DOI:
10.1039/B103851F
(Paper)
Lab Chip, 2001,
1, 61-63
Preliminary evaluation of electrochemical PNA array for detection of single base mismatch mutations
Received
30th April 2001
, Accepted 13th July 2001
First published on 9th August 2001
Abstract
We report here a preliminary evaluation of a microfabricated disposable-type peptide nucleic acid (PNA) array, with a 20-channel electrode, for the detection of cancer gene c-Ki-ras. Synthetic 15-mer PNA probes complimentary to ras sequence modified with cysteine were immobilized on the gold electrodes on the array. The electrochemical PNA array was reacted with 20-mer oligonucleotide target or 128 bp PCR product for 1 h. The anodic current derived from an electrochemically active DNA binder Hoechst 33258 was measured using the PNA array in the 50 μL of reaction chamber. The anodic current from Hoechst 33258 increased with increasing the concentration of PCR product of ras gene in the range from 1011 to 1015 copy mL−1. The single base mismatch mutations of c-Ki-ras/61 were also detected using the electrochemical PNA array.
1. Introduction
The DNA microarray is now a key device in the field of gene analyses and is expected to apply to DNA diagnostics such as cancers and common diseases. Both Affymetrix and the Stanford type of microarrays are now in popular use for gene expression and SNP analyses; however these require complicated fluorochrome labeling, expensive chips and fluorescence analysis equipment.1–4 On the other hand, development of new DNA microarrays, which have solved the problems mentioned above have also been furthered.5–7
The electrochemical methods for a sequence specific detection of the target DNA have been reported since the early 1990s.8–12 Some of these methods use an electrochemically active DNA binder (e.g. intercalator) as a hybridization indicator instead of labeling of the target DNA as used for conventional methods. We found that Hoechst 33258, which is known as a minor groove binder and specifically binds to double stranded DNA, is electrochemically active and useful for electrochemical DNA detection.13,14 The complicated labeling and expensive equipment are not necessary for these electrochemical methods.15 However, these methods have to solve the low S/N problem since most of DNA binder bind to not only formed hybrids but also single stranded DNA probes by electrostatic interaction.
Peptide nucleic acid (PNA), which is a synthetic and neutral oligomer, binds to complementary DNA with greater stability than the DNA–DNA duplex with high specificity.16 Hoechst 33258 hardly binds to PNA (data not shown) due to its neutral properties. It is expected that the PNA probe prevents the electrostatic adsorption of the DNA binder to the immobilized probe and reduces the background signal with the electrochemical DNA detection method.
We report here the preliminary results of the electrochemical array using a PNA probe and an electrochemically active intercalator, Hoechst 33258, for determining the single base mismatch mutations of the cancer gene c-Ki-ras/61.
2. Materials and methods
2.1 Disposable PNA array
The 4 in Pyrex glass plate (d = 0.8 mm) coated with both a titanium thin film (99.9%, 50 nm) and a gold thin film (99.99%, 200 nm) was purchased from Kawakita-riko (Kawasaki, Japan). The electrodes were photo-lithographically processed on the plate using a photo-resist, AZP4620A (Hoechst Industry Corp., Tokyo, Japan). The photo-resist was baked at 200 °C for 30 min for the purpose of post baking and permanent insulating of the electrochemical array. The array (20 × 30 mm) consists of 20 working electrodes (ϕ = 200 μm, 2 mm in pitch) including immobilized PNA probes, a reference electrode (0.2 × 9.3 mm), and a counter electrode (0.2 × 6.4 mm) (Fig. 1). The working electrodes are connected to the pad for connection with an electrochemical analyzer. All electrodes are made of gold. The characteristics of the disposable array were estimated by measuring the cathodic current in a 0.5 mol L−1 sulfuric
acid solution by cyclic voltammetry scanned from −0.2 to 1.5 V at 50 V s−1. This value reflects the surface area and the surface conditions such as the crystal structure of the electrode.
