DOI:
10.1039/D3QM00273J
(Research Article)
Mater. Chem. Front., 2023,
7, 3398-3405
Peripheral engineering of platinum(II) dicarbene pincer complexes for efficient blue hyperphosphorescent organic light-emitting diodes†
Received
17th March 2023
, Accepted 9th May 2023
First published on 10th May 2023
Abstract
Pt(II) dicarbene pincer complexes have been explored as deep-blue phosphors with narrowband emissions. Generally, they form excimers easily and their relatively long emission lifetimes also limit their applications as OLED emitters. Herein, we developed a simple method to modify 1,3-bisimidazol-3-ium pro-chelates and obtained three Pt(II) dicarbene pincer complexes, thus studying the issue of excimer formation vs. peripheral appendages. As a result, blue emissions can be maintained at doping concentrations of up to 20 wt% for IPrtBuPt. Moreover, the emission of IPrtBuPt was found to significantly overlap with the absorption of ν-DABNA, an efficient blue boron/nitrogen based multi-resonant TADF molecule. A hyper-OLED using IPrtBuPt as a sensitizer and ν-DABNA as a terminal emitter achieved EQEs of up to 33.59%, FWHM of 20 nm and CIE coordinates of (0.124, 0.148). These results demonstrated a successful example of utilizing both structural engineering and smart device architecture for getting efficient blue devices with suppressed efficient roll-off.
Introduction
While there has been significant progress in the utilization of both red and green phosphors for fabrication of organic light-emitting diodes (OLEDs),1–5 the development of blue phosphors is significantly delayed. Therefore, blue fluorophores, which can only utilize one quarter of electrogenerated excitons, are still being used in modern commercial OLED products.6 Thus, novel blue emitters and device architectures are urgently needed for boosting the performance of blue OLED devices.7,8
Multi-resonant thermally activated delayed fluorescent (MR-TADF) molecules have become the most intensively researched topics due to their merits including fast radiative decay,9–11 narrow full width at half maximum (FWHM), proneness to adapt horizontal alignment and high luminescent efficiency.12,13 Thus, MR-TADF molecules possess great potential to improve external quantum efficiency (EQE) towards 40% with color purity comparable to quantum dot LEDs,14–17 simultaneously. Another type of promising emitter is an N-heterocyclic carbene (C^CNHC) coordinated iridium(III) complex (Ir(C^CNHC)3),18 a representative emitter of which, i.e., mer-Ir(CF3pbp)3, has delivered an EQEmax of 21.2% with Commission Internationale de L'Eclairage (CIE) coordinates of (0.16, 0.05),19 showing performances comparable to the state-of-the-art TADF emitters. This result is ascribed to the strong σ-donating C^CNHC chelate, which greatly destabilizes the metal-centered (MC) dd excited state and enlarged energy offset between the emitting excited states and upper lying MC dd quenching states.7,8
Compared to TADF emitters and Ir(III) complexes, the development of deep-blue Pt(II) complexes is relatively lagging behind. In fact, the large spin-orbital coupling (SOC) constant of the Pt atom (4881 vs. 3450 cm−1 for Ir)20 confirmed its potential for achieving efficient phosphorescence. Incorporating an N-heterocyclic carbene entity, Li et al. demonstrated a tetradentate N^C^C^CNHC coordinated Pt(II) complex with an EQEmax of 24.8% and CIE coordinates of (0.148, 0.079).21,22 However, its square-planar geometry and long radiative lifetime resulted in a low luminance and increased efficiency roll-off at high luminance (e.g., 1000 cd m−2). Modifications to alleviate this problem are impeded by the sophisticated synthetic procedure of tetradentate chelates.23,24 In contrast, the dicarbene pincer chelate can be modified much more easily. A pioneering research study shows a decent blue emission with a narrow FWHM down to ∼20 nm and a blue device with an EQEmax of 14.8% and CIE coordinates of (0.16, 0.13) using Pt(II) based CNHC^C^CNHC pincer complexes as dopants,25,26 although they gave dimers (or excimers) at higher doping ratios. On the other hand, lowered doping ratios may lead to unbalanced transport of carriers, which undermine device performance at high brightness (e.g., 100 and 1000 cd m−2). In fact, the emission of our Pt(II) pincer complexes exerts good spectral overlaps with absorption bands of the blue terminal emitter, i.e., ν-DABNA (N7,N7,N13,N13,5,9,11,15-octaphenyl-5,9,11,15-tetrahydro-5,9,11,15-tetraaza-19b,20b-diboradinaphtho [3,2,1-de:1′,2′,3′-jk]pentacene-7,13-diamine).15 This encouraged us to employ the Förster resonance energy transfer (FRET) process in realizing efficient blue OLEDs.27–29
Herein, we modify the peripheral groups of the CNHC^C^CNHC chelate to minimize intermolecular interactions and to develop three Pt(II) (CNHC^C^CNHC) pincer complexes, namely MeCF3Pt, IPrCF3Pt and IPrtBuPt. These modifications have successfully addressed the issue on excimer formation and given pure blue emission with EQEmax = 13.96% and CIE coordinates of (0.159, 0.241) from an IPrtBuPt-based OLED device. More importantly, the emission of IPrtBuPt shows a significant overlap with the absorption of MR-TADF blue emitter – ν-DABNA. A hyperphosphorescent device with IPrtBuPt as a dopant sensitizer and ν-DABNA as a terminal emitter is demonstrated to show a maximum EQE of 33.59% and color coordinates of (0.124, 0.148). It also revealed very mild efficiency roll-offs comparable to other hyper-OLED devices in the literature,7,8,15,23,24,30–38 together with a high max. brightness of ∼30
000 cd m−2. Eventually, this study shows that a combination of simple structural modifications of Pt(II) dicarbene pincer complexes and smart device engineering can be promising in the generation of high performance blue OLEDs.
