DOI:
10.1039/C6RA04016K
(Communication)
RSC Adv., 2016,
6, 44029-44033
The dicarbonylation of indoles via Friedel–Crafts reaction with dicarbonyl nitrile generated in situ and retro-cyanohydrination†
Received
14th February 2016
, Accepted 27th April 2016
First published on 28th April 2016
Abstract
A novel one-pot tandem reaction of indole and β-carbonyl nitrile to generate dicarbonyl indoles has been developed. This tandem process involves α-oxonation of the β-carbonyl nitrile to afford α,β-dicarbonyl nitrile, Friedel–Crafts reaction with indoles and subsequent retro-cyanohydrination of cyano tertiary alcohol form dicarbonyl indoles.
The indole nucleus is a core structural scaffold found in numerous bioactive natural products, pharmaceutical molecules, and functional materials.1 Owing to their ubiquitous nature, the synthesis and further functionalization of indoles have been and continue to be the focus of abundant synthetic studies.2 Among numerous efforts, the C(3)–H functionalization reactions of indoles occupy an important place in the synthesis of natural products and drugs.3
Nitroxides, as stoichiometric and catalytic oxidant, have been broadly used in oxidation reactions of organic compounds.4 Past decade, nitroxides also been applied in transition metal-catalyzed C–C bond forming reactions,5 the α-C–H activation of carbonyl compounds,6 the α-oxygenation of active methylene compounds7 and functionalization of indoles8 and indanes.9 Recently, our research results showed that indole could react with 1,3-dicarbonyl compounds to afford tertiary alcohols via the reactive intermediate vicinal tricarbonyl compounds generated in situ from 1,3-dicarbonyl compounds and TEMPO.10 We envisaged that β-carbonyl nitrile, as a precursor, could react with indole to form novel functional indoles. In order to test this proposal, a mixture of indole (1a), benzoylacetonitrile (2a) (1.1 equiv.), and 2,2,6,6-tetramethyl-1-piperidinyl-oxide (TEMPO, 2.2 equiv.) in acetic acid was stirred at 50 °C open to air for 20 h (eqn (1)). Under these conditions, the process did occur and generated the dicarbonyl indole 3aa in 32% yield. The dicarbonyl indoles are an important class of natural products11 and synthetic intermediate for functional molecules12 and drugs,13 and exhibit different biological activities such as antiparasitic activity,14 antiatherosclerosis,15 anticancer,16 antivirus.17 The traditional synthetic method of C-3 dicarbonyl indoles is mainly through glyoxylation of indoles,18 recent developed procedures include oxidative cross-coupling of indole with α-amino carbonyl compounds,19 aromatic methylketone or α-hydroxyketone,20 the cross-coupling of indole with α-keto-aldehyde.21 Although these valuable results, to develop novel procedure for the dicarbonylation of indole, with a broad functional group tolerance, is still highly needed. Below, we described our results a novel and interesting dicarbonylation process of indole.
|
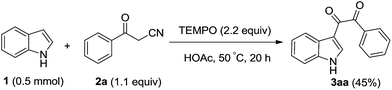 | (1) |
Firstly, studies were carried out to determine optimal conditions for the dicarbonyl indole formation by using indole and benzoylacetonitrile. Increasing the amount of benzoylacetonitrile to 1.5 equivalents and TEMPO to 3.0 equivalents could improve yield obviously (Table 1, entry 1). CuCl could promote the transformation remarkably, adding 0.1 equiv. CuCl into the reaction solution of indole, benzoylacetonitrile and TEMPO, the transformation could complete at room temperature for only 2 hours in 70% yield (Table 1, entry 2). Nitroxides (Table 1, entry 3–6) including 4-hydroxy-2,6-tetramethyl-piperidine-1-oxide (4-hydroxy TEMPO), 4-acetamido-2,2,6,6-tetramethylpiperidine 1-oxide (4-acetamido TEMPO), 4-amino-2,2,6,6-tetramethylpiperidine 1-oxide (4-amino TEMPO), and 4-oxo-2,2,6,6-tetramethylpiperidine 1-oxide (4-oxo-TEMPO) all also could promote the reaction, and 4-oxo-TEMPO was the optimal option. The promoting effect of a series of copper reagents, including CuBr, CuCl2, CuBr2, Cu(OTf)2, were examined (Table 1, entry 7–10). CuBr, CuCl2 and Cu(OTf)2 all displayed efficient promotive action for this transformation, but were inferior to CuCl, CuBr2 gave the poor results. The results of screening solvents indicated that acetic acid was optimal choice (Table 1, entry 6), propionic and butyric acid gave a decreased yield (Table 1, entry 11–12). The exploratory comprehensive results show that the optimized reaction conditions of indole (1 equiv.) with benzoylacetonitrile (1.5 equiv.) to forming dicarbonyl indole involve the use of 3.0 equivalents 4-oxo-TEMPO, CuCl (0.1 equiv.) as a promoter, acetic acid as solvent, at room temperature and open to air for 2 h.
