9, 218.7, 224.7 and 330.3 °C, respectively. The Tm values of complexes 1 and 6 are significantly larger than those of the others, presumably due to the formation of the 3-D and 2-D frameworks, respectively, supported by the SO42− anions.
The thermogravimetric analyses (TGA) have been performed on compounds 1–5 by heating each compound to 600 °C, while compound 6 was heated to 1000 °C in an atmosphere of flowing nitrogen. TGA for 1 showed the loss of pyrimidine rings in the range 202–278 °C (calculated 37.9%, observed 37.1%), followed by the loss of the SO42− anions above 278 °C. TGA for 2 shows that the ClO4− anions were lost in the temperature range 94–133 °C (calculated 28.4%, observed 29.1%). Above 133 °C, the frameworks of compound 2 decomposed. TGA for 3 showed the first loss took place between 89–149 °C and was associated with the loss of the H2O molecules (calculated 12.2%, observed 12.4%). The second weight loss took place between 149–200 °C, corresponding to the loss of the OAc− anions (calculated 20.4%, observed 20.2%). Above 200 °C, the pyrimidine rings decomposed (calculated 32.2%, observed 31.8%). TGA for 4 showed the loss of the NO3− anions and the methyl groups of ligand in the range 179–223 °C (calculated 31.4%, observed 31.3%), followed by the loss of the pyrimidine rings above 223 °C. TGA for 5 showed that the first loss took place between 153–225 °C and was associated with the loss of the H2O molecules (calculated 5.2%, observed 4.9%). The second loss took place between 225–250 °C, corresponding to the loss of the ClO4− anions and the methyl groups of ligands (calculated 37.1%, observed 37.2%). The loss of the pyrimidine rings took place above 250 °C. TGA for 6 showed the first loss took place between 144–207 °C and was associated with the loss of the H2O molecules (calculated 7.6%, observed 7.3%). The second loss took place between 207–550 °C, corresponding to the loss of the SO42− anions (calculated 20.4%, observed 20.5%), while the weight loss between 550–900 °C (instrument limitation) is 7.7%, indicating that complex 6 is thermally stable.
Concluding remarks
In this study, the coordination chemistry of Ag(I) salts with the rigid ligands L and L′ was investigated. Six polymeric complexes involving 1-D, 2-D and 3-D frameworks were obtained. Table 3 shows the cation–anion interaction, metal geometry and structure in complexes 1–6. It was shown that the self-assembly frameworks of these Ag(I) coordination polymers can be drastically altered by changing their counter ions and the nature of the rigid ligand has also significant effect on the structure. It was also shown that the coordination ability of the ClO4− anion to the Ag(I) atom is weaker than the other anions, whereas the bonded oxygen atom of the OAc− ligand shows shortest distance to the Ag(I) atom. The L ligand in complex 1 adopts the unique tridentate bonding mode and the μ1-O, μ2-O′- bonding mode of the SO42− anion in complex 6 is also unique for Ag(I) complexes. The 3-D and 2-D frameworks of 1 and 6, respectively, which are supported by the SO42− anions show better thermal stabilities.
Table 3 Cation–anion interaction, metal geometry and structure in complexes 1–6
Complex |
Anion |
Metal geometry |
Structure |
Cation–anion interaction |
1
|
SO42− |
Tetrahedral |
3-D network |
Ag–O = 2.407 (3) Å, N–H⋯O hydrogen bond |
2
|
ClO4− |
Trigonal planar |
2-D sheet |
N–H⋯O and C–H⋯O hydrogen bonds |
3
|
OAc− |
Trigonal planar |
1-D linear chain |
Ag–O = 2.239 (5) Å, N–H⋯O hydrogen bond |
4
|
NO3− |
Tetrahedral |
1-D helical chain |
Ag–O = 2.666(4), 2.709(6) and 2.590(3) Å, N–H⋯O hydrogen bond |
Trigonal planar |
5
|
ClO4− |
Linear |
1-D zigzag chain |
N–H⋯O hydrogen bond, Ag–O = 2.836 Å |
6
|
SO42− |
Trigonal planar |
2-D sheet |
Ag–O = 2.476 (7), 2.353 (8), 2.265 (7), 2.425 (7) Å, N–H⋯O hydrogen bond |
Tetrahedral |
Acknowledgements
We are grateful to the National Science Council of the Republic of China for support.
