DOI:
10.1039/B413554G
(Emerging Area)
Org. Biomol. Chem., 2005,
3, 15-19
New chiral anion mediated asymmetric chemistry†
Received
6th September 2004
, Accepted 29th October 2004
First published on 29th November 2004
Abstract
Chemical reactions and processes often involve chiral, yet racemic, cationic reagents, intermediates or products. To afford instead non racemic or enantiopure compounds, an asymmetric ion pairing of the cations with enantiopure anions can be considered—the counter ions behaving as asymmetric auxiliaries, ligands or reagents. Detailed herein is a short review of our approach towards gaining reliable and predictable control over stereoselective ion pairing phenomena through the synthesis and the use of novel configurationally stable hexacoordinated phosphate anions.
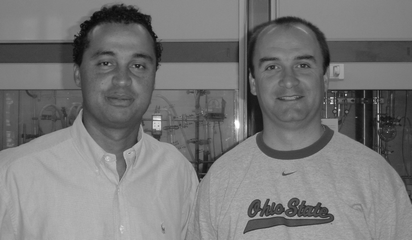 Jérôme Lacour (left) and Richard Frantz (right) | Jérôme Lacour was educated at the Ecole Normale Supérieure (Ulm, Paris) and obtained in 1993 his PhD in Chemistry at the University of Texas at Austin under the supervision of Professor Philip D. Magnus. After post-doctoral studies in the laboratory of Professor David A. Evans at Harvard University, he joined the Organic Chemistry Department of the University of Geneva in 1995 as a Maitre-Assistant (lecturer). He now holds a full professor position in the department. |
| Richard Frantz received his Doctorate in Chemistry from Université Montpellier II in 2001 under the supervision of Dr Gerard Lanneau. After post-doctoral studies in the laboratory of Professor Antonio Togni at ETH Zürich, he joined in 2003 the group of Professor Lacour as a post-doctoral assistant. |
Introduction
Cations, ubiquitous in coordination, organic, organometallic, and supramolecular chemistry, are often involved in chemical reactions and processes as reagents, intermediates or products. Cations are frequent intermediates along reaction pathways that react with nucleophiles to produce interesting fragments and functional groups. Cations are often Lewis acidic and numerous applications have been developed using these reagents. A large range of important synthetic and biological processes are mediated by ammonium and imidazolium ions. Cations are also efficient templates for the construction of complex supramolecular arrays, such as catenanes, knots, helicates, etc.
For our purpose, cations can also be chiral and many of the above-mentioned applications lead to racemic molecular or supramolecular assemblies. To afford instead non-racemic or enantiopure adducts, and benefit from new possible applications, a stereoselective ion pairing of these cations with enantiopure anions can be considered—the counter ions behaving as asymmetric auxiliaries, ligands or reagents.1 A wealth of evidence suggests that an ion electrostatically removed from its counterion is never formed in low-polarity solvents but, instead, an ion pair is produced. The association of racemic cations with enantiopure counterions leads to the formation of diastereomeric ion pairs.2 As a result, large chemical and physical differences can happen among the salts of the tightly associated ions.
In early approaches, chiral anions issued or derived from the chiral pool have been essentially considered. Numerous applications have been developed, especially in the field of enantiomeric resolutions.3 Today, these anions are still used with much success.1a Recent developments in this field have, however, also made use of new synthetic anions.1 This review will thus survey our efforts towards the preparation and the use of chiral hexacoordinated phosphates as anionic auxiliaries and reagents. Applications of these moieties as NMR chiral solvating reagents, as resolving agents for organic and inorganic cations and as chiral auxiliaries for stereoselective processes will be presented.
Enantiopure hexacoordinated phosphorus anions
The octahedral geometry of pentavalent hexacoordinated phosphorus allows the formation of chiral anions by complexation of a central phosphorus atom with three identical dianionic bidentate ligands. These compounds exist as Λ or Δ enantiomers with left- and right-handed propeller shape (M and P helicity) respectively (Fig. 1).4
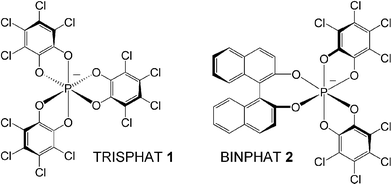 |
| Fig. 1 Hexacoordinated phosphate anions TRISPHAT 1 and BINPHAT 2; Λ and Δ enantiomers respectively. | |
Tris(benzenediolato)phosphate anion, of particular interest for its easy preparation from catechol, PCl5 and an amine, is unfortunately configurationally labile in solution as an ammonium salt, due to an acid-induced racemization mechanism.5 Previously, we could show that the introduction of electron-withdrawing chlorine atoms on the aromatic nuclei increases the configurational stability of the resulting tris(tetrachlorobenzenediolato)phosphate(V) derivative.6,7 This D3-symmetric anion 1, known as TRISPHAT, can be resolved by association with a chiral ammonium cation.