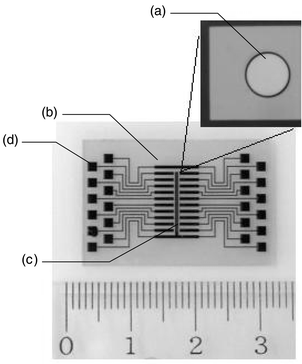 |
| Fig. 1
Structure of the microfabricated PNA array. The working electrode (a), the counter electrode (b), the reference electrode (c) and connecting pad (d).
| |
2.2 Apparatus
Electrochemical analyses were carried out using an electrochemical analyzer (Model CV-50 W) manufactured by Bioanalytical Systems Inc. (West Lafayette, IN). The scanner (Model 7001, Keithley Instruments Inc., Cleveland, OH), which is inserted in the 40 channel multi-plex card (7011-S), was used for switching. The electrochemical signals derived from each electrode on the array were sequentially measured by use of the switching scanner and the electrochemical analyzer.
2.3 Preparation of probes and targets
PNA probes having a cysteine at the N-terminal end listed in Table 1 were purchased from Sawady Technology (Tokyo, Japan). An oligonucleotide target, c-Ki-ras gene primer set, c-Ki-ras mutant set, and thermo stable polymerase Takara Ex-Taq were obtained from Takara-Shuzo Co. Ltd. (Tokyo, Japan). A PCR was cycled 30 times using the thermal cycler PC 700 (Astec, Fukuoka, Japan) and the PCR cycle included denaturation at 94 °C for 30 s, the primer annealing at 55 °C for 120 s, and the primer extension at 72 °C for 30 s. The amplified DNA was purified using a Suprec-02 spin column (Takara-Shuzo).
Table 1
Sequences of probes and target
|
Name |
Sequencea |
Cys: cysteine; O: ethylene glycol linker.
Ribosomal DNA of E. coli.
|
Probe |
W-Gln (wild type) |
NH2–Cys–O–O–CTCCTCTTTACCTGC–H |
|
M-Lys (mutant) |
NH2–Cys–O–O–CTCCTCTTCACCTGC–H |
|
M-Glu (mutant) |
NH2–Cys–O–O–GACCTGCTTCTGACT–H |
|
rDNAb |
NH2–Cys–O–O–CTCCTCTTGACCTGC–H |
Target |
w-Gln (wild type) |
5′-ACAGCAGGTCAAGAGGAGTA-3′ |
2.4 Immobilization of PNA probe
The PNA probe was immobilized on the gold electrode through a thiol group in the cysteine introduced into the N-terminal end. 0.2 μL of 20 μg mL−1 PNA probe solution [10 mmol L−1 phosphate buffer (pH 7)] was spotted on the electrode surface using a micro pipet (Model P-2, Gilson Medical Electronics S.A., Villiers-le-Bel, France) and the array was covered with a reaction chamber (50 μL, Grace Bio-Labs Inc., Bend, OR) to prevent drying and left standing for 1 h at room temperature. Then, the array was washed with distilled water and reacted with 1 mmol L−1 2-mercaptoethanol (Wako Pure Chemicals) in the reaction chamber for 30 min to prevent non-specific adsorption of both target DNA and Hoechst 33258.11 After washing with distilled water, the array was stored at 4 °C.
2.5 c-Ki-ras detection with the electrochemical PNA array
The PNA array was reacted with a hybridization buffer [300 mmol L−1 sodium chloride, 30 mmol L−1 sodium citrate (pH 7), 1 mmol L−1 EDTA, denoted as 2 × SSC-1 mmol L−1 EDTA] containing the oligonucleotide or PCR product denatured in boiling water for 3 min. The hybridization reaction was carried out at 50 °C for 1 h in the reaction chamber using thermal cycler Crocodille III (Appligene S.A., Illkirch, France). After the reaction, the array was washed with 0.2 × SSC-1 mmol L−1 EDTA buffer at 50 °C for 30 min to remove the non-specifically bound DNA. Then, the array was reacted with 10 μmol L−1 Hoechst 33258 (Wako Pure Chemicals Industries Ltd., Tokyo, Japan) solution [7 mmol L−1 sodium phosphate (pH 7.5), 180 mmol L−1 sodium chloride, ion strength µ
= 0.2] in the reaction chamber at room temperature for 15 min
under dark conditions. Hoechst 33258 binds to the hybrids formed on the electrode. Then, the anodic peak current (ipa) derived from Hoechst 33258 was measured by linear sweep voltammetry (LSV), and used to determine the quantity of target DNA. LSV is sequentially operated for each working electrode using a switching scanner. The potential is scanned from −300 to 900 mV at 100 mV s−1 to measure the anodic current correctly. The anodic peak potentials of Hoechst 33258, which derived from the electrode array using the gold reference electrode, are ranging from 520 to 590 mV on the array. These are almost the same potential as using a Ag/AgCl reference electrode (550 ± 5 mV).
