DOI:
10.1039/C6RA19069C
(Communication)
RSC Adv., 2016,
6, 80718-80722
B(C6F5)3 catalysed 1,6-conjugate allylation of para-quinone methides: expedient access to allyl diarylmethanes†
Received
27th July 2016
, Accepted 19th August 2016
First published on 19th August 2016
Abstract
An effective method for the synthesis of unsymmetrical allyldiaryl methanes is portrayed. This protocol involves a Lewis acid catalysed 1,6-conjugate addition of allyltrimethyl silanes to para-quinone methides, which enabled us to synthesize a variety of unsymmetrical allyl diarylmethanes in good to excellent yields in a very short reaction period.
Allylation of carbon electrophiles is acknowledged to be one of the important methods in carbon–carbon bond forming transformations.1 Especially, the 1,2-allylation of carbonyl compounds and imines leading to homoallyl derivatives is very well explored, as many of these derivatives serve as valuable synthons in natural product synthesis.2 The 1,4-conjugate allylation of enones is another well studied transformation that could be utilized to access γ-allylated carbonyl compounds.3 However, the vinylogous allylation, i.e., the 1,6-conjugate allylation of dienones, is under explored till now though there are a few reports available for the 1,6-conjugate addition of other alkylmetal reagents (Scheme 1).4
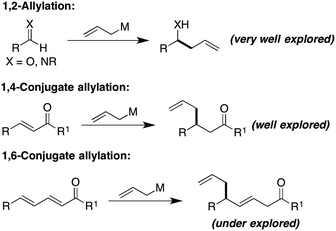 |
| Scheme 1 Various allylation reactions. | |
In fact, the regioselective 1,6-conjugate addition of metal-allyl reagents to α,β,γ,δ-unsaturated Michael acceptors is a challenging task, as this reaction would potentially generate 1,2- and 1,4-adducts as by-products along with 1,6-addition products. There are several parameters which affect the regioselectivity in 1,6-conjugate addition reaction, which include the choice of the catalyst, nature of the nucleophilic reagent, and the structure of the Michael acceptor.4b Due to these limiting parameters, the intermolecular 1,6-conjugate allylation of dienones is under explored. In fact, only one example each is reported for the intermolecular (with very poor regioselectivity)5a as well as intramolecular5b 1,6-conjugate allylation of dienone systems. While working on the 1,6-conjugate addition of various nucleophiles to p-quinone methides (p-QMs),6 we envisioned that it was possible to achieve the 1,6-conjugate allylation of p-QMs7 using an appropriate Lewis acid. The advantage of choosing p-QM as an acceptor is that this particular electrophile exists in the form of a 1,6-dipole,8 so it is feasible to accomplish this transformation in a regioselective manner. The 1,6-conjugate allylation of p-QMs would generate allyl diarylmethanes, which have shown significant applications in medicinal chemistry (Fig. 1).9
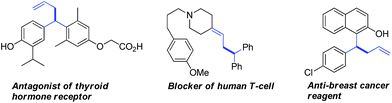 |
| Fig. 1 Some medicinally important allyl diarylmethanes. | |
Of course, there are several methods available in the literature for the synthesis of allyl diarylmethanes, which include Lewis acid catalysed electrophilic substitution of diarylmethanol and its derivatives,10 palladium11 or nickel12 catalysed allylic substitution reactions, metal catalysed direct arylation of homo-allyl arenes13 and other miscellaneous reactions.14 However, the synthesis of allyl diarylmethanes through a highly regioselective intermolecular vinylogous allylation of p-QMs with metal-allyl reagents is not reported so far. Hence, we have decided to investigate this challenging transformation in detail.