References
-
(a) G. R. Desiraju, Angew. Chem., Int. Ed. Engl., 1995, 34, 2311 CrossRef CAS;
(b) G. R. Desiraju, Chem. Commun., 1997, 1475 RSC;
(c)
J. M. Lehn, Supramolecular Chemistry, VCH, Weinheim, 1995 Search PubMed;
(d) M. Nishio, CrystEngComm, 2004, 6, 130 RSC.
-
(a)
R. Robson, B. E. Abrahams, S. R. Batten, R. W. Gable, B. F. Hoskins and J. Lieu, Supramolecular Architecture, ACS publications, Washington, DC, 1992 Search PubMed;
(b) S. R. Batten and R. Robson, Angew. Chem., Int. Ed., 1998, 37, 1460 CrossRef;
(c) O. M. Yaghi, H. Li, C. Davis, D. Richardson and T. L. Groy, Acc. Chem. Res., 1998, 31, 474 CrossRef CAS;
(d) H. Gudbjartson, K. Biradha, K. M. Poirier and M. J. Zaworotko, J. Am. Chem. Soc., 1999, 121, 2599 CrossRef CAS;
(e) C. B. Aakeröy and K. R. Seddon, Chem. Soc. Rev., 1993, 397 RSC;
(f) M. Fujita and K. Ogura, Coord. Chem. Rev., 1996, 148, 249 CrossRef CAS;
(g) S.-I. Noro, R. Kitauta, M. Kondo, S. Kitagawa, T. Ishii, H. Matauzaka and M. Yamashita, J. Am. Chem. Soc., 2002, 124, 2568 CrossRef CAS;
(h) S. Kitagawa and M. Kondo, Bull. Chem. Soc. Jpn., 1998, 71, 1739 CAS.
-
(a) A. J. Blake, N. R. Champness, P. Hubberstey, W.-S. Li, M. A. Withersby and M. Schröder, Coord. Chem. Rev., 1999, 183, 117 CrossRef CAS;
(b) S. Muthu, J. H. K. Yip and J. J. Vittal, J. Chem. Soc., Dalton Trans., 2002, 4561 RSC and references therein;
(c) Y.-F. Hsu and J.-D. Chen, Eur. J. Inorg. Chem., 2004, 1488 CrossRef CAS;
(d) H.-L. Hu, C.-W. Yeh and J.-D. Chen, Eur. J. Inorg. Chem., 2004, 4696 CrossRef CAS.
-
XSCANS, Release, 2.1, Siemens Energy & Automation, Inc, Madison, Wisconsin, USA, 1995 Search PubMed.
-
SHELXTL 5.10, Bruker Analytical X-ray Instruments Inc., Karlsruche, Germany, 1997 Search PubMed.
- A. Michaelides, V. Kiritsis, S. Skoulika and A. Aubry, Angew. Chem., Int. Ed. Engl., 1993, 32, 1495 CrossRef.
-
(a) M.-L. Tong, S.-L. Zheng and X.-M. Chen, Chem. Commun., 1999, 561 RSC;
(b) R. L. LaDuca, Jr., R. S. Rarig, Jr., P. J. Zapf and J. Zubieta, Solid State Sci., 2000, 2, 39 CrossRef;
(c) G. A. Bowmaker, J. V. Hanna, C. E. F. Richard and A. S. Lipton, J. Chem. Soc., Dalton Trans., 2001, 20 RSC;
(d) N. R. Brooks, A. J. Blake, N. R. Champness, J. W. Cunningham, P. Hubberstey, S. J. Teat, C. Wilson and M. Schroder, J. Chem. Soc., Dalton Trans., 2001, 2530 RSC;
(e) M.-L. Tong, J.-X. Shi and X.-M. Chen, New J. Chem., 2002, 26, 814 RSC;
(f) M.-L. Tong, S.-L. Zheng and X.-M. Chen, Chem.-Eur. J., 2000, 6, 3729 CrossRef CAS;
(g) J. A. R. Navarro, J. M. Salas, M. A. Romero and R. Faure, J. Chem. Soc., Dalton Trans., 1998, 901 RSC;
(h) L. Brammer, C. S. Rodger, A. J. Blake, N. R. Brooks, N. R. Champness, J. W. Cunningham, P. Hubberstey, S. J. Teat, C. Wilson and M. Schroder, J. Chem. Soc., Dalton Trans., 2002, 4134 RSC.