Table 1 Optimization of the dicarbonylation reaction of indole with benzoylacetonitrilea
Entry |
Catalyser |
Oxidizer |
Temp/time |
Yieldb |
Reaction conditions: a mixture of indole 1a (0.5 mmol), benzoylacetonitrile 2a (0.75 mmol), corresponding nitroxides (1.5 mmol) and 0.1 equiv. copper in the acetic acid (5.0 mL) was stirred at the indicated temperatures for the times indicated open to air. Isolated yields. The reaction was stirred in propionic acid. The reaction was stirred in butyric acid. |
1 |
— |
TEMPO |
50 °C/20 h |
52% |
2 |
CuCl |
TEMPO |
r.t./2 h |
70% |
3 |
CuCl |
4-Hydroxy TEMPO |
50 °C/2 h |
20% |
4 |
CuCl |
4-Acetamido TEMPO |
50 °C/2 h |
51% |
5 |
CuCl |
4-Amino TEMPO |
50 °C/2 h |
65% |
6 |
CuCl |
4-Oxo-TEMPO |
r.t./2 h |
87% |
7 |
CuBr |
4-Oxo-TEMPO |
r.t./2 h |
77% |
8 |
Cu(OTf)2 |
4-Oxo-TEMPO |
r.t./2 h |
71% |
9 |
CuCl2 |
4-Oxo-TEMPO |
r.t./2 h |
65% |
10 |
CuBr2 |
4-Oxo-TEMPO |
50 °C/2 h |
21% |
11c |
CuCl |
4-Oxo-TEMPO |
50 °C/6 h |
35% |
12d |
CuCl |
4-Oxo-TEMPO |
50 °C/6 h |
49% |
Next, various β-carbonyl nitriles 2 were used to explore the substrate scope of this reaction under the optimized conditions. The results (Table 2) showed that the efficiency of the reaction is not influenced by both the steric bulkiness and the electronic properties of the benzoylacetonitrile derivatives. Specifically, benzoylacetonitriles possessing a variety of electronically different substituents, including fluoro, chloro, bromo, methyl, and methoxy groups at different positions on the benzene ring undergo the reaction to produce the corresponding substituted indoles in good to excellent yields (Table 2, compounds 3ab–3ah). Moreover, β-carbonyl nitrile substrates containing heterocycle moieties, for example 2-furoylacetonitrile and 2-thienoylacetonitrile, also react with indole to generate the corresponding dicarbonylation indoles in excellent yields (Table 2, compounds 3ai and 3aj). However, when alkyl substituted β-carbonyl nitrile such as pivaloylacetonitrile or ethyl 2-cyanoacetate subjected to the optimized reaction conditions, no desired target product generated (Table 2, 3ak and 3al).22 Additionally, malononitrile also react with indole to result in 1H-indole-3-carbonyl cyanide in perfect yield under the standard conditions (Table 2, 3am).
