DOI:
10.1039/B411922C
(Tutorial Review)
Green Chem., 2005,
7, 9-14
Part II. Effect of the anion and toxicology†
Received
3rd August 2004
, Accepted 18th November 2004
First published on 13th December 2004
Abstract
Although ionic liquids are generally referred to in a ‘green’ context, little is known about their degradation in the environment. Part I of this series focused on the biodegradability of the commonly used dialkylimidazolium ionic liquids and the effect of the imidazolium cation on biodegradability. We now report the influence of the anion on biodegradability. Preliminary investigations into the toxicology of ionic liquids are also described.
M. Teresa Garcia | Dr M. Teresa Garcia received her Ph.D. in chemistry from the University of Barcelona, Spain in 1990. She is currently a research associate in the Surfactant Technology Department of the Chemical and Environmental Research Institute of Barcelona (IIQAB) belonging to the Spanish National Research Council (CSIC). Her research interests include environmental chemistry and ecotoxicology of surfactants. |
Nicholas Gathergood | Dr Nicholas Gathergood completed his PhD at Southampton University with Prof. Richard Whitby in 1998. His first post-doctorial position was with Prof. Karl Anker Jørgensen at Aarhus University, Denmark. Dr Gathergood joined Prof. Scammells group in 2001, working initially at Deakin University, then at the Victorian College of Pharmacy, Monash University. In November 2004 Dr Gathergood was appointed as lecturer in organic chemistry at Dublin City University. His research interests include medicinal chemistry, asymmetric catalysis, chiral macrocycles and potential applications for ionic liquids. |
Peter J. Scammells | Prof. Peter Scammells completed his PhD at Griffith University under the supervision of Prof. Ron Quinn in 1991. After research appointments at the University of South Florida and the Technische Universität Darmstadt, he took up a lectureship at Deakin University in 1993. Prof. Scammells is currently Head of the Department of Medicinal Chemistry and Professor of Medicinal Chemistry at the Victorian College of Pharmacy, Monash University. His research interests include the medicinal chemistry of adenosine and opiate receptors and potential applications for ionic liquids in pharmaceutical synthesis. |
Introduction
Although there has been intense interest in the use of ionic liquids (ILs) as green solvents,1 relatively little is known about their biodegradability and toxicity, basic properties in the environmental risk assessment of any organic compound. We recently conducted the first study of IL biodegradability2 and other groups have begun looking at their toxicology.3 In Part I of this series, we reported that the commonly used dialkylimidazolium ILs (bmimX) showed negligible biodegradability in the Closed Bottle Test (OECD 301D). The incorporation of an ester in the side chain of the imidazolium cation significantly increased biodegradability. 3-Methyl-1-(pentoxycarbonylmethyl)imidazolium bromide (1) proved to be the most biodegradable compound in this series (32% degradation after 28 days). It is postulated that this improved biodegradation is due to an enzymatic hydrolysis step which initiates a pathway to further breakdown products. Consistent with this suggestion, the corresponding amide analogs (compound 2) were poorly biodegradable. In this paper we examine the effect of the anion on a series of ILs containing an ester group in the side chain and compare these to BmimBr examples. Levels of biodegradation are usually dependent on compound stability and toxicity. To determine which of these factors is dominant a toxicology study of a series of ionic liquids was also performed. The effect of both the counter-ion and the alkyl chain length on the aquatic toxicity of alkylmethylimidazolium ionic liquids was investigated. For this purpose, two short-term bioassays, luminescent bacteria and Daphnia magna tests, were applied. These bioassays show good sensitivity and have been used to evaluate the toxicity of many classes of chemical contaminants.4
Results and discussion
Two types of ILs were initially prepared. The 1-butyl-3-methylimidazolium series (compounds 3a–f) were prepared using straightforward literature methodology.5 The 1-(propoxycarbonyl)methyl-3-methylimidazole series were prepared using the synthetic approach described in Part I of this series.2b Briefly, this involved the alkylation of methyl imidazole with propyl bromoacetate followed by metathesis to introduce the counter-ion of choice.