A general and efficient one-pot process was also developed for the preparation of C2-symmetric enantiopure anions, namely BINPHAT 2, HYPHAT, and TARPHAT, containing BINOL, hydrobenzoin and tartrate ligands respectively;8 all these anions being isolated as their dimethylammonium salts in good yields and chemical purity.
Finally, mannose derived hexacoordinated phosphate anion 3, prepared in two steps from commercially available materials, is an interesting alternative to 1 and 2. It is highly chemically stable, rapidly and stereoselectively synthesized and asymmetrically efficient with both organic and metallo-organic cations (see below).9
For NMR enantiomeric purity determination
As mentioned, chiral cations are involved in many areas of chemistry and, unfortunately, only few methods are available to determine with precision their optical purity. In the last decades, NMR has evolved as one of the methods of choice for the measurement of the enantiomeric purity of chiral species and,10 over the past few years, we could demonstrate that anions 1, 2 and 3 are efficient NMR chiral solvating agents. They form tightly associated diastereomeric ion pairs with chiral cations and the short-range interactions that occur lead to efficient NMR enantiodifferentiations. Well separated signals are usually observed on the spectra of the diastereomeric salts. In Fig. 2 are represented some organic cations that have been analyzed with success: quaternary ammonium 4, [4]-helicenium 5
(see also Fig. 3), thiiranium 6, phosphonium 7.11 Enantiodifferentiation of ruthenium(II)
8 and (η6-arene)manganese 9 complexes was also achieved.121H, 31P and 15N NMR spectroscopy can be used in these studies. The latter method proved to be particularly useful to distinguish the D2 from the S4 symmetry of chiral cation 10
(Fig. 4).13 TRISPHAT anion 1 seems to be more efficient with cationic metallo-organic and organometallic substrates. BINPHAT 2 has often-superior chiral shift properties when associated with organic cations. Recently, several independent reports have confirmed the efficiency of these chiral shift agents.14
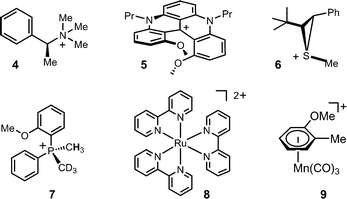 |
| Fig. 2 Examples of chiral cations efficiently analyzed with anions 1 or 2. | |
![1H NMR spectra (parts, 400 MHz, δ 8.16–6.25 ppm) in CDCl3 of (a)
[rac-5][BF4]; (b)
[5][Δ-2], dr 1 : 1; (c)
[P-5][Δ-2], dr > 49 : 1. Plain and dashed arrows indicate some of the signals of [P-5][Δ-2] and [M-5][Δ-2] salts respectively. □ represents the signals of anion 2.](/image/article/2005/OB/b413554g/b413554g-f3.gif) |
| Fig. 3
1H NMR spectra (parts, 400 MHz, δ 8.16–6.25 ppm) in CDCl3 of (a)
[rac-5][BF4]; (b)
[5][Δ-2], dr 1 : 1; (c)
[P-5][Δ-2], dr > 49 : 1. Plain and dashed arrows indicate some of the signals of [P-5][Δ-2] and [M-5][Δ-2] salts respectively. □ represents the signals of anion 2. | |
![1H and 15N NMR (500 MHz, CD2Cl2 parts, 233K) of salts of the highly symmetric bis(dibenzoazepinium) cation: (a)
[10][rac-1], CD2Cl2; (b)
[10][Δ-2], CD2Cl2, dr 1.6 : 1.](/image/article/2005/OB/b413554g/b413554g-f4.gif) |
| Fig. 4
1H and 15N NMR (500 MHz, CD2Cl2 parts, 233K) of salts of the highly symmetric bis(dibenzoazepinium) cation: (a)
[10][rac-1], CD2Cl2; (b)
[10][Δ-2], CD2Cl2, dr 1.6 : 1. | |