Table 2 The β-carbonyl nitrile scopea
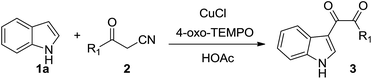
|
All reactions were run under optimized conditions, isolated yields after column chromatography. |
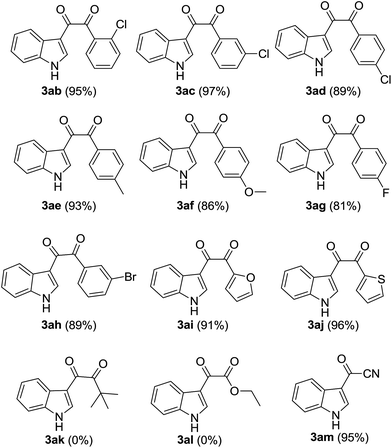 |
Finally, the indole scope of this process was also examined (Table 3). During the exploratory study, we found that the electronic effect of substituents on indole ring greatly affect the transformation results and reaction conditions. Indoles containing electron-donating groups on benzene ring could react with benzoylacetonitrile to afford dicarbonylation products in good to excellent yields at 80 °C for 2 hours (Table 3, compounds 4ba, 4ca and 4la). 5-Hydroxyindole also transforms smoothly in the reaction and results in the target product in reasonable yield (Table 3, 4ga). Indoles possessing fluoro, chloro, and bromo at different place on the benzene ring need to maintain the reaction solution at 100 °C for 3 h to transform completely and afford the corresponding dicarbonyl indole derivatives in moderate yields (Table 3, compounds 4da–4fa, 4ia–4ka). Strong electron-withdrawing group substituted indole didn't transform effectively and no corresponding product generated (Table 3, 4ha). 2-Methyl-indole could be transformed into dicarbonyl indole compound in only 35% yield. N-methyl- and N-benzyl-indole also react with benzoylacetonitrile in the presence of 4-oxo-TEMPO to form the coupling products in 92% and 65% yields, respectively (Table 3, 4na and 4oa). Interestingly, N-methyl indole reacted with pivaloylacetonitrile to generate corresponding dicarbonyl indole in perfect yield when the reaction was conducted at 80 °C for 2 hours (eqn (2)).
|
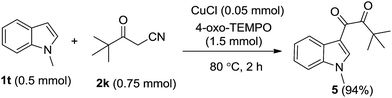 | (2) |
Table 3 Indole scope of the dicarbonylation reactiona
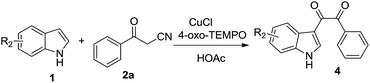
|
Reactions conditions: 0.5 mmol indoles, 0.75 mmol benzoylacetonitrile, and 0.05 mmol CuCl in HOAc were stirred at the indicated temperature for the times indicated yields after column chromatography. Reaction at 80 °C for 2 h. Reaction at 50 °C for 1 h. |
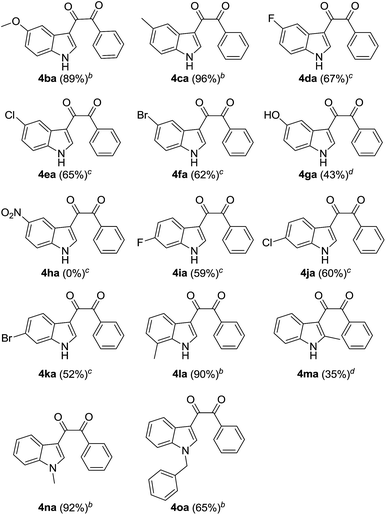 |
A plausible mechanism for this process was suggested in Scheme 1. Firstly, 4-oxo-TEMPO through disproportionation reaction generate the N-oxo-piperidinium ion A under acidic conditions.23 Accordingly, reaction between the active β-carbonyl nitrile and N-oxo-piperidinium ion produces α,β-dicarbonyl nitrile B undergoing aminoxylation of benzoylacetonitrile and loss of 4-oxo-tetramethylpiperidine (4-oxo-TEMPH).24 In presence of acetic acid, through the Friedel–Crafts reaction of indole and B to form adduct cyano tertiary alcohol C.25 Finally, the retro cyanohydrination26 of active intermediate C results in the final product 3aa.
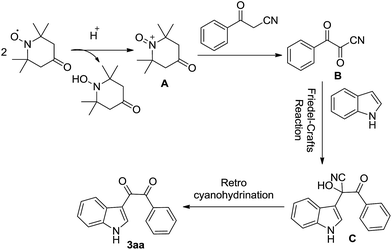 |
| Scheme 1 Plausible reaction mechanism. | |
In conclusion, we have developed a novel tandem reaction of indole and β-carbonyl nitrile to generate dicarbonyl indoles. The process, involving the disproportionation of 4-oxo-TEMPO/α-aminoxylation of β-carbonyl nitrile and loss of 4-oxo-TEMPH to form α,β-dicarbonyl nitrile/Friedel–Crafts reaction with indole/retro-cyanohydrination of cyano tertiary alcohol, leads to the formation of dicarbonyl indoles in a domino one-pot manner. This method provides a new pathway for synthesis of functional dicarbonyl indoles under simple conditions. Further investigations are now underway to probe the further scope and applications of the method.