Ultimate biodegradability
The ILs prepared in this study were evaluated using the ‘Closed Bottle Test’
(OECD 301D).6 In this test the IL was added to an aerobic aqueous medium inoculated with wastewater microorganisms and the depletion of dissolved molecular oxygen was measured for a defined period of time and reported as a percentage of the theoretical maximum. Duplicate bottles of each series were analysed at the start of the test for dissolved oxygen and the remaining bottles were incubated at 20 °C ± 1 °C in the dark. Bottles of all series were withdrawn in duplicate for dissolved oxygen analysis over the 28 day incubation period. A control with inoculum, but without test chemicals was run in parallel for the determination of oxygen blanks. Sodium n-dodecyl sulfate (SDS) was used as reference substance. Compounds which reached a biodegradation level higher than 60% are referred to as “readily biodegradable”. The results obtained for the butylmethylimidazolium cation with a range of anions are shown in Fig. 1.
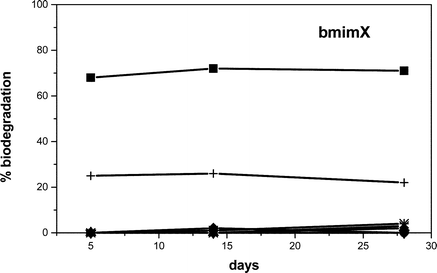 |
| Fig. 1 Biodegradation curves of the butylmethylimidazolium salts with different counter-ion: bmimBr (●), bmimCl (▲), bmimBF4
(▼), bmimPF6
(♦), bmimoctyl-OSO3
(+), bmimN(CN)2
(×), bmimNTf2(*); reference substance: SDS (■). | |
1-Butyl-3-methylimidazolium octylsulfate underwent a modest amount of biodegradation (25%) after 28 days, while the other ILs remained largely intact (less than 5% biodegradation).
Clearly none of the ILs tested were “readily biodegradable”. The higher percentage of biodegradation observed for the octyl sulfate anion is not surprising as linear alkyl sulfates are well known to be readily biodegradable.7 BOD/COD for sodium dodecyl sulfate was more than 60% within 5 days incubation in the Closed Bottle Test (Fig. 1) confirming the excellent and very rapid ultimate biodegradation of linear alkyl sulfates even in this relatively stringent test system. Initial attack of these molecules is effected by enzymatic cleavage of the sulfate ester bond leaving inorganic sulfate and fatty acid. The further degradation of the fatty alcohol sulfate intermediates, the fatty acids, proceeds via
β-oxidation enabling the utilization of the organic moiety of the molecule for energy and biomass production.8
The 3-methyl-1-(propoxycarbonyl)imidazolium series showed higher biodegradability for all corresponding anions (Fig. 2). This result was consistent with the findings from our earlier study2 in which the incorporation of a side chain ester moiety increased biodegradation. Once again, the most biodegradable compound in this series possessed the octyl sulfate anion (49% biodegradation after 28 days).
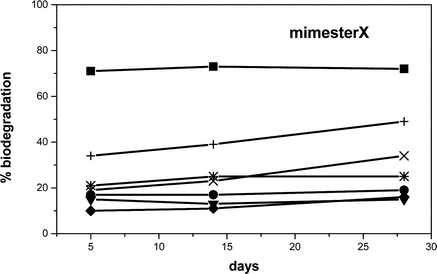 |
| Fig. 2 Biodegradation curves of the 3-methyl-1-(propyloxycarbonyl)-imidazolium X; X = Br (●), BF4
(▼), PF6
(♦), octyl-OSO3
(+), N(CN)2
(×), NTf2
(*); reference substance: SDS (■). | |
Although modifications of the anion led to changes in the physical and chemical properties of the ionic liquids, the effect of the counter-ion on biodegradability was generally not pronounced. The octyl sulfate anion proved to be an exception, showing significantly higher biodegradability in both test sets. The introduction of an organic anion readily catabolised clearly improved the extent of ultimate biodegradation.