Furthermore, in collaboration with the group of Professor Kündig (Geneva) and Drs Djukic and Pfeffer (Strasbourg), it was recently shown that anions 1 and 2 can be used to determine the enantiomeric purity of planar chiral (η6-arene)chromium and palladium complexes. This result broadens the field of application of the anions to neutral species.15
For chiral resolution
The lipophilicity of TRISPHAT anion 1 confers to its salts an affinity for organic solvents and, once dissolved, the ion pairs do not partition in aqueous layers. This rather uncommon property was used to develop a practical resolution procedure of racemic cationic substrates by preferential extraction of one enantiomer from water into immiscible organic solvents.16 Ruthenium(II) tris(diimine) derivatives of type 8 were selected for high water solubility as chloride salts.17 Selectivity ratios as high as 35 : 1 were obtained for the enantiomers of the cations in the organic and aqueous layers demonstrating the efficiency of the process. The extraction and the resulting selectivity arise from the preferential binding in the organic phase of one enantiomer of the substrate with the lipophilic selector 1. An extension of this protocol was developed for a diiron(II) triple helicate and afforded in separated phases the P or M enantiomers of the [Fe2L3]4+ helix.18
The lipophilicity of anion 1 also modifies profoundly the chromatographic properties of the cations associated with it and the resulting ion pairs are usually poorly retained on polar chromatographic phases.19 Using enantiopure TRISPHAT anion, the chromatographic resolution of chiral cations is feasible as the diastereomeric ion pairs often possess different retardation factors. The resolution is best performed on preparative thin-layer chromatographic plates and it is more particularly effective with coordination complexes.20
Finally, resolution of chiral cations by selective precipitation of one diastereomeric salt is, of course, still a possibility. TRISPHAT 1 was recently used in such a manner with success by the groups of Amouri, Gruselle, Hamelin and Fontecave for the resolution of metal-containing moieties.21 BINPHAT 2 was more efficient than TRISPHAT for the isolation of enantiopure helicenium cation 5
(Fig. 3).11
For supramolecular stereocontrol
Chiral compounds are sometimes configurationally stable as solids and configurationally labile in solution. When optically active samples of these derivatives are solubilized, a racemization occurs due to the free interconversion of the enantiomers in solution. To obtain these compounds in one predominant configuration over time, one strategy is to add stereogenic elements to their backbone; intramolecular diastereoselective interactions happen and favor one of the equilibrating diastereomers. If the chiral compounds are charged, an alternative strategy is to consider their ion pairing with chiral counter-ions; intermolecular- rather than intramolecular-diastereoselective interactions then control the stereoselectivity (Pfeiffer effect).22
The induction of optical activity by chiral anions onto cationic racemic substrates has been previously considered.23 Unfortunately, in most of these and other previous examples, the extent of the asymmetry-induction was determined by chiroptical measurements (ORD, CD) that gave qualitative and not exact quantitative information. The NMR chiral shift efficiency of anions 1, 2 and 3 is therefore an excellent analytical tool to provide accurate measurement of the induced selectivity.