Acknowledgements
This work was financially supported by Post-Doctoral Research Projects of Henan Province (No. 2013039), the National Natural Science Foundation of China (No. 21371148) and Key Scientific and Technological Project of Henan Province (No. 1221023710196).
Notes and references
- J. C. Badenock and G. W. Gribble, Heterocyclic Scaffolds II: Reactions and Applications of Indoles, Springer, Science & Business Media, 2010, vol. 2, p. 2 Search PubMed.
- For examples of selected reviews, see:
(a) S. Cacchi and G. Fabrizi, Chem. Rev., 2005, 105, 2873–PR215 CrossRef CAS PubMed;
(b) L. Joucla and L. Djakovitch, Adv. Synth. Catal., 2009, 351, 673–PR215 CrossRef CAS;
(c) M. Bandini and A. Eichholzer, Angew. Chem., Int. Ed., 2009, 48, 9608–PR215 CrossRef CAS PubMed;
(d) S. Cacchi and G. Fabrizi, Chem. Rev., 2011, 111 CrossRef PubMed;
(e) G. Bartoli, R. Dalpozzo and M. Nardi, Chem. Soc. Rev., 2014, 43, 4728–PR215 RSC.
- For recent reviews:
(a) M. Ishikura, T. Abe, T. Choshi and S. Hibino, Nat. Prod. Rep., 2015, 32, 1389–PR215 RSC;
(b) M. E. Tanner, Nat. Prod. Rep., 2015, 32, 88–PR215 RSC;
(c) M. Ishikura, T. Abe, T. Choshi and S. Hibino, Nat. Prod. Rep., 2013, 30, 694–PR215 RSC.
- For selected reviews:
(a) R. A. Sheldon, I. W. C. E. Arends, G.-J. ten Brink and A. Dijksman, Acc. Chem. Res., 2002, 35, 774–PR215 CrossRef CAS PubMed;
(b) R. A. Sheldon and I. W. C. E. Arends, Adv. Synth. Catal., 2004, 346, 1051–PR215 CrossRef CAS;
(c) T. Vogler and A. Studer, Synthesis, 2008, 1979–PR215 CAS;
(d) L. Tebben and A. Studer, Angew. Chem., Int. Ed., 2011, 50, 5034–PR215 CrossRef CAS PubMed.
-
(a) T. Vogler and A. Studer, Org. Lett., 2007, 10, 129–PR215 CrossRef PubMed;
(b) S. Kirchberg, T. Vogler and A. Studer, Synlett, 2008, 2841–PR215 CAS;
(c) S. Kirchberg, R. Fröhlich and A. Studer, Angew. Chem., Int. Ed., 2009, 48, 4235–PR215 CrossRef CAS PubMed;
(d) S. Kirchberg, R. Fröhlich and A. Studer, Angew. Chem., Int. Ed., 2010, 49, 6877–PR215 CrossRef CAS PubMed;
(e) S. Kirchberg, S. Tani, K. Ueda, J. Yamaguchi, A. Studer and K. Itami, Angew. Chem., Int. Ed., 2011, 50, 2387–PR215 CrossRef CAS PubMed;
(f) Z. He, S. Kirchberg, R. Fröhlich and A. Studer, Angew. Chem., Int. Ed., 2012, 51, 3699–PR215 CrossRef CAS PubMed;
(g) Z. He, B. Wibbeling and A. Studer, Adv. Synth. Catal., 2013, 355, 3639–PR215 CrossRef CAS.
