DOI:
10.1039/D0RA07219B
(Review Article)
RSC Adv., 2020,
10, 42084-42097
Traditional uses, chemical components and pharmacological activities of the genus Ganoderma P. Karst.: a review†
Received
24th August 2020
, Accepted 10th November 2020
First published on 18th November 2020
Abstract
In recent years, some natural products isolated from the fungi of the genus Ganoderma have been found to have anti-tumor, liver protection, anti-inflammatory, immune regulation, anti-oxidation, anti-viral, anti-hyperglycemic and anti-hyperlipidemic effects. This review summarizes the research progress of some promising natural products and their pharmacological activities. The triterpenoids, meroterpenoids, sesquiterpenoids, steroids, alkaloids and polysaccharides isolated from Ganoderma lucidum and other species of Ganoderma were reviewed, including their corresponding chemical structures and biological activities. In particular, the triterpenes, polysaccharides and meroterpenoids of Ganoderma show a wide range of biological activities. Among them, the hydroxyl groups on the C-3, C-24 and C-25 positions of the lanostane triterpenes compound were the necessary active groups for the anti-HIV-1 virus. Previous study showed that lanostane triterpenes can inhibit human immunodeficiency virus-1 protease with an IC50 value of 20–40 μM, which has potential anti-HIV-1 activity. Polysaccharides can promote the production of TNF α and IFN-γ by macrophages and spleen cells in mice, and further inhibit or kill tumor cells. Some meroterpenoids contain oxygen-containing heterocycles, and they have significant antioxidant activity. In addition, Ganoderma has been used as a medicine to treat diseases for more than 2000 years, and we also reviewed its traditional uses.
1. Introduction
With the increasing public awareness of drug safety, people are more inclined to choose medicinal natural products extracted from plants and fungi because of their obvious advantages of being less toxic and having less side effects.1 Biological natural products are secondary metabolites derived from organisms. These sources can be either complex mixtures extracted from raw materials or single compounds.2,3 Among the well-known medicinal fungi, Ganoderma lucidum (Curtis) P. Karst. and other species of Ganoderma showed greater advantages than other chemical drugs. Ganoderma originated from Greek meaning shining skin, ganos meaning brightness and derma meaning skin. It was named Ganoderma by Finnish mycologist Petter Adolf Karsten in 1881.4 With the development of molecular biology, the definition of Ganoderma species has changed. For example, G. lucidum, which is widely used in commercial cultivation in China, is now considered to be a new species of G. lingzhi.5 At present, there are 131 species of Ganoderma in the world.6–8 Among them, 20 kinds of G. lucidum, G. lingzhi Sheng H. Wu, Y. Cao & Y. C. Dai., G. sinense J. D. Zhao, L. W. Hsu & X. Q. Zhang. and others have been proved by modern studies to have medical value.9 Ganoderma is widely distributed in tropical and subtropical regions in Africa, America, Asia and Oceania, and it is also distributed in Europe (Fig. 1).10,11 As shown in Fig. 2, there are many species of Ganoderma in southern China, especially in the southern tropical and subtropical regions.6,12,13
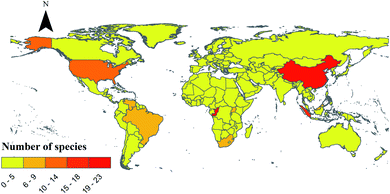 |
| Fig. 1 The distribution information of species of Ganoderma in world. | |
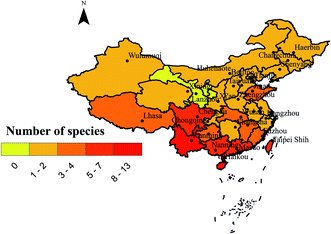 |
| Fig. 2 The distribution information of species of Ganoderma in China. | |
G. lingzhi and other species of Ganoderma are medicinal and edible tonic with a history of more than 2000 years in China. There are many traditional appellations of Ganoderma such as “Rui-cao”, “Lin-zhi-cao”, “Wan-nian-rong” or “Xian-cao” in China and “Reishi” in Japan due to their efficacy.5,14 It mainly regulates human immune function, prolongs longevity and exerts anti-tumor and neuroprotective functions.5,14 This is one of the reasons for the increasing demand for Ganoderma. The main medicinal part of Ganoderma is the fruiting body, however, its spore powder and mycelium can also be used as medicine. Currently, G. lucidum and G. sinense were considered legal crude drug medicine in the Chinese Pharmacopoeia (2015 edition), and they have the effect of preventing cancer, neurodegeneration and cardiovascular in the clinic.16 In the United States, Ganoderma appears in the US market as a dietary supplement, and it is cataloged in the Dietary Supplements and Herbal Medicines of United States Pharmacopoeia.17,18 According to recent studies, the pharmacological activity of this genus is similar to that described in ancient classic monographs.19,20 It was recorded as the top grade in the classical Chinese herbal literature Shennong's herbal classic (Shennong Ben Cao Jing) (Dong Han Dynasty, A.D. 25–220). This literature recorded that G. lingzhi has the effect of strengthening and restoring health.21 In other medical monographs, Xin Xiu Ben Cao records that G. lingzhi has the effect of “Buzhong Yiqi, Strengthen muscles and bones, Prolong life”. According to CNKI statistical analysis of patents related to China's “Lingzhi” industry from 1994 to 2019 shows that the number of “Lingzhi” patents had grown rapidly, reaching the highest peak of 1753 in 2016 (Fig. 3). There were 4338 preparation methods, accounting for 40% of the total. The patent focuses on practical applications and provides more valuable information for the development of “Lingzhi” industry. With the development of the industry, the spores and mycelium of Ganoderma are also used as new medicinal parts. For example, the spores of G. lucidum proved to have satisfactory anti-tumor and antioxidant activities.22,23 So far, major compounds have been isolated from the genus Ganoderma, including triterpenoids, polysaccharides, meroterpenoids, sesquiterpenoids, steroids and alkaloids.24,25 Although the use of Ganoderma lucidum in different national medical systems is different, triterpenes and polysaccharides are considered to be the main active ingredients.26,27
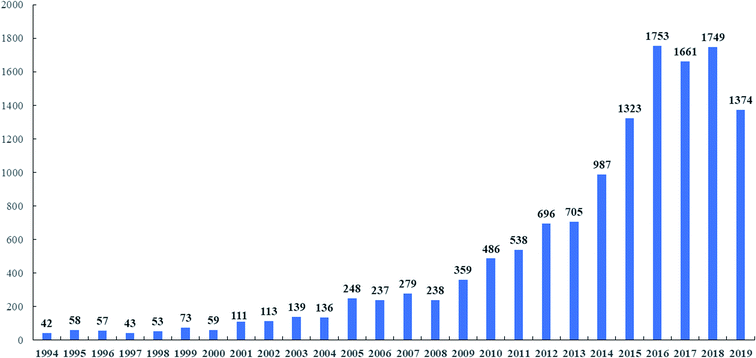 |
| Fig. 3 The number of patents of Ganoderma applied in China during 1994–2019. | |
G. lingzhi and other species of Ganoderma has been considered a precious Chinese medicine since ancient times. However, the quality of medicinal materials will be affected by species, cultivation methods and environmental factors, which is one of the important factors for extensive research on medicinal fungi. Summarizing various traditional uses, chemical components and pharmacological activity are important indicators for the quality assessment of medicinal fungi, which can provide better guidance for the rational use of Ganoderma. In this review, it mainly introduces the compounds found in the genus of Ganoderma fungi in the past 35 years, including the corresponding chemical structure, biological activity and a comprehensive compilation of its traditional uses, cultivation methods and historical culture. Therefore, this study aims to provide a certain theoretical basis for the quality control and rational use of Ganoderma by searching relevant literature and medicinal historical records, and to tap into potential development value.