Results and discussion
Synthesis of the dicarbene pincer pro-chelates follows the same protocol reported in our previous works.39,40 The Pt(II) complexes are obtained by refluxing K2PtCl4 and hexafluorophosphate salts of their corresponding 1,3-bisimida-zol-3-ium pro-chelates (Scheme 1 and the Experimental section) in the presence of potassium carbonate in propionic acid (yield: 34–54%, Scheme 1). It is noted that this method is simpler and more straightforward than the other literature precedents, which needs expensive reagents and afforded low product yields (<10%).25,26 Finally, all products were characterized using 1H and 19F NMR spectroscopies, high-resolution mass spectrometry (HR-MS), and single-crystal X-ray crystallographic analysis.
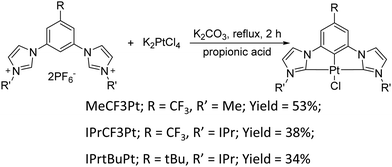 |
| Scheme 1 Synthetic route and chemical structures of MeCF3Pt, IPrCF3Pt and IPrtBuPt. | |
UV-Vis absorption spectra of these Pt(II) complexes show two main absorption bands (Fig. 1), namely π–π* absorption of the aromatic entities at ∼320 nm and the mixed ligand-centered π–π* absorption and metal-to-ligand charge transfer (MLCT) at the region ≥330 nm. Compared to the CF3-substituted complexes (MeCF3Pt and IPrCF3Pt), tBu-substituted IPrtBuPt presents a slightly redshifted absorption peak (Table 1) and decreased MLCT intensity, implying a smaller energy gap and less MLCT involvement in transition. Thus, the substitution of the electron-rich group at the benzene ring will reduce the mix between the π* orbital of the ligand and dπ orbital of the metal center.41 Consistently, a slightly redshift emission in IPrtBuPt (458 vs. 444 nm) with an enhanced 0–1 transition band (FWHM increases from 16 to 44 nm, ascribed to a greater contribution from ligand-centred π–π* transition)42 is observed in the photoluminescent (PL) spectrum in CH2Cl2 solution (10−5 M). Concomitantly, the longest radiative lifetime (7.84 μs) is observed from IPrtBuPt. Isopropyl substituents in IPrCF3Pt and IPrtBuPt introduce an extra vibrational degree of freedom to the molecule and result in lower photoluminescence quantum yields (PLQYs) compared to MeCF3Pt. Upon increasing the concentration from 10−5 to 10−2 M (Fig. 2), obvious excimer emission (peak at ∼600 nm) is observed for MeCF3Pt and IPrCF3Pt. Notably, no such lower energy shoulder was observed for IPrtBuPt.
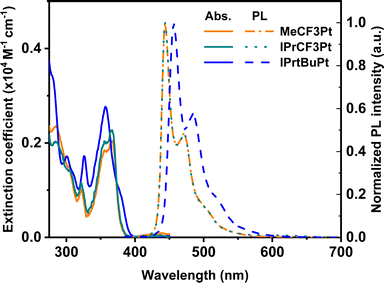 |
| Fig. 1 Normalized UV-vis absorption (solid) and emission (dashed) spectra of the new Pt(II) complexes in DCM solution. | |
Table 1 Photophysical data of the Pt(II) complex in DCM (10−5 M)
Name |
λ
Abs
[nm] |
λ
em
[nm] |
PLQYab [%] |
FWHMa [nm] |
τ
[ns] |
Measured in degassed DCM.
Coumarin 102 (C102) in MeOH (10−5 M, Q.Y. = 87% and λmax = 480 nm) was employed as the standard.
|
MeCF3Pt |
320, 356, 367 |
444, 471 |
73.2 |
16 |
3187 |
IPrCF3Pt |
320, 356, 367 |
444, 471 |
46.4 |
16 |
2334 |
IPrtBuPt |
326, 357, 376 (sh) |
458, 485 |
46.7 |
44 |
3660 |
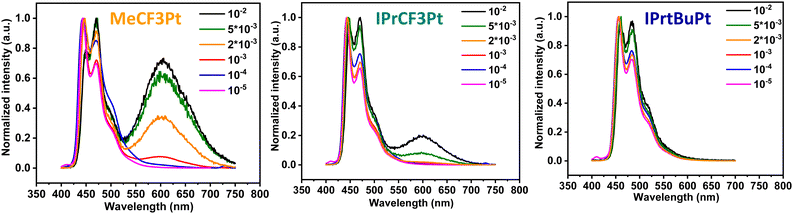 |
| Fig. 2 Concentration-dependent PL spectra of the new Pt(II) complexes in DCM solution. | |
To better understand their photophysical properties, density functional theory (DFT) and time dependent-DFT (TD-DFT) calculations were carried out at the B3LYP/def2SVP level using the Gaussian16 program package.43 As presented in Fig. 3, the highest occupied molecular orbitals (HOMOs) are localized on the central phenyl group of the pincer chelate and the Pt–Cl vector, while the lowest unoccupied molecular orbitals (LUMOs) are distributed over the whole molecules. Calculated HOMO and LUMO gaps followed an ascending order MeCF3Pt < IPrCF3Pt < IPrtBuPt, confirming the increasing electron-donating ability of alkyl substituents between the chelates (i.e., CF3/Me to CF3/IPr then tBu/IPr). As the HOMO–LUMO hopping account for over 80% of the lowest energy process, the transitions between S0 and S1/T1 are consequently assigned to an admixture of MLCT/ILCT/XLCT (X denotes a halide), and with MLCT contributions being 12.9%, 11.5% and 11.8% for MeCF3Pt, IPrCF3Pt and IPrtBuPt (Table S1, ESI†), respectively. These percentages are considerably small compared to those of Pt(II) complexes documented in literature reports (ca. 20%),44–46 which explains the relatively long radiative lifetimes of our Pt(II) carbene pincer emitters.
