A baseline survey of potentially toxic elements in the soil of north-west Syria following a decade of conflict
Received
31st December 2022
, Accepted 14th April 2023
First published on 17th April 2023
Abstract
We present the first region-wide chemical survey of soils in NW Syria following more than a decade of ongoing conflict. We sampled the topsoil at 66 sites, typically located in marginal agricultural (orchards, arable) or peri-urban settings, grouped around 21 localities covering the whole area of NW Syria currently under Syrian Opposition control. Samples were analysed in the UK using inductively coupled plasma mass spectrometry (ICP-MS) and inductively coupled plasma optical emission spectrometry (ICP-OES). Topsoil total concentrations of potentially toxic elements (previously referred to as “heavy metals”) are broadly consistent with pre-war data from Aleppo and recent data from nearby Turkey. Principal Components Analysis (PCA) of associations among the sampling sites identified three groupings. Ni (133.30 ± 72.12 mg kg−1) and Cr (122.14 ± 52.25 mg kg−1) exist in all samples at levels in excess of typical European guideline thresholds for agricultural soil. Observed Cd (0.57 ± 0.93 mg kg−1), Co (23.07 ± 18.48 mg kg−1) and As (6.65 ± 4.51 mg kg−1) concentrations are up to three times comparable values from nearby agricultural regions in southern Turkey. Maximum observed values for Cd, As, and Co, which exceed EU thresholds, are concentrated in a corridor around Sarmada to the west of Aleppo which has seen some of the most intense conflict-related impacts. Cu (28.33 ± 17.11 mg kg−1), Pb (15.65 ± 10.85 mg kg−1) and Zn (73.64 ± 40.15 mg kg−1) also observe maxima in the Sarmada corridor, but show a more even distribution across the region, widely at values above comparable regional values for agriculture but below EU threshold concentrations. We interpret the occurrence of Ni–Cr as consistent with intensive agriculture using wastewater-contaminated irrigation and fertilisers. Cd–As–Co and Cu–Pb–Zn are likely anthropogenic and reflect intense pressures of conflict, informal settlement, unregulated industry and untreated wastewater irrigation on a historically agricultural region. The sampling method was designed to capture regional variations from a minimal dataset and it is likely that local topsoil concentrations at specific points of impact (proximal to locations of shelling, industry, effluent release or population) will be considerably higher than those reported here. This study establishes an important baseline reference for further targeted studies to identify and mitigate specific pollution hazards in this region of ongoing, extreme humanitarian and ecological threat.
Environmental significance
Armed conflicts have a significant impact on both urban and rural environments, yet remarkably little is known about the impact on soil geochemistry, and the long-term fate of conflict-related pollutants in the soil environment. This study presents the first baseline chemical survey of soils in north-west Syria following more than a decade of ongoing conflict. It is a crucial first step in monitoring the impacts of the Syrian conflict on the terrestrial environment and addressing environmental dimensions of the urgent humanitarian crisis in the region. Whilst this manuscript was in review the study area was impacted by a magnitude 7.8 earthquake on 6th February 2023. This event led to the further very significant displacement of local populations, creating an additional housing, agriculture, water and sanitation crisis and highlighting the significance to the region of understanding and protecting its soil environment.
|
Introduction
The Syrian civil war (2011–ongoing) is one of the most devastating humanitarian crises of modern times. The extensive use of weapons has affected large areas with explosive ordnance including landmines, improvised explosive devices, and other explosive remnants of war,1 and has led to infrastructure destruction and massive waves of internal displacement. In north-west Syria (NWS), more than 6.5 million people live in a confined region (approximately 7500 km2) and half of them are food-insecure2 (Fig. 1). More than 2 million people are living in 1760 informal settlements and camps with a lack of basic services such as sewage and waste management.3 The collapse of the economy and infrastructure has proliferated informal, unregulated industries such as petroleum refining and electroplating industries, tanneries, use of poor-quality agricultural fertilizers and the burning of leaded fossil fuels and coal.4 These indirect impacts of the devastating conflict exacerbate the direct environmental impacts of munitions, shelling, and military manoeuvres5 and are set against long-term regional drought, placing severe pressure on water resources and increasing the incidence of forest fires.6,7 In combination, these factors create an extraordinary level of threat to soil quality, human health and the agricultural economy, both as direct hazards and longer-term risks to socio-environmental recovery and restoration in a region where agriculture is historically the primary source of income and is now a principal factor in food security.8
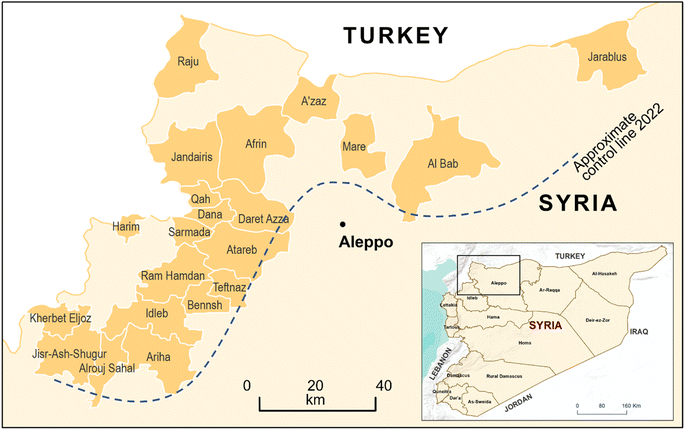 |
| Fig. 1 Regional map showing 21 sub-districts sampled in this survey and their locations in the opposition-controlled region of North-West Syria. The dashed line indicates schematically the contested limit of Syrian Government control at the time of this study, shown for illustration only. | |
The elements Pb, As, Hg, Cd, Co, Zn, Ba, Cu, Cr, Ni, Pd, Sn, V, and Pt are a group of metals and metalloids that have a relatively high density and are toxic even at parts per million (ppm) levels.9 These potentially toxic elements are natural components of the Earth's crust and are ubiquitous at low levels in terrestrial ecosystems. Heavy metal contamination refers to the excessive deposition of these toxic elements in the soil caused by anthropogenic activities. Potentially toxic elements are not only a source of soil pollution but also a threat to food security, water quality, and human health when they enter the food chain.10 Unlike organic pollutants, potentially toxic elements are non-biodegradable and accumulate in living tissues.11,12 They can cause several acute and chronic effects in humans and animals such as gastrointestinal and kidney dysfunction, nervous system disorders, skin lesions, vascular damage, immune system dysfunction, birth defects, and cancers.13–16 Exposure pathways leading to risks to human health from soils or food crops are complex and soil- and plant-specific, but many national and international governments have established guidelines for acceptable threshold soil concentrations which serve to highlight areas of concern if exceeded. In Europe, the EU LUCAS Topsoil Survey adopted Finnish Ministry of Environment threshold and guideline values in its assessment of agricultural soil contamination across the continent17 (Table 1).