- For selected examples:
(a) M. P. Sibi and M. Hasegawa, J. Am. Chem. Soc., 2007, 129, 4124–PR215 CrossRef CAS PubMed;
(b) M. Pouliot, P. Renaud, K. Schenk, A. Studer and T. Vogler, Angew. Chem., Int. Ed., 2009, 48, 6037–PR215 CrossRef CAS PubMed;
(c) K. Akagawa, T. Fujiwara, S. Sakamoto and K. Kudo, Org. Lett., 2010, 12, 1804–PR215 CrossRef CAS PubMed;
(d) K. Akagawa, T. Fujiwara, S. Sakamoto and K. Kudo, Chem. Commun., 2010, 46, 8040–PR215 RSC;
(e) T. Kano, H. Mii and K. Maruoka, Angew. Chem., Int. Ed., 2010, 49, 6638–PR215 CrossRef CAS PubMed;
(f) J. F. Van Humbeck, S. P. Simonovich, R. R. Knowles and D. W. C. MacMillan, J. Am. Chem. Soc., 2010, 132, 10012–PR215 CrossRef CAS PubMed;
(g) S. P. Simonovich, J. F. Van Humbeck and D. W. C. MacMillan, Chem. Sci., 2012, 3, 58–PR215 RSC;
(h) Y. Li, M. Pouliot, T. Vogler, P. Renaud and A. Studer, Org. Lett., 2012, 14, 4474–PR215 CrossRef CAS PubMed.
-
(a) U. Jahn, P. Hartmann, I. Dix and P. G. Jones, Eur. J. Org. Chem., 2002, 2002, 718–PR215 CrossRef;
(b) M. Schämann and H. J. Schäfer, Synlett, 2004, 2004, 1601–PR215 Search PubMed;
(c) E. Dinca, P. Hartmann, J. Smrček, I. Dix, P. G. Jones and U. Jahn, Eur. J. Org. Chem., 2012, 2012, 4461–PR215 CrossRef CAS;
(d) P. Feng, S. Song, L.-H. Zhang and N. Jiao, Synlett, 2014, 25, 2717–PR215 CrossRef CAS.
- S. Kirchberg, R. Fröhlich and A. Studer, Angew. Chem., Int. Ed., 2009, 48, 4235–PR215 CrossRef CAS PubMed.
- S. Kirchberg, R. Fröhlich and A. Studer, Angew. Chem., Int. Ed., 2010, 49, 6877–PR215 CrossRef CAS PubMed.
- J. Yan, T. Ni, F. Yan, J. Zhang and F. Zhuang, RSC Adv., 2015, 5, 89906–PR215 RSC.
-
(a) J. Bergman, T. Janosik and A.-L. Johnsson, Synthesis, 1999, 1999, 580–PR215 CrossRef;
(b) M. J. McKay, A. R. Carroll, R. J. Quinn and J. N. A. Hooper, J. Nat. Prod., 2002, 65, 595–PR215 CrossRef CAS PubMed;
(c) H.-S. Lee, K.-M. Yoon, Y.-R. Han, K. J. Lee, S.-C. Chung, T.-I. Kim, S.-H. Lee, J. Shin and K.-B. Oh, Bioorg. Med. Chem. Lett., 2009, 19, 1051–PR215 CrossRef CAS PubMed;
(d) L.-h. Zhu, C. Chen, H. Wang, W.-c. Ye and G.-x. Zhou, Chem. Pharm. Bull., 2012, 60, 670–PR215 CrossRef CAS PubMed;
(e) R. Finlayson, A. N. Pearce, M. J. Page, M. Kaiser, M.-L. Bourguet-Kondracki, J. L. Harper, V. L. Webb and B. R. Copp, J. Nat. Prod., 2011, 74, 888–PR215 CrossRef CAS PubMed.
-
(a) J. L. Sessler, D.-G. Cho and V. Lynch, J. Am. Chem. Soc., 2006, 128, 16518–PR215 CrossRef CAS PubMed;
(b) T. Wang, Y. Bai, L. Ma and X.-P. Yan, Org. Biomol. Chem., 2008, 6, 1751–PR215 RSC;
(c) C. Bejger, J. S. Park, E. S. Silver and J. L. Sessler, Chem. Commun., 2010, 46, 7745–PR215 RSC.
-
(a) E. R. Pereira, M. Sancelme, A. Voldoire and M. Prudhomme, Bioorg. Med. Chem. Lett., 1997, 7, 2503–PR215 CrossRef CAS;
(b) M. Prudhomme, M. Sancelme, A. Bonnefoy, D. Fabbro and T. Meyer, Eur. J. Med. Chem., 1999, 34, 161–PR215 CrossRef CAS;
(c) Y.-J. Lee, Y.-R. Han, W. Park, S.-H. Nam, K.-B. Oh and H.-S. Lee, Bioorg. Med. Chem. Lett., 2010, 20, 6882–PR215 CrossRef CAS PubMed.