- K. J. LaChance-Galang, I. Maldonado, M. L. Gallagher, W. Jian, A. Prock, J. Chacklos, R. D. Galang and M. J. Clarke, Inorg. Chem., 2001, 40, 485 CrossRef CAS.
-
(a) J.-H. P. Lee, B. D. Lewis, J. M. Mendes, M. M. Turnbull and F. F. Awwadi, J. Coord. Chem., 2003, 56, 1425 CrossRef CAS;
(b) S. Akyuz, Supramol. Chem., 2003, 2, 401;
(c) E. Altin, R. Kirchmaier and A. Lentz, Z. Kristallogr., 2004, 219, 35 CAS.
-
(a) K. Aoki, M. Inaba, S. Teratani, H. Yamazaki and Y. Miyashita, Inorg. Chem., 1994, 33, 3018 CrossRef CAS;
(b) D. Kovala-Demertzi, Transition Met. Chem., 1990, 15, 23 CrossRef CAS;
(c) D. Kovala-Demertzi, Bull. Chem. Soc. Jpn., 1991, 64, 744 CAS.
-
(a) P. P. Singh, J. N. Seth and S. A. Khan, Inorg. Nucl. Chem. Lett., 1975, 11, 525 CrossRef CAS;
(b) P. P. Singh, U. P. Shukla and J. N. Seth, Ind. J. Chem., Sect. A, 1976, 14A, 684 CAS;
(c) P. Lumme, H. Elo and J. Janne, Inorg. Chim. Acta, 1984, 92, 245.
- S.-P. Chen, Q. Wei, B.-J. Jiao and S.-L. Gao, Wuji Huaxue Xuebao, 2003, 19, 885 Search PubMed.
-
(a) Q.-H. Jin, X.-M. Lu, H.-J. Zhu and X.-P. Ji, Chin. J. Chem., 2000, 18, 928 CAS;
(b) A. I. Stetsenko, K. I. Yakovlev and A. L. Vais, Koord. Khim., 1979, 5, 1229 CAS;
(c) C. J. Chang, Z.-H. Loh, Y. Deng and D. G. Nocera, Inorg. Chem., 2003, 42, 8262 CrossRef CAS.
-
(a) L. Horner and E. Pliefke, Z. Naturforsch., B, 1981, 36B, 989 CAS;
(b) A. I. Stetsenko, K. I. Yakovlev and A. L. Iais, Koord. Khim., 1979, 5, 1129.
- D. R. Armstrong, F. Benevelli, A. D. Bond, N. Feeder, E. A. Harron, A. D. Hopkins, M. McPartlin, D. Moncrieff, D. Saez, E. A. Quadrelli, A. D. Woods and D. S. Wright, Inorg. Chem., 2002, 41, 1492 CrossRef CAS.
- C. Kachi-Terajima, H. Miyasaka, T. Ishii, K. Sugiura and M. Yamashita, Inorg. Chim. Acta, 2002, 332, 210 CrossRef CAS.
- R. E. Shepherd and S. Zhang, Transition Met. Chem., 1994, 19, 146 CAS.
- G. Smith, B. A. Cloutt, D. E. Lynch, K. A. Byriel and C. H. L. Kennard, Inorg. Chem., 1998, 37, 3236 CrossRef CAS.
-
(a) C. V. K. Sharma, S. T. Griffin and R. D. Rogers, Chem. Commun., 1998, 215 RSC;
(b) C. V. K. Sharma and R. D. Rogers, Cryst. Eng., 1998, 1, 18;
(c) H. Cai, H.-M. Hu, W.-Z. Chen, H.-G. Zhu and X.-Z. You, Chem. Lett., 1999, 221 CrossRef CAS.
|
This journal is © The Royal Society of Chemistry 2006 |
Click here to see how this site uses Cookies. View our privacy policy here.