Table 1 Summary data for regional and comparable soils, including summary headlines from this study. Ranges where quoted are 2 standard deviations about a mean
Location |
Reported total soil concentration (mg kg−1) |
Reference |
As |
Ba |
Cd |
Co |
Cr |
Cu |
Ni |
Pb |
Zn |
Regional, contemporary
|
North-West Syria, mean (n = 21, maximum in brackets) |
6.69 ± 4.47 (11.42) |
175.70 ± 133.74 (303.29) |
0.62 ± 1.17 (2.58) |
23.51 ± 21.28 (60.96) |
122.49 ± 52.63 (166.0) |
28.45 ± 17.28 (42.95) |
137.70 ± 80.64 (222.25) |
15.81 ± 11.57 (28.68) |
70.62 ± 37.81 (141.75) |
This study |
Harran Plain, Turkey |
6.36 ± 8.6 |
— |
— |
16 ± 6.8 |
85 ± 36 |
27 ± 11.2 |
89 ± 48 |
10.6 ± 4 |
68 ± 36 |
21
|
![[thin space (1/6-em)]](https://www.rsc.org/images/entities/char_2009.gif) |
Pre-conflict Syria
|
Gouta (intensive agriculture) |
— |
— |
— |
— |
57 |
34 |
39 |
17 |
103 |
18
|
Pre-conflict Syria (industrial) |
— |
— |
9.8 |
— |
— |
127 |
— |
95 |
760 |
19
|
Aleppo – GW irrigated |
— |
— |
1.78 |
— |
96 |
41 |
92 |
50 |
63 |
20
|
Aleppo – WW irrigated |
— |
— |
1.86 |
— |
112 |
46 |
94 |
72 |
74 |
20
|
Alsweida Governorate |
— |
— |
0.12 |
— |
2 |
— |
— |
0.12 |
4.37 |
52
|
![[thin space (1/6-em)]](https://www.rsc.org/images/entities/char_2009.gif) |
Global (non conflict)
|
EU (Finland soil threshold values) |
5 |
— |
1 |
20 |
100 |
100 |
50 |
60 |
200 |
17 and 44 |
Turkey |
— |
— |
0.3 |
— |
9.6 |
— |
— |
7.2 |
34.4 |
51
|
Spain |
— |
— |
0.14 |
— |
— |
28.1 |
— |
14.1 |
49.94 |
53
|
Lebanon |
— |
— |
0.28 |
— |
— |
28.6 |
— |
15.5 |
95.7 |
50
|
Bangkok |
— |
— |
— |
— |
26 |
42 |
25 |
48 |
118 |
18
|
Palermo |
— |
— |
— |
— |
— |
63 |
— |
202 |
138 |
18
|
European soils background (max quoted) |
— |
— |
— |
— |
56 |
24 |
39 |
63 |
100 |
18
|
Iran (specifically ‘unpolluted by conflict’) |
— |
— |
4.76 |
— |
— |
— |
25.78 |
— |
|
49
|
![[thin space (1/6-em)]](https://www.rsc.org/images/entities/char_2009.gif) |
Post-conflict
|
Iraq (oil field) |
— |
— |
— |
14.9 |
— |
124.8 |
131.2 |
16.8 |
154.7 |
54
|
Iran (‘polluted by conflict/armaments’) |
— |
— |
15.4 |
— |
— |
— |
132.4 |
— |
|
49
|
Croatia (low-intensity conflict area) |
7.97 |
59.68 |
0.21 |
5.66 |
20.36 |
16.15 |
15.03 |
10.95 |
55.77 |
30
|
Croatia (high-intensity conflict area) |
9.88 |
71.55 |
0.28 |
6.39 |
24.09 |
20.56 |
784.56 |
20.19 |
82.29 |
30
|
Several studies assessing potentially toxic element contamination in Syrian soils pre-dating the recent conflict exist (Table 1), although none of these present a broad regional survey. Möller et al.18 assessed the extent and severity of heavy metal contamination of arable soils in the Damascus Ghouta whilst Husein et al.19 measured the spatial distributions of Cu, Cd, Pb, Zn in the soil of the industrial Orontes floodplain around Hama, although the date of sampling is not stated. The concentrations of potentially toxic elements in cultivated soils around Aleppo following 25 years of untreated wastewater applications, in comparison to sites irrigated by groundwater, were presented by Sato et al.20 Studies from southern Turkey, immediately adjacent to the border with north-west Syria, form the basis of a regional baseline. In 2021 Varol et al.21 provided the most comprehensive recent survey of agricultural soils in the Harran Plain, 150 km to the north-east of our study region centroid (Table 1). Abaci Bayar and Yilmaz22 presented data from Amik Lake, Turkey (50 km west of our study area centroid), evidencing very high concentrations of Ni and Cr (in excess of 1500 mg kg−1) in wetland soils and sediments and concluding that these are geologically-derived from the serpentinite-bearing Kizildag Mesozoic Ophiolite Complex (e.g.,ref. 23).
Although there is a large body of research on potentially toxic element contamination in soils worldwide (e.g. ref. 24 and 25), relatively few studies have looked at the impact of conflict on soil pollution. The impact of the First World War on European soils was recently examined by Williams and Rintoul-Hynes26 who showed increased levels of Cu and Pb in bombed areas compared with the regional baseline. Azogh et al.27 showed ten-fold increase in levels of Pb and Cr in Iranian soils contaminated by weapons during the Iran–Iraq War compared to uncontaminated samples, a result similar to that observed in abandoned military training sites in Lithuania.28 Sultan measured the concentrations of metals within the soil in the city of Baghdad,29 confirming the impact on conflict-zone soils not just of munitions but of the range of uncontrolled industrial, waste disposal and domestic activities left unregulated during a conflict. Vidosavljević et al. examined the potential long-term impacts of war on soil quality in Croatia and found a positive association between areas of high conflict activity and elevated heavy metal concentrations in soils.30
Indirect impacts of conflict include the breakdown of physical and regulatory infrastructure for environmental protection, including sanitation and water treatment, waste management and industrial production. Untreated wastewater is a well-studied source of potentially toxic elements into receiving waters and transferred to agricultural soils via abstraction for irrigation.31–34 In north-west Syria, a report on sewage irrigation in the Idlib region found that dozens of farmers in the Al Roj plain area irrigate their summer crops with sewage, despite their knowledge of the health and environmental risks.35 Lack of maintenance of sewage networks in Idlib and the western and southern countryside of Aleppo results in uncontrolled leakage and storm-induced discharge into nearby valleys and swamps, with winter flooding of these areas spreading contaminated waters to roads, settlements, and agricultural land.36 Makeshift oil refineries have become an integral part of the north-west Syria wartime economy.37 Although many refineries have been forced out of service in recent years by Russian and regime airstrikes, dozens are still concentrated in Tarhin, on the outskirts of Al Bab, where a quarter of the refineries – around 100 burners – are still active.38 Cocârţă et al. (2017) suggested that oil contamination of the soil could be a significant contributor to human environmental health risks associated with these activities.39
Addressing soil quality and associated risks to human health in north-west Syria should form part of urgent humanitarian relief work in the short term and economic and environmental restoration efforts in the long term. Critical to the planning, implementation, and success of such work is a robust understanding of the scale, extent, and sources of soil contamination. Integral to this understanding is the availability of baseline data against which specific observations can be referenced. Here, we address this important need by reporting the first coordinated survey of topsoil concentrations of potentially toxic elements across north-west Syria following more than a decade of conflict.