We began the optimisation studies using a readily available p-quinone methide (1) and allyltrimethylsilane (2), employing a wide range of Lewis acids under various reaction conditions. Our initial efforts toward the 1,6-allylation of 1 was discouraging as the reaction did not generate the expected product 3 when Lewis acids such as Fe(OAc)2, Fe(acac)2 and CuCl were used (entries 1–3). Considering the initial unsuccessful attempts, we assumed that this transformation may require a strong Lewis acid. So, we shifted our attention toward boron based strong Lewis acids such as B(C6F5)3. Although B(C6F5)3 has been widely utilized in frustrated Lewis pair chemistry,15 its catalytic property has also been explored in organic transformations16 including allylation.17 When 1 mol% B(C6F5)3 was used as a catalyst in CH2Cl2, 3 was isolated in 5% yield after 24 h (entry 4). Invigorated by this observation, the optimization study was extended with increased catalyst loading of B(C6F5)3. When 10 mol% of the B(C6F5)3 was used, the allylated product 3 was obtained in 98% isolated yield within 5 minutes at room temperature after quenching the reaction with TBAF (entry 5).18 The popular boron based Lewis acid, BF3·OEt2 also catalysed this transformation, but was found to be less effective when compared to B(C6F5)3 (entry 6). Though scandium triflate was also found to be equally competent, the reaction took a relatively longer period to complete (entry 7). Other Lewis acids such as AlCl3 and FeCl3 were found to be relatively inferior in catalysing this transformation when compared to B(C6F5)3 (entry 8 & 9). Surprisingly, no product was obtained when Yb(OTf)3 was used as a catalyst (entry 10). Further optimization studies were carried out using B(C6F5)3 as a catalyst in other solvents such as toluene and chloroform, but the yield of the product 3 was found to be inferior when compared to entry 5 (entries 11 & 12). Surprisingly, the reaction did not work in solvents such as Et2O and DMF (entries 13 & 14). The formation of a complex mixture was observed when the reaction was carried out in THF (entry 15). Notably, no product was observed when the reaction was carried out without any catalyst, which clearly indicates a Lewis acid catalyst is essential for this transformation (entry 16).
After finding an optimal condition for this transformation (entry 5, Table 1), we focused on evaluating the substrate scope of p-QMs toward allylation. In this regard, a range of p-QMs were prepared and subjected to 1,6-conjugate allylation under standard conditions, and the results are summarized in Table 2. In most of the cases, the reaction was completed within a few minutes. In general, this protocol worked well in cases of p-QMs derived from both electron-rich as well as electron-poor aromatic aldehydes, and the corresponding allyl diarylmethanes (3a–3d, 3o, 3u & 3v) were obtained in very good yields (80–98%). Haloarene substituted p-QMs also underwent 1,6-allylation to yield the allyl diarylmethanes (3f, 3g, 3k & 3l) in moderate yields (60–70%). In cases of p-QMs (1h & 1i) derived from 4-trifluoromethyl benzaldehyde and 4-trifluoromethoxy benzaldehyde, the respective products 3h and 3i were obtained in 65 and 85% yields, respectively. Under the optimal conditions, other allyl diarylmethanes (3m, 3n, 3p–3s) could be synthesized in moderate to good yields (62–89%) from their respective p-QM precursors. Unfortunately, the p-QM (1t), derived from thiophene-2-carboxaldehyde, resulted in the formation of complex mixture under the reaction conditions. On the other hand, the p-QMs derived from 2,6-diisopropyl phenol (1w) and 2,6-dimethyl phenol (1x) underwent 1,6-allylation and generated the allyl diarylmethanes 3w and 3x in 65 and 50% yields, respectively.
Table 1 Optimisation of reaction conditionsa

|
Entry |
Lewis acid (10 mol%) |
Solvent |
Time |
Yield of 3b [%] |
Reaction conditions: all reactions were carried out with 0.067 M of 1 in solvent at room temperature (25–28 °C).
Isolated yield.
1 mol% of catalyst was used.
35.6 mg from 32 mg of 1.