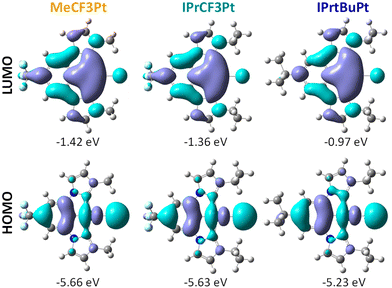 |
| Fig. 3 Frontier molecular orbital distributions of MeCF3Pt, IPrCF3Pt and IPrtBuPt. | |
Single crystal analysis reveals that MeCF3Pt, IPrCF3Pt and IPrtBuPt possessed similar planar geometries but distinct packing behaviours. Selected bond lengths and angles are summarized in Table S2 (ESI†). The Pt–Cl, Pt–Cph and Pt–CNHC lengths are 2.38–2.41, 1.93–1.94 and 2.02–2.04 Å, respectively. The Cl–Pt–CPh and CNHC–Pt–CNHC bond angles were in the range of 177–179° and 156–158° respectively, presenting similar coordinating properties to other carbene Pt(II) pincer complexes in literature reports.25,26 However, as shown in Fig. 4, intimately close Pt⋯Pt and π–π/Pt–π interactions are found in the unit cell of MeCF3Pt, confirming formation of excimers even at low doping concentrations. When the bulky tert-butyl group was added, only π–π interactions were observed, as indicated in the crystal cells of IPrCF3Pt. The addition of the tert-butyl group in IPrtBuPt also eliminated the respective face-to-face stacking interaction, explaining the photophysical properties observed earlier.
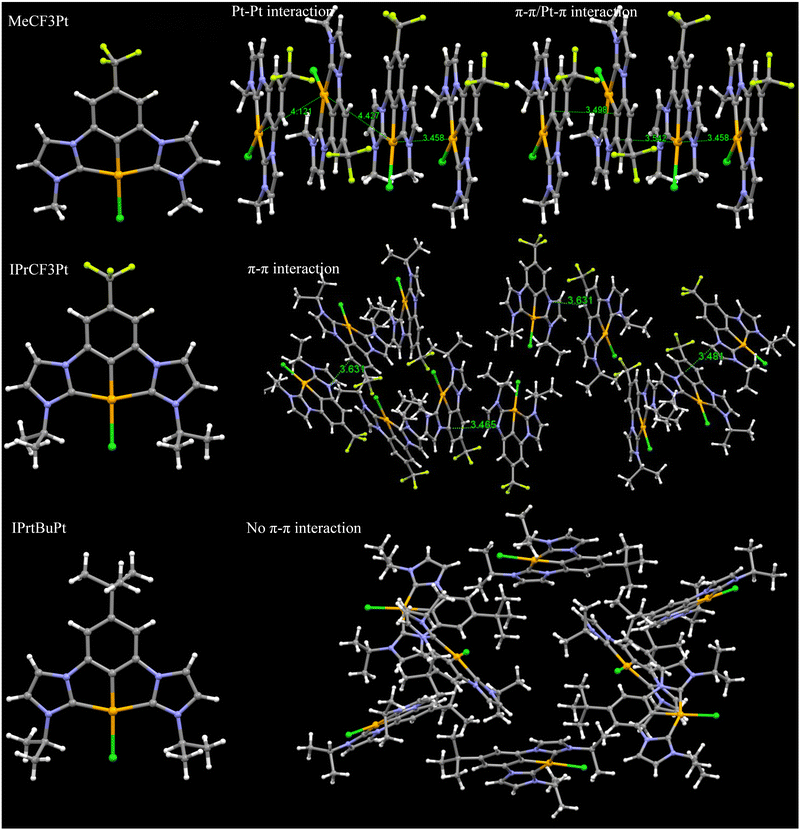 |
| Fig. 4 Structure and intermolecular packing observed in single crystals of MeCF3Pt, IPrCF3Pt and IPrtBuPt. | |
Before device fabrication, decomposition temperatures (Td) of all studied complexes were analysed via thermogravimetric measurements (Fig. S1, ESI†) and all of them present good thermal stability with Td exceeding 300 °C, indicating they are suitable for vacuum-deposition processes. These Pt(II) complexes were employed as dopant emitters with an OLED configuration of ITO/TAPC(20 nm)/TCTA(10 nm)/mCP(10 nm)/EML (20 nm)/3TPYMB(40 nm)/LiF(1 nm)/Al, in which bipolar mCPCN is employed as the host material in the EML. As presented in Fig. S2 (ESI†) and Table 2, being consistent in their packing properties, MeCF3Pt exhibited a broadened lower energy emission even at a doping level of 1 wt%. This excimer emission (peak at 597 nm, similar to the PL observed in doped thin films, excluding the contribution of the electromer, Fig. S3, ESI†) has gained intensity in comparison to the monomer emission (peak at 447 nm) vs. doping concentration dramatically. The higher intensity of long wavelength peak in EL (Fig. S2c, ESI†) compared to PL (Fig. S3, ESI†) revealed using EL spectra to detect the excimer signal is more sensitive and efficient. Its EQEmax was increased from 3.51 to 17.0%, which is ascribed to a favourable energy transfer from the host to the dopant and balanced carrier transport when doping concentration increased from 1 to 5 wt% and greater. Similarly, IPrCF3Pt exhibited excimer emissions starting from 8 wt% (Fig. S2f, ESI†). In sharp contrast, IPrtBuPt-based device displayed only higher energy, structured monomer emission even at 20 wt% (Fig. S2i, ESI†) and maintained a fairly stable CIE-y and EQEmax data of 0.221 and 12.38% @5 wt%, to 0.241 and 13.96% @20 wt%. However, the blue devices have low maximum brightness and show a dramatic efficiency roll-off with increased current density.
