DOI:
10.1039/B108322H
(Perspective)
Green Chem., 2002,
4, 1-4
Developing metal-mediated and catalyzed reactions in air and water
Received 13th September 2001
First published on 14th January 2002
Abstract
Chao-Jun Li gives a personal perspective on research that won the 2001 Presidential Green Chemistry Challenge Award (academic category)
| Chao-Jun Li received a Ph.D at McGill University and did postdoctoral research at Stanford University. Since 1994, he has been at the Chemistry Department of Tulane University as an Assistant Professor, Associate Professor (1998), and Professor (2000–present). He received a National Science Foudnation’s Early CAREER Award (1998), a Tulane's Eli Lilly Teaching Fellowship (1996), an Outstanding Young Scientist Award (Overseas) from the NFS of China (2000). He served as an International Co-coordinator for the Green Chemistry Workshop in China. |
Green ContextIt is unthinkable that Grignard and similar reactions could proceed in water. Decades of "common knowledge" has made this idea ridiculous, meaning that synthetic chemists prepare anhydrous solvents regularly, generating large quantities of waste in the process, every time they attempt one of these reactions. This article challenges this accepted wisdom, and shows that it is indeed possible to carry out many of these reactions in water itself, leading to significant advances in the environmental performance of these reaction types.DJM |
Introduction
The importance of carbanions in synthesis is exemplified by the Grignard and Wittig reactions. Although they are among the most versatile and useful reactions in organic chemistry, carbanion-based reactions are generally known to be very sensitive towards air and water. The required deviation from ambient conditions along with the commonly used methods for their generation leads to several fundamental drawbacks when the Principles of Green Chemistry1 are applied (Fig. 1): (1) large volumes of anhydrous organic solvents are required which could potentially increase volatile organic emissions; (2) anhydrous conditions require excess drying agents which leads to unwanted waste; (3) anhydrous conditions also require the protection of functional groups such as hydroxy groups and carboxylic acids; (4) the requirement of inert gas atmosphere implies that the catalyst is prone to deactivation, which will increase the amount of heavy metal waste; (5) requirement of stoichiometric amounts of metals also leads to a large amount of metal waste; and (6) the general use of organic halides as the carbanion source will generate a stoichiometric amount of halide waste, as well as requiring multi-step synthesis of the halides. |
| Fig. 1 Stepwise greening of Grignard-type reactions | |
Project goal
To green carbanion-based reactions, with a focus on carbonyl additions (Barbier–Grignard type reactions), through stepwise elimination of all the auxiliary factors and evaluate their scope in synthesis (Fig. 1). The research was divided into two stages: (1) the factors below the scheme in Fig. 1 and (2) the factors above the scheme in Fig. 1.Stage 1: Eliminating the requirements for inert atmosphere, anhydrous solvent and protection
Carbonyl allylation/allenylation/propargylation. Organometallic chemistry and transition-metal catalysis have traditionally been carried out in dry-boxes where the use of an inert gas atmosphere and the exclusion of moisture have been essential. However, Nature′s use of metals catalytically under ambient conditions of air and water have played key roles in various enzymatic reactions including biocatalysis, biodegradation, photosynthesis, nitrogen fixation, digestion, as well as in the evolution of bioorganisms. Since Wolinsky et al.2 made an observation in 1977 that the allylation reaction of carbonyl compounds with allyl bromide mediated by zinc could be carried out in 95% ethanol and tert-butyl alcohol, metal-mediated Barbier–Grignard type carbonyl additions in aqueous media have received increased interest. Extensive research has been carried out by many groups on the allylation of carbonyl compounds in water,3 in which one of the characteristics of these reactions