35.1 mg from 32 mg of 1. 1.5 equivalents of 2 with respect to 1 was found to be optimal. The reaction was quenched with 1.5 equivalents of TBAF after completion.
|
1 |
Fe(OAc)2 |
CH2Cl2 |
24 h |
0 |
2 |
Fe(acac)2 |
CH2Cl2 |
24 h |
0 |
3 |
CuCl |
CH2Cl2 |
24 h |
0 |
4c |
B(C6F5)3 |
CH2Cl2 |
24 h |
5 |
5 |
B(C6F5)3 |
CH2Cl2 |
5 min |
98d |
6 |
BF3·OEt2 |
CH2Cl2 |
5 min |
60 |
7 |
Sc(OTf)3 |
CH2Cl2 |
30 min |
97e |
8 |
AlCl3 |
CH2Cl2 |
1 h |
85 |
9 |
FeCl3 |
CH2Cl2 |
2 h |
80 |
10 |
Yb(OTf)3 |
CH2Cl2 |
12 h |
0 |
11 |
B(C6F5)3 |
PhMe |
1 h |
88 |
12 |
B(C6F5)3 |
CHCl3 |
40 min |
94 |
13 |
B(C6F5)3 |
Et2O |
24 h |
0 |
14 |
B(C6F5)3 |
DMF |
24 h |
0 |
15 |
B(C6F5)3 |
THF |
24 h |
Decomposed |
16 |
— |
CH2Cl2 |
24 h |
0 |
Table 2 Substrate scope with different p-QMsa

|
Reaction conditions: all reactions were carried out with 0.067 M of 1 in CH2Cl2.
CM = complex mixture. Yields reported are isolated yields.
|
|
To elaborate the substrate scope further, 1 was subjected to 1,6-conjugate addition reaction with various allyltrimethylsilane derivatives under optimized reaction conditions, and the results are summarized in Table 3. It is evident from Table 3 that this protocol worked well for all the allyltrimethylsilane derivatives tried. For instance, the reaction of 1 with allyltrimethylsilane 2a (X = 4-cumylphenyl, Y = H) provided the desired allyl diarylmethane 4a in 72% yield within 5 min. Other allyltrimethylsilane derivatives such as 2b (X = 4-alkynylphenyl, Y = H) and 2c (X = 4-biphenyl, Y = H) reacted with 1 and provided the products 4b and 4c in 85% and 77% yields, respectively. Under the standard conditions, cinnamyl trimethylsilane 2d (X = H, Y = Ph) gave the allylated product 4d in 79% isolated yield as an inseparable diastereomeric mixture (dr = 2
:
1). The allyl diarylmethane 4e was isolated in 71% yield when 1 was treated with allyltrimethylsilane 2e (X = 2-naphthyl, Y = H). Unfortunately, the reactivity of quinoline based allyltrimethylsilane 2f (X = 6-quinolinyl, Y = H) toward 1 was found to be lower as the expected product 4f was isolated only in 20% yield even after 2 days. On the other hand, the electron-poor aryl-substituted allyltrimethylsilane derivative 2g (X = 3-naphthyl-2-carboxylic ester, Y = H) produced the allyl diarylmethane 4g in 80% yield in 5 min. In the case of allylation of p-QM 1g with 2h (X = 4-tert-butylphenyl, Y = H), the desired product 4h was isolated in 72% yield in 6 h.
Table 3 Substrate scope with various allyl reagentsa
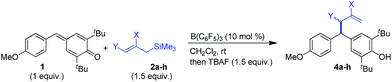
|
Reaction conditions: all reactions were carried out with 0.067 M of 1 in CH2Cl2. Yields reported are isolated yields.
|
|
To depict the application potential of this transformation, one of the allyl diarylmethanes was elaborated to a natural product like core (Scheme 2). In this experiment, the allyl diarylmethane 3d was subjected to ring closing metathesis reaction with 10 mol% of the second generation Grubbs catalyst and, as expected, the 8-membered ring ether 5 was obtained in 54% yield after 12 h (Scheme 2).
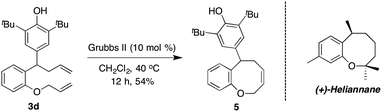 |
| Scheme 2 Synthesis of 8-membered ring ether. | |
Conclusions
In summary, we have shown the application of B(C6F5)3 as a Lewis acid catalyst for the synthesis of unsymmetrical allyl diarylmethanes through 1,6-conjugate allylation of p-quinone methides. By taking the advantage of the reactivity of p-quinone methides, we could achieve this transformation in a highly regioselective manner. This protocol worked generally well for all kinds of substituted p-QMs as well as allyltrimethylsilanes.