The ionic liquid containing an ester moiety in the side chain of the imidazolium cation and the octyl sulfate anion (compound 4f) reached the highest extent of biodegradation. The incorporation of chemical bonds susceptible to enzymatic hydrolysis, resulted in a significant enhancement of biodegradability compared with commonly used dialkylimidazolium ionic liquids bmimBF4 and bmimPF6.
Toxicity assessment
Acute toxicity tests on freshwater crustacea (Daphnia magna)9 as well as on saltwater bacteria (Photobacterium phosphoreum)10 were carried out to assess the aquatic toxicity. The results of both Daphnia magna 24 h immobilisation test (IC50) and Photobacterium phosphoreum 30 min luminescence reduction test (EC50) for the 1-alkyl-3-methylimidazolium cation with different anions are given in Table 1.
|
D. magna, IC50/µmol L−1 |
P. phosphoreum, EC50/µmol L−1 |
log IC50, 95% CI |
log EC50, 95% CI |
bmimBr |
1.78 ± 0.07 |
3.27 ± 0.09 |
bmimCl |
1.85 ± 0.06 |
3.34 ± 0.13 |
bmimBF4 |
1.79 ± 0.05 |
3.10 ± 0.17 |
bmimPF6 |
1.95 ± 0.03 |
3.07 ± 0.29 |
hmimCl |
1.09 ± 0.05 |
2.32 ± 0.16 |
hmimBF4 |
1.13 ± 0.05 |
— |
hmimPF6 |
— |
2.17 ± 0.06 |
omimCl |
0.54 ± 0.07 |
1.19 ± 0.11 |
omimBF4 |
0.66 ± 0.07 |
— |
omimPF6 |
— |
0.95 ± 0.12 |
The estimated concentration able to immobilise 50% of the crustacea population (IC50) ranged from 4 to 90 µmol L−1
(from 0.54 to 1.85 in logarithmic form) whereas the effective concentration resulting in a 50% reduction of light produced by the bacteria (EC50) ranged from 10 to 2200 µmol L−1
(from 0.95 to 3.34 in logarithmic form).
These data indicate that the Daphnia magna are much more sensitive to the toxic effects of 1-butyl-3-methylimidazolium salts than bacteria (i.e. the IC50 values are an order of magnitude less than EC50 values). Differences in toxicity of 1-alkyl-3-methylimidazolium ILs become less pronounced with increasing alkyl chain length.
The toxicity data were compared with corresponding data for commonly used organic solvents (Table 2) and other quaternary ammonium compounds (Table 3).
Table 2 Acute toxicity of organic solvents on Daphnia magna and Photobacterium phosphoreum
Organic solvent |
D. magna, IC50/µmol L−1 |
P. phosphoreum,EC50/µmol L−1 |
log IC50a |
log EC50b |
D. magna data taken from Kaiser.11a
P. phosphoreum data taken from Kahru.11b
|
Methanol |
5.85 |
6.51 |
Ethanol |
5.33 |
5.89 |
Isopropanol |
5.22 |
5.72 |
Acetonitrile |
5.39 |
5.57 |
Acetone |
5.37 |
5.17 |
Dichloromethane |
3.42 |
4.57 |
Chloroform |
2.24 |
4.16 |
Table 3 Acute toxicity of quaternary ammonium surfactants on Daphnia magna and Photobacterium phosphoreum
Surfactanta |
D. magna, IC50/µmol L−1 |
P. phosphoreum. EC50/µmol L−1 |
log IC50 |
log EC50 |
DT, dodecyl trimethyl ammonium bromide; TT, tetradecyl trimethyl ammonium bromide; HT, hexadecyl trimethyl ammonium bromide; DBD dodecyl benzyl dimethyl ammonium bromide; Im-1, 1-decyl-3-hexyloxymethylimidazolium chloride; Im-2, 1-decyl-3cyclohexyloxymethyl imidazolium chloride; Bis-Im, 3,3′-(2,7-dioxyoctylmethylene)bis(1-octyl imidazolium) chloride.