2. Medicinal history and experience in ethnic minorities of Ganoderma
Ganoderma is a medicinal fungus and has been recorded in Shennong's herbal classic for more than 2000 years. The Shennong's herbal classic recorded “Chizhi”, “Qingzhi”, “Heizhi”, “Baizhi”, “Huangzhi” and “Zizhi”. These six species of Ganoderma have the effects of improving eyesight, strengthen muscles and bones, reinforcing kidney, soothe the nerves.28 Regular consumption of Ganoderma has been linked to the relaxed body, alleviate aging and prolong life. However, recent researches have shown that Ganoderma can be traced back to more than 6000 years ago. Five prehistoric samples of G. lucidum were found from three Neolithic sites in Zhejiang Province, China. The samples in the Tianluoshan site were 6817 ± 44 years old.29 It was speculated that Ganoderma was already used by witch doctors at that time. And along with the formation of early agriculture, people have continued exploration and exploitation of Ganoderma. During the Dongjin Dynasty, Ganoderma was ranked as panacea in an important Taoist work of Ge Hong's Bao Puzi (317 A.D.), and it was believed that the edible Ganoderma could extraordinary power and became immortal. Since then, Ganoderma has been endowed with a mysterious color, and the praise of Ganoderma reached a climax. Due to the limitations of world view and scientific level at that time, people's understanding and research on Ganoderma was limited and superstitious. Later, during the Ming Dynasty, some critical views emerged. Li Shizhen's Compendium of Materia Medica (1578 A.D.) believed that Ganoderma, which grows on decaying wood, was like a tumour on a human body. But ancients use it as panacea, which is too ridiculous. Li Shizhen denied the rumors that eating Ganoderma could live forever, and pointed out the functions of improving eyesight, strengthening muscles and bones, reinforcing kidney and soothing the nerves. Ganoderma was even exaggerated to have the function of resurrection and become immortal, in some ancient Chinese legends. However, since the Tang and Song Dynasties, Ganoderma is gradually recognized as a medicinal fungus by people's continuous understanding of herbs. Nowadays, G. lucidum and G. lingzhi are widely cultivated in China and neighboring countries, both of which can be used to soothe the nerves, relieve cough, treat insomnia and anorexia.30–32 Since ancient times, people's understanding of Ganoderma is a gradual process. From discovery to utilization and then to dialectical understanding, it is a piecemeal process of evolution, which also reflects the process of development and change of Chinese medicine. It shows how the ancients used the surrounding herbs and fungi to prevent and treat various diseases. The medicinal history of Ganoderma is summarized in Table 1.
Table 1 Medicinal history of Ganoderma
Date of origin |
Chinese ancient literatures |
Medicinal part |
Application |
About 206 B.C. to 220 A.D. |
Shennong's herbal classic |
Fruiting body |
Adjusting the function of spleen and stomach, amnesia, prolong life |
About 317 A.D. |
Bao puzi |
Fruiting body |
Live forever and become an immortal |
About 492 A.D. to 536 A.D. |
Shennong's herbal classic ji zhu |
Fruiting body |
Adjusting the function of spleen and stomach, amnesia, prolong life, improving eyesight, soothe the nerves |
659 A.D. |
Xin xiu ben cao |
Fruiting body |
Cure deafness, lubricate joints, tonifying middle-Jiao and qi, strengthen muscles and bones, prolong life |
682 A.D. |
Qian jin yi fang |
Fruiting body |
Improving eyesight, nourishing the liver, soothe the nerves |
992 A.D. |
Tai ping sheng hui fang |
Fruiting body |
Relax physically and psychologically |
1098 A.D. |
Zheng lei ben cao |
Fruiting body |
Cure deafness, lubricate joints, tonifying middle-jiao and qi, strengthen muscles and bones, prolong life |
1406 A.D. |
Pu ji fang |
Fruiting body |
Treatment of gingival bleeding |
1436 A.D. |
Dian nan ben cao |
Fruiting body |
Tonifying middle-jiao and qi, relieve cough, oppression in chest |
1505 A.D. |
Ben cao ping hui jing yao |
Fruiting body |
Strengthen muscles and bones, improving eyesight, soothe the nerves, nourishing the liver. |
1565 A.D. |
Materia medica companion |
Fruiting body |
Relax physically and psychologically, oppression in chest |
1578 A.D. |
Compendium of materia medica |
Fruiting body |
Tonifying middle-jiao and qi, strengthen muscles and bones, lubricate joints, protecting the kidney, |
1604 A.D. |
Wai ke qi xuan |
Fruiting body |
Carbuncle-abscess |
1647 A.D. |
Ben cao cheng ya ji |
Fruiting body |
Cure deafness, lubricate joints, strengthen muscles and bones, protecting the kidney, prolong life |
1765 A.D. |
Compendium of materia medica shi yi |
Fruiting body |
Relax physically and psychologically, rectal prolapse |
1769 A.D. |
Ben cao qiu zhen |
Fruiting body |
Purging heat |
Among the medical local knowledge of ethnic minority communities, Ganoderma has a unique value. China is a multi-ethnic country, and all ethnic groups people have accumulated their own drug experience in long-term life practice. Therefore, they formed rich and colorful national traditional medicine. Due to the influence of the environment, culture and medical theory about the medicinal methods of Ganoderma in ethnic minorities, different drug habits and experiences have been formed, such as Shui, Miao, Yi, Jingpo, Achang. In the Jingpo ethnic group, Ganoderma is called as Natmauchi, which is used to treat dizziness, insomnia, neurasthenia, hypertension, hypercholesterolemia and hepatitis. The Hani name for Ganoderma is Ahonglana, which is used for treating neurasthenia, hypertension, chronic bronchitis, silicosis in Hani medicine. Regarding the Chinese names and usage of Ganoderma in different ethnic minorities, Jia and Li summarized the use of this medicinal material by some China ethnic minority groups (Table 2).33 Some medicinal uses of Ganoderma (soothing the nerves, relieving cough and treating insomnia, etc.) were shared by some ethnic minorities and compliance were also record in Chinese Pharmacopoeia. Ganoderma has different uses in many ethnic groups. Because different geographical factors and ethnic cultural traditions influence differences in medical knowledge, they have different experiences in using the crude drug.