Acknowledgements
We gratefully acknowledge the IISER Mohali for the financial support and for providing infrastructure. SM thanks the UGC, New Delhi for a research fellowship. GK thanks the CSIR, New Delhi for a research fellowship. The NMR and HRMS facilities at IISER Mohali are gratefully acknowledged.
Notes and references
-
W. R. Roush, in Comprehensive Organic Synthesis, ed. B. M. Trost and I. Fleming, Pergamon Press, New York, 1991, vol. 2, pp. 1–53 Search PubMed.
- For reviews, see:
(a) Y. Yamamoto and N. Asao, Chem. Rev., 1993, 93, 2207 CrossRef CAS;
(b) D. G. Hall, Synlett, 2007, 1644 CrossRef CAS;
(c) M. Yus, J. C. González-Gómez and F. Foubelo, Chem. Rev., 2011, 111, 7774 CrossRef CAS;
(d) H.-X. Huo, J. R. Duvall, M.-Y. Huang and R. Hong, Org. Chem. Front., 2014, 1, 303 RSC and references cited therein.
- For selected examples, see:
(a) A. Hosomi, H. Kobayashi and H. Sakurai, Tetrahedron Lett., 1980, 21, 955 CrossRef CAS;
(b) T. A. Blumenkopf and C. H. Heathcock, J. Am. Chem. Soc., 1983, 105, 2354 CrossRef CAS;
(c) G. Majetich, A. M. Casares, D. Chapman and M. Behnke, Tetrahedron Lett., 1983, 24, 1909 CrossRef CAS;
(d) R. L. Danheiser and D. M. Fink, Tetrahedron Lett., 1985, 26, 2509 CrossRef CAS;
(e) H. Uno, J. Org. Chem., 1986, 51, 350 CrossRef CAS;
(f) J. W. Herndon, J. Am. Chem. Soc., 1987, 109, 3165 CrossRef CAS;
(g) G. Majetich, A. Casares, D. Chapman and M. Behnke, J. Org. Chem., 1986, 51, 1745 CrossRef CAS;
(h) J. D. Winkler and D. Subrahmanyam, Tetrahedron, 1992, 48, 7049 CrossRef CAS;
(i) P. H. Lee, D. Seomoon, S. Kim, K. Nagaiah, S. V. Damle and K. Lee, Synthesis, 2003, 2189 CrossRef CAS;
(j) C. W. Huh, G. K. Somal, C. E. Katz, H. Pei, Y. Zeng, J. T. Douglas and J. Aubé, J. Org. Chem., 2009, 74, 7618 CrossRef CAS;
(k) N. R. Ball-Jones, J. J. Badillo, N. T. Tran and A. K. Franz, Angew. Chem., Int. Ed., 2014, 53, 9462 CrossRef CAS;
(l) K. Okamoto, E. Tamura and K. Ohe, Angew. Chem., Int. Ed., 2014, 53, 10195 CrossRef CAS.
- For reviews, see:
(a) A. G. Csákÿ, G. de la Herrán and M. C. Murcia, Chem. Soc. Rev., 2010, 39, 4080 RSC;
(b) T. E. Schmid, S. Drissi-Amraoui, C. Crévisy, O. Baslé and M. Mauduit, Beilstein J. Org. Chem., 2015, 11, 2418 CrossRef CAS PubMed and references cited therein.
-
(a) A. Jellal and M. Santelli, Tetrahedron Lett., 1980, 21, 4487 CrossRef CAS;
(b) S. R. Angle and K. D. Turnbull, J. Am. Chem. Soc., 1989, 111, 1136 CrossRef CAS.
-
(a) V. Reddy and R. V. Anand, Org. Lett., 2015, 17, 3390 CrossRef CAS;
(b) B. T. Ramanjaneyulu, S. Mahesh and R. V. Anand, Org. Lett., 2015, 17, 3952 CrossRef CAS;
(c) P. Arde and R. V. Anand, Org. Biomol. Chem., 2016, 14, 5550 RSC;
(d) P. Goswami and R. V. Anand, ChemistrySelect, 2016, 1, 2556 CrossRef CAS.