Data reported by Garcia et al..12a
Data reported by Nalecz-Jawecki et al..12b
|
DT |
0.09b |
−0.11b |
TT |
−0.38b |
−0.08b |
HT |
−0.45b |
0.24b |
DBD |
−0.47b |
−0.33b |
Im-1 |
— |
−0.41c |
Im-2 |
— |
−0.23c |
Bis-Im |
— |
−0.18c |
For Daphnia magna, the 1-alkyl-3-methylimidazolium ionic liquids generally proved to be much more toxic than the non-chlorinated organic solvents (as evidenced by differences in IC50 values of more than three orders of magnitude). These ILs also proved to be more toxic than the chlorinated solvents, dichloromethane and chloroform, though the difference was less pronounced (30–45 and 2–3 times more toxic, respectively). Likewise, imidazolium salts are clearly more toxic than organic solvents against Photobacterium phosphoreum, although the differences in the effective concentrations are lower than for crustacea.
The structural similarity between dialkylimidazolium ionic liquids and cationic surfactants possessing an imidazolium core prompted us to compare their aquatic toxicity values. In both bioassays, cationic surfactants were more toxic than dialkyimidazolium ionic liquids. In the Daphnia magna test, cationic surfactants have effective concentrations between 50 and 300 times lower than the butylmethylimidazolium ionic liquids investigated. In luminescent bacteria, differences in EC50 values are of three orders of magnitude. As the alkyl chain length of the ionic liquids increases, toxicity differences with the cationic surfactants decrease significantly.
Bearing in mind that biological membranes are essentially non-polar interfaces, evidence exists that the toxicity of chemicals against the aquatic species is caused by the ability of the molecules to disrupt the integral membrane by a hydrophobic/ionic adsorption phenomenon at the cell membrane–water interface. Hydrophobic molecules have a greater ability to accumulate at this interface. Therefore, higher toxicity would be expected with increasing hydrophobicity in the molecule, as reported for anionic surfactants on Daphnia magna13 and for alcohol ethoxylated surfactants on Photobacterium phosphoreum.14 Accordingly, the aquatic toxicity increased with the alkyl chain length for the 1-alkyl-3-methylimidazolium ionic liquids investigated and linear relationships between both parameters were established (Fig. 3). This finding is in keeping with other recently reported investigations into IL toxicity.3
The quantitative structure–activity relationships obtained between toxicity and alkyl chain length provides evidence that the potency of the biological activity is closely related to structural parameters that determine the hydrophobicity of the molecule and hence, its organism–water partitioning properties. On the contrary, our results suggest that the nature of the inorganic anion has only a little effect.
Conclusions
Series of butylmethylimidazolium [bmimX, X = Br, BF4, PF6, NTf2, N(CN)2 and octylOSO3] and methyl (propoxycarbonyl)imidazolium ionic liquids were prepared and evaluated using the Closed Bottle Test (OECD 301 D). The bmim series generally proved to be poorly biodegradable, with all but one member of this series showed little or no degradation after 28 days. The corresponding 3-methyl-1-(propoxymethylcarbonyl)imidazolium series showed higher levels of biodegradability. However, none of the compounds that were tested could be classified as “readily biodegradable”. In both series ILs with the octyl sulfate anion conferred higher levels of biodegradability.
Two short term bioassays were used to evaluate IL toxicity—the Daphnia magna immobilisation test and the Photobacterium phosphoreum luminescence reduction test. The dialkylimidazolium ILs that were analysed proved to be more toxic than conventional organic solvents in both bioassays.