Table 2 Summary of device performance of MeCF3Pt, IPrCF3Pt and IPrtBuPt with different doping ratios
Dopant |
V
on
[V] |
CEmaxb [cd A−1] |
EQEmax [%] |
CIEc [x, y] |
Turn-on voltage at 1 cd m−2.
Maximum current efficiency.
Recorded at 100 cd m−2.
|
MeCF3Pt 1 wt% |
3.5 |
5.36 |
3.51 |
0.326, 0.26 |
MeCF3Pt 3 wt% |
3.5 |
20.36 |
9.77 |
0.454, 0.363 |
MeCF3Pt 5 wt% |
3.5 |
37.52 |
17.0 |
0.529, 0.419 |
IPrCF3Pt 5 wt% |
3.5 |
5.29 |
5.03 |
0.163, 1.18 |
IPrCF3Pt 8 wt% |
3.5 |
10.26 |
9.12 |
0.187, 0.138 |
IPrCF3Pt 10 wt% |
3 |
17.39 |
13.3 |
0.191, 0.145 |
IPrtBuPt 5 wt% |
3.5 |
20.48 |
12.38 |
0.153, 0.221 |
IPrtBuPt 10 wt% |
3.5 |
23.2 |
13.93 |
0.156, 0.223 |
IPrtBuPt 20 wt% |
3.0 |
24.26 |
13.96 |
0.159, 0.241 |
In view of its good resistance to excimer formation and good EL performance at high concentrations, IPrtBuPt was further employed as a dopant sensitizer for a narrow band MR-TADF terminal emitter: ν-DABNA. Importantly, the emission spectrum of IPrtBuPt shows a good overlap with the absorption spectrum of ν-DABNA. By integrating the overlap as shown in the ESI,† a large FRET radius of 7.1 nm (Fig. S4, ESI†) is estimated,47,48 implying the potentially effective energy transfer processes. A hyperphosphorescent device with a configuration of ITO/HATCN/HATCN
:
TPAPB (3
:
7 30 nm)/TPAPB (10 nm)/PCzAc (10 nm)/16 wt% IPrtBuPt & 1 wt% ν-DABNA in mCBP
:
SiCzTrz 1
:
1 (30 nm)/DBFTrz (5 nm)/TmPyPB/LiF/Al was next fabricated. As shown in Fig. 5, a sharp EL profile peak at 471 nm and a narrow FWHM of 20 nm were achieved. EL spectra of the hyper device remained the same until high voltage (≥13 V), where the brightness was too high and pixels near the peaks were saturated (Fig. S5, ESI†). Impressively, a much-relieved roll-off with EQEmax and EQE1000 (EQE at 1000 cd m−2) of 33.59 and 26.61%, respectively, comparable to the best hyper-OLED ever reported in the literature (Fig. S6, ESI†) were achieved.7,8,15,24,30–34,36–38,49,50 However, the device lifetime of the hyper-device is only ∼0.3 h (Fig. S7, ESI†), which may be attributed to the nucleophilic vulnerability of the Pt–Cl bond.
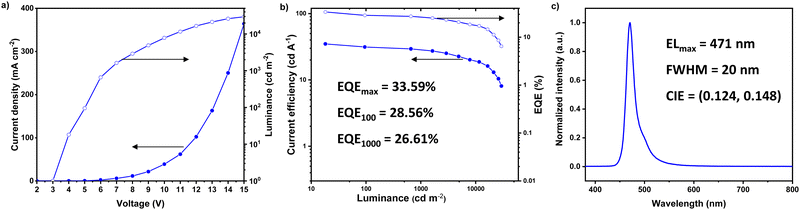 |
| Fig. 5 (a) Current density–voltage–luminance plots, (b) current efficiency–luminance–EQE curves and (c) EL spectrum of an IPrtBuPt based hyper-OLED. | |
Conclusions
In summary, Pt(II) dicarbene pincer complexes were modified using different peripheral bulky substituents. The annoying issue of excimer formation for planar Pt(II) complexes is addressed by these peripheral groups, and blue emissions can be maintained even at a high concentration of 20 wt% in the doped device of IPrtBuPt. Notably, IPrtBuPt has successfully served as a dopant sensitizer for a ν-DABNA based hyper-OLED, giving max. EQE up to 33.59%, FWHM of 20 nm, and CIE coordinates of (0.124, 0.148). These results demonstrated a utilization of both the engineered emitters and smart device architecture to foster efficient blue OLED devices with mild efficiency roll-offs.