Monomethine dyes 11, diquats 12 and configurationally labile ammonium cation 10 were thus studied with success in conjunction with anions 1 and 2
(Fig. 5).8a,9,13,24 Copper(I) bis(diimine) complexes 13, iron(II) tris(diimine) moieties such as compound 14
(Fig. 6), and dicobalt(II) helicate 15 were also paired with the chiral counterions.9,20c,25 The NMR signals of the chiral cations were split by the presence of the anions and diastereomeric ratios up to 49 : 1 were measured for some of the substrates (Fig. 6). The existence of Pfeiffer effects upon addition of anions 1 and 2 was also confirmed by recent reports of Shionoya, Stoddart and co-workers; the configurationally labile molecules being sandwich-shaped trinuclear silver complexes and catenanes respectively.26 Furthermore, in a recent study performed in collaboration with Professors Constable and Housecroft (Basle), it was shown that the configuration of ribose-decorated iron(II) metallostar 16 was better controlled though interionic diastereomeric interactions with TRISPHAT than by the intrinsic proximity of the chiral sugars.27
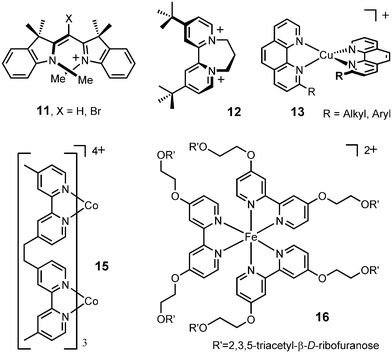 |
| Fig. 5 Configurationally labile cations. | |
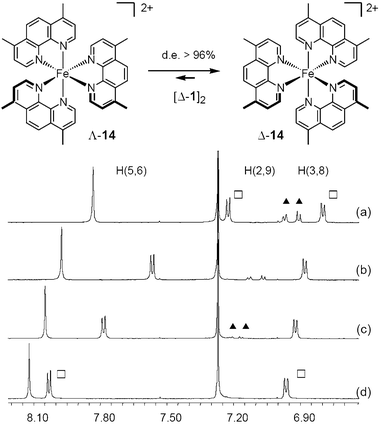 |
| Fig. 6 Diastereoselective interaction of 14 and anions Δ-1. 1H NMR (400 MHz) in DMSO-d6–CDCl3: (a) 30%, de 48%; (b) 20%, de 79%; (c) 15%, de 95%; (d) 10%, de >97%. Signals of aromatic protons of homochiral (□) and heterochiral (▲) salts. | |
Conclusion and future work
In conclusion, hexacoordinated phosphate anions like TRISPHAT 1 and BINPHAT 2 are able NMR solvating, resolving and asymmetry-inducing reagents when paired with configurationally stable or labile chiral cations. The organic, inorganic or organometallic nature of the cationic moieties is not influential as good anionic matches can usually be found. We believe that there is much to gain from this supramolecular approach to stereoselective synthesis as both configurations of a chiral cationic complex can be generated with no need to prepare two sets of enantiomeric ligands. It is a priori sufficient to form the cationic derivative with achiral ligands and exchange the traditional achiral anions (PF6−, BF4−, etc.) for chiral versions.
However, there is still much ground to be covered. For instance, enantiopure anionic counterions have so far displayed no or little influence on the stereochemical outcome of reactions involving cationic intermediates or reagents (eemax 15%).1b,1c,1d,24b,28 Most probably, this is due to a lack of effective chiral discrimination by the anions of (i) reactive prochiral intermediates or (ii) chiral cationic catalytic species. In the first case, it will require a better selection of the pairing partners and the development of less-polar reactive conditions. In the second case, as most of cationic catalytic species are metallo-organic or organo-metallic, it might mean moving the chiral anion from the second to the first coordination sphere of Lewis acidic transition metals. In fact, anions like TRISPHAT or BINPHAT can be essentially considered as non-coordinating counterions.29 It would be quite interesting to introduce Lewis basic atoms on the skeleton of the anions (e.g.17, Fig. 7) to induce tighter binding and, possibly, stronger chiral recognition and asymmetric induction events.
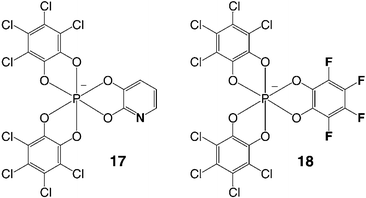 |
| Fig. 7 Possible nitrogen and fluorine containing hexacoordinated phosphate anions 17 and 18. | |
Recently, there has been much progress in the detailed structural analyses of ion pairs using NMR spectroscopy.2,30 On one hand, homonuclear 1H,1H-NOESY and heteronuclear 19F,1H-HOESY experiments have allowed qualitative and quantitative structural investigations on the interactions of cationic moieties and their counterions. On the other hand, diffusion data from pulse-field gradient spin echo (PGSE) experiments have given useful information of the intimate (contact, tight) or loose (solvent separated) nature of ion pairs. However, as anion 1 possesses only the core phosphorus as a strong NMR sensitive atom, no direct qualitative and quantitative studies were so far feasible on the peripheral interactions of 1 with chiral cations. It would be interesting to synthesize highly symmetrical fluorine or hydrogen-containing hexacoordinated phosphate anions (e.g.18, Fig. 7) which will be used to demonstrate effectively the geometry of interactions of chiral cations and anions.