Methodology
Situational challenges to data collection
The samples were obtained during a period of relative quiet in the skirmishes on the fronts and the aerial bombardment (01.07.2021–09.08.2021). The study design was led by the authors who are Syrian academics with intimate knowledge of the region and conducted according to a detailed risk assessment. The sampling team comprised researchers and students from the Agricultural Engineering Department at Sham University, Syria who belong to well-known families in every location and are familiar with the roads. Numerous security checkpoints and the sensitivity of some local authorities to sampling operations required careful negotiation to permit access to sample sites. Prioritizing the reduction of potential risks of unexploded ordnance limited the quantity of material collected, time spent on site and the depth of sampling.
Geological setting and soil characteristics
Baseline soil geochemistry and mineralogy is fundamentally controlled by the bedrock and superficial geology upon which the soil profile develops along with any subsequent anthropogenic inputs. Regional geological mapping at a scale of 1
:
200
000 ref. 40 shows that the area sampled in this study is predominantly underlain by Mesozoic and Cenozoic sedimentary units, dominated by carbonates (limestones and marls) and less common siliciclastic sediments (sandstones and mudstones). Fault bounded units assigned to the Mesozoic Ophiolite Series occur in the northern part of the study area, and may include radiolarites and basic (basaltic) lavas and tuffs. Bedrock units are overlain by Quaternary alluvial sediments.
Sample collection and processing
Soil samples were collected from 21 districts selected to cover most areas of north-west Syria (Fig. 1). Samples were collected from three locations in each district and these three samples were then mixed by hand to obtain a homogenised soil representative of each district. Sampling locations were typically at least 1 km apart. Sample site selection was constrained by local access and safety concerns as noted above. Most sites were located on the margins of agricultural land (olive orchards or arable fields) and/or peripheral to formal or informal settlements (Fig. 2). Samples were obtained using a hand trowel from a depth of 10–20 cm below the surface and placed in double sealed polyethylene bags. The sampling team wore disposable nitrile gloves for personal protection and to ensure the integrity of the sample (to avoid cross-contamination between samples). Sampling tools were cleaned with bottled drinking water and disposable towelettes between samples and between sample sites to avoid cross-contamination following standard practice.41 Following sampling, photographs of the sample site and the location context were taken and referenced to a written note of the site soil conditions, weather, and other relevant observations (e.g. activities taking place at the site). The soil sample bags were sealed by hand and taken initially to Harran University, Turkey for processing. Soil chemical properties including electrical conductivity (EC), pH and organic matter (OM) content were determined in soil samples using standard laboratory procedures.29 EC in each soil sample was determined using standard methods.42,43
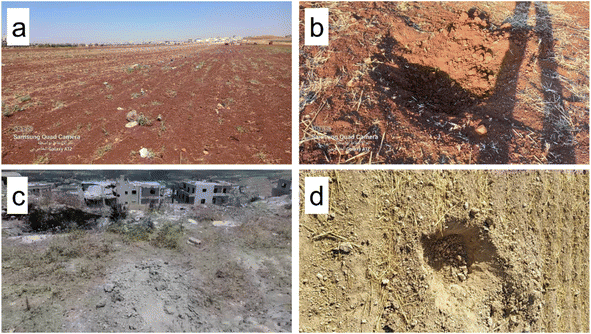 |
| Fig. 2 Examples of typical sampling sites. (a) Sarmada_3 (36°12′06.3′′N 36°44′13.6′′E). (b) Dartazze _2 (36°18′26.7′′N 36°50′31.5′′E). (c) Ariha_1, Missile impact site (35°48′17.4′′N 36°36′48.5′′E). (d) Jarabulus_2 (36°42′51.0′′N 38°00′55.6′′E). | |
Elemental analysis
Samples were transferred to The James Hutton Institute and the University of South Wales, UK for chemical analysis. The soil samples were prepared and analysed as part of the UKAS accredited ISO17025:2017 method at the James Hutton Institute; full QC data are available on request from the corresponding author. Samples were digested in a mixture of hydrochloric and nitric acid (aqua regia) under reflux, for two hours. The digest was allowed to cool, filtered and made up to a known volume with 0.5 M HNO3 to a final matrix of 21% HCl and 9% HNO3. The digest was analysed by Inductively Coupled Plasma-Optical Emission Spectroscopy (ICP-OES) and Inductively Coupled Plasma-Mass Spectrometry (ICP-MS) to determine the aqua regia soluble element concentration. The results were verified in accordance with the relevant SAM and SOP XP002. Two samples were randomly chosen and resampled to assess in-sample variance. A principal component analysis (XLSTAT http://www.xlstat.com/, Spearman correlation, no rotation) of element concentrations was performed to allow the differentiation of sample groups with common patterns of association between elements.
Enhancement ratios
Enhancement ratios were calculated for each element at each site as the observed concentration divided by the values indicated by the Finnish Ministry of Environment as threshold soil concentrations which identify a site as requiring further local assessment44 (Table 1). These values were adopted as representative and transferable by the EU LUCAS Topsoil Survey in its assessment of potentially toxic elements in agricultural soils17 and by the UNEP.44 To highlight locations of concern for contamination across a range of elements, enhancement ratios were averaged across As, Co, Cd, Cr, Cu, Ni, Pb, Zn to give a Combined Enhancement Index (CEI) after ref. 21.
Results
Table 1 and Fig. 3 show summary statistics for potentially toxic element concentrations in this study, in comparison with international guideline values and relevant previous studies. Table 2 shows pH, EC and organic matter. Table 3 presents the observed concentration of each element in each sub-district together with the calculated CEI.
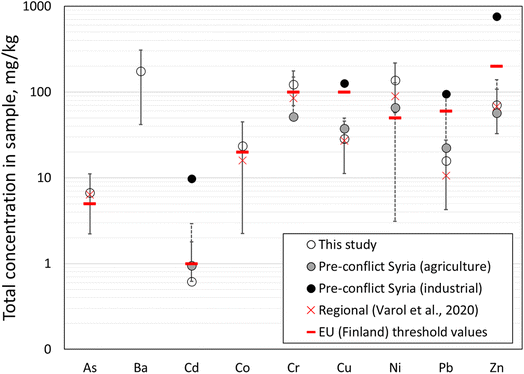 |
| Fig. 3 Mean aqua regia soluble soil concentrations of nine potentially toxic elements in topsoils from 66 locations in NW Syria (open circles). Also shown are data collated from previous studies in pre-conflict Syria in agricultural (grey circles) and urban/industrial regions (solid circles). Error bars are two standard deviations. Red bars indicate the threshold values for these elements in soils derived from values published by the European Union.17 Dashed lines are error bars for data reported by ref. 21. | |
Table 2 List of sub-districts with site characteristics recorded at sampling points and measured topsoil EC (mS m−1) and pH. Sampling site character(s) as recorded by field teams during sampling (three sites per sub-district): RES (residential), IND (industrial), AGR (agricultural), CAA (conflict-affected area), VTA (vehicle transport area), CMP (camp)
Sub-district |
Sampling site character(s) |
Conductivity, mS m−1 |
pH |
Afrin |
RES |
139.80 |
8.10 |
Al Bab |
IND/RES |
147.53 |
8.22 |
Alruj Plain |
AGR |
208.90 |
7.78 |
Ariha |
CAA/VTA/RES |
103.67 |
8.39 |
Atareb |
CAA/RES |
238.07 |
8.16 |
A'zaz |
VTA/RES |
204.40 |
8.14 |
Bennish |
CAA/AGR |
154.67 |
8.44 |
Dana |
VTA/RES/IND |
223.93 |
7.97 |
Daret Azza |
RES |
148.13 |
7.90 |
Harim |
RES |
458.00 |
8.00 |
Idlib |
VTA/RES |
213.00 |
8.33 |
Jandairis |
RES |
233.07 |
8.36 |
Jarablus |
IND |
123.90 |
8.34 |
Jisr-Ash Shugur |
VTA/RES |
853.33 |
7.96 |
Kherbet Eljoz |
CAA |
1165.00 |
8.09 |
Mare |
AGR |
152.73 |
8.36 |
Qah |
CMP |
129.97 |
7.97 |
Raju |
AGR |
156.00 |
8.30 |
Ram Hamdan |
CAA/AGR |
129.90 |
7.93 |
Sarmada |
VTA/RES |
182.03 |
8.26 |
Teftnaz |
CAA/AGR |
225.93 |
8.15 |
Table 3 Total topsoil concentrations (mg kg−1) for 9 potentially toxic elements, and Combined Enhancement Index (CEI) against thresholds specified in Finnish legislation adopted by ref. 17 and 44a
Sub-district |
Aqua regia soluble topsoil concentration, mg kg−1 |
CEI |
As |
Ba |
Cd |
Co |
Cr |
Cu |
Hg |
Mo |
Ni |
Pb |
Pt |
Se |
Zn |
Values in bold are the maximum observed within this sample set. Italic text indicates values exceeding threshold values (shown in Table 1). Where shown, errors are standard error on replicate analyses performed as part of QC/QA.