Experimental details
Synthesis
All solvents and reagents were purchased from commercial resources and used as received. All reactions were carried out in the air unless otherwise stated. Hexafluorophosphate salts of 1,3-bisimidazol-3-ium pro-chelates (denoted as [(mimf)H3·(PF6)2], [(pimf)H3·(PF6)2] and [(pimb)H3·(PF6)2]), namely 1,1′-(5-(trifluoromethyl)-1,3-phenylene)bis(3-methyl-1H-imidazol-3-ium), 1,1′-(5-(trifluoromethyl)-1,3-phenylene)bis(3-isopropyl-1H-imidazol-3-ium) and 1,1′-(5-(tert-butyl)-1,3-phenylene)bis(3-isopropyl-1H-imidazol-3-ium), were obtained using the method depicted in our previous report.40,41
Synthesis of the Pt(II) chlorides
A mixture of [(mimf)H3 (PF6)2] (1.1 eq.), K2PtCl4 (1 eq.) and K2CO3 (10 eq.) was heated in refluxing propionic acid for 2 h. After cooled to RT, propionic acid was evaporated and the residue was dissolved in CH2Cl2. The solution was washed with deionized water, dried over anhydrous Na2SO4, filtered and concentrated to dryness. The crude product was purified by silica gel column chromatography, eluting with a (1
:
4) mixture of ethyl acetate and hexane to afford the targeted Pt(II) carbene pincer complexes.
Spectral data of MeCF3Pt.
1H NMR (400 MHz, CDCl3, 296 K): δ 7.37 (d, J = 2.0 Hz, 2H), 7.06 (s, JPtH = 16.8 Hz, 2H), 6.98 (d, J = 2.0 Hz, 2H), 4.26 (s, 6H); 19F NMR (376 MHz, CDCl3, 296 K): δ −61.17 (s, 3F). HRMS (Q-TOF) m/z for [M − Cl]+ calcd 500.0657, found 500.0639.
Spectral data of IPrCF3Pt.
1H NMR (400 MHz, CDCl3, 296 K): δ 7.43 (d, J = 2.0 Hz, 2H), 7.14 (d, J = 2.0 Hz, 2H), 7.12 (s, JPtH = 19.2 Hz, 2H), 6.18 (q, J = 6.8 Hz, 2H), 1.52 (d, J = 6.8 Hz, 12H); 19F NMR (376MHz, CDCl3, 296 K): δ −61.22 (s, 3F). HRMS (Q-TOF) m/z for [M − Cl]+ calcd 556.1283, found 556.1272.
Spectral data of IPrtBuPt.
1H NMR (400 MHz, CDCl3, 296 K): δ 7.39 (d, J = 2.0 Hz, 2H), 7.07 (d, J = 2.0 Hz, 2H), 6.90 (s, JPtH = 16.0 Hz, 2H), 6.15 (q, J = 6.8 Hz, 2H), 1.50 (d, J = 6.8 Hz, 12H), 1.36 (s, 9H). HRMS (Q-TOF) m/z for [M − Cl]+ calcd 544.2040, found 544.2020.
Author contributions
Y. C. and C. S. L. initiated and provided financial support for the project. Y. C. designed the complexes. J. Y., Z. L. Z. and L.W. F. synthesized and characterized the new molecules. Y. J, C. C. and Z. L. Z. carried out photophysical measurements. J. H. T performed the computational calculation under the supervision of Z. L. Z. J. Y. and Z. L. Z. contributed to crystal growth and crystal data analysis. Z. L. Z. fabricated the OLEDs and measured the device performance. Y. J. and Z.L. Z. wrote the first draft. Z. L. Z., Y. C. and C.S. L. provided suggestions on experiments, revised manuscript and supervised the project. All authors discussed the experimental result and reviewed the manuscript.
Conflicts of interest
There are no conflicts to declare.
Acknowledgements
C. S. L. is thankful for the financial support from the Research Grants Council of the Hong Kong Special Administrative Region, China (Project No. C1009-17E, CityU 11300320 and N_CityU104/20). Y. C. is supported by the Research Grants Council funding: CityU 11304221 and CityU 11312722.
Notes and references
- Y. Im, S. Y. Byun, J. H. Kim, D. R. Lee, C. S. Oh, K. S. Yook and J. Y. Lee, Recent Progress in High-Efficiency Blue-Light-Emitting Materials for Organic Light-Emitting Diodes, Adv. Funct. Mater., 2017, 27, 1603007 CrossRef.
- S. K. Jeon, H. L. Lee, K. S. Yook and J. Y. Lee, Recent Progress of the Lifetime of Organic Light-Emitting Diodes Based on Thermally Activated Delayed Fluorescent Material, Adv. Mater., 2019, 31, 1803524 CrossRef PubMed.
- A. Maheshwaran, V. G. Sree, H.-Y. Park, H. Kim, S. H. Han, J. Y. Lee and S.-H. Jin, High Efficiency Deep-Blue Phosphorescent Organic Light-Emitting Diodes with CIE x, y (≤0.15) and Low Efficiency Roll-Off by Employing a High Triplet Energy Bipolar Host Material, Adv. Funct. Mater., 2018, 28, 1802945 CrossRef.
- A. K. Pal, S. Krotkus, M. Fontani, C. F. R. Mackenzie, D. B. Cordes, A. M. Z. Slawin, I. D. W. Samuel and E. Zysman-Colman, High-Efficiency Deep-Blue-Emitting Organic Light-Emitting Diodes Based on Iridium(III) Carbene Complexes, Adv. Mater., 2018, 30, 1804231 CrossRef PubMed.
- H.-Y. Park, A. Maheshwaran, C.-K. Moon, H. Lee, S. S. Reddy, V. G. Sree, J. Yoon, J. W. Kim, J. H. Kwon, J.-J. Kim and S.-H. Jin, External Quantum Efficiency Exceeding 24% with CIEy Value of 0.08 using a Novel Carbene-Based Iridium Complex in Deep-Blue Phosphorescent Organic Light-Emitting Diodes, Adv. Mater., 2020, 32, 2002120 CrossRef CAS PubMed.