Table 2 Medicinal record of Ganoderma in ethnic minority
Ethnic minorities |
Name |
Chinese name |
Medicinal part |
Indications |
Record |
G. sinense |
Yi ( ) |
Zilingzhi |
 |
Fruiting body |
Testicular swelling, prostatitis |
Ai Lao Ben Cao |
Miao ( ) |
Zilingzhi |
 |
Fruiting body |
Cough, asthma, insomnia, dyspepsia |
Hunansheng Lanshanxian Zhong Cao Yao Zi Yuan Kao Cha Yu Yan Jiu |
Tibetan ( ) |
Guoxia mobu |
 |
Fruiting body |
Neurasthenia, chronic bronchitis, insomnia, hypertension, dizziness, hepatitis, trachitis, arthralgia |
Zhong Hua Zang Ben Cao |
![[thin space (1/6-em)]](https://www.rsc.org/images/entities/char_2009.gif) |
G. lucidum |
Aching ( ) |
Maone |
 |
Fruiting body |
Dizziness, insomnia, neurasthenia, hypertension, hypercholesterolemia, hepatitis |
De Hong Ming Zu Yao Lu |
Lisu ( ) |
Miqiguo |
 |
Fruiting body |
Stomachache, neurasthenia, chronic bronchitis, detoxification of mycotoxins |
Nu Jiang Zhong Cao Yao |
Hani ( ) |
Ahong lana |
 |
Fruiting body |
Dizziness, insomnia, neurasthenia, hypertension, chronic bronchitis, silicosis |
Yuan Jiang Ha Ni Zu Yao |
Tibetan ( ) |
Guoxia saibu |
 |
Fruiting body |
Neurasthenia, stomachache, chronic bronchitis, insomnia, hypertension, dizziness, hepatitis, trachitis, arthralgia |
Zhong Hua Zang Ben Cao |
Chaoxian ( ) |
Lingzhi/bulaocao |
 |
Fruiting body |
Asthenia, neuropathy, arteriosclerosis/dizziness, insomnia, neurasthenia, hypertension, hepatitis, chronic bronchitis, asthma, silicosis, rheumatic arthritis, rhinitis, asthenia, neuralgia, arteriosclerosis |
Chao yao zhi/Chao Xian Zu Ming Zu Yao Cai Lu |
Man ( ) |
Shabingacan |
 |
Fruiting body |
Coronary heart disease, trachitis, bronchial asthma |
Man Zu Chang Yong Yao Jian Jie |
Bai ( ) |
Lingzhi/lingzhi cao |
 |
Fruiting body |
Dizziness, insomnia, cough, neurasthenia, bronchitis, dyspepsia |
Da Li Zhong Yao Zi Yuan Zhi |
De’ ang ( ) |
Diguozhong |
 |
Fruiting body |
Dizziness, insomnia, neurasthenia, hypertension, hypercholesterolemia, hepatitis |
De Hong Ming Zu Yao Lu |
Jingpo ( ) |
Natmauchi |
Natmauchi |
Fruiting body |
Dizziness, insomnia, neurasthenia, hypertension, hypercholesterolemia, hepatitis |
De Hong Ming Zu Yao Lu |
Jinuo ( ) |
Mulumuka |
 |
Fruiting body |
Hypertension, hepatitis, rheumatic arthritis, body aches |
Ji Nuo Zu Yi Yao |
3. Cultivation methods and cultural connotations of Ganoderma
As a widely distributed fungus, Ganoderma has attracted extensive attention for its economic value. Ganoderma was developed into a variety of products such as powders, teas and dietary supplements, with global sales of these products estimated to exceed $2.5 billion,34 with sales growing at an annual rate of 18%.35,36 The wild fungus resources are abundant in Yunnan, China. Local residents often collect various wild fungus in the mountains and sell them in the mushroom market as part of their economic income. Ganoderma is also an important part of wild fungus resources, and it often appears in these markets (Fig S1†). Ganoderma appears in the modern market in the form of health care products or ornamentals. Due to the high medicinal and ornamental value of Ganoderma, people try to grow it. Before the 1990s, there were not any reports on artificial cultivation of Ganoderma in the world. Although some people tried to cultivate it, they failed because it could not form pileus. As shown in Fig. 4a and b, wild Ganoderma usually grows on the trunks, stumps, fallen and rotten wood of broad-leaved trees in diffused sunlight woodland with high humidity requirements. In the natural environment, they can easily form a complete fruit body, but cannot form a cap in the laboratory. The Fungal Research Laboratory of the Institute of Microbiology, Chinese Academy of Sciences firstly discovered in 1991 that high air relative humidity (85–95%) was the key factor for the formation of basidiocarps and the release of the spores of Ganoderma.37 The method and technology of large-scale artificial cultivation of Ganoderma were realized in 1992, and it was also the first example of the successful use of modern scientific methods to cultivate Ganoderma. The research institute popularized this method free of charge, which has made an important contribution to the rapid development of Ganoderma industry in China. Rani et al. found that the sawdust supplemented with dextrose medium improved the quality and biological efficiency of G. lucidum because dextrose provided a good carbon source for the Mycelial growth.38 Using wheat straw as substrate to form G. lucidum fruiting bodies shorter time, higher yield, higher biological efficiency and lower cost.39 Ganoderma is not only used as traditional Chinese medicine but also has a high ornamental value. Contemporarily, shapely Ganoderma is made into the potted landscape in the home for viewing. In traditional Chinese culture, Ganoderma symbolizes auspiciousness and fortune, and “ruyi” (a kind of ornament that represents auspicious) is made according to the shape of G. lucidum. The image of Ganoderma also appears in paintings, railings, furniture, wood carvings, carpets and handicrafts.40 It is precise because of the medicinal value and cultural symbols of Ganoderma that it is deeply loved by people, which makes the Ganoderma industry flourish.41
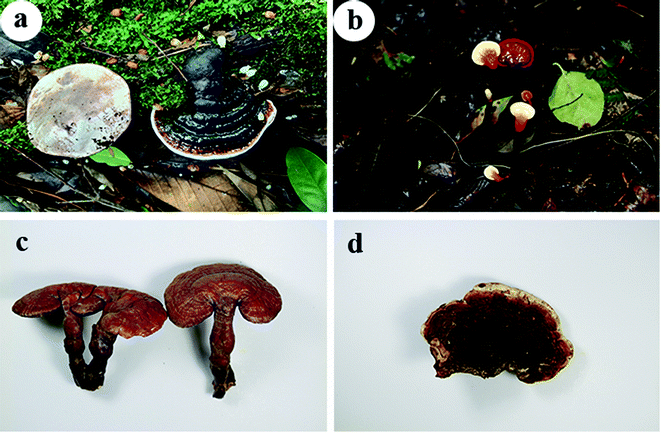 |
| Fig. 4 Fruiting body of Ganoderma. (a) G. cochlear; (b) G. kunmingense J.D. Zhao.; (c) G. lingzhi; (d) G. tropicum (Jungh.) Bres. | |
The successful tamed of Ganoderma had laid the foundation for the rapid development of the Ganoderma industry. In addition, it is beneficial to the human body and has ornamental value, making it a popular medicinal fungus. Ganoderma culture has a major and far-reaching impact on the cultural fields of philosophy, medicine, literature, and art in China. However, modern pharmacological research is mainly focused on the chemical constituent activity of Ganoderma. Many studies proved that Ganoderma has a variety of pharmacological activities, and chemical components are the premise for studying these pharmacological activities and their mechanisms.24,42,43 Therefore, it is necessary to review the chemical components and pharmacological activities of Ganoderma.