Acknowledgements
We thank the University of Geneva, the Swiss National Science Foundation, the Federal Office for Education and Science and Sandoz Family Foundation for support.
References
-
(a) J. Lacour and V. Hebbe-Viton, Chem. Soc. Rev., 2003, 32, 373 RSC;
(b) D. B. Llewellyn and B. A. Arndtsen, Can. J. Chem., 2003, 81, 1280 CrossRef CAS;
(c) C. Carter, S. Fletcher and A. Nelson, Tetrahedron: Asymmetry, 2003, 14, 1995 CrossRef CAS;
(d) D. B. Llewellyn and B. A. Arndtsen, Organometallics, 2004, 23, 2838 CrossRef CAS and references therein.
- A. Macchioni, Eur. J. Inorg. Chem., 2003, 195 CrossRef CAS and references therein.
-
(a)
E. L. Eliel and S. H. Wilen, Stereochemistry of Organic Compounds, 1st edn., John Wiley & Sons, New York, USA, 1994 Search PubMed;
(b)
J. Jacques, A. Collet and S. H. Wilen, Enantiomers, Racemates, and Resolutions, 2nd edn., Krieger, Melbourne, USA, 1994 Search PubMed.
-
A. Von Zelewsky, Stereochemistry of Coordination Compounds, 1st edn., John Wiley & Sons, Chichester, UK, 1996 Search PubMed.
-
(a) A. Klaebe, M. Koenig, R. Wolf and P. Ahlberg, J. Chem. Soc., Dalton Trans., 1977, 570 RSC;
(b) J. Cavezzan, G. Etemad-Moghadam, M. Koenig and A. Klaebe, Tetrahedron Lett., 1979, 795 CrossRef CAS;
(c) M. Koenig, A. Klaebe, A. Munoz and R. Wolf, J. Chem. Soc., Perkin Trans. 2, 1979, 40 RSC.
-
(a) J. Lacour, C. Ginglinger, C. Grivet and G. Bernardinelli, Angew. Chem., Int. Ed. Engl., 1997, 36, 608 CrossRef CAS;
(b) J. Lacour, C. Ginglinger and F. Favarger, Tetrahedron Lett., 1998, 4825 CrossRef CAS;
(c) F. Favarger, C. Goujon-Ginglinger, D. Monchaud and J. Lacour, J. Org. Chem., 2004, 69, 8521 CrossRef CAS.
- In 1993 Schmutzler and co-workers isolated the racemic dimethylammonium salt of 1 as a product of spontaneous decomposition of a spirophosphorane in CDCl3. I. V. Shevchenko, A. Fischer, P. G. Jones and R. Schmutzler, Chem. Ber., 1992, 125, 1325 Search PubMed.
-
(a) J. Lacour, A. Londez, C. Goujon-Ginglinger, V. Buß and G. Bernardinelli, Org. Lett., 2000, 2, 4185 CrossRef CAS;
(b) J. Lacour and A. Londez, J. Organomet. Chem., 2002, 643–644, 392 CrossRef CAS;
(c) J. Lacour, A. Londez, D.-H. Tran, V. Desvergnes-Breuil, S. Constant and G. Bernardinelli, Helv. Chim. Acta, 2002, 85, 1364 CrossRef CAS.
- C. Pérollier, S. Constant, J. J. Jodry, G. Bernardinelli and J. Lacour, Chem. Commun., 2003, 2014 RSC.
-
(a) D. Parker, Chem. Rev., 1991, 91, 1441 CrossRef CAS;
(b) T. J. Wenzel and J. D. Wilcox, Chirality, 2003, 15, 256 CrossRef CAS.
-
(a) J. Lacour, L. Vial and C. Herse, Org. Lett., 2002, 4, 1351 CrossRef CAS;
(b) G. Heinrichs, L. Vial, J. Lacour and S. Kubik, Chem. Commun., 2003, 1252 RSC;
(c) C. Herse, D. Bas, F. C. Krebs, T. Bürgi, J. Weber, T. Wesolowski, B. W. Laursen and J. Lacour, Angew. Chem., Int. Ed., 2003, 42, 3162 CrossRef;
(d) C. Ginglinger, D. Jeannerat, J. Lacour, S. Jugé and J. Uziel, Tetrahedron Lett., 1998, 39, 7495 CrossRef CAS;
(e) V. Hebbe, A. Londez, C. Goujon-Ginglinger, F. Meyer, J. Uziel, S. Jugé and J. Lacour, Tetrahedron Lett., 2003, 44, 2467 CrossRef CAS;
(f) L. Pasquato, C. Herse and J. Lacour, Tetrahedron Lett., 2002, 43, 5517 CrossRef CAS.