- For recent examples, where p-QMs has been utilized as an electrophile, see:
(a) W.-D. Chu, L.-F. Zhang, X. Bao, X.-H. Zhao, C. Zeng, J.-Y. Du, G.-B. Zhang, F.-X. Wang, X.-Y. Ma and C.-A. Fan, Angew. Chem., Int. Ed., 2013, 52, 9229 CrossRef CAS;
(b) L. Caruana, F. Kniep, T. K. Johansen, P. H. Poulsen and K. A. Jørgensen, J. Am. Chem. Soc., 2014, 136, 15929 CrossRef CAS;
(c) K. Gai, X. Fang, X. Li, J. Xu, X. Wu, A. Lin and H. Yao, Chem. Commun., 2015, 51, 15831 RSC;
(d) A. López, A. Parra, C. Jarava-Barrera and M. Tortosa, Chem. Commun., 2015, 51, 17684 RSC;
(e) Z. Yuan, X. Fang, X. Li, J. Wu, H. Yao and A. Lin, J. Org. Chem., 2015, 80, 11123 CrossRef CAS;
(f) A. Parra and M. Tortosa, ChemCatChem, 2015, 7, 1524 CrossRef CAS;
(g) Y. Lou, P. Cao, T. Jia, Y. Zhang, M. Wang and J. Liao, Angew. Chem., Int. Ed., 2015, 54, 12134 CrossRef CAS;
(h) Z. Wang, Y. F. Wong and J. Sun, Angew. Chem., Int. Ed., 2015, 54, 13711 CrossRef CAS;
(i) C. Jarava-Barrera, A. Parra, A. Lopóz, F. Cruz-Acosta, D. Collado-Sanz, D. J. Cárdenas and M. Tortosa, ACS Catal., 2016, 6, 442 CrossRef CAS;
(j) F.-S. He, J.-H. Jin, Z.-T. Yang, X. Yu, J. S. Fossey and W.-P. Deng, ACS Catal., 2016, 6, 652 CrossRef CAS;
(k) K. Zhao, Y. Zhi, A. Wang and D. Enders, ACS Catal., 2016, 6, 657 CrossRef CAS;
(l) Y.-H. Deng, X.-Z. Zhang, K.-Y. Yu, X. Yan, J.-Y. Du, H. Huang and C.-A. Fan, Chem. Commun., 2016, 52, 4183 RSC;
(m) X.-Z. Zhang, J.-Y. Du, Y.-H. Deng, W.-D. Chu, X. Yan, K.-Y. Yu and C.-A. Fan, J. Org. Chem., 2016, 81, 2598 CrossRef CAS;
(n) Y. Shen, J. Qi, Z. Mao and S. Cui, Org. Lett., 2016, 18, 2722 CrossRef CAS;
(o) Y. F. Wong, Z. Wang and J. Sun, Org. Biomol. Chem., 2016, 14, 5751 RSC.
- L. Caruana, M. Fochi and L. Bernardi, Molecules, 2015, 20, 11733 CrossRef CAS.
-
(a) H. A. I. Yoshihara, J. W. Apriletti, J. D. Baxter and T. S. Scanlan, Bioorg. Med. Chem. Lett., 2001, 11, 2821 CrossRef CAS;
(b) L. E. Burgess, K. Koch, K. Cooper, M. S. Biggers, M. Ramchandani, J.-H. Smitrovich, E. J. Gilbert, M. J. Bruns, R. J. Mather, C. B. Donovan and D. C. Hanson, Bioorg. Med. Chem. Lett., 1997, 7, 1047 CrossRef CAS;
(c) B. Das, C. R. Reddy, J. Kashanna, S. K. Mamidyala and C. G. Kumar, Med. Chem. Res., 2012, 21, 3321 CrossRef CAS.