4. Chemical components of Ganoderma
In the past 35 years, chemical components have been separated from the fruiting bodies, sporophytes and mycelia of Ganoderma (Table S1†) including triterpenoids (1–561), meroterpenoids (562–792), sesquiterpenoids (793–815), steroids (816–875), alkaloids (876–906), polysaccharide, volatile oils and other types. These chemical structural formulas are listed (Fig. S2†). Clinical studies have been confirmed that triterpenoids and polysaccharides have a good effect. According to the Chinese Pharmacopoeia (2015 edition), the dry fruiting body of Ganoderma, polysaccharides shall not be less than 0.90%, and the triterpenoids not be less than 0.50%.30
4.1 Triterpenoids
Most triterpenoids are a class of terpenoids with a basic mother nucleus of 30 carbon atoms, which can be considered to polymerize from six isoprene units. Most of the triterpenoids that have been discovered so far are tetracyclic triterpenoids and pentacyclic triterpenoids, and a few are chain, monocyclic, bicyclic and tricyclic triterpenoids.44,45 The tetracyclic triterpenes of Ganoderma are highly oxidized lanosterane-typetriterpenoids derivatives. Depending on the structure and functional groups, it is further divided into ganoderic acid, methyl ganoderate, ganodermic acid, ganosporeri cacid, ganodsporelactone, ganolucidic acid, ganoderal, lucidenic acid, methyl lucidenate, lucidone, ganolactone, lucialdehyde. The double bonds on the ring are mostly located at C8-9, C9-11, C22-23, and C24-25. There is often a methyl group on C25, and the carbonyl group is mostly located at C11 and C23, C3, C7 and C15 are also often replaced by carbonyl or hydroxyl.46
Ganoderma contains a large number of triterpenoids, most of which have a bitter taste. More than half of triterpenoids are isolated from G. lucidum. Among the common species G. lucidum, G. Lingzhi, G. sinense and G. leucocontextum, 61 percent of 561 triterpenoids were isolated (as shown in Fig. 5). It is found in Ganoderma fruit bodies and spores, such as ganoderic acid A (10), ganoderic acid B (11), ganoderic acid G (17), ganoderic acid H (18).47 Pharmacological studies have confirmed that Ganoderma triterpenes (GTs) can inhibits tumor cells, lowers blood pressure, protects the liver, lowers cholesterol, and resists biological activities such as human immunodeficiency virus.42,48 The structural changes of the side chains of lanostane-type triterpenoids have significant effects on their hypoglycemic activity, especially the OH substituents at C-3 and the double bond parts at C-24 and C-25.49 In addition, ganoderic acid MK (92) and T (111) have the same parent ring structure, but their structures have different degrees of acetylation. 92 has 2 acetyl groups and 111 has 3 acetyl groups. These structural changes have varying degrees of influence on the pharmacological activities of triterpenes.50
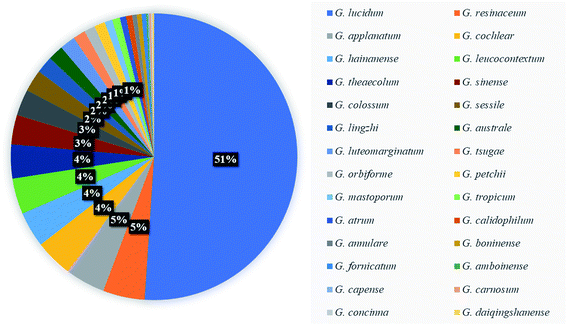 |
| Fig. 5 The percentage of triterpenes isolated from 28 species of Ganoderma. | |
4.2 Meroterpenoids
Meroterpenoid is a natural product widely distributed in plants, fungi and marine invertebrates. The biosynthesis of meroterpenoids originates from a mixed pathway of the shikimate pathway and the polyisoprene pathway.51 Meroterpenoids is often introduced into many heteroatoms in the process of biosynthesis, which makes the molecular structure form different polar centers, the molecular properties are more active and have strong reactivity. Ganoderma meroterpenoids are a class of meroterpenoids containing 1,2,4-trisubstituted phenyl and polyunsaturated terpenoid part.52 Some meroterpenoids contain oxygen-containing heterocycles, and these compounds were mainly concentrated in oxygen-containing five-membered heterocycles and oxygen-containing six-membered heterocycles. They generally have good biological activity, for example, the oxygen-containing five-membered heterocyclic compound fornicin E (590) had a stronger antioxidant effect than the positive control vitamin E.53
A large number of meroterpenoids are currently found in the fruiting bodies of Ganoderma. The meroterpenoids isolated from G. cochlear, G. applanatum, G. lucidum and G. theaecolum accounted for 74% of the 231 meroterpenoids (as shown in Fig. 6). The meroterpenoids isolated from G. cochlear have received widespread attention and proved to have a wide range of biological activities. The fungal-derived meroterpenoids have various biological activities such as antibacterial,54 antifibrotic, cytotoxic and NO production inhibitory.55,56 Wang et al. found that cochlearoid F-I, K (669–672, 674) have significant renoprotective activity to reduce oxidative stress through Nrf2 activation.57 At present, there are few studies on meroterpenoids, but they have great potential to be developed into a new framework for epigenetic agents.58 It can be used as one of the important research objects to evaluate the efficacy of Ganoderma in the future.
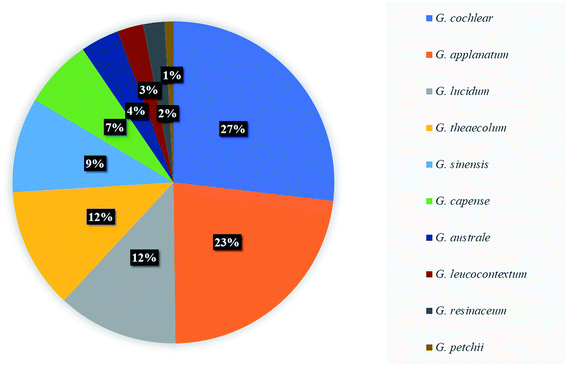 |
| Fig. 6 The percentage of triterpenes isolated from 10 meroterpenoids of Ganoderma. | |
4.3 Sesquiterpenoids
The basic carbon frame of sesquiterpenoids is composed of 15 carbon atoms, that is, 3 isoprene units. It is a volatile oil component, and the sesquiterpene oxygenated derivatives have strong aroma and biological activity. Farnesyl pyrophosphate (FPP) is a precursor of sesquiterpene biosynthesis. Pyrophosphate group is removed form cis, trans-FPP and trans and trans-FPP, and cyclize with their corresponding double bonds to form appropriate cyclic positive carbon ions. Finally, the Wagner–Meerwein rearrangement or the 1, 2-displacement (or elimination) of the methyl group results in the formation of sesquiterpenoids of various carbon framework types. The sesquiterpenoids can be classified into acyclic sesquiterpenoids, monocyclic sesquiterpenoids, bicyclic sesquiterpenoids and tricyclic sesquiterpenoids. Most of Ganoderma is a double-ringed sesquiterpenoid, and Zhen et al. isolated 11 sesquiterpenoids from the mycelium of G. lucidum, ganodermanol A–K (796–806).59 Among them, 796–803 are cadinane-type sesquiterpenoids, and 804–806 are eudesmane-type sesquiterpenoids. The sesquiterpene in Polyporus brumalis has antifungal activity,60 but there are few studies on the sesquiterpene of Ganoderma and it is worthy of further study.