Experimental
Synthesis
1-n-Butyl-3-methylimidazolium ionic liquids (3)
[bmimX, where X = Cl, Br, BF4, PF6, NTf2, N(CN)2 and octylOSO3] were prepared according to literature methods.5 1-n-Hexyl-3-methylimidazolium and 1-n-octyl-3-methylimidazolium ionic liquids (X = Cl, BF4 and PF6) were also prepared using literature methods.2b
The syntheses of 3-methyl-1-(propyloxycarbonyl)imidazolium ionic liquids (4a–f) were as reported in Part I of this series2 or as described below.
Sodium dicyanamide (96%) and octyl sulfate, sodium salt (95%) were purchased from Aldrich. All organic solvents were dried and distilled before use. ILs were washed with distilled water.
All NMR spectra of ILs were recorded in CD3CN (Aldrich 15,180-7, 99.8 atom%D) on a Bruker Avance DPX 300 spectrometer. 1H and 13C NMR spectra were recorded at 300 MHz and 75.4 MHz, respectively.
1-n-Butyl-3-methylimidazolium bromide dicyanamide (3e)
The title compound was prepared analogously to (4e) using 1-n-butyl-3-methylimidazolium bromide (3a)
(3.00 g, 13.7 mmol) and NaN(CN)2
(1.46 g, 16.4 mmol) to give a clear oil in 78% yield (2.19 g, 10.7 mmol). 1H NMR (300 MHz, CD3CN)
δ 9.20 (s, 1H), 7.50 (s, 1H), 7.46 (s, 1H), 4.20 (t, J
= 7.0 Hz, 2H), 3.89 (s, 3H), 1.82 (tt, J
= 7.5, 7.5 Hz, 2H), 1.30 (tt, J
= 7.5, 7.5 Hz, 3H), 0.92 (t, J
= 7.0 Hz, 3H). 13C NMR (75 MHz, CD3CN)
δ 137.55, 124.54, 123.23, 50.14, 36.84, 32.62, 19.94, 13.68. Peaks for N(CN)2− not cited.
1-n-Butyl-3-methylimidazolium octylsulfate (3f)
The title compound was prepared according to a literature procedure using 1-butyl-3-methylimidazolium bromide (3a)
(3.02 g, 14 mmol) and Na(n–C8H17O–SO3)
(2.70 g, 11.6 mmol) in distilled water (5 mL) in 72% yield (2.92 g, 8.4 mmol).5d1H NMR (300 MHz, CD3CN)
δ 8.89 (s, 1H), 7.45 (s, 1H), 7.41 (s, 1H), 4.16 (t, J
= 7.0 Hz, 2H), 3.86 (s, 3H), 3.82 (t, J
= 7.0 Hz, 2H), 1.82 (tt, J
= 7.5, 7.5 Hz, 2H), 1.56 (tt, J
= 7.5, 7.5 Hz, 2H), 1.34 (tt, J
= 7.5, 7.5 Hz, 2H), 1.35–1.24 (m, 10H), 0.93 (t, J
= 7.0 Hz, 3H), 0.87 (t, J
= 7.0 Hz, 3H). 13C NMR (75 MHz, CD3CN)
δ 137.82, 124.69, 123.35, 67.38, 50.27, 36.85, 32.73, 32.65, 30.52, 30.16, 30.08, 26.90, 23.44, 20.07, 14.47, 13.78.