- A. Monkman, Why Do We Still Need a Stable Long Lifetime Deep Blue OLED Emitter?, ACS Appl. Mater. Interfaces, 2021, 14, 20463 CrossRef PubMed.
- J. Jin, Z. Zhu, J. Yan, X. Zhou, C. Cao, P.-T. Chou, Y.-X. Zhang, Z. Zheng, C.-S. Lee and Y. Chi, Iridium(III) Phosphors–Bearing Functional 9-Phenyl-7,9-dihydro-8H-purin-8-ylidene Chelates and Blue Hyperphosphorescent OLED Devices, Adv. Photonics Res., 2022, 3, 2100381 CrossRef CAS.
- Z.-L. Zhu, P. Gnanasekaran, J. Yan, Z. Zheng, C.-S. Lee, Y. Chi and X. Zhou, Efficient Blue Electrophosphorescence and Hyperphosphorescence Generated by Bis-tridentate Iridium(III) Complexes, Inorg. Chem., 2022, 61, 8898 CrossRef CAS PubMed.
- T. Hatakeyama, K. Shiren, K. Nakajima, S. Nomura, S. Nakatsuka, K. Kinoshita, J. Ni, Y. Ono and T. Ikuta, Ultrapure Blue Thermally Activated Delayed Fluorescence Molecules: Efficient HOMO–LUMO Separation by the Multiple Resonance Effect, Adv. Mater., 2016, 28, 2777 CrossRef CAS PubMed.
- M. Yang, I. S. Park and T. Yasuda, Full-Color, Narrowband, and High-Efficiency Electroluminescence from Boron and Carbazole Embedded Polycyclic Heteroaromatics, J. Am. Chem. Soc., 2020, 142, 19468 CrossRef CAS PubMed.
- Y. Yuan, X. Tang, X.-Y. Du, Y. Hu, Y.-J. Yu, Z.-Q. Jiang, L.-S. Liao and S.-T. Lee, The Design of Fused Amine/Carbonyl System for Efficient Thermally Activated Delayed Fluorescence: Novel Multiple Resonance Core and Electron Acceptor, Adv. Opt. Mater., 2019, 7, 1801536 CrossRef.
- A. Hofmann, M. Schmid and W. Brütting, The Many Facets of Molecular Orientation in Organic Optoelectronics, Adv. Opt. Mater., 2021, 9, 2101004 CrossRef CAS.
- H. J. Kim and T. Yasuda, Narrowband Emissive Thermally Activated Delayed Fluorescence Materials, Adv. Opt. Mater., 2022, 10, 2201714 CrossRef CAS.
- X.-C. Fan, K. Wang, Y.-Z. Shi, Y.-C. Cheng, Y.-T. Lee, J. Yu, X.-K. Chen, C. Adachi and X.-H. Zhang, Ultrapure green organic light-emitting diodes based on highly distorted fused π-conjugated molecular design, Nat. Photonics, 2023, 17, 280–285 CrossRef CAS.
- Y. Kondo, K. Yoshiura, S. Kitera, H. Nishi, S. Oda, H. Gotoh, Y. Sasada, M. Yanai and T. Hatakeyama, Narrowband deep-blue organic light-emitting diode featuring an organoboron-based emitter, Nat. Photonics, 2019, 13, 678 CrossRef CAS.
- Y.-C. Cheng, X.-C. Fan, F. Huang, X. Xiong, J. Yu, K. Wang, C.-S. Lee and X.-H. Zhang, A Highly Twisted Carbazole-Fused DABNA Derivative as an Orange-Red TADF Emitter for OLEDs with Nearly 40% EQE, Angew. Chem., Int. Ed., 2022, 134, e202212575 Search PubMed.
- Y. X. Hu, J. Miao, T. Hua, Z. Huang, Y. Qi, Y. Zou, Y. Qiu, H. Xia, H. Liu, X. Cao and C. Yang, Efficient selenium-integrated TADF OLEDs with reduced roll-off, Nat. Photonics, 2022, 16, 803 CrossRef CAS.
- G. Ni, J. Yan, Y. Wu, F. Zhou, P.-T. Chou and Y. Chi, Transition-metal phosphors with emission peak maximum on and beyond the visible spectral boundaries, Inorg. Chem. Front., 2023, 10, 1395 RSC.
- R. Kumaresan, H.-Y. Park, A. Maheshwaran, H. Park, Y. Do, M. Song, J. Yoon, S. I. Ahn and S.-H. Jin, High Performance Solution-Processed Deep-Blue Phosphorescence Organic Light-Emitting Diodes with EQE Over 24% by Employing New Carbenic Ir(III) Complexes, Adv. Opt. Mater., 2021, 10, 2101686 CrossRef.
-
S. L. Murov, I. Carmichael and G. L. Hug, Handbook of photochemistry, CRC Press, 1993 Search PubMed.
- T. Fleetham, G. Li and J. Li, Phosphorescent Pt(II) and Pd(II) Complexes for Efficient, High-Color-Quality, and Stable OLEDs, Adv. Mater., 2017, 29, 1601861 CrossRef PubMed.
- T. Fleetham, G. Li, L. Wen and J. Li, Efficient “Pure” Blue OLEDs Employing Tetradentate Pt Complexes with a Narrow Spectral Bandwidth, Adv. Mater., 2014, 26, 7116 CrossRef CAS PubMed.
- J. Liu, T.-L. Lam, M.-K. Sit, Q. Wan, C. Yang, G. Cheng and C.-M. Che, Pure blue phosphorescent platinum(II) emitters supported by NHC-based pincer type ligands with unitary emission quantum yields, J. Mater. Chem. C, 2022, 10, 10271 RSC.