-
(a) J. McNulty, K. Keskar, C. Bordon, R. Yolken and L. Jones-Brando, Chem. Commun., 2014, 50, 8904–PR215 RSC;
(b) J. McNulty, K. Keskar, H. A. Jenkins, N. H. Werstiuk, C. Bordon, R. Yolken and L. Jones-Brando, Org. Biomol. Chem., 2015, 13, 10015–PR215 RSC.
-
(a) J. S. Sawyer, D. W. Beight, E. C. R. Smith, D. W. Snyder, M. K. Chastain, R. L. Tielking, L. W. Hartley and D. G. Carlson, J. Med. Chem., 2005, 48, 893–PR215 CrossRef CAS PubMed;
(b) S. Taliani, M. L. Trincavelli, B. Cosimelli, S. Laneri, E. Severi, E. Barresi, I. Pugliesi, S. Daniele, C. Giacomelli, G. Greco, E. Novellino, C. Martini and F. Da Settimo, Eur. J. Med. Chem., 2013, 69, 331–PR215 CrossRef CAS PubMed.
- H. E. Colley, M. Muthana, S. J. Danson, L. V. Jackson, M. L. Brett, J. Harrison, S. F. Coole, D. P. Mason, L. R. Jennings, M. Wong, V. Tulasi, D. Norman, P. M. Lockey, L. Williams, A. G. Dossetter, E. J. Griffen and M. J. Thompson, J. Med. Chem., 2015, 58, 9309–PR215 CrossRef CAS PubMed.
-
(a) M. J. Thompson, V. Borsenberger, J. C. Louth, K. E. Judd and B. Chen, J. Med. Chem., 2009, 52, 7503–PR215 CrossRef CAS PubMed;
(b) T. Wang, Z. Yin, Z. Zhang, J. A. Bender, Z. Yang, G. Johnson, Z. Yang, L. M. Zadjura, C. J. D'Arienzo, D. DiGiugno Parker, C. Gesenberg, G. A. Yamanaka, Y.-F. Gong, H.-T. Ho, H. Fang, N. Zhou, B. V. McAuliffe, B. J. Eggers, L. Fan, B. Nowicka-Sans, I. B. Dicker, Q. Gao, R. J. Colonno, P.-F. Lin, N. A. Meanwell and J. F. Kadow, J. Med. Chem., 2009, 52, 7778–PR215 CrossRef CAS PubMed.
-
(a) E. Merkul, J. Dohe, C. Gers, F. Rominger and T. J. J. Müller, Angew. Chem., Int. Ed., 2011, 50, 2966–PR215 CrossRef CAS PubMed;
(b) C. F. Gers, J. Nordmann, C. Kumru, W. Frank and T. J. J. Müller, J. Org. Chem., 2014, 79, 3296–PR215 CrossRef CAS PubMed.
-
(a) J.-C. Wu, R.-J. Song, Z.-Q. Wang, X.-C. Huang, Y.-X. Xie and J.-H. Li, Angew. Chem., Int. Ed., 2012, 51, 3453–PR215 CrossRef CAS PubMed;
(b) R.-Y. Tang, X.-K. Guo, J.-N. Xiang and J.-H. Li, J. Org. Chem., 2013, 78, 11163–PR215 CrossRef CAS PubMed.
-
(a) Q. Gao, J. Zhang, X. Wu, S. Liu and A. Wu, Org. Lett., 2015, 17, 134–PR215 CrossRef CAS PubMed;
(b) Z. Zhang, Z. Dai and X. Jiang, Asian J. Org. Chem., 2015, 4, 1370–PR215 CrossRef CAS.
-
(a) N. Mupparapu, N. Battini, S. Battula, S. Khan, R. A. Vishwakarma and Q. N. Ahmed, Chem.–Eur. J., 2015, 21, 2954–PR215 CrossRef CAS PubMed;
(b) J.-M. Yang, Z.-J. Cai, Q.-D. Wang, D. Fang and S.-J. Ji, Tetrahedron, 2015, 71, 7010–PR215 CrossRef CAS.