3-Methyl-1-(propoxycarbonylmethyl))imidazolium N(CN)2
(4e)
A dry flask was charged with 3-methyl-1-(propoxycarbonyl)imidazolium bromide (4a)
(2.10 g, 7.7 mmol) and dry acetonitrile (5 mL) under a nitrogen atmosphere. NaN(CN)2
(821 mg, 9.3 mmol) was added in one portion and the suspension was stirred vigorously for 4 days at rt. The fine white precipitate was filtered quickly in air and washed with dry acetonitrile (2 × 1 mL). The filtrate and washings were combined, solvent removed by rotary evaporation then in vacuo. The product was dried at 60 °C at 0.01 mmHg for 72 h to give a clear viscous oil in 93% yield (1.79 g, 7.2 mmol). 1H NMR (300 MHz, CD3CN)
δ 9.16 (s, 1H), 7.56 (s, 1H), 7.47 (s, 1H), 5.23 (s, 2H), 4.15 (t, J
= 7.0 Hz, 2H), 3.91 (s, 3H), 1.67 (tt, J
= 7.0, 7.0 Hz, 2H), 0.94 (t, J
= 7.0 Hz, 3H). 13C NMR (75 MHz, CD3CN)
δ 167.89, 139.01, 125.06, 124.70, 69.10, 51.27, 37.56, 22.94, 10.90. Peaks for NCNCN− not cited. MS (ESI): m/z, 183.1 [M–NCNCN−]+; MS (ESI): m/z, 66 [N(CN)2−].
3-Methyl-1-(propoxycarbonylmethyl))imidazolium octylsulfate (4f)
The title compound was prepared according to a literature procedure using 3-methyl-1-(propoxycarbonyl)imidazolium bromide (4a, 1.40 g, 5.3 mmol) and Na(n–C8H17O–SO3)
(1.04 g, 4.5 mmol) in distilled water (2 mL) in 58% yield (1.02 g, 2.6 mmol).5d1H NMR (300 MHz, CD3CN)
δ 8.74 (s, 1H), 7.44 (s, 1H), 7.40 (s, 1H), 5.05 (s, 2H), 4.16 (t, J
= 7.0 Hz, 2H), 3.89 (s, 3H), 3.80 (t, J
= 7.0 Hz, 2H), 1.68 (tt, J
= 7.5, 7.5 Hz, 2H), 1.65–1.52 (m, 2H), 1.40–1.20 (m, 10H), 0.95 (t, J
= 7.0 Hz, 3H), 0.89 (t, J
= 7.0 Hz, 3H). 13C NMR (75 MHz, CD3CN)
δ 168.16, 139.48, 125.76, 124.61, 68.72, 67.97, 50.80, 37.19, 32.84, 30.34, 30.25, 26.97, 23.61, 22.80, 14.80, 10.95. MS (ESI): m/z, 183.1 [M–C8H17OSO3−]+; MS (ESI): m/z, 209.1 [C8H17OSO3−].
Closed bottle test6
The biodegradability of the test compounds was evaluated using the “Closed Bottle Test”
(OECD 301 D). In this method, the chemical being evaluated is added to an aerobic aqueous medium inoculated with wastewater microorganisms and the depletion of dissolved molecular oxygen is measured for a defined period of time and reported as a percentage of the theoretical maximum. Compounds which reach a biodegradation level higher than 60% are referred to as “readily biodegradable”.
Sodium n-dodecyl sulfate (SDS) was used as reference substance. Solutions containing 2 mg L−1 of the test ionic liquids and the reference chemical as sole sources of organic carbon were prepared, separately, in previously aerated mineral medium. The solutions were then inoculated with secondary effluent collected from an activated sludge treatment plant and each well-mixed solution was carefully dispensed into a series of BOD bottles so that all the bottles were completely full. A control with inoculum, but without test chemicals was run parallel for the determination of oxygen blanks. Duplicate bottles of each series were analysed immediately for dissolved oxygen and the remaining bottles were incubated at 20 °C ± 1 °C in the dark. Bottles of all series were withdrawn in duplicate for dissolved oxygen analysis over the 28-day incubation period. The biodegradation after n days was expressed as the ratio of the biochemical oxygen demand (BOD) to the chemical oxygen demand (COD) both of them expressed as mg O2 per mg compound. The chemical oxygen demand was determined by the dichromate reflux method.15 For the calculation of the biochemical oxygen demand the determined oxygen depletions were divided by the concentration of ionic liquid.