- K.-W. Lo, G. S. M. Tong, G. Cheng, K.-H. Low and C.-M. Che, Dinuclear PtII Complexes with Strong Blue Phosphorescence for Operational Stable Organic Light-Emitting Diodes with EQE up to 23% at 1000 cd m−2, Angew. Chem., Int. Ed., 2022, 61, e202115515 CrossRef CAS PubMed.
- T. Fleetham, Z. Wang and J. Li, Efficient deep blue electrophosphorescent devices based on platinum(II) bis(n-methyl-imidazolyl)benzene chloride, Org. Electron., 2012, 13, 1430 CrossRef CAS.
- X. Zhang, A. M. Wright, N. J. DeYonker, T. K. Hollis, N. I. Hammer, C. E. Webster and E. J. Valente, Synthesis, Air Stability, Photobleaching, and DFT Modeling of Blue Light Emitting Platinum CCC-N-Heterocyclic Carbene Pincer Complexes, Organometallics, 2012, 31, 1664 CrossRef CAS.
- M. A. Baldo, M. E. Thompson and S. R. Forrest, High-efficiency fluorescent organic light-emitting devices using a phosphorescent sensitizer, Nature, 2000, 403, 750 CrossRef CAS PubMed.
- D. Zhang, L. Duan, Y. Li, H. Li, Z. Bin, D. Zhang, J. Qiao, G. Dong, L. Wang and Y. Qiu, Towards High Efficiency and Low Roll-Off Orange Electrophosphorescent Devices by Fine Tuning Singlet and Triplet Energies of Bipolar Hosts Based on Indolocarbazole/1, 3, 5-Triazine Hybrids, Adv. Funct. Mater., 2014, 24, 3551 CrossRef CAS.
-
Thermally activated delayed fluorescence organic light-emitting diodes (TADF-OLEDs), ed. L. Duan, Woodhead Publishing, An imprint of Elsevier, Duxford, DK Cambridge, MA Kidlington, OX, 2022 Search PubMed.
- R. Braveenth, H. Lee, J. D. Park, K. J. Yang, S. J. Hwang, K. R. Naveen, R. Lampande and J. H. Kwon, Achieving Narrow FWHM and High EQE Over 38% in Blue OLEDs Using Rigid Heteroatom-Based Deep Blue TADF Sensitized Host, Adv. Funct. Mater., 2021, 31, 2105805 CrossRef CAS.
- D. Zhang, Y. Wada, Q. Wang, H. Dai, T. Fan, G. Meng, J. Wei, Y. Zhang, K. Suzuki, G. Li, L. Duan and H. Kaji, Highly Efficient and Stable Blue Organic Light-Emitting Diodes based on Thermally Activated Delayed Fluorophor with Donor-Void-Acceptor Motif, Adv. Sci., 2022, 2106018 CrossRef CAS PubMed.
- S. O. Jeon, K. H. Lee, J. S. Kim, S.-G. Ihn, Y. S. Chung, J. W. Kim, H. Lee, S. Kim, H. Choi and J. Y. Lee, High-efficiency, long-lifetime deep-blue organic light-emitting diodes, Nat. Photonics, 2021, 15, 208 CrossRef CAS.
- C.-Y. Chan, M. Tanaka, Y.-T. Lee, Y.-W. Wong, H. Nakanotani, T. Hatakeyama and C. Adachi, Stable pure-blue hyperfluorescence organic light-emitting diodes with high-efficiency and narrow emission, Nat. Photonics, 2021, 15, 203 CrossRef CAS.
- T. Huang, Q. Wang, G. Meng, L. Duan and D. Zhang, Accelerating Radiative Decay in Blue Through-Space Charge Transfer Emitters by Minimizing the Face-to-Face Donor–Acceptor Distances, Angew. Chem., Int. Ed., 2022, 134, e202200059 Search PubMed.
- K. Stavrou, A. Danos, T. Hama, T. Hatakeyama and A. Monkman, Hot Vibrational States in a High-Performance Multiple Resonance Emitter and the Effect of Excimer Quenching on Organic Light-Emitting Diodes, ACS Appl. Mater. Interfaces, 2021, 13, 8643 CrossRef CAS PubMed.
- W. J. Chung, K. H. Lee, M. Jung, K. M. Lee, H. C. Park, M.-S. Eum and J. Y. Lee, Over 30 000 h Device Lifetime in Deep Blue Organic Light-Emitting Diodes with y Color Coordinate of 0.086 and Current Efficiency of 37.0 cd A−1, Adv. Opt. Mater., 2021, 9, 2100203 CrossRef CAS.
- M. Mamada, H. Katagiri, C.-Y. Chan, Y.-T. Lee, K. Goushi, H. Nakanotani, T. Hatakeyama and C. Adachi, Highly Efficient Deep-Blue Organic Light-Emitting Diodes Based on Rational Molecular Design and Device Engineering, Adv. Funct. Mater., 2022, 32, 2204352 CrossRef CAS.
- S. Nam, J. W. Kim, H. J. Bae, Y. M. Maruyama, D. Jeong, J. Kim, J. S. Kim, W.-J. Son, H. Jeong, J. Lee, S.-G. Ihn and H. Choi, Improved Efficiency and Lifetime of Deep-Blue Hyperfluorescent Organic Light-Emitting Diode using Pt(II) Complex as Phosphorescent Sensitizer, Adv. Sci., 2021, 8, 2100586 CrossRef CAS PubMed.