-
(a) J. Lacour, C. Ginglinger, F. Favarger and S. Torche-Haldimann, Chem. Commun., 1997, 2285 RSC;
(b) O. Maury, J. Lacour and H. Le Bozec, Eur. J. Inorg. Chem., 2001, 201 CrossRef CAS;
(c) J. Giner Planas, D. Prim, F. Rose-Munch, E. Rose, D. Monchaud and J. Lacour, Organometallics, 2001, 20, 4107 CrossRef;
(d) G. Bruylants, C. Bresson, A. Boisdenghien, F. Pierard, A. Kirsch-De Mesmaeker, J. Lacour and K. Bartik, New. J. Chem., 2003, 27, 748 RSC.
- L. Vial and J. Lacour, Org. Lett., 2002, 4, 3939 CrossRef CAS.
-
(a) H. Amouri, R. Thouvenot, M. Gruselle, B. Malezieux and J. Vaissermann, Organometallics, 2001, 20, 1904 CrossRef CAS;
(b) H. Amouri, R. Thouvenot and M. Gruselle, C. R. Chim., 2002, 5, 257 Search PubMed;
(c) J. J. Jodry and K. Mikami, Tetrahedron Lett., 2004, 45, 4429 CrossRef CAS.
-
(a) H. Ratni, J. J. Jodry, J. Lacour and E. P. Kündig, Organometallics, 2000, 19, 3997 CrossRef CAS;
(b) A. Berger, J.-P. Djukic, M. Pfeffer, A. de Cian, N. Kyritsakas-Gruber, J. Lacour and L. Vial, Chem. Commun., 2003, 658 RSC;
(c) A. Berger, J.-P. Djukic, M. Pfeffer, J. Lacour, L. Vial, A. de Cian and N. Kyritsakas-Gruber, Organometallics, 2003, 22, 5243 CrossRef CAS.
-
(a) M. V. Martínez-Díaz, J. de Mendoza and T. Torres, Tetrahedron Lett., 1994, 35, 7669 CrossRef CAS;
(b) V. Andrisano, G. Gottarelli, S. Masiero, E. H. Heijne, S. Pieraccini and G. P. Spada, Angew. Chem., Int. Ed., 1999, 38, 2386 CrossRef CAS and references therein.
- J. Lacour, C. Goujon-Ginglinger, S. Torche-Haldimann and J. J. Jodry, Angew. Chem., Int. Ed., 2000, 39, 3695 CrossRef CAS.
- J. J. Jodry and J. Lacour, Chem. Eur. J., 2000, 6, 4297 CrossRef CAS.
- J. Lacour, S. Barchéchath, J. J. Jodry and C. Ginglinger, Tetrahedron Lett., 1998, 39, 567 CrossRef CAS.
-
(a) J. Lacour, S. Torche-Haldimann, J. J. Jodry, C. Ginglinger and F. Favarger, Chem. Commun., 1998, 1733 RSC;
(b) D. Monchaud, J. Lacour, C. Coudret and S. Fraysse, J. Organomet. Chem., 2001, 624, 388 CrossRef CAS;
(c) D. Monchaud, J. J. Jodry, D. Pomeranc, V. Heitz, J.-C. Chambron, J.-P. Sauvage and J. Lacour, Angew. Chem., Int. Ed., 2002, 41, 2317 CrossRef CAS.
-
(a) M. Brissard, O. Convert, M. Gruselle, C. Guyard-Duhayon and R. Thouvenot, Inorg. Chem., 2003, 42, 1378 CrossRef CAS;
(b) R. Caspar, H. Amouri, M. Gruselle, C. Cordier, B. Malezieux, R. Duval and H. Leveque, Eur. J. Inorg. Chem., 2003, 499 CrossRef CAS;
(c) M. Chavarot, S. Menage, O. Hamelin, F. Charnay, J. Pecaut and M. Fontecave, Inorg. Chem., 2003, 42, 4810 CAS;
(d) O. Hamelin, J. Pecaut and M. Fontecave, Chem. Eur. J., 2004, 10, 2548 CrossRef CAS;
(e) H. Amouri, R. Caspar, M. Gruselle, C. Guyard-Duhayon, K. Boubekeur, D. A. Lev, L. S. B. Collins and D. B. Grotjahn, Organometallics, 2004, 4338 CrossRef CAS;
(f) L. Mimassi, C. Guyard-Duhayon, M. N. Rager and H. Amouri, Inorg. Chem., 2004, 43, 6644 CrossRef CAS.