4.4 Steroids and alkaloids
Steroids are found in Ganoderma fruit bodies and spores. The B/C rings of natural steroid compounds are trans, C/D rings are mostly trans, and A/B rings are cis and reverse. Therefore, the steroids can be divided into two types, the A/B ring cis-fused mode. That is the hydrogen atom on C5 and the horn methyl group on C10 extend to the front of the ring plane, on the same side, which is a β configuration. The A/B ring trans-fused mode (allo), that is, the hydrogen atom on C5 extends to the rear of the plane of the ring, and is not on the same side as the horn methyl group on C10, which is an α configuration. The C10, C13 and C17 side chains of steroids are mostly β configurations, C3 has hydroxyl group, and most of them are β configuration.61
Alkaloids are a class of nitrogen-containing organic compounds, most of which have complex cyclic structures. The nitrogen atoms are bound in the ring and are mostly alkaline, which can react with acids to form salts. Two novel pyrrole alkaloids ganoine and ganodine have been isolated from the mycelium of G. capense obtained by submerged fermentation.62 In the biosynthetic pathway of alkaloids, amino acids are generally considered to be their starting substances. Most of the skeleton of these amino acids remains in the alkaloids synthesized. At present, there are few alkaloids isolated from G. lucidum. The 32 alkaloids collected in this paper are listed in Table S1,† including pyrrolidine alkaloids, indoles alkaloids, quinolones alkaloids.
4.5 Polysaccharides
Polysaccharide is a polymer compound composed of ten or more monosaccharides which are polymerized by glycosidic bond, and is usually composed of several hundred to several thousands of monosaccharides and has a large molecular weight. GPs can be isolated from fruiting bodies, mycelia and spore powders, such as heteropolysaccharides (1β → 6,1β → 3 bindings of arabinogalactan), dextran (1β → 6,1β → 3 binding), peptide polysaccharides, mannose and galactose. Bao et al. found that the degree of substitution in the main chain and the length of the side chain may be important factors affecting the conformation and biological activity of G. lucidum polysaccharides (GLPs).63 In general, the molecular weight of GPs is generally greater than 1 × 104 to be biologically active. There is no significant difference in the activity between these GPs, but the disruption of the three-dimensional helical structure affects its activity.
GPs are divided into two types, α-type and β-type. The former has no biological activity, and the β-type structure of GPs has biological activity. The glycosidic bond is linked by β (1 → 3), (1 → 6), or β (1 → 4), (1 → 6). Biological activity is not only related to the primary structure but also largely affected by secondary and tertiary structures, in which intra- or extra-chain hydrogen bonding is an important factor in determining the level of biological activity.64
4.6 Others
There are also a large number of volatile oils such as alcohols, aldehydes and ketones, hydrocarbons and their derivatives, acids, esters, etc., which are found in Ganoderma fruit bodies and spore powders. Among them, the spore powder has many volatile components and little amount, most of them are aliphatic compounds.65–67 Al-Fatimi et al. reported for the first time the composition and biological activity of volatile oils from G. pfeifferi, indicating that volatile oils have strong free radical scavenging activity.43 Ganoderma contains various trace elements such as Al, B, Ca, Cu, Fe, K, Na, Mg, Mn, Pb, Zn, P, Ni, Co, Mo, Li, V, Sn, Ge, Ti, Se, Cr, containing a variety of human essential elements. This Ganoderma organic selenium is deserved a further study of pharmacological effect.68,69 Ganoderma also contains nucleosides, vitamins C and E, carotene, etc.70
5. Pharmacological activities
Ganoderma is a popular traditional Chinese medicine, and people pay attention to its true pharmacological effects. Several recent systematic studies have established the therapeutic potential of Ganoderma as antitumour, hepatoprotective, anti-inflammatory, immunomodulatory, antioxidant and antiviral.15,24,71
5.1 Antitumour activity
The anti-tumor activity of Ganoderma have gradually attracted the attention of researchers. The anti-tumor effect is related to promoting tumor cell apoptosis and enhancing the immune regulatory function of host cell.
Ganoderma extract (GEs) and Ganoderma spore oil (GSO) proved definite anti-tumor effects in the in vitro and in vivo studies. GEs and GSO inhibited the growth of human leukemia cells (K562 and HL60) and human gastric cancer cells (SGC-7901). Topoisomerases I and II may be the target of GEs and GSO actions.72 Triterpenoids plays an important role in the anti-tumor activity of Ganoderma. Wu et al. isolated two new triterpenoids from G. lucidum, lucidenic acid N (162) and methyl lucidenate F (173). It was confirmed that 162, lucidenic A (154) and ganoderic acid E (15) had obvious cytotoxicity to Hep G2, Hep G2, 2, 15 and P-388 tumor cells.73 The lanosteranes triterpenes isolated from G. lucidum showed good cytotoxic activity to gastric cancer cells HGC-27, cervical cancer cells HeLa, lung cancer cells A449, liver cancer cells SMMC-7721 by MTT method.74 The results showed that most of the triterpenoids inhibited the proliferation of four kinds of cancer cells. Among them, (5α,24E)-3β-acetoxyl-26-hydroxylanosta-8,24-dien-7-one (241) showed good cytotoxic activity against four kinds of cancer cells, and the IC50 value of inhibiting cervical cancer HeLa proliferation could reach 1.29 μM. At the same time, the study also showed that the C-26 and C-3 substituents of triterpenes in G. lucidum affected cytotoxic activity.75 For example, when the hydroxyl group or acetoxy group of C-3 was oxidized to ketone, the cytotoxicity was weakened. Nguyen et al. found that lucidadiol (265) and ganodermanondiol (318) have significant cytotoxic activity in anti-tumor in vitro tests, and ganoderic acid F (16) can control cancer by inhibiting angiogenesis.76 In addition, the alkaloid compound lucidimine B (904) with nitrogen-containing heterocycles and oxygen-containing heterocycles induces DNA fragmentation to arrest the cell cycle of human breast cancer MCF-7 in S phase, thereby reducing the mitochondrial membrane potential. It has the effect of inhibiting the proliferation of MCF-7 cells, with an EC50 value of 0.27 ± 0.02 μmol mL−1.77
GPs also plays an important role in anti-tumor activity. Huang et al. found that G. lucidum polysaccharide has no direct inhibitory effect on tumor cells cultured in vitro, but has an obvious inhibitory effect on tumor cells in vivo.78 It indicates inhibitory activity may be achieved by enhancing immune function to achieve antitumour activity. GLPs can promote the production of TNF α and IFN-γ by macrophages and spleen cells in mice, and further inhibit or kill tumor cells.79 Cao and Lin proved that GLPs have the effect of inhibited growth of Sarcoma 180 in tumor-bearing mice in vivo.80 GLPs still have high antitumor activity in immune-deficient mice. It is suggested that the anti-tumor activity of GPs involves other pathways, and anti-angiogenesis may be one of the mechanisms of antitumour effect of GPs. Triterpenoids and polysaccharides play an important role in antitumor activity, and the specific antitumour mechanism is still unclear, which needs further study.