|
Afrin |
6.45
|
177.5 |
0.35 |
25.69
|
162.0
|
33.78 |
<0.09 |
0.99 |
187.7
|
19.62 |
<0.02 |
<0.10 |
78.96 |
1.17 |
Al Bab |
6.74
|
303.9
|
0.39 |
24.10
|
101.2
|
29.63 |
<0.09 |
0.92 |
119.8
|
16.31 |
<0.02 |
3.51 |
81.64 |
0.92 |
Alruj Plain |
8.83
|
188.2 |
0.65 |
33.31
|
146.7
|
30.28 |
<0.09 |
0.86 |
145.2
|
22.47 |
<0.02 |
0.43 |
75.12 |
1.19 |
Ariha |
3.38 |
76.3 |
0.52 |
9.20 |
109.3
|
15.65 |
<0.09 |
0.87 |
54.5
|
9.23 |
<0.02 |
0.48 |
49.16 |
0.55 |
Atareb |
7.87
|
244.9 |
0.57 |
23.12
|
121.9
|
37.98 |
<0.09 |
0.87 |
130.7
|
16.58 |
<0.02 |
4.87
|
74.58 |
1.02 |
A'zaz |
6.40
|
197.2 |
0.31 |
24.81
|
153.9
|
39.50 |
<0.09 |
0.90 |
183.0
|
16.30 |
<0.02 |
<0.10 |
88.51 |
1.14 |
Bennish |
6.69
|
156.2 |
0.37 |
18.37 |
102.5
|
23.66 |
<0.09 |
1.08 |
98.8
|
14.39 |
<0.02 |
<0.10 |
62.87 |
0.80 |
Dana |
9.08
|
225.2 |
0.68 |
26.53
|
132.5
|
32.78 |
<0.09 |
<0.30 |
142.9
|
20.04 |
<0.02 |
3.37 |
81.52 |
1.13 |
Daret Azze |
11.42
|
194.4 |
1.86
|
33.92
|
166.0
|
42.95
|
<0.09 |
0.93 |
168.9
|
22.20 |
<0.02 |
0.98 |
117.50 |
1.53 |
Harim |
9.34
|
124.2 |
1.27
|
21.96
|
147.3
|
28.43 |
<0.09 |
1.46 |
127.3
|
19.62 |
<0.02 |
<0.10 |
85.62 |
1.16 |
Idlib |
4.70 |
96.1 |
0.26 |
11.76 |
71.0 |
20.66 |
0.40 |
0.87 |
67.2
|
11.80 |
<0.02 |
<0.10 |
48.95 |
0.56 |
Jandairis |
7.05
|
208.3 |
0.32 |
20.04
|
108.5
|
29.96 |
<0.09 |
<0.30 |
128.1
|
17.99 |
<0.02 |
<0.10 |
85.29 |
0.93 |
Jarablus |
4.73 |
151.9 |
0.27 |
19.97 |
107.0
|
22.94 |
<0.09 |
<0.30 |
137.3
|
8.58 |
<0.02 |
<0.10 |
56.43 |
0.84 |
Jisr-Ash Shugur |
3.71 |
136.5 |
0.42 |
21.89
|
123.8
|
20.26 |
<0.09 |
1.71
|
157.8
|
5.56 |
<0.02 |
<0.10 |
61.02 |
0.91 |
Kherbet Eljoz |
4.60 |
61.3 |
0.35 |
11.90 |
141.5
|
15.95 |
0.45
|
1.39 |
177.5
|
6.79 |
<0.02 |
<0.10 |
44.07 |
0.92 |
Mare |
3.40 |
111.5 |
0.32 |
18.86 |
102.7
|
21.69 |
<0.09 |
<0.30 |
174.3
|
21.21 |
<0.02 |
0.50 |
72.63 |
0.92 |
Qah |
5.10
|
116.3 |
0.38 |
14.36 |
87.2 |
17.99 |
<0.09 |
0.82 |
95.9
|
11.16 |
0.02
|
<0.10 |
49.30 |
0.69 |
Raju |
6.81
|
156.1 |
0.37 |
24.92
|
116.4
|
41.52 |
<0.09 |
1.18 |
129.2
|
16.21 |
<0.02 |
2.46 |
99.07 |
0.99 |
Ram Hamdan |
7.29
|
245.2 |
0.37 |
24.59
|
107.3
|
24.80 |
<0.09 |
<0.30 |
113.4
|
13.87 |
<0.02 |
0.41 |
59.59 |
0.90 |
Sarmada |
10.29 ± 0.09 |
294.10 ± 59.96 |
2.58 ± 1.73 |
60.96 ± 11.79 |
159.70 ± 6.36 |
41.40 ± 2.08 |
<0.09 |
0.95 |
222.25 ± 99.49 |
28.68 ± 4.96 |
<0.02 |
<0.10 |
141.75 ± 45.18 |
1.86
|
Teftnaz |
6.57 ± 1.26 |
224.55 ± 7.85 |
0.37 ± 0.1 |
23.55 ± 1.28 |
103.90 ± 3.96 |
25.66 ± 1.41 |
0.33 |
<0.30 |
129.85 ± 31.32 |
13.26 ± 0.16 |
<0.02 |
<0.10 |
72.74 ± 11.17 |
0.91 |
Fig. 2 shows photographs of four typical sampling locations. Table 2 provides a qualitative indication of the dominant site characteristics in each sub-district. Sites were typically located on agricultural land of low slope between 50 m and 250 m distant from roads, residential units or other human activities. Field descriptions of topsoil recorded by sampling teams varied from brown to light-brown (Munsell) with less than 10% gravel on cultivated sites, to grey with more than 30% gravel on some uncultivated sites. Salinity is generally low (EC < 400 mS m−1, Table 2) and mean pH 8.15 ± 0.19. Rainfall in the region is typically 300–600 mm y−1, falling mostly as intense rain or snowfall in winter months.