3. Results and discussion
3.1 Characterization of the disposable DNA array
Before the probe immobilization, the electrochemical property of the disposable array was estimated by measuring the cathodic current in a sulfuric acid solution. The cyclic voltammogram showed the typical wave of gold in a sulfuric acid solution. It was assumed that the clean surface of the gold electrode was obtained for the PNA probe immobilization.17 Furthermore, a low scatter of the cathodic current with a coefficient of variation (CV) of less than 5% (n = 20 in the array) indicated the uniformity of the electrodes in the array. We speculated that the diffusion layers of neighboring electrodes are not perturbed in this array.
3.2 Probe immobilization
Fig. 2 shows the linear sweep voltammogram of Hoechst 33258 using a bare and a PNA probe-immobilized electrode. After immobilization, the ipa of Hoechst 33258 decreased. The anodic current derived from each working electrode after probe immobilization is 31.5 ± 0.7 nA for W-Gln (n = 2). We guess that the electrode covered with neutral PNA probe prevents the electrochemical reaction of Hoechst 33258. In the case of DNA probe, the ipa increased after immobilization because of electrostatic interaction between a negatively charged DNA probe and a positively charged Hoechst 33258.12
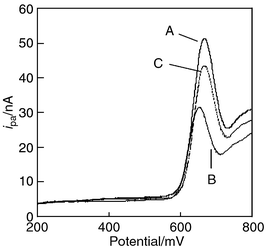 |
| Fig. 2
Linear sweep voltammograms of Hoechst 33258 using a bare electrode (A), a PNA probe immobilized electrode (B), and the PNA probe immobilized electrode which is hybridized with target DNA w-Gln (C). Hoechst 33258: 10 μmol L−1. Sweep rate: 100 mV s−1.
| |
3.3 c-Ki-ras detection
We estimated the sensitivity of the disposable PNA array for detection of the oligonucleotide and PCR product containing c-Ki-ras sequence. The probe (W-Gln) immobilized electrodes in the array were reacted with 0.2 μL of hybridization buffer. Both intercalation and electrochemical measurements were carried out in a single electrolyte solution. The dilution experiments with oligonucleotide indicated that the detection threshold of the DNA array was 1013 copy mL−1 (3 fmol) in the hybridization buffer (Fig. 3). Furthermore, the ipa values derived from the Hoechst 33258 bound to the hybrids formed on the electrodes increased with increasing concentration of target oligonucleotide in the range 1013 to 1015 copy mL−1. In the case of PCR product, the detection limit was 1011 copy mL−1 (30 amol) and ipa values related
to the concentration ranging from 1011 to 1015 copy mL−1. Higher sensitivity for the PCR product was explained by the fact that Hoechst 33258 binds more strongly to the longer chain of the PCR product compared with the oligonucleotide.
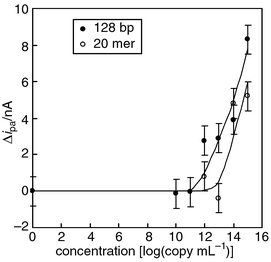 |
| Fig. 3
Calibration curve for the 20-mer oligonucleotide (w-Gln) and the PCR product containing c-Ki-ras sequence using the electrochemical PNA array.
| |
3.4 Detection of single base mismatch mutation of c-Ki-ras/61
Since the PNA array is expected for the detection of single nucleotide polymorphism (SNP), the single base mismatch mutation of c-Ki-ras/61 was detected as a model using the electrochemical array. The PNA probes for ras (wild type: W-Gln, mutant: M-Lys and M-Glu) and rDNA of E. coli as a negative control were immobilized on the electrode. Then, the array was reacted with 50 μL of the target, 20-mer oligonucleotide (w-Gln), in the reaction chamber. The ipa from the rDNA probe immobilized electrode didn’t change, while the ipa from the fully compliment probe (wild type) immobilized electrode increased and 3 or more times higher values were observed over the other probe (mutant) immobilized electrodes (Table 2). It was shown that detection of the single base mismatch mutations is possible using the electrochemical PNA array.