5.2 Hepatoprotective activity
The liver of the human body has the function of degrading alcohol, but frequent and excessive drinking will cause alcoholic liver damage. Triterpenoids are the main components of the ethanol extract of G. lucidum, which can regulate the conversion of CYP2E1 to regulate the conversion of ethanol into acetaldehyde/acetate and up-regulation alcohol dehydrogenase 1 of tautomerism between aldehydes and alcohols or ketone in humans.81 Peng et al. found that fornicatin A, D, and F (392, 394, 398) can effectively lower the alanine aminotransferase (ALT) and aspartate aminotransferase (AST) levels in H2O2-treated HepG2 cells.82 The detection indexes of liver injury include ALT, AST and lactate dehydrogenase,83 which suggests that Ganoderma has liver protective activity in vivo. GPs have hepatoprotective activity against carbon tetrachloride-induced liver cell damage in carp by inhibiting lipid peroxidation, increasing antioxidant enzyme activity and inhibiting apoptosis.84
5.3 Anti-inflammatory activity and anti-microbial activity
GTs have anti-inflammatory activity against mouse macrophage line (RAW264.7) inflammation caused by lipopolysaccharide. Wu et al. isolated six triterpenoids from G. lucidum.85 Among them, the compound methyl lucidenate L (198) has a strong inhibitory effect on the NO-induced by LPS and attenuates the production of pro-inflammatory cytokines including IL-6 and IL-1β in RAW264.7 cells. At the same time, 198 effectively reduced the expression levels of iNOS, COX-2 and NF-κB, and inhibited the phosphorylation of IκBα and IKKβ in LPS-induced macrophages. Li et al. showed that triterpenoids isolated from G. lucidum have anti-inflammatory activity, which could significantly reduce the accumulation of cadmium in chicken liver, thereby reducing oxidative stress and inflammatory response.86 In the future, the total triterpenoids of G. lucidum are expected to become a functional food for preventing inflammation.
G. pfeifferi and other species of Ganoderma have been confirmed to have broad spectrum activity and bactericidal effects.87 The meroterpenoids compound ganomycins A and B (720 and 721) isolated from G. pfeifferi showed antibacterial activity against Gram-negative and Gram-positive bacteria. Especially for Gram-positive bacteria, such as Bacillus subtilis, Staphylococcus aureus and Micrococcus flavus, the inhibition zone is 15–25 mm.88 D-glucose is the main component of GLPs and plays an important role in inhibiting the growth of pathogenic bacteria.24 GLPs also show broad-spectrum activity and bactericidal effects, among which Micrococcus luteus was the most sensitive, with a minimal inhibitory concentration (MIC) value of 0.63–1.25 mg mL−1.89 The volatile oil of mushroom seldom had antibacterial activity, but the volatile oil extracted from G. Pfeifferi showed strong antibacterial activity against S. aureus (30 mm, MIC 0.3 mg mL−1) and B. subtilis (20 mm, MIC 0.6 mg mL−1). In addition, it had a significant inhibitory effect on Candida albicans (MIC 0.6 mg mL−1). The natural products of G. pfeifferi and other species of Ganoderma may have a synergistic effect, which means that the clinical effect usually depends on the combination of several ingredients.
5.4 Immunomodulatory activity
There have been many studies on the characteristics that Ganoderma can improve the immune ability, among which GPs have an important effect on immune regulation. The immune system is an important system to perform immune response and immune function, which is composed of immune organs, immune cells and immune molecules. GLPs can achieve immunomodulatory activity by activating a variety of immune-related cells, such as B lymphocytes, T lymphocytes, dendritic cells (DCs), macrophages and natural killer cells (NKs).90 The immune regulation mechanism of Ganoderma lucidum mainly depends on the following aspects.
5.4.1 Effect on macrophages. The macrophage is an important kind of immune cell in the body. The phagocytic ability of macrophages reflects the immune level of the body. Macrophages can directly destroy bacteria or cancer cells, or presenting them to T cells. The levels of interleukin-1β, tumor necrosis factor (TNF)-α and IL-6 in cultured macrophages treated with GLPs (100 mg mL−1) were significantly higher than those in the control group.91 These cytokines enhance the immune ability of the body and play the role of anti-inflammation and antitumour. Yu et al. studied the immunomodulatory effects of polysaccharides isolated from G. atrum on mouse macrophage cell line RAW264.7, and found that G. atrum polysaccharides can promote macrophage phagocytosis and increase NO production.92 In addition, GLPs have a protective effect on macrophage injury induced by reactive oxygen species.93
5.4.2 Effects on natural killer cells and dendritic cells. NKs are immune cells that can directly kill pathogens. It can be used as one of the indicators for judging the body's anti-tumor and anti-viral infections. The amount of it and the strength of its activity have a very close relationship with the body's ability to resist disease. Zhu et al. showed that the cytotoxic activities of NKs and lymphokine-activated killer (LAK) were enhanced after the intragastric administration of GLPs for 7–9 days.94 NKs can enhance the expression of the domain (CD86) on DCs, which plays an important role in tumor response.95,96DCs are a kind of leukocyte derived from bone marrow pluripotent hematopoietic stem cells. Wang et al. found that polysaccharide (PSG-1) isolated from G. atrum could stimulate the maturation of DCs in mice.97 DCs were induced to produce cytokines (IL-12p70, IL-10 and IL-6) to enhance immune regulation. Besides, GLPs combined with gold to form nanocomposites can efficiently induce (DC) activation in dendritic cells.98
5.4.3 Effects on T lymphocytes and B lymphocytes. Both T cells and B cells are derived from pluripotent stem cells of bone marrow. T cells play various roles in immunity, such as directly killing target cells, assisting or inhibiting B cells from producing antibodies and producing cytokines. In the presence of PSG-1, the release of IFN-γ in T cells is greatly promoted.91 PSG-1 has an immunomodulatory effect on splenic lymphocytes. Xiang et al. (Xiang et al., 2017). found that the polysaccharide PSG-1 extracted from G. atrum has the function of promoting the proliferation of T lymphocytes, and the content of IL-12, IL-2 and IFN-γ is increased. In addition, PSG-1 increased intracellular Ca2+ concentration, calcineurin activity and increased expression levels of p-ERK, p-JNK and p-p38 in the cell.99 Zhang et al. used successful chromatographic steps to extract a biologically active component from G. lucidum.100 The biologically active component-treated mouse spleen lymphocytes showed a significant increase, increasing the percentage of B cells by 3–4 times and activation.
5.5 Antioxidant activity
Free radicals can cause lipid peroxidation, which can damage cell membranes, damaged tissue cells and cell death lead to great harm to the human body. Studies have confirmed that Ganoderma is a very promising fungus with antioxidant activity. Seven new compounds isolated from G. cochlear.101 Among them, ganocochlearin A (627), ganocochlearin B (628), ganocochlearin C (629), and ganocochlearin D (630) have strong scavenging effects on DPPH radical, ABTS+ radical and hydroxyl radical. The volatile oil component isolated from G. pfeifferi and the alkaloid component isolated from G. lucidum also have a strong scavenging effect on DPPH radical, such as nitrogen-containing heterocyclic compounds lucidimines B and C (904 and 905).43,77
These nitrogen-containing heterocycles may play a crucial role. In chicken liver, GTs had resistance to cadmium-induced peroxidation damage. The contents of superoxide dismutase and glutathione peroxidase in chicken liver treated with GTs were significantly higher than those in the control group, and had better antioxidant activity.86 The meroterpenoids compounds fornicin E (590), ganocapensin A (711), B (712) and ganomycin F (723) isolated from G. capense can effectively scavenge DPPH free radicals with IC50 values of 6.00 ± 0.09, 7.79 ± 0.15, 8.20 ± 0.13, 7.44 ± 0.13 μg mL−1, respectively.53 In addition, Ganoderma polysaccharides reduce the oxidation of low-density lipoproteins and exhibited antioxidant properties by scavenging reactive oxygen species in mice.18
5.6 Antiviral activity
AIDS is a highly harmful infectious disease caused by HIV infection. There is currently no way around the world to cure it completely, and it can only be controlled to become AIDS by limiting and reducing the concentration of HIV. GTs are potent anti-HIV drugs that can effectively inhibit HIV-1 protease activity. Ganoderiol F (142), ganodermanontriol (322), ganolucidic acid A (38), ganolucidic acid B (39), ganoderic acid GS-2 (84), 15α-acetoxy-3α-hydroxylanosta-8,24-dien-26-oic acid (445), colossolactone V (297) and ganoderiol F (142) have anti-HIV-1 activity.102–105 The hydroxyl groups at positions C-3, C-24 and C-25 in lanostane triterpenoids are the necessary active groups for anti-HIV-1 virus.106 Among them, compounds 84 and 142 inhibit human immunodeficiency virus-1 protease with IC50 values of 20–40 μM.107 Ganoderic acid Y (113) isolated from G. lucidum inhibits virus replication by preventing virus from uncoating, with a maximum inhibition rate of 83.9% at 20 μg mL−1, thereby preventing enterovirus 71 infection.108 Besides, Ganoderma has certain inhibitory effects on the dengue virus109 and influenza virus,110 but the antiviral mechanism needs further study.