- For selected examples, see:
(a) M. Yasuda, T. Saito, M. Ueba and A. Baba, Angew. Chem., Int. Ed., 2004, 43, 1414 CrossRef CAS;
(b) S. K. De and R. A. Gibbs, Tetrahedron Lett., 2005, 46, 8345 CrossRef CAS;
(c) G. V. M. Sharma, K. L. Reddy, P. S. Lakshmi, R. Ravi and A. C. Kunwar, J. Org. Chem., 2006, 71, 3967 CrossRef CAS;
(d) T. Saito, Y. Nishimoto, M. Yasuda and A. Baba, J. Org. Chem., 2006, 71, 8516 CrossRef CAS;
(e) G. W. Kabalka, M.-L. Yao, S. Borella and L. K. Goins, Organometallics, 2007, 26, 4112 CrossRef CAS;
(f) J. Wang, Y. Masui and M. Onaka, Tetrahedron Lett., 2010, 51, 3300 CrossRef CAS;
(g) H. T. Dao, U. Schneider and S. Kobayashi, Chem. Commun., 2011, 47, 692 RSC;
(h) H.-L. Yue, W. Wei, M.-M. Li, Y.-R. Yang and J.-X. Ji, Adv. Synth. Catal., 2011, 353, 3139 CrossRef CAS;
(i) H. T. Dao, U. Schneider and S. Kobayashi, Chem.–Asian J., 2011, 6, 2522 CrossRef CAS;
(j) Y. Sawama, R. Goto, S. Nagata, Y. Shishido, Y. Monguchi and H. Sajiki, Chem.–Eur. J., 2014, 20, 2631 CrossRef CAS.
-
(a) B. M. Trost and D. A. Thaisrivongs, J. Am. Chem. Soc., 2009, 131, 12056 CrossRef CAS;
(b) J. Zhang, C. Stanciu, B. Wang, M. M. Hussain, C.-S. Da, P. J. Carroll, S. D. Dreher and P. J. Walsh, J. Am. Chem. Soc., 2011, 133, 20552 CrossRef CAS PubMed;
(c) S.-C. Sha, J. Zhang, P. J. Carroll and P. J. Walsh, J. Am. Chem. Soc., 2013, 135, 17602 CrossRef CAS.
- S.-C. Sha, H. Jiang, J. Mao, A. Bellomo, S. A. Jeong and P. J. Walsh, Angew. Chem., Int. Ed., 2016, 55, 1070 CrossRef CAS.
-
(a) G. Chen, N. Tokunaga and T. Hayashi, Org. Lett., 2005, 7, 2285 CrossRef CAS PubMed;
(b) G. Islas-González, J. Benet-Buchholz, M. A. Maestro, A. Riera and M. A. Pericàs, J. Org. Chem., 2006, 71, 1537 CrossRef;
(c) Y. Miyake, S. Uemura and Y. Nishibayashi, ChemCatChem, 2009, 1, 342 CrossRef CAS;
(d) R. Shintani, K. Takatsu, M. Takeda and T. Hayashi, Angew. Chem., Int. Ed., 2011, 50, 8656 CrossRef CAS;
(e) H.-Q. Do, E. R. R. Chandrashekar and G. C. Fu, J. Am. Chem. Soc., 2013, 135, 16288 CrossRef CAS.
-
(a) H. Mayr, T. Bug, M. F. Gotta, N. Hering, B. Irrgang, B. Janker, B. Kempf, R. Loos, A. R. Ofial, G. Remennikov and H. Schimmel, J. Am. Chem. Soc., 2001, 123, 9500 CrossRef CAS;
(b) I. Shiina, M. Suzuki and K. Yokoyama, Tetrahedron Lett., 2002, 43, 6395 CrossRef CAS;
(c) J. A. Wilkinson, S. B. Rossington, J. Leonard and N. Hussain, Tetrahedron Lett., 2004, 45, 1191 CrossRef CAS;
(d) J. A. Wilkinson, S. B. Rossington, S. Ducki, J. Leonard and N. Hussain, Tetrahedron, 2006, 62, 1833 CrossRef CAS;
(e) B. C. Ranu, S. Banerjee and L. Adak, Tetrahedron Lett., 2007, 48, 7374 CrossRef CAS;
(f) G.-Z. Wang, J. Jiang, X.-S. Bu, J.-J. Dai, J. Xu, Y. Fu and H.-J. Xu, Org. Lett., 2015, 17, 3682 CrossRef CAS;
(g) L. M. Gooch, S. B. Rossington and J. A. Wilkinson, Tetrahedron
Lett., 2015, 56, 4025 CrossRef CAS;
(h) H. Kawashima, N. Ogawa, R. Saeki and Y. Kobayashi, Chem. Commun., 2016, 52, 4918 RSC.