Table 2
Detection of single base mismatch mutations of c-Ki-ras/61a
Probe |
Δipab |
Target: 20-mer oligonucleotide (w-Gln) fully matched for W-Gln probe, 1015 copy mL−1.
Anodic current derived from Hoechst 33258 (n = 2).
Ribosomal DNA of E. coli.
|
W-Gln (wild type) |
7.9 ± 0.5 |
M-Lys (mutant) |
2.6 ± 0.6 |
M-Glu (mutant) |
1.3 ± 0.2 |
rDNAc |
−1.4 ± 0.4 |
4. Conclusion
We have demonstrated the electrochemical PNA array for detection of the cancer gene ras. The PNA array using electrochemically active DNA binder can provide a quick and convenient method for determining target DNAs specifically and quantitatively. The PNA has stronger binding affinity for complimentary DNA than its DNA counterpart and a neutral backbone, the single base mismatch mutation (typing) is also detectable by carrying out both interaction of DNA binder and electrochemical measurements in a single electrolyte solution using the array. We emphasize that the electrochemical array will be a powerful device for the medical field in the near future.
References
- S. P. A. Fodor, R. P. Rava, X. C. Huang, A. C. Pease, C. P. Holmes and C. L. Adams, Nature, 1993, 364, 555 CrossRef CAS.
- M. Schena, D. Shalon, R. W. Davis and P. O. Brown, Science, 1995, 270, 467 CrossRef CAS.
- D. Y. Guschin, B. K. Mobarry, D. Proudnikov, D. A. Stahl, B. E. Rittmann and A. D. Mirzabekov, Appl. Environ. Microbiol., 1997, 63, 2397 CAS.
- R. G. Sosnowski, E. Tu, W. F. Butler, J. P. O’Connel and M. J. Heller, Proc. Natl. Acad. Sci. USA, 1997, 94, 1119 CrossRef CAS.
- K. Beattie, M. Eggers and J. Shumaker, Clin. Chem., 1993, 39, 719.
- Y. Okahata, Y. Matsunobu, K. Ijiro, M. Mukae, A. Murakami and K. Makino, J. Am. Chem. Soc., 1992, 114, 8299 CrossRef CAS.
- P. A. E. Piunno, U.
J. Krull, R. H. E. Hudson, M. J. Damha and H. Cohen, Anal. Chim. Acta, 1994, 288, 205 CrossRef.
- K. Hashimoto, K. Miwa, M. Goto and Y. Ishimori, Supramol. Chem., 1993, 2, 265 CrossRef CAS .
This paper was first to highlight the electrochemical method for sequence specific detection of DNA using the electrochemically active DNA binder.
- S.
R. Mikkelsen, Electroanalysis, 1996, 8, 15 CAS .
This paper reviewed the electrochemical DNA detection method.
- M. Maeda, Y. Mitsuhashi, K. Nakano and M. Takagi, Anal. Sci., 1992, 8, 83 CAS.
- S. Takenaka, K. Yamashita, M. Takagi, Y. Uto and H. Kondo, Anal. Chem., 2000, 72, 1334 CrossRef CAS .
This paper reported high sensitive detection of target DNA by a novel electrochemical intercalator.
- K. Hashimoto, K. Ito and Y. Ishimori, Sens. Actuators B, 1998, 46, 220 CrossRef.
- C. Labarca and K. Paigen, Anal. Biochem., 1980, 102, 344 CrossRef CAS.
- K. Hashimoto, K. Ito and Y. Ishimori, Anal. Chem., 1994, 66, 3830 CrossRef CAS.
-
E. K. Wilson, Chem. Eng. News, 1998, May 25, 47 Search PubMed.
- M. Egholm, O. Buchardt, L. Christensen, C. Behrens, S. M. Freier, D. A. Driver, R. H. Berg, S. K. Kim, B. Norden and P. E. Nielsen, Nature, 1993, 365, 566 CrossRef CAS.
-
D. T. Sawyer and J. L. Roberts Jr., Experimental Electrochemistry for Chemists, John Wiley & Sons, New York, 1974, p. 67 Search PubMed.
|
This journal is © The Royal Society of Chemistry 2001 |