Ni (mean 133.30 ± two standard deviations 72.12 mg kg−1) and Cr (122.14 ± 52.25 mg kg−1) exist in all samples at levels in excess of typical European guideline thresholds for agricultural soil. Observed Co (23.07 ± 18.48 mg kg−1), and As (6.65 ± 4.51 mg kg−1) are typically above EU thresholds and up to three times comparable values from southern Turkey.21 Maximum observed values for As and Cd are observed in Sarmada (As 10.35 mg kg−1, Cd 1.73 mg kg−1), Daret Azze (As 11.42 mg kg−1, Cd 1.86 mg kg−1) and Harim (As 9.34 mg kg−1, Cd 1.27 mg kg−1) districts. Cu (28.33 ± 17.11 mg kg−1), Pb (15.65 ± 10.85 mg kg−1) and Zn (73.64 ± 40.15 mg kg−1) also observe maxima in Daret Azze widely at values above comparable regional values for agricultural soils (Tables 1 and 3).
PCA analysis of co-variance in metal concentrations across the 21 districts sampled indicated two principal factors accounting together for 75% of cumulative variability within the dataset. In terms of these two principal factors, the elements clustered in distinct groups. The first group includes Cr and Ni, while the second group includes Cu, Pb, and Zn, elements which are commonly found to be anthropogenically enriched in the topsoil of urban environments (e.g. ref. 18). Intermediate between these two groups are Cd, Co and As. Fig. 4 shows the spatial distributions of heavy metal concentrations across north-west Syria. Clear differences are evident between the distributions of groups Ni–Cr (Fig. 4a and b), Cd–Co–As (Fig. 4c and d; Co not shown), and Cu–Pb–Zn (Fig. 4e and f; Cu not shown). In particular, Ni and Cr are distributed across the region at generally high concentrations with a relatively few sites of significantly lower concentration; Cd and As have overall lower concentrations across the region with a few sites, spatially-clustered around Sarmada and Daret Azza, which have much higher concentrations.
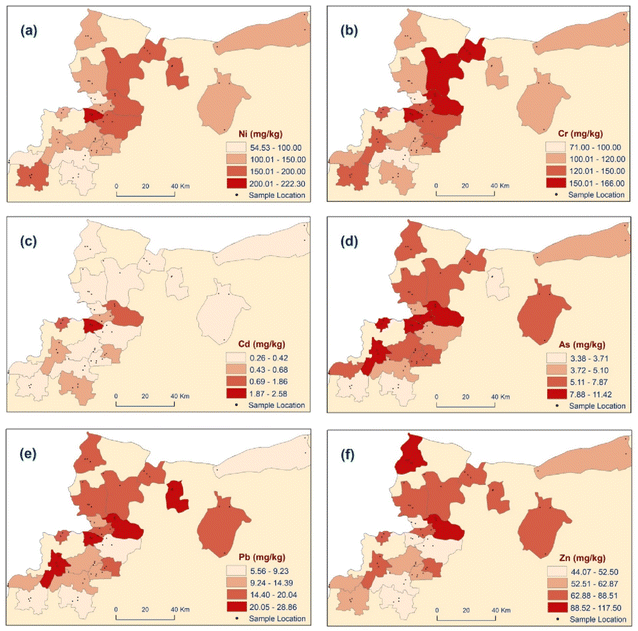 |
| Fig. 4 Chloropleth maps showing observed concentrations of six potentially toxic elements at sub-district level across north-west Syria. PCA analysis indicates co-variance in (a and b) Ni and Cr, (c and d) Cd, As, Co (Co not shown), (e and f) Pb, Zn, Cu (Cu not shown). | |
Fig. 5a shows enhancement ratios and Combined Enhancement Index (CEI), highlighting the areas of particular concern due to high contaminant levels in the region around Sarmada and Daret Azze. At Sarmada, Cd concentration is 2.58 times the EU (Finnish) threshold value (1 mg kg−1) and Co is more than 3 times the threshold value (20 mg kg−1). CEI exceeds 1.0 in eight of 21 sub-districts, including Daret Azze (1.53) and Sarmada (1.86). These regions lie on the main east-west road from Aleppo to the Turkish border at Bab Al-Hawa and have some of the highest regional concentrations of internally displaced people in need (PIN, Fig. 5b, data from ref. 3). A further 10 sub-districts present a CEI in excess of 0.8, indicating significant cause for concern across a range of contaminants.21
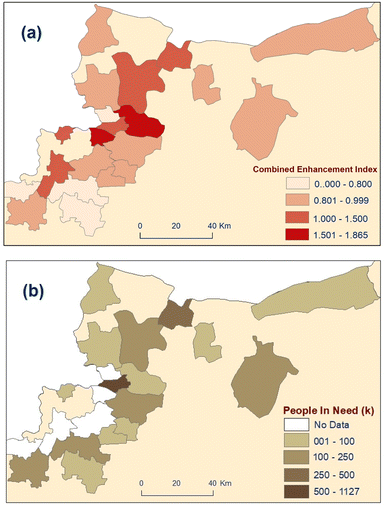 |
| Fig. 5 Chloropleth maps showing (a) combined contamination index, and (b) population in need (PIN, thousands, data from ref. 3) at sub-district level across north-west Syria. | |
Discussion
This is the first widespread and coordinated survey of potentially toxic elements in samples from north-west Syria to be published since the start of the conflict in 2011. The scarcity of published observations covering the NWS region from before this time makes this an important dataset, but frustrates any attempt to systematically compare pre- and post-conflict conditions. The observations across all elements tested are broadly consistent with available pre-war data for agricultural and urban/industrial soils in Syria and recent datasets from neighbouring regions in southern Turkey (Table 1). The sampling strategy by necessity combined samples from three sites distributed across each of the 21 sub-districts to achieve regional coverage. This averaging likely makes the reported values a conservative baseline; it is possible that local topsoil concentrations at specific locations may be considerably higher than those reported here.
As, Co, Ni and Cr exist at levels in excess of international threshold values for soil quality at almost every location across the region (Table 3). Concentrations everywhere are consistent with, but typically higher than, pre-war data on soils irrigated with wastewater in Aleppo20 (Table 1). Raised Ni and Cr concentrations are also observed in non-conflict agricultural soils in proximal areas of Turkey21 and may be geologically influenced across the region by the proximity of serpentinite-bearing ultramafic bedrock to the west and north-west. However, the bedrock directly underlying the region belongs to the Tertiary Arabian Plateau,45 comprising largely carbonate sedimentary sequences which are not expected to have naturally-elevated Ni or Cr concentrations. The concentrations of Ni are close to those observed in post-conflict soils in Iraq and Iran (Table 1) and in high-intensity conflict areas in Croatia.30
Of particular note in north-west Syria is the observation of elevated Cr and Ni (among other elements) in a controlled pre-conflict study of wastewater-irrigated fields near Aleppo,20 and in other studies of wastewater-irrigated soils globally. The collapse of sanitation infrastructure in north-west Syria since 2011 has led to widespread and continuous release of untreated wastewater into terrestrial waters which are then used for irrigation. The likelihood of accumulation of potentially toxic elements due to relatively immobility in high-pH soils (c. pH 8, Table 2) raises the possibility that progressive contamination from prolonged exposure to untreated wastewaters and low-quality phosphate fertilisers (e.g., ref. 46 and 47) applied in agriculture widely throughout the region post-2011 is exacerbating potentially high regional baseline concentrations of soil Ni and Cr and accumulating As, Co and other elements. Regardless of the source, the observed values for As, Co, Ni and Cr across the region are in excess of acceptable thresholds for agricultural soils and should be subject to further local assessment to identify potential risks to food chains.17
Cd across most of the area occurs at levels close to global averages but is elevated above EU threshold values at locations in the region west of Aleppo (Sarmada, Daret Azza, Harim). This spatial distribution is broadly shared with As and Co, which are widely above EU threshold values as noted above. We interpret the occurrence of Cd and associated As, Co as most likely to be anthropogenic arising from both agricultural and industrial/conflict activities and our data are likely conservative estimates of local concentrations at specific sites impacted by point source pollution. The spatial clustering of locations with elevated concentrations of these elements in the region west of Aleppo is notable considering the intensity of direct military activity, internally displaced people and people in need, transport, forest fires, and unregulated industrial activities in this area over the last decade of conflict. The widespread exceedance of thresholds for As and Co, and the high CEI values for locations in the Sarmada ‘corridor’, demand further local assessment to identify direct and indirect risks to human health.