- For a recent review, see: D. W. Stephan and G. Erker, Angew. Chem., Int. Ed., 2015, 54, 6400 CrossRef CAS and references cited therein.
- For selected examples, see:
(a) D. J. Parks and W. E. Piers, J. Am. Chem. Soc., 1996, 118, 9440 CrossRef CAS;
(b) J. M. Blackwell, K. L. Foster, V. H. Beck and W. E. Piers, J. Org. Chem., 1999, 64, 4887 CrossRef CAS;
(c) S. Chandrasekhar, C. R. Reddy and G. Chandrashekar, Tetrahedron Lett., 2004, 45, 6481 CrossRef CAS;
(d) S. Chandrasekhar, Y. S. Rao and N. R. Reddy, Synlett, 2005, 1471 CrossRef CAS;
(e) P. Srihari, S. R. Yaragorla, D. Basu and S. Chandrasekhar, Synthesis, 2006, 2646 CrossRef CAS;
(f) S. Chandrasekhar, Y. S. Rao, L. Sreelakshmi, B. Mahipal and C. R. Reddy, Synthesis, 2008, 1737 CrossRef CAS;
(g) C. R. Reddy, G. Rajesh, S. V. Balaji and N. Chethan, Tetrahedron Lett., 2008, 49, 970 CrossRef CAS;
(h) G. Rajagopal and S. S. Kim, Tetrahedron, 2009, 65, 4351 CrossRef CAS;
(i) P. Thirupathi, L. N. Neupane and K.-H. Lee, Tetrahedron, 2011, 67, 7301 CrossRef CAS;
(j) B. V. S. Reddy, S. Ghanty, C. Kishore and B. Sridhar, Tetrahedron Lett., 2014, 55, 4298 CrossRef CAS;
(k) I. Chatterjee and M. Oestreich, Angew. Chem., Int. Ed., 2015, 54, 1965 CrossRef CAS;
(l) S. D. Guggilapu, S. K. Prajapti and B. N. Babu, Tetrahedron Lett., 2015, 56, 889 CrossRef CAS;
(m) V. Fasano, J. E. Radcliffe and M. J. Ingleson, ACS Catal., 2016, 6, 1793 CrossRef CAS.
-
(a) J. M. Blackwell, W. E. Piers and M. Parvez, Org. Lett., 2000, 2, 695 CrossRef CAS;
(b) M. Rubin and V. Gevorgyan, Org. Lett., 2001, 3, 2705 CrossRef CAS;
(c) J. M. Blackwell, W. E. Piers and R. McDonald, J. Am. Chem. Soc., 2002, 124, 1295 CrossRef CAS;
(d) T. Schwier, M. Rubin and V. Gevorgyan, Org. Lett., 2004, 6, 1999 CrossRef CAS;
(e) P. Thirupathi, L. N. Neupane and K.-H. Lee, Bull. Korean Chem. Soc., 2012, 33, 1275 CrossRef CAS.
- Since a mixture of phenol 3 and its corresponding TMS–ether were always observed, the reaction mixture was quenched with TBAF to deprotect the TMS–ether in all the cases.
Footnote |
† Electronic supplementary information (ESI) available: Experimental procedures and spectra of all new compounds. See DOI: 10.1039/c6ra19069c |
|
This journal is © The Royal Society of Chemistry 2016 |
Click here to see how this site uses Cookies. View our privacy policy here.