5.7 Anti-hyperglycemic and anti-hyperlipidemic
Ganoderma has the effect of improving pancreatic blood circulation, reducing blood glucose and improving the symptoms of diabetic patients, as well as reducing blood lipids and improving various symptoms of hyperlipidemia. Sarker et al. showed that G. lucidum had good anti-hyperglycemic and anti-hyperlipidemic activity on alloxan and glucocorticoid-induced diabetic rats.111 Petroleum ether extract and methanol extract of G. lucidum can significantly increase plasma insulin levels in rats and reduce fasting plasma glucose. It is speculated that G. lucidum extract enabled cells to continuously produce insulin, restores normal cholesterol and triglyceride levels in rats, and has the function of significantly lowering blood lipids. G. atrum polysaccharide PSG-1 has the effect of lowering blood sugar and blood lipids. In high-fat diet and streptozotocin-induced type 2 diabetic rats, PSG-1 can significantly reduce fasting blood glucose and total cholesterol.71 The structural changes of the side chain of lanostane triterpenoids have a more significant effect on the activity than the structural changes of the four-ring skeleton. The carboxyl group on the side chain is the key to identifying the inhibitory activity of α-glucosidase, and it has the effect of reducing blood sugar.112 Seven compounds 40, 41, 136, 138, 139, 142 and 265 showed stronger activity than the positive control acarbose, with IC50 values of 15.75, 29.30, 33.52, 19.51, 24.98, 28.04 and 10.99 μM, respectively.49 The OH substituent at C-3 and the double bond at C-24 and C-25 are necessary conditions to increase the inhibitory activity of α-glucosidase. In addition, the double bond portion of C-20 and C-22 on the side chain of ganoderic acid and the hydroxyl substituent on C-3 increase the inhibitory activity of α-glucosidase.113 Diabetes leads to a chronic complication – diabetes foot ulcer, which leads to difficulty in healing wounds.114 The water extract of G. lucidum was abundant in polysaccharides, which can increase the antioxidant capacity in vivo and promote wound healing in diabetic rats.115 Meroterpenes also showed strong inhibitory activity on α-glucosidase, but the mechanism of action needs further study.116,117 Hu et al. found that G. lucidum triterpenes might improve hyperlipidemia by regulating intestinal flora and promoting the growth of beneficial bacteria.118 Xu et al. compared the natural polysaccharides and degraded polysaccharides of G. lucidum found that the structure of ultrasonic degradation polysaccharides did not change, and the hypolipidemic activity of degrading polysaccharides was higher than that of natural polysaccharides.119 GLPs significantly decreased fasting plasma glucose and fasting serum insulin in diabetic mice. The mechanism of action of GLPs may be related to the reduction of hepatic glucose regulator enzyme mRNA levels through activation of AMPK, which improves insulin resistance.120
6. Summary and future perspectives
Ganoderma widely distributed in Africa, North America, Asia, and has a long history of medicinal use in Asia. Although there are many reports on the chemical composition and pharmacological activity of Ganoderma, according to the latest edition of the Chinese Pharmacopoeia (2015 Edition), the content of triterpenoids and polysaccharides is the main evaluation index. This has led to the current research on Ganoderma mainly focusing on the pharmacological effects of triterpenoids and polysaccharides, which is similar to the method of chemical drug research. This method ignores the integrity of Chinese medicine, which means a clinical efficacy usually depends on the combination of multiple components. However, the quality of Ganoderma is affected by a variety of factors, such as cultivated varieties, cultivation substrate, processing methods, storage time. Only through the two types of components can not accurately reflect the quality of Ganoderma. Therefore, it is imperative to establish a more scientific and systematic evaluation system for quality evaluation of Ganoderma drugs and health products. Nowadays, the Ganoderma industry has great potential because of its medicinal and cultural values. In the future, Ganoderma is expected to become natural medicine source for treating various diseases. In this paper, 906 chemical components of Ganoderma medicinal fungi were reviewed, which were divided into triterpenoids, polysaccharides, meroterpenoids, sesquiterpenoids, steroids, alkaloids and their biological activities and pharmacological effects were reviewed. Among them, the meroterpenoids compound has a variety of biological activities and is worthy of further study. This provides a basis for further research into its quality control, chemical components and pharmacological mechanisms.
Abbreviations
AMPK | Adenosine 5'-monophosphate (AMP)-activated protein kinase |
AIDS | Acquired immunodeficiency syndrome |
ALT | Alanine aminotransferase |
AST | Aspartate aminotransferase |
COX-2 | Cyclooxygenase-2 |
CYP2E1 | Cytochrome P450 2E1 |
DCs | Dendritic cells |
ERK | Extracellular regulated protein kinases |
FPP | Farnesyl pyrophosphate |
GEs | Ganoderma extracts |
GLPs | Ganoderma lucidum polysaccharides |
GPs | Ganoderma polysaccharides |
GSO | Ganoderma spores oil |
GTs | Ganoderma triterpenes |
HIV | Human immunodeficiency virus |
IC50 | Half inhibitory concentration |
IFN-γ | Interferon γ |
IKKβ | Inhibitor kappa B kinaseβ |
IL | Interleukin |
iNOS | Nitric oxide synthase |
I-κB | Nuclear factor kappa B inhibitor |
JNK | C-Jun amino terminal kinase |
LAK | Lymphokine-activated killer |
LPS | Lipopolysaccharide |
MTT | 3-(4,5)-Dimethylthiahiazo (-z-y1)-2,5-di-phenytetrazoliumromide |
MIC | Minimal inhibitory concentration |
NF-κB | Nuclear factor kappa B |
NKs | Natural killer cells |
NO | Nitric oxide |
TNF | Tumor necrosis factor |
Author contributions
Li Wang, Jie-qing Li, Ji Zhang, Zhi-min Li, Hong-gao Liu and Yuan-zhong Wang conceived the review. Li Wang, Jie-qing Li and Zhi-min Li collected literature and drafted manuscript. Ji Zhang, Hong-gao Liu and Yuan-zhong Wang helped in manuscript revision. All authors read and approved the final manuscript for publication.