is that they can also be carried out in air. Based upon my earlier work with T. H. Chan in the development of indium mediated allylation in water, my research group has recently developed various new synthetic methodologies.4 They include generation of a trimethylenemethane dianion equivalent by a metal-mediated bisalkylation process [eqn. (1)],5 alkylation of 1,3-dicarbonyl type compounds [eqn. (2)]),6 a [3 + 2] cyclo-addition reaction to generate five-membered carbocycles [eqn. (3)],7 a two-atom8 as well as a one-atom9 carbocycle-enlargement [eqn. (4)], a gem-allyl dianion equivalent [eqn. (5)],10 and a synthesis of β-hydroxy esters [eqn. (6)]11 which were all developed in air and water. A mechanistic investigation of these reactions was also carried out12 along with their application in the synthesis of three types of natural products: polyhydroxylated natural products [eqn. (7)],13 medium-sized ring natural products,14 and macrocyclic compounds.15 |  | (1) |
|  | (2) |
|  | (3) |
|  | (4) |
|  | (5) |
|  | (6) |
| 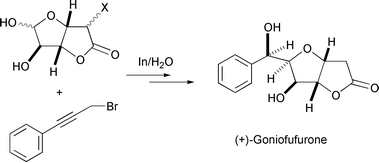 | (7) |
Catalytic reactions in air/water. Early successes in developing aqueous Barbier–Grignard type reactions have been mostly limited to the use of organic halides involving an activated C–X bond (mainly allyl halides). An effort was thus made to extend the scope of the reactions by using transition metals to activate non-activated C–X bonds in air and water. Although a number of transition-metal catalyzed reactions are successful in aqueous media,16 these catalyses are known to be very sensitive to air. Noting that the widely used Mn–Cu batteries operate in air and water, the combination of these two metals was examined for catalyzed C–C reactions in water. It was found that the combination of manganese and a catalytic amount of copper was a highly regioselective mediator for the allylation of aryl aldehydes in air and water [eqn. (8)].17 An exclusive selectivity was also observed when both aliphatic and aromatic aldehyde functionalities were present in the same molecule. The reaction however is still limited to an allylation reaction. Additionally, in the presence of acetic acid or ammonium chloride, manganese and magnesium were found to effect pinacol-coupling reactions in water18 and also proceeded selectively with aryl aldehydes. |  | (8) |
The use of a palladium catalyst was due to an effort in effecting coupling between carbonyls and organic halides involving a sp2-C–halogen bond in air and water. Palladium catalysts are known to be particularly effective in activating such bonds, which is the basis of many important synthetic reactions.19 When iodobenzene was reacted with benzaldehyde and zinc together with a palladium catalyst in air and water, the desired carbonyl addition product was not generated, and instead a Ullmann-type phenyl–phenyl coupling product was obtained [eqn. (9)].20 The palladium catalyst was also effective for Stille coupling, Sonigashira coupling and Suzuki coupling in air and water, although with lower conversions. The study also led to the development of a simple synthesis of unsymmetrical biaryls through palladium-catalyzed coupling of arylsilanes with aryl halides in air and water [eqn. (10)]. 21 On a different front, we have also developed a highly efficient aryl halide–acetylene gas coupling reaction catalyzed by palladium in water [eqn. (11)],22 which has been used for the synthesis of various poly(arene ethylene) materials [eqn. (12)].23
|  | (9) |
|  | (10) |
|  | (11) |
|  | (12) |
Although the use of palladium catalysts provided us with a way of activating organic halides involving a sp2-C–halogen bond in air and water, further reaction of the transient organopalladium intermediate with aldehydes was not successful. Thus the remaining obstacle was the addition of the sp2-C–M organometallic intermediate on to carbonyl groups. In order to choose a suitable organometallic reagent, the general requirement for the success of the desired reactions was that this reagent be stable in air and water. A variety of aryl and vinyl derivatives of metals including B, In, Si, Sn, Ge, Pb, As, Sb, and Bi, were tested.