Conclusion and recommendations
This dataset provides a consistent, standardized soil baseline for potentially-toxic elements covering most of north-west Syria. To our knowledge, this is the first published and accessible dataset for the region following a decade of conflict, and indeed since well before 2011. We intend that it will provide an important reference for the urgent future work necessary to assess and remediate specific direct and indirect conflict impacts on soils. The data are consistent with expected trends for intensively cultivated, human-impacted and post-conflict soils regionally and globally, and highlight some specific areas of concern.
Risks to health
Widespread contamination of water resources in north-west Syria with untreated wastewater is likely to be contributing to the accumulation of potentially toxic elements in agricultural topsoils, leading to potential human health risks via soil-to-plant transfers or dust inhalation, in addition to other impacts via pathogens such as cholera.3 The spatial clustering of elevated concentrations for all observed elements, particularly Cd in the corridor to the west of Aleppo and As, Co, Ni and Cr throughout the region in our conservative sampling is a cause for concern in respect of the potential for locally much-higher concentrations associated with specific exposure pathways or polluting activities. The generally alkaline pH of soils in the region mitigates somewhat against dispersion of soil contaminants from point sources into the wider aquatic environment (surface and groundwaters) but presents the risk of higher local accumulations leading to risks from direct exposure, soil-to-plant transfers, and dust inhalation. Of particular concern is the fact that most of the region is historically agricultural and subject to intense demand for food and land in the midst of the ongoing conflict (e.g., ref. 48), factors which place extreme pressure on marginal and potentially contaminated sites to be turned over to food production or dwellings where soil exposure risks are highest.
Mitigation and remediation priorities
We make three recommendations for short-term mitigation of risks from soil contamination in north-west Syria. Firstly, NGOs working with farmers should prioritise a systematic regional audit of the use of wastewater for irrigation, coupled with monitoring and publication of soil and water quality data for wells and rivers used as water sources for irrigation, even where these are not considered to be directly impacted by wastewaters. Secondly, a focused independent programme of soil sampling and analysis in the region around Sarmada and Daret Azze should be carried out, targeting both potential source and receptor locations to assess specific risks for potentially toxic elements, particularly Cd, Co and As in soils. Thirdly, we recommend exposure pathway studies to assess heavy metal concentrations and/or soil-to-plant uptake in key crop plants in the region, and in airborne dust or smoke deposits generated from soils and fires.
Strategic regional investment in agricultural and environmental engineering, looking to longer-term post-conflict environmental restoration, should be focused on three essential challenges: (i) a better understanding of specific risks to human health from soil exposures under north-west Syrian conditions. Of importance to this effort is the development and coordination of a consistent sampling and analytical protocol across organisations and projects, to enable the establishment of a regional database against which individual measurements can be interpreted. We view the EU LUCAS Topsoil Survey (e.g. ref. 17) as an important reference methodology in this regard and encourage the international academic community to support this through access to certificated analytical facilities for soil analysis; (ii) research to establish the potential for remediation of contaminated soils in north-west Syria using sustainable, low-cost methods such as biochar and phytoremediation; and (iii) coordinated action at the system level to develop climate- and contaminant-resistant crop varieties together with micro-irrigation, solar distillation and nature-based solutions for water quality management to reduce demand for contaminated water from conflict-impacted sources and underpin the eventual environmental recovery of the region.
Author contributions
Miassar Alhasan led the project team in the design and conduct of the research. Abdulkarim Lakmes led the sample processing at Harran University. Mohammad Gazy Alobaidy, Safwan AlHaeek and Muhammed Assaf were integral to the design and conduct of the sampling in the field. All members of the Syria team were involved in data analysis and manuscript drafting. Lorna Dawson and Duncan Pirrie conducted the elemental analyses in the UK, data analysis and manuscript revision and editing. Ziad Abdeldayem and Jonathan Bridge worked through the Cara Syria Programme to provide academic mentorship to the Syria team during research design and bid-writing, contributed to the analysis and interpretation of data, the production of figures and tables, and the revision and editing of the final manuscript.
Conflicts of interest
The authors declare no potential conflicts of interest with respect to the research, authorship, and/or publication of this article.
Acknowledgements
This research was supported by Cara (the Council for At-Risk Academics, http://www.cara.ngo/), a UK charity, via a grant made through its Syria Programme. The authors would like to thank Cara staff led by Ms. Kate Robertson for their support and feedback. Special thanks to the many volunteer field researchers who carried out the sampling and recording in north-west Syria. Ethics approval was granted within the Sheffield Hallam University Research Ethics framework. Many of the authors of this article and their families have been significantly impacted by the February 6th 2023 earthquake and related after-shocks; the UK authors acknowledge their continued commitment to this work given current circumstances. For the purpose of open access, the authors have applied a Creative Commons Attribution (CC BY) licence to any Author Accepted Manuscript version arising from this submission. We thank the editor and two reviewers for critical comments which led to a substantial improvement to the manuscript.
References
-
The Carter Center, A Call for Action: Data on Unexploded Ordnance in Syria and Its Implications, December 2012 – May 2021, March 2022, p. 29, https://www.cartercenter.org/resources/pdfs/peace/conflict_resolution/syria-conflict/2022/uxo-rpt5-a-call-for-action-data-on-unexploded-ordnance-in-syria-and-its-implications.pdf Search PubMed.
-
Food Security Cluster, Food and Agricultural Organization – World Food Programme, Gaziantep, 2022, https://fscluster.org/gaziantep, accessed 19/12/22 Search PubMed.
-
UNOCHA, Humanitarian Needs Overview: Syrian Arab Republic, UN Office for the Coordination of Humanitarian Affairs OCHA, 2022, p. 108, https://reliefweb.int/report/syrian-arab-republic/2022-humanitarian-needs-overview-syrian-arab-republic-february-2022, accessed 19/12/22 Search PubMed.
-
UN-Habitat, Recovery of Services and Infrastructure in Syria. “Not If, But How?”, Urban Recovery Framework Thematic Paper, July 2022, p. 92https://unhabitat.org/sites/default/files/2022/09/infrastructure.pdf, Accessed 19/12/22 Search PubMed.