-
(a) P. Pfeiffer and K. Quehl, Chem. Ber., 1931, 64, 2667 Search PubMed;
(b) M. M. Green, C. Khatri and N. C. Peterson, J. Am. Chem. Soc., 1993, 115, 4941 CrossRef CAS;
(c) R. M. Yeh, M. Ziegler, D. W. Johnson, A. J. Terpin and K. N. Raymond, Inorg. Chem., 2001, 40, 2216 CrossRef CAS.
-
(a) S. Kirschner, N. Ahmad, C. Munir and R. J. Pollock, Pure Appl. Chem., 1979, 51, 913 CrossRef CAS;
(b) B. Norden and F. Tjerneld, FEBS Lett., 1976, 67, 368 CrossRef CAS.
-
(a) C. Pasquini, V. Desvergnes-Breuil, J. J. Jodry, A. Dalla Cort and J. Lacour, Tetrahedron Lett., 2002, 43, 423 CrossRef CAS;
(b) L. Vial, M.-H. Gonçalves, P.-Y. Morgantini, J. Weber, G. Bernardinelli and J. Lacour, Synlett, 2004, 1565 CAS.
-
(a) V. Desvergnes-Breuil, V. Hebbe, C. Dietrich-Buchecker, J.-P. Sauvage and J. Lacour, Inorg. Chem., 2003, 42, 255 CrossRef CAS;
(b) J. Lacour, J. J. Jodry, C. Ginglinger and S. Torche-Haldimann, Angew. Chem., Int. Ed. Engl., 1998, 37, 2379 CrossRef CAS;
(c) J. J. Jodry, R. Frantz and J. Lacour, Inorg. Chem., 2004, 43, 3329 CrossRef CAS;
(d) J. Lacour, J. J. Jodry and D. Monchaud, Chem. Commun., 2001, 2302 RSC.
-
(a) S. Hiraoka, K. Harano, T. Tanaka, M. Shiro and M. Shionoya, Angew. Chem., Int. Ed., 2003, 42, 5182 CrossRef CAS;
(b) S. A. Vignon, J. Wong, H.-R. Tseng and J. F. Stoddart, Org. Lett., 2004, 6, 1095 CrossRef CAS.
- E. C. Constable, R. Frantz, C. E. Housecroft, J. Lacour and A. Mahmood, Inorg. Chem., 2004, 43, 4817 CrossRef CAS.
- J. Lacour, D. Monchaud and C. Marsol, Tetrahedron Lett., 2002, 43, 8257 CrossRef CAS.
- I. Krossing and I. Raabe, Angew. Chem., Int. Ed., 2004, 43, 2066 CrossRef CAS.
-
(a) For recent references, see: C. Zuccaccia, N. G. Stahl, A. Macchioni, M. C. Chen, J. A. Roberts and T. J. Marks, J. Am. Chem. Soc., 2004, 126, 1448 Search PubMed;
(b) P. Dotta, P. G. A. Kumar, P. S. Pregosin and A. Albinati, Helv. Chim. Acta, 2004, 87, 272 CrossRef CAS;
(c) T. J. Geldbach, F. Breher, V. Gramlich, P. G. A. Kumar and P. S. Pregosin, Inorg. Chem., 2004, 43, 1920 CAS;
(d) F. Ribot, V. Escax, J. C. Martins, M. Biesemans, L. Ghys, I. Verbruggen and R. Willem, Chem. Eur. J., 2004, 10, 1747 CrossRef CAS;
(e) E. Martínez-Viviente, P. S. Pregosin, L. Vial, C. Herse and J. Lacour, Chem. Eur. J., 2004, 10, 2912 CrossRef CAS and references therein.
|
This journal is © The Royal Society of Chemistry 2005 |
Click here to see how this site uses Cookies. View our privacy policy here.