Conflicts of interest
The authors declare that there is no conflict of interest regarding publication of this paper.
Acknowledgements
This work was supposed by National Natural Science Foundation of China (grant number: U1802231).
References
- W. Tan, J. Lu, M. Huang, Y. Li, M. Chen, G. Wu, J. Gong, Z. Zhong, Z. Xu, Y. Dang, J. Guo, X. Chen and Y. Wang, Chin. Med., 2011, 6, 27 CrossRef CAS.
- H. Lyu, H. Liu, N. P. Keller and W. Yin, Nat. Prod. Rep., 2020, 37, 6–16 RSC.
- L. Liu, F. R. Xu and Y. Z. Wang, J. Ethnopharmacol., 2020, 263, 112792 CrossRef CAS.
- P. KARSTEN, Rev. Mycol., 1881, 3, 16–19 Search PubMed.
- Y. Cao, S. H. Wu and Y. C. Dai, Fungal Divers., 2012, 56, 49–62 CrossRef.
- J. H. Xing, Beijing Forestry University, 2019, https://kns.cnki.net/kcms/detail/detail.aspx?dbcode=CDFD%26dbname=CDFDLAST2020%26filename=1019182997.nh%26v=wjDhBW5fk96GqbhDXbDG1MkqXpwym%25mmd2BU3RGmryu7PL1di98TzwxQgB0il0YwkU62V.
- C. Richter, K. Wittstein, P. M. Kirk and M. Stadler, Fungal Divers., 2014, 71, 1–15 Search PubMed.
- J. H. Xing, Y. F. Sun, Y. L. Han, B. K. Cui and Y. C. Dai, MycoKeys, 2018, 34, 93–108 CrossRef.
- Y. C. Dai, Y. Cao, L. W. Zhou and S. H. Wu, Mycosystema, 2013, 32, 947–952 Search PubMed.
- L. W. Zhou, K. K. Nakasone, H. H. Burdsall Jr, J. Ginns, J. Vlasák, O. Miettinen, V. Spirin, T. Niemelä, H. S. Yuan, S. H. He, B. K. Cui, J. H. Xing and Y. C. Dai, Mycol. Prog., 2016, 15, 771–790 CrossRef.
- Y. F. Sun, D. H. Costa-Rezende, J. H. Xing, J. L. Zhou, B. Zhang, T. B. Gibertoni, G. Gates, M. Glen, Y. C. Dai and B. K. Cui, Persoonia, 2020, 44, 206–239 CrossRef.
- X. L. Wu, Y. C. Dai and Y. H. Lin, J. Guizhou Sci., 2004, 22, 27–33 Search PubMed.
- J. H. Zhang, R. Yang and D. S. Rao, J. Southwest Univ., 2014, 36, 74–78 Search PubMed.
- F. M. Shi, Z. M. Ding, S. L. Chen and X. R. Tong, World J. Sci. Technol., 2012, 14, 1473–1480 Search PubMed.
- B. S. Sanodiya, G. S. Thakur, R. K. Baghel, G. B. K. S. Prasad and P. S. Bisen, Curr. Pharm. Biotechnol., 2009, 10, 717 CAS.
- C. Sharma, N. Bhardwaj, A. Sharma, H. S. Tuli, P. Batra, V. Beniwal, G. K. Gupta and A. K. Sharma, J. Herb. Med., 2019, 100268 CrossRef.
- D. T. Wu, Y. Deng, L. X. Chen, J. Zhao, A. Bzhelyansky and S. P. Li, Sci. Rep., 2017, 7, 1–10 CrossRef.
- R. R. M. Paterson, Phytochemistry, 2006, 67, 1985–2001 CrossRef CAS.
- S. Huang, J. Mao, K. Ding, Y. Zhou, X. Zeng, W. Yang, P. Wang, C. Zhao, J. Yao, P. Xia and G. Pei, Stem Cell Rep., 2017, 8, 84–94 CrossRef CAS.
- W. Tang, Y. Gao, G. Chen, H. Gao, X. Dai, J. Ye, E. Chan, M. Huang and S. Zhou, J. Med. Food, 2005, 8, 53 CrossRef.
- J. D. Zhao and X. Q. Zhang, Acta Mycol. Sin., 1992, 11, 55–62 Search PubMed.
- B. S. Min, N. Nakamura, H. Miyashiro, K. W. Bae and M. Hattori, Chem. Pharm. Bull., 1998, 46, 1607–1612 CrossRef CAS.
- S. A. Heleno, L. Barros, A. Martins, M. J. R. P. Queiroz, C. Santos-Buelga and I. C. F. R. Ferreira, Food Res. Int., 2012, 46, 135–140 CrossRef CAS.
- D. Cör, Ž. Knez and M. Knez Hrnčič, Molecules, 2018, 23, 649 CrossRef.
- S. Baby, A. J. Johnson and B. Govindan, Phytochemistry, 2015, 114, 66–101 CrossRef CAS.
- C. R. Soccol, L. Y. Bissoqui, C. Rodrigues, R. Rubel, S. R. B. R. Sella, F. Leifa, L. P. de Souza Vandenberghe and V. T. Soccol, Int. J. Med. Mushrooms, 2016, 18, 757 CrossRef.
- Y. H. Gao, S. F. Zhou, M. Huang and A. L. Xu, Int. J. Med. Mushrooms, 2003, 5, 12 CrossRef.
- C. Zhao, C. C. Zhang, Z. Xing, Z. Ahmad, J. S. Li and M. W. Chang, Int. J. Biol. Macromol., 2019, 121, 1160–1178 CrossRef CAS.
- Y. Yuan, Y. J. Wang, G. P. Sun, Y. R. Wang, L. J. Cao, Y. M. Shen, B. Yuan, D. Han and L. Q. Huang, Chin. Sci. Bull., 2018, 63, 1180–1188 CrossRef.
- Chinese Pharmacopoeia Commission, Pharmacopoeia of the People's Republic of China, Beijing, China Medical Science Press, 2015 Search PubMed.
- M. Siwulski, K. Sobieralski, I. Golak-Siwulska, S. Sokół and A. Sękara, Herba Pol., 2015, 61, 105–118 Search PubMed.
- Y. Dai, L. Zhou, T. Hattori, Y. Cao, J. A. Stalpers, L. Ryvarden, P. Buchanan, F. Oberwinkler, N. Hallenberg, P. Liu and S. Wu, Mycol. Prog., 2017, 16, 1051–1055 CrossRef.
- M. Jia and X. Li, Zhong Guo Min Zu Yao Zhi Yao, Beijing, China, The Medicine Science and Technology Press, edn 2005, 2005 Search PubMed.
- J. Q. Li, J. H. Zhang, H. M. Chen, X. D. Chen, J. Lan and C. Liu, PLoS One, 2013, 8, e72038 CrossRef CAS.
- S. Sudheer, W. K. Yeoh, S. Manickam and A. Ali, Postharvest Biol. Technol., 2016, 117, 81–88 CrossRef CAS.
- K. M. de Mattos-Shipley, K. L. Ford, F. Alberti, A. M. Banks, A. M. Bailey and G. D. Foster, Stud. Mycol., 2016, 85, 125–157 CrossRef CAS.
- Y. N. Yu and M. Z. Shen, presented in part at the The Third Congress of the Chinese Fungi Society and