- V. Zwijnenburg, D. Hochhauser, O. Dewachi, R. Sullivan and V. K. Nguyen, Solving the jigsaw of conflict-related environmental damage: Utilizing open-source analysis to improve research into environmental health risks, J. Public Health, 2020, 42(3), e352–e360, DOI:10.1093/pubmed/fdz107.
- R. Homsi, M. S. Shiru, S. Shahid, T. Ismail, S. B. Harun, N. Al-Ansari, K.-W. Chau and Z. M. Yaseen, Precipitation projection using a CMIP5 GCM ensemble model: A regional investigation of Syria, Eng. Appl. Comput. Fluid Mech., 2020, 14, 90–106, DOI:10.1080/19942060.2019.1683076.
- H. G. Abdo, H. Almohamad, A. A. Al Dughairi and M. Al-Mutiry, GIS-based frequency ratio and analytic hierarchy process for forest fire susceptibility mapping in the Western Region of Syria, Sustainability, 2022, 14(8), 4668, DOI:10.3390/su14084668.
-
UNOCHA, North-West Syria Situation Report, 2022, https://reports.unocha.org/en/country/syria/, accessed 18/12/2022 Search PubMed.
- R. A. Wuana and F. E. Okieimen, Heavy metals in contaminated soils: a review of sources, chemistry, risks and best available strategies for remediation, Int. Scholarly Res. Not., 2011, 20, DOI:10.5402/2011/402647.
-
FAO & ITPS, Status of the World's Soil Resources (SWSR): Main Report, Food and Agriculture Organization of the United Nations and Intergovernmental Technical Panel on Soils, FAO, Rome, Italy, 2015, p. 650, https://www.fao.org/documents/card/en/c/c6814873-efc3-41db-b7d3-2081a10ede50/, accessed 18.02.2022 Search PubMed.
- R. Singh, N. K. Ahirwar, J. Tiwari and J. Pathak, Review on sources and effect of heavy metal in soil: its bioremediation, Int. J. Res. Appl. Nat. Soc. Sci., 2018, 1–22 Search PubMed.
-
M. Yadav, R. Gupta and R. K. Sharma, Green and sustainable pathways for wastewater purification, ed. S. Ahuja, in Advances in water purification techniques: meeting the needs of developed and developing countries, Elsevier, Amsterdam, 2019, 355–383, DOI:10.1016/B978-0-12-814790-0.00014-4.
- B. Fernandes Azevedo, L. Barros Furieri, F. M. Peçanha, G. A. Wiggers, P. Frizera Vassallo, M. Ronacher Simões, J. Fiorim, P. Rossi de Batista, M. Fioresi, L. Rossoni, I. Stefanon, M. J. Alonso, M. Salaices and D. Valentim Vassallo, Toxic effects of mercury on the cardiovascular and central nervous systems, J. Biomed. Biotechnol., 2012, 949048, DOI:10.1155/2012/949048.
- S. J. Cobbina, Y. Chen, Z. Zhou, X. Wu, T. Zhao, Z. Zhang, W. Feng, W. Wang, Q. Li, X. Wu and L. Yang, Toxicity assessment due to sub-chronic exposure to individual and mixtures of four toxic heavy metals, J. Hazard. Mater., 2015, 30(294), 109–120, DOI:10.1016/j.jhazmat.2015.03.057.
- M. Costa, Review of arsenic toxicity, speciation and polyadenylation of canonical histones, Toxicol. Appl. Pharmacol., 2019, 375(15), 1–4, DOI:10.1016/j.taap.2019.05.006.
- H. S. S. Gazwi, E. E. Yassien and H. M. Hassan, Mitigation of lead neurotoxicity by the ethanolic extract of Laurus leaf in rats, Ecotoxicol. Environ. Saf., 2020, 192, 110297, DOI:10.1016/j.ecoenv.2020.110297.
- G. Tóth, T. Hermann, M. R. da Silva and L. Montanarella, Heavy metals in agricultural soils of the European Union with implications for food safety, Environ. Int., 2016, 88, 299–309, DOI:10.1016/j.envint.2015.12.017.
- A. Möller, H. W. Müller, A. Abdullah, G. Abdelgawad and J. Utermann, Urban soil pollution in Damascus, Syria: concentrations and patterns of heavy metals in the soils of the Damascus Ghouta, Geoderma, 2005, 124(1–2), 63–71, DOI:10.1016/j.geoderma.2004.04.003.
- H. Husein, M. Kalkha, A. Jrdi and R. Bäumler, Urban soil pollution with heavy metals in Hama floodplain, Syria, Nat. Resour., 2019, 10, 187–201, DOI:10.4236/nr.2019.106013.
- T. Sato, S. Yamamoto, M. Qadir, T. Endo, T. Masanaga and Z. Ahmed, Long-term effects of wastewater irrigation on soil heavy metal contamination in peri-urban areas of Aleppo, Syria, Int. J. Agric. Biol., 2014, 16, 1153–1158 CAS.
- M. Varol, M. R. Sünbül, H. Aytop and C. H. Yılmaz, Environmental, ecological and health risks of trace elements, and their sources in soils of Harran Plain, Turkey, Chemosphere, 2020, 245, 125592, DOI:10.1016/j.chemosphere.2019.125592.
- A. A. Abaci Bayar and K. Yilmaz, Assessment of some heavy metal pollution in the wetland soils of Adıyaman, Kahramanmaraş and Hatay, BSJ Agri., 2020, 3(2), 128–134 Search PubMed.
- Y. Dilek and M. Delaloye, Structure of the Kizildag ophiolite, a slow-spread Cretaceous ridge segment north of the Arabian promontory, Geology, 1992, 20(1), 19–22, DOI:10.1130/0091-7613(1992)020<0019:SOTKOA>2.3.CO;2.
- G. N. Salomāo, A. Figueiredo, R. Dall'Agnol, P. K. Sahoo, P. W. M. S. Filho, M. F. da Costa and R. S. Angelica, Geochemical mapping and background concentrations of iron and potentially toxic elements in active stream sediments from Carajás, Brazil – implications for risk management, J. South Am. Earth Sci., 2019, 92, 161–166 CrossRef.
- P. K. Sahoo, R. Dall'Agnol, G. N. Salomao, J. da S. Ferreira Junior, M. S. da Silva, G. C. Martins, P. W. M. S. Filho, M. A. Powell, C. W. Maurity, R. S. Angelica, M. F. da Costa and J. O. Siqueira, Source and background threshold values of potentially toxic elements in soils by multivariate statistics and GIS-base mapping a high density sampling survey in the Parauapebas basin, Brazilian Amazon, Environ. Geochem. Health, 2020, 42, 255–282 CrossRef CAS PubMed.
- O. H. Williams and N. L. J. Rintoul-Hynes, Legacy of war: Pedogenesis divergence and heavy metal contamination on the WWI front line a century after battle, Eur. J. Soil Sci., 2022, 73(4), e13297, DOI:10.1111/ejss.13297.
- A. Azogh, S. K. Marashi and T. Babaeinejad, Effect of zeolite on absorption and distribution of heavy metal concentrations in roots and shoots of wheat under soil contaminated with weapons, Toxin Rev., 2019, 40(4), 1301–1307, DOI:10.1080/15569543.2019.1684949.
- S. Vasarevičius and K. Greičiūte, Investigation of soil pollution with heavy metals in Lithuanian military grounds, J. Environ. Eng. Landsc. Manag., 2004, 12(4), 132–137, DOI:10.1080/16486897.2004.9636834.