A screening of various transition-metal catalysts available in our laboratory was carried out, Rh2(COD)2Cl2 and Rh(COD)2BF4 [and to a lesser extent, Ni(acac)2] were found to provide the desired aldehyde addition and conjugated addition products smoothly in air and water [eqn. (13)]. Except for organoarsenic and organoantimony reagents, aryl or vinyl derivatives of all the other metals (and metalloids) examined were able to generate the desired carbonyl addition and conjugated addition products with varied efficiencies. Among them, aryl and vinyl tin,24 silicon,25 boron,26 lead,27 and bismuth28 derivatives were found to be the most effective. The corresponding indium and germanium reagents provided only low yields of the products. The conjugated additions were found to be highly selective—when either a mono- or di-substituted alkene was involved, the reaction proceeded rapidly whereas essentially no reaction was observed with tri-substituted derivatives. This led to a new synthesis of amino acids [eqn. (14)].29 The success of both the carbonyl addition and the conjugated addition was found to be highly dependent upon both the metal and the substituents on the metal.30
|  | (13) |
|  | (14) |
Stage 2: Eliminating the requirements of stoichiometric metals and organic halides
Reactions through electrochemistry. Although the above successes have overcome the major concerns related to carbanion reactions, the use of stoichiometric amounts of metal has the disadvantage of leading to a significant amount of metal waste. One way to overcome this is through the use of electricity as the electron source to regenerate the metal mediator so that only a catalytic amount of metal need be used. There has been extensive literature on the reductive generation of metals via electrochemistry31 and various aqueous methodologies have provided the theoretical foundation for performing the desired electrochemistry-based reactions. However, the drawbacks to this method are the requirement to synthesize the organic halide and the generation of stoichiometric halide waste. Reactions through C–H activation in air and water. The ultimate goal of our research is to eliminate all the concerns outlined in Fig. 1 (and carbanion-based reactions) and to obtain a theoretical 100% atom-efficiency.32 To do this, we decided to develop related reactions through C–H activation33 in air and water. The study of the stoichiometric reactions already provided us with the foundation for this ultimate endeavor.The first investigation was on the activation of sp3 allylic C–H bonds by using ruthenium catalysts in air and water. Through the catalysis of RuCl2(PPh3)3, allylic C–H bonds can be activated, and the functional groups of homoallyl alcohols are repositioned to give allyl alcohols [eqn. (15)].34 Under the same reaction conditions, the functional groups of allyl alcohols also underwent allylic rearrangements. With a water-soluble ruthenium catalyst, a living catalytic system is possible in which the catalyst can be (in principle) recycled many times.35 It still remains to be seen whether the organoruthenium intermediate resulting from the allylic C–H activation can be captured by carbonyls to form C–C bonds in air and water.
|  | (15) |
The second investigation is on the activation of sp C–H bonds in air and water. Our studies show an effective addition reaction of acetylenes to aldehydes in air and water via C–H activation [eqn. (16)],36 and the reaction has been extended to other electrophiles. Also, significant progress has been made in the activation of sp2 C–H bonds for related reactions in air and water.37
|  | (16) |
Conclusion and outlook
We have shown that it is possible to eliminate all the auxiliary factors in the classical carbanion-based reactions in a stepwise manner and to transform them into more atom-economical reactions. As Anastas and Warner pointed out that green chemistry is a journey rather than a conclusion,1 the long-term objective of this endeavor is ultimately to develop C–H activation based C–C bond formations in air and water by using water-soluble catalysts.In addition to the usual advantage of water being not flammable, explosive or toxic, water-soluble substrates can be used directly avoiding protection/deprotection of functional groups, thus saving many synthetic steps. In large industrial processes, isolation of the organic products can be performed by simple phase-separation (as shown by other aqueous catalysis).38 The open-air feature offers convenience in the operations of chemical synthesis and catalyst recycling.
Acknowledgements
I wish to thank all the colleagues who have contributed to the field of Organic Reactions in Water. Particular acknowledgements are given to coworkers in my group whose names appear in the references. I am especially grateful to the support provided over many years by the National Science Foundation (Early CAREER Award), the NSF-EPA Joint Program for a Sustainable Environment, the Petroleum Research Fund administered by the American Chemical Society, the Louisiana Board of Regents, and Tulane University.References
- P. T. Anastas and J. C. Warner, Green Chemistry: Theory and Practice, Oxford University Press, Oxford, 1998 Search PubMed.
- T. A. Killinger, N. A. Boughton, T. A. Runge and J. Wolinsky, J. Organomet. Chem., 1977, 124, 131 CrossRef CAS.
- C. J. Li and T. H. Chan, Organic Reactions in Aqueous Media, John Wiley & Sons, New York, 1997 Search PubMed; C. J. Li, Tetrahedron, 1996, 52, 5643 Search PubMed; T. H. Chan and M. B. Isaac, Pure Appl. Chem., 1996, 68, 919 CrossRef CAS; C. J. Li and T. H. Chan, Tetrahedron, 1999, 55, 11149 CrossRef CAS; A. Lubineau, J. Auge and Y. Queneau, in Organic Synthesis in Water, ed. P. A. Grieco, Blackie Academic & Professional, Glasgow, 1998 CrossRef CAS.