Daphnia magna immobilisation test
Daphnia magna, laboratory bred, not more than 24 hours old were used in this test, where swimming incapability is the end point. The pH of the medium was 8.0 and the total hardness was 250 mg L−1
(as CaCO3), with a Ca ∶ Mg ratio of 4 ∶ 1. Tests were performed in the dark at 20 °C. Twenty Daphnia, divided into four groups of five animals each, were used at each test concentration. For each surfactant, ten concentrations in a geometric series were tested in the concentration range first established in a preliminary test. The percentage immobility at 24 hours was plotted against concentration on logarithmic-probability paper, a linear relationship was obtained and the IC50 was calculated from the corresponding equation.
Photobacterium phosphoreum luminescence reduction test (Microtox test)10
Photobacterium phosphoreum is a marine luminescent bacterium naturally adapted to a saline environment. These bacteria liberate energy in the form of visible light (intensity maximum at 490 nm) as a consequence of a series of metabolic reactions. On exposure to toxic substances, the light output is reduced and this reduction is proportional to the toxicity of the sample. Therefore, the toxicity bioassay is based on the light emission of these bacteria, as a measurement of their metabolic activity. In the Microtox bioassay the concentration of an aqueous solution of a chemical that causes a 50% reduction of the light emitted by the bacteria (EC50) is calculated from a concentration-response curve by regression analysis. The osmotic pressure of the samples was adjusted by NaCl addition (2%). Toxicity data were based on a 30 min exposure of bacteria to the surfactant solution at 15 °C.
References
-
(a) P. Wasserscheid and W. Keim, Angew. Chem., Int. Ed., 2000, 39, 3772 CrossRef;
(b) T. Welton, Chem. Rev., 1999, 99, 2071 CrossRef CAS;
(c) J. D. Holbury and K. R. Seddon, Clean Prod. Process., 1999, 1, 223 CrossRef.
-
(a) N. Gathergood and P. J. Scammells, Aust. J. Chem., 2002, 55, 557 CrossRef CAS;
(b) N. Gathergood, M. T. Garcia and P. J. Scammells, Green Chem., 2004, 6, 166 RSC.
-
(a) J. Pernak, K. Sobaszkiewicz and I. Mirska, Green Chem., 2003, 5, 52 RSC;
(b) B. Jastorff, R. Störmann, J. Ranke, K. Mölter, F. Stock, B. Oberheitmann, W. Hoffmann, J. Hoffmann, M. Nüchter, B. Ondruschka and J. Filser, Green Chem., 2003, 5, 136 RSC;
(c) R. P. Swatloski, J. D. Holbrey, S. B. Memon, G. A. Caldwell, K. A. Caldwell and R. D. Rogers, Chem. Commun., 2004, 668 RSC;
(d) F. Stock, J. Hoffman, J. Ranke, R. Stormann, B. Ondruschka and B. Jastorff, Green Chem., 2004, 6, 286–290 RSC;
(e) J. Ranke, K. Molter, F. Stock, U. Bottin-Weber, J. Poczobutt, J. Hoffmann, B. Ondruschka, J. Filser and B. Jastorff, Ecotoxicol. Environ. Saf., 2004, 58, 396–404 CrossRef CAS.
-
(a) C. J. Keddy, J. C. Greene and M. A. Bonnell, Ecotoxicol. Environ. Saf., 1995, 30, 221–251 CrossRef CAS;
(b)
N. A. Chester, G. R. Famini, M. V. Haley, C. W. Kurnas, P. A. Sterling and L. Y. Wilson, in Biomarkers for agrochemicals and toxic substances, ACS Symp. Ser., 1996, 643, 191–204 Search PubMed;
(c) S. M. Steinberg, E. J. Poziomek, W. H. Engelmann and K. R. Rogers, Chemosphere, 1995, 30, 2155–2197 CrossRef CAS.