-
M. A. Sultan, Evaluation of Soil pollution by heavy metals in Baghdad city using GIS, The 1st International Applied Geological Congress, Department of Geology, Islamic Azad University, Mashad Branch, Iran, April 2010, pp. 852–863 Search PubMed.
- D. Vidosavljević, D. Puntarić, V. Gvozdić, M. Jergović, M. Miškulin, I. Puntarić, E. Puntarić and S. Šijanović, Soil contamination as a possible long-term consequence of war in Croatia, Acta Agric. Scand., Sect. B, 2013, 63(4), 322–329, DOI:10.1080/09064710.2013.777093.
- R. K. Sharma, M. Agrawal and F. Marshall, Heavy metal contamination in vegetables grown in wastewater irrigated areas of Varanasi, India, Bull. Environ. Contam. Toxicol., 2006, 77(2), 312–318, DOI:10.1007/s00128-006-1065-0.
- M. Muchuweti, J. W. Birkett, E. Chinyanga, R. Zvauya, M. D. Scrimshaw and J. N. Lester, Heavy metal content of vegetables irrigated with mixture of wastewater and sewage sludge in Zimbabwe: Implications for human health, Agric., Ecosyst. Environ., 2006, 112, 41–48 CrossRef CAS.
- Z. F. Chen, Y. Zhao, Y. Zhu, X. Yang, J. Qiao, Q. Tian and Q. Zhang, Health risk of heavy metals in sewage-irrigated soils and edible seeds in Langfang of Hebei province, China, J. Sci. Food Agric., 2009, 90, 314–320 CrossRef PubMed.
- A. Singh, R. K. Sharma, M. Agrawal and F. M. Marshall, Health risk assessment of heavy metals via dietary intake of foodstuffs from the wastewater irrigated site of a dry tropical area of India, Food Chem. Toxicol., 2010, 48, 611–619 CrossRef CAS PubMed.
-
North-Press, Farmers in Idlib irrigate their crops from the sewage system, 2021, https://npasyria.com/73517/, accessed: 30.09.2022 Search PubMed.
-
Alghourbal together for Syria. Its stagnant water harms people and soil: a sewage river threatens life in the villages and towns of northern Syria, 2019. https://www.algherbal.com/archives/5076, Accessed: 30.09.2022 Search PubMed.
-
Syria Direct. ‘A ticking time bomb’: Hellish work at northwestern Syria's makeshift oil refineries, 2022, https://syriadirect.org/a-ticking-time-bomb-hellish-work-at-northwestern-syrias-makeshift-oil-refineries/, accessed: 29.09.2022 Search PubMed.
-
The Guardian, Makeshift oil refineries a necessary evil for locals in north-west Syria, 2020, https://www.theguardian.com/global-development/2020/apr/24/makeshift-oil-refineries-a-necessary-evil-for-locals-in-north-east-syria-study-finds, accessed: 19/12/2022 Search PubMed.
- D. Cocârţă, M. Stoian and A. Karademir, Crude Oil Contaminated Sites: Evaluation by Using Risk Assessment Approach, Sustainability, 2017, 9(8), 1365, DOI:10.3390/su9081365.
-
L. Protasevich, A. Oufland, B. Ponomarev, A. Galaktionov, V. Shatsky, V. Kazmin, I. Mikhailov, V. Medvedev, V. Kozlov, V. Faradzhev, E. Soulidi-Kondratyiev, V. Petrov, A. Razvaliayez and A. Krasnov, Geological map of Syria, Syrian Arab Republic, Ministry of Petroleum and Mineral Resource, 1964 Search PubMed.
-
EPA, Residential sampling for lead: protocols for dust and soil sampling final report, 747-R-95-001, US Environmental Protection Agency, Washington, D.C., vol. 29, March 1995, p. 38, https://www.epa.gov/sites/production/files/2014-03/documents/20012quz.pdf, Accessed 19/12/22 Search PubMed.
-
L. A. Richards, Diagnosis and Improvement of Saline and Alkali Soils, Agriculture Handbook, United State Department of Agriculture, 1954, vol. 60, pp. 69–82 Search PubMed.
-
M. L. Jackson, Soil chemical analysis, Prentice-Hall of India Private Limited, New Delhi, 1973 Search PubMed.
-
UNEP, Environmental Risks and Challenges of Anthropogenic Metals Flows and Cycles, A Report of the Working Group on the Global Metal Flows to the International Resource Panel, ed. van der Voet, E., Salminen, R., Eckelman, M., Mudd, G., Norgate, T. and Hischier, R., United Nations Environment Programme, 2013, p. 234, ISBN 978-92-807-3266-5 Search PubMed.
- A. T. Al-Mishwat and S. J. Nasir, Composition of the lower crust of the Arabian Plate: A xenolith perspective, Lithos, 2004, 72, 45–72 CrossRef CAS.
- T. L. Roberts, Cadmium and phosphorous fertilizers: the issues and the science, Procedia Eng., 2014, 83, 52–59, DOI:10.1016/j.proeng.2014.09.012 ..
- V. Azzi, V. Kazpard, B. Lartiges, A. Kobeissi, A. Kanso and A. G. El Samrani, Trace Metals in Phosphate Fertilizers Used in Eastern Mediterranean Countries, Clean: Soil, Air, Water, 2017, 45(1) DOI:10.1002/clen.201500988.
- H. H. Jaafar and E. Woertz, Agriculture as a funding source of ISIS: A GIS and remote sensing analysis, Food Policy, 2016, 64, 14–25, DOI:10.1016/j.foodpol.2016.09.002.
- A. Azough, S. K. Marashi and T. Babaeinejad, Growth characteristics and response of wheat to cadmium, nickel and magnesium sorption affected by zeolite in soil polluted with armaments, J. Adv. Environ. Health Res., 2017, 5(3), 163–171 CAS.
- T. M. Darwish, I. Jomaa, M. Awad and R. Boumetri, Preliminary contamination hazard assessment of land resources in Central Bekaa Plain of Lebanon, Leban. Sci. J., 2008, 9(2), 3–15 CAS.
- A. Hanedar, Assessment of airborne heavy metal pollution in soil and lichen in the Meric-Ergene Basin, Turkey, Environ. Technol., 2015, 36(20), 2588–2602 CrossRef CAS PubMed.
- S. Mohammed, K. Alsafadi, S. Hennawi, S. M. N. Mousavi, F. B. Kamal-Eddin and E. Harsanyie, Effects of long-term agricultural activities on the availability of heavy metals in Syrian soil: A case study in southern Syria, J. Saudi Soc. Agric. Sci., 2021, 20(8), 497–505 Search PubMed.
- F. Santos-Francés, A. Martínez-Graña, C. Zarza, A. Sánchez and P. Rojo, Spatial distribution of heavy metals and the environmental quality of soil in the Northern Plateau of Spain by geostatistical methods, Int. J. Environ. Res. Public Health, 2017, 14(6), 568 CrossRef PubMed.
- S. O. M. Al Mayyahi, Assessment of heavy metals pollution in the sediments surrounding of Badra oil field, Wasit, Iraq, Plant Arch., 2010, 18(2), 1889–1894 Search PubMed.
|
This journal is © The Royal Society of Chemistry 2023 |
Click here to see how this site uses Cookies. View our privacy policy here.