- C. J. Li and T. H. Chan, Tetrahedron Lett., 1991, 32, 7017 CrossRef CAS; L. A. Paquette, in Green Chemistry: Frontiers in Benign Chemical Synthesis and Progresses, ed. P. T. Anastas and T. C. Williamson, Oxford University Press, 1998 Search PubMed.
- C. J. Li, Tetrahedron Lett., 1995, 36, 517 CrossRef CAS.
- C. J. Li and Y. Q. Lu, Tetrahedron Lett., 1995, 36, 2721 CrossRef CAS.
- Y. Q. Lu and C. J. Li, Tetrahedron Lett., 1996, 37, 471 CrossRef CAS.
- C. J. Li, D. L. Chen, Y. Q. Lu, J. X. Haberman and J. T. Mague, J. Am. Chem. Soc., 1996, 118, 4216 CrossRef CAS; C. J. Li, D. L. Chen, Y. Q. Lu, J. X. Haberman and J. T. Mague, Tetrahedron, 1998, 54, 2347 CrossRef CAS.
- J. X. Haberman and C. L. Li, Tetrahedron Lett., 1997, 38, 4735 CrossRef CAS.
- D. L. Chen and C. J. Li, Tetrahedron Lett., 1996, 37, 295 CrossRef CAS.
- X. H. Yi, Y. Meng and C. J. Li, Tetrahedron Lett., 1997, 38, 4731 CrossRef CAS.
- C. J. Li and W. C. Zhang, J. Am. Chem. Soc., 1998, 120, 9102 CrossRef CAS.
- X. H. Yi, Y. Meng and C. J. Li, J. Org. Chem., 1998, 63, 7472 CrossRef CAS; X. H. Yi, Y. Meng and C. J. Li, Chem. Commun., 1998, 449 RSC; X. G. Hua and C. J. Li, Main Group Met. Chem., 1999, 22, 533 Search PubMed; X. G. Hua, J. T. Mague and C. J. Li, Tetrahedron Lett., 1998, 39, 6837 CrossRef CAS.
- X. H. Yi, Ph.D Dissertation, Tulane University, 1998 Search PubMed; X. G. Hua, Ph.D Dissertation, Tulane University, 2000 Search PubMed; Y. Meng, Ph.D Dissertation, Tulane University, 2001 Search PubMed.
- J. X. Haberman, Ph.D Dissertation, Tulane University, 2001 Search PubMed.
- Aqueous-Phase Organometallic Catalysis: Concepts and Applications, ed. W. A. Herrmann and B. Cornils, Wiley-VCH, Weinheim, New York, 1998; B. Cornils and E. Wiebus, CHEMTECH, 1995, 25, 33 Search PubMed; E. G. Kuntz, CHEMTECH, 1987, 17, 570 Search PubMed.
- C. J. Li, Y. Meng, X. H. Yi, J. H. Ma and T. H. Chan, J. Org. Chem., 1997, 62, 8632 CrossRef CAS; C. J. Li, Y. Meng, X. H. Yi, J. H. Ma and T. H. Chan, J. Org. Chem., 1998, 63, 7498 CrossRef CAS.
- W. C. Zhang and C. J. Li, J. Chem. Soc., Perkin Trans. 1, 1998, 3131 RSC; W. C. Zhang and C. J. Li, J. Org. Chem., 1999, 43, 3230 CrossRef CAS.
- For representative reviews, see: B. M. Trost, Tetrahedron, 1977, 33, 2615 Search PubMed; B. M. Trost, Acc. Chem. Res., 1990, 23, 34 CrossRef CAS; J. Tsuji, Top. Curr. Chem., 1980, 91, 29 CrossRef CAS; M. Kumada, Pure Appl. Chem., 1980, 52, 669 Search PubMed; E. I. Negishi, Acc. Chem. Res., 1982, 15, 340 CAS; J. Tsuji, Organic Synthesis with Palladium Compounds, Springer-Verlag, Berlin, 1980 CrossRef CAS; J. E. Baeckvall, Pure Appl. Chem., 1983, 55, 1669 CrossRef CAS; R. H. Heck,Palladium Reagents for Organic Synthesis, Academic Press, New York, 1985 Search PubMed; R. C. Larock, Adv. Met.-Org. Chem., 1994, 3, 97 Search PubMed.