-
(a) Y. Matsuda, H. Gotou, K. Katou, H. Matsumoto, M. Yamashita, K. Takahashi, S. Ide, K. Furuno and K. Torisu, Heterocycles, 1991, 32, 2217 CAS;
(b) R. A. Jones, J. Sepulveda Arques, E. Zaballos Garcia, P. A. Bates and M. B. Hutsthouse, J. Chem. Soc., Chem. Commun., 1986, 24, 1745 RSC;
(c) D. R. MacFarlane, J. Golding, S. Forsyth, M. Forsyth and G. B. Deacon, Chem. Commun., 2001, 16, 1430 RSC;
(d) P. Wasserscheid, R. van Hal and A. Bosmann, Green Chem., 2002, 4, 400 RSC.
- OECD Chemical Group, Ready Biodegradability: Closed Bottle Test. Method 301 D, OECD Revised Guidelines for Tests for Ready Biodegradability, Paris, France, 1993 Search PubMed.
-
R. D. Swisher, in Surfactant Biodegradation, 2nd edn., ed. M. J. Schick and F. M. Fowkes, Marcel Dekker, New York & Basel, 1987, Search PubMed.
-
(a) O. R. T. Thomas and G. F. White, Biotechnol. Appl. Biochem., 1989, 11, 318–327 CAS;
(b)
J. Steber and H. Berger, in Biodegradability of Surfactants., ed. D.R. Karsa and M.R. Porter, Blackie Academic & Professional, London, UK, 1995 Search PubMed.
- OECD, Daphnia sp. Acute Immobilisation Test. Method 202, OECD Guidelines for Testing of Chemicals, Paris, France, 1984 Search PubMed.
- DIN (Deutches Institut für Normung), German standard methods for the examination of water, waste water and sludge. Bio-assays (group L) Part-34. Determination of the inhibitory effect of waste
water on the light emission of Photobacterium phosphoreum; luminescent bacteria waste water test using conserved bacteria (L34), DIN 38412-34, 1997 Search PubMed.
-
(a)
K. L. E. Kaiser, QSAR in Environmental Toxicology II, ed. K.L.E. Kaiser, Reidel Publishing Company, Kluwer Academic Publishers, Dordrecht, Holland, 1987;
(b) K. L. E. Kaiser and V. S. Palabrica, Water Pollut. Res. J. Can., 1991, 26, 361–431 Search PubMed;
(c) A. Karhu, ATLA, 1993, 21, 210–215 Search PubMed.
-
(a) M. T. García, I. Ribosa, T. Guindulain, J. Sánchez-Leal and J. Vives-Rego, Environ. Pollut., 2001, 111, 169 CrossRef CAS;
(b) G. Nalecz-Jawecki, E. Grabinska-Sota and P. Narkiewicz, Ecotoxicol. Environ. Saf., 2003, 54, 87–91 CrossRef CAS.
-
H. A. Painter, in The Handbook of Environmental Chemistry, 3, Part F, Springer-Verlag, Berlin-Heilderberg, 1992 Search PubMed.
- I. Ribosa, M. T. García, J. Sanchez Leal and J. J. Gonzalez, Toxicol. Environ. Chem., 1993, 39, 237–241 CAS.
- APHA (American Public Health), AWWA (American Water Works Association), and WPCF (Water Pollution Control Federation), Method 508B, Standard Methods for the Examination of Water and Wastewater, 16th edn., Washington, 1985, pp. 532–537 Search PubMed.
Footnotes |
† For Part I see ref. 2b |
‡ Current address: School of Chemical Sciences, Dublin City University, Glasnevin, Dublin 9, Ireland. E-mail: Nick.Gathergood@dcu.ie; Fax: +353 1 700 5503; Tel: +353 1 700 7860. |
|
This journal is © The Royal Society of Chemistry 2005 |
Click here to see how this site uses Cookies. View our privacy policy here.