- C.-Y. Kuei, W.-L. Tsai, B. Tong, M. Jiao, W.-K. Lee, Y. Chi, C.-C. Wu, S.-H. Liu, G.-H. Lee and P.-T. Chou, Bis-Tridentate Ir(III) Complexes with Nearly Unitary RGB Phosphorescence and Organic Light-Emitting Diodes with External Quantum Efficiency Exceeding 31%, Adv. Mater., 2016, 28, 2795 CrossRef CAS PubMed.
- Z.-L. Zhu, L.-Y. Hsu, W.-S. Tai, S.-F. Ni, C.-S. Lee and Y. Chi, Revealing the role of 1,2,4-triazolate fragment of blue-emitting bis-tridentate Ir(III) phosphors: photophysical properties, photo-stabilities, and applications, Mater. Today Energy, 2021, 20, 100636 CrossRef CAS.
- Z.-L. Zhu, W.-C. Chen, S.-F. Ni, J. Yan, S. F. Wang, L.-W. Fu, H.-Y. Tsai, Y. Chi and C.-S. Lee, Constructing deep-blue bis-tridentate Ir(III) phosphors with fluorene-based dianionic chelates, J. Mater. Chem. C, 2021, 9, 1318 RSC.
- J. Li, P. I. Djurovich, B. D. Alleyne, M. Yousufuddin, N. N. Ho, J. C. Thomas, J. C. Peters, R. Bau and M. E. Thompson, Synthetic Control of Excited-State Properties
in Cyclometalated Ir(III) Complexes Using Ancillary Ligands, Inorg. Chem., 2005, 44, 1713 CrossRef CAS PubMed.
-
M. J. Frisch, G. W. Trucks, H. B. Schlegel, G. E. Scuseria, M. A. Robb, J. R. Cheeseman, G. Scalmani, V. Barone, G. A. Petersson, H. Nakatsuji, X. Li, M. Caricato, A. V. Marenich, J. Bloino, B. G. Janesko, R. Gomperts, B. Mennucci, H. P. Hratchian, J. V. Ortiz, A. F. Izmaylov, J. L. Sonnenberg Williams, F. Ding, F. Lipparini, F. Egidi, J. Goings, B. Peng, A. Petrone, T. Henderson, D. Ranasinghe, V. G. Zakrzewski, J. Gao, N. Rega, G. Zheng, W. Liang, M. Hada, M. Ehara, K. Toyota, R. Fukuda, J. Hasegawa, M. Ishida, T. Nakajima, Y. Honda, O. Kitao, H. Nakai, T. Vreven, K. Throssell, J. A. Montgomery Jr., J. E. Peralta, F. Ogliaro, M. J. Bearpark, J. J. Heyd, E. N. Brothers, K. N. Kudin, V. N. Staroverov, T. A. Keith, R. Kobayashi, J. Normand, K. Raghavachari, A. P. Rendell, J. C. Burant, S. S. Iyengar, J. Tomasi, M. Cossi, J. M. Millam, M. Klene, C. Adamo, R. Cammi, J. W. Ochterski, R. L. Martin, K. Morokuma, O. Farkas, J. B. Foresman and D. J. Fox, Gaussian 16, Rev. C.01, 2016 Search PubMed.
- Z.-L. Zhu, S.-F. Wang, L.-W. Fu, J.-H. Tan, C. Cao, Y. Yuan, S.-M. Yiu, Y.-X. Zhang, Y. Chi and C.-S. Lee, Efficient Pyrazolo[5,4-f]quinoxaline Functionalized Os(II) based Emitter with Electroluminescence Peak Maximum at 811 nm, Chem. – Eur. J., 2022, 28, e202103202 CAS.
- L.-M. Huang, G.-M. Tu, Y. Chi, W.-Y. Hung, Y.-C. Song, M.-R. Tseng, P.-T. Chou, G.-H. Lee, K.-T. Wong, S.-H. Cheng and W.-S. Tsai, Mechanoluminescent and efficient white OLEDs for Pt(II) phosphors bearing spatially encumbered pyridinyl pyrazolate chelates, J. Mater. Chem. C, 2013, 1, 7582 RSC.
- K.-Y. Liao, C.-W. Hsu, Y. Chi, M.-K. Hsu, S.-W. Wu, C.-H. Chang, S.-H. Liu, G.-H. Lee, P.-T. Chou, Y. Hu and N. Robertson, Pt(II) Metal Complexes Tailored with a Newly Designed Spiro-Arranged Tetradentate Ligand; Harnessing of Charge-Transfer Phosphorescence and Fabrication of Sky Blue and White OLEDs, Inorg. Chem., 2015, 54, 4029 CrossRef CAS PubMed.
- C. Zhang, Y. Lu, Z. Liu, Y. Zhang, X. Wang, D. Zhang and L. Duan, A π–D and π–A Exciplex-Forming Host for High-Efficiency and Long-Lifetime Single-Emissive-Layer Fluorescent White Organic Light-Emitting Diodes, Adv. Mater., 2020, 32, 2004040 CrossRef CAS PubMed.
-
N. Hildebrandt and I. L. Medintz, FRET-Forster Resonance Energy Transfer: From Theory to Applications, Wiley-VCH Verlag GmbH, 2014 Search PubMed.
- D. Zhou, S. Wu, G. Cheng and C.-M. Che, A gold(III)–TADF emitter as a sensitizer for high-color-purity and efficient deep-blue solution-processed OLEDs, J. Mater. Chem. C, 2022, 10, 4590 RSC.
- K. J. Yang, H. Lee, R. Braveenth, S. J. Hwang, S. J. Kim and J. H. Kwon, Analysis of efficiency variations in ν-DABNA based thermally activated delayed fluorescence OLED devices, J. Ind. Eng. Chem., 2022, 108, 47 CrossRef CAS.
|
This journal is © the Partner Organisations 2023 |