- The trimerization of indole took place and resulted in 2-(1H-indol-3-yl)-2,3′-biindolin-3-one, see reference: W.-B. Qin, Q. Chang, Y.-H. Bao, N. Wang, Z.-W. Chen and L.-X. Liu, Org. Biomol. Chem., 2012, 10, 8814–PR215 CAS.
-
(a) J. M. Bobbitt, J. Org. Chem., 1998, 63, 9367–PR215 CrossRef CAS;
(b) M. Schämann and H. J. Schäfer, Synlett, 2004, 2004, 1601–PR215 Search PubMed.
-
(a) D. H. Hunter, D. H. R. Barton and W. J. Motherwell, Tetrahedron Lett., 1984, 25, 603–PR215 CrossRef CAS;
(b) M. Hayashi, M. Shibuya and Y. Iwabuchi, Synlett, 2012, 23, 1025–PR215 CrossRef CAS.
- For the representative examples of Friedel-Crafts reactions of indole with active ketone, see:
(a) W. Zhuang, N. Gathergood, R. G. Hazell and K. A. Jørgensen, J. Org. Chem., 2001, 66, 1009–PR215 CrossRef CAS PubMed;
(b) B. Török, M. Abid, G. London, J. Esquibel, M. Török, S. C. Mhadgut, P. Yan and G. K. S. Prakash, Angew. Chem., Int. Ed., 2005, 44, 3086–PR215 CrossRef PubMed;
(c) H. Li, Y.-Q. Wang and L. Deng, Org. Lett., 2006, 8, 4063–PR215 CrossRef CAS PubMed;
(d) Y. Hui, W. Chen, W. Wang, J. Jiang, Y. Cai, L. Lin, X. Liu and X. Feng, Adv. Synth. Catal., 2010, 352, 3174–PR215 CrossRef CAS;
(e) G. Grach, A. Dinut, S. Marque, J. Marrot, R. Gil and D. Prim, Org. Biomol. Chem., 2011, 9, 497–PR215 RSC;
(f) C. Wolf and P. Zhang, Adv. Synth. Catal., 2011, 353, 760–PR215 CrossRef CAS;
(g) W. J. Moran, K. L. MacRory and A. Rodriguez, RSC Adv., 2012, 2, 8962–PR215 RSC;
(h) B. C. Loosley, R. J. Andersen and G. R. Dake, Org. Lett., 2013, 15, 1152–PR215 CrossRef CAS PubMed;
(i) D. Carmona, P. Lamata, A. Sánchez, P. Pardo, R. Rodríguez, P. Ramírez, F. J. Lahoz, P. García-Orduña and L. A. Oro, Organometallics, 2014, 33, 4016–PR215 CrossRef CAS;
(j) B. C. Loosley, R. J. Andersen and G. R. Dake, Org. Lett., 2013, 15, 1152–PR215 CrossRef CAS PubMed;
(k) D. Carmona, M. P. Lamata, A. Sanchez, F. Viguri, R. Rodriguez, L. A. Oro, C. Liu, S. Diez-Gonzalez and F. Maseras, Dalton Trans., 2014, 43, 11260–PR215 RSC.
- Cuprous chloride may promote the retro cyanohydrination of cyano tertiary alcohol C, see related references:
(a) A. K. Basak, N. Shimada, W. F. Bow, D. A. Vicic and M. A. Tius, J. Am. Chem. Soc., 2010, 132, 8266–PR215 CrossRef CAS PubMed;
(b) P. Nowak, D. Malwitz and D. C. Cole, Synth. Commun., 2010, 40, 2164–PR215 CrossRef CAS;
(c) N. Shimada, C. Stewart, W. F. Bow, A. Jolit, K. Wong, Z. Zhou and M. A. Tius, Angew. Chem., Int. Ed., 2012, 51, 5727–PR215 CrossRef CAS PubMed;
(d) Q. Wen, P. Lu and Y. Wang, RSC Adv., 2014, 4, 47806–PR215 RSC.
Footnote |
† Electronic supplementary information (ESI) available: Experimental details and characterization data of new compounds. See DOI: 10.1039/c6ra04016k |
|
This journal is © The Royal Society of Chemistry 2016 |