- S. Venkatraman and C. J. Li, Tetrahedron Lett., 2000, 41, 4831 CrossRef CAS; S. Venkatraman and C. J. Li, Org. Lett., 1999, 1, 1133 CrossRef CAS.
- T. Huang and C. J. Li, Tetrahedron Lett., 2002, 43, 403 CrossRef CAS.
- C. J. Li, D. L. Chen and C. W. Costello, Org. Res. Process. Dev.., 1997, 1, 315 Search PubMed.
- C. J. Li, W. T. Slaven, V. T. John and S. Banerjee, Chem. Commun., 1997, 1569 RSC; C. J. Li, W. T. IV. Slaven, Y. P. Chen, V. T. John and S. H. Rachakonda, Chem. Commun., 1998, 1351 RSC.
- C. J. Li and Y. Meng, J. Am. Chem. Soc., 2000, 122, 9538 CrossRef CAS; S. Venkatraman, Y. Meng and C. J. Li, Tetrahedron Lett., 2001, 42, 4459 CrossRef CAS.
- T. S. Huang and C. J. Li, Chem. Commun., 2001, 2348 Search PubMed.
- C. J. Li, T. S. Huang, S. Venkatraman, Y. Meng, D. Kort, R. Ding and D. Wang, Pure Appl. Chem., 2001, 73, 1315 Search PubMed ; see also independent work: M. Ueda and N. Miyaura, J. Org. Chem., 2000, 65, 4450 Search PubMed; T. Hayashi and M. Ishigedani, J. Am. Chem. Soc., 2000, 122, 976 CrossRef CAS.
- R. Ding, Y. J. Chen, D. Wang and C. J. Li, Synlett, 2001, 1470 CrossRef CAS.
- S. Venkatraman and C. J. Li, Tetrahedron Lett., 2001, 42, 781 CrossRef CAS.
- T. S. Huang and C. J. Li, Org. Lett., 2001, 3, 2037 CrossRef CAS.
- T. S. Huang, S. Venkatraman, Y. Meng, D. Wang and C. J. Li, J. Am. Chem. Soc., 2001, 121, 7451 CrossRef CAS.
- R. H. Prenner, W. H. ; Binder and W. Schmid, Justus Liebigs Ann. Chem., 1994, 73 Search PubMed; S. Marshall, W. C. Zhang and C. J. Li, unpublished results.
- B. M. Trost, Science, 1991, 254, 1471 CrossRef CAS; R. A. Sheldon, CHEMTECH, 1994, 24, 38 Search PubMed; P. A. Wender and B. L. Miller, in Organic Synthesis: Theory and Applications, ed. T. Hudlicky, JAI Press, 1993, vol. 2 Search PubMed.
- G. Dyker, Angew. Chem., Int. Ed., 1999, 38, 1698 CrossRef; T. Naota, H. Takaya and S. I. Murahashi, Chem. Rev., 1998, 98, 2599 CrossRef CAS; K. Horn, Chem. Rev,, 1995, 95, 1317 CrossRef CAS.
- C. J. Li, D. Wang and D. L. Chen, J. Am. Chem. Soc., 1995, 117, 12867 CrossRef CAS; C. J. Li, D. Wang and D. L. Chen, Tetrahedron, 1998, 54, 5159.
- J. X. Haberman, Ph.D Thesis, Tulane University, 2001 Search PubMed.
- C. J. Li and C. M. Wei, Chem. Commun., 2002, in press Search PubMed; C. M. Wei and C. J. Li, Green Chem., 2002, 4, in press Search PubMed.
- S. Ventrakaman, G. Viswanathan, C. M. Wei, J. H. Zhang, T. S. Huang and C. J. Li, unpublished results.
- J. Hagen, Industrial Catalysis: A Practical Approach, Wiley-VCH, Weinheim, New York, 1999 Search PubMed; J. P. Arhancet, M. E. Davis, J. S. Merola and B. E. Hanson, Nature, 1989, 339, 454 Search PubMed.
|
This journal is © The Royal Society of Chemistry 2002 |
Click here to see how this site uses Cookies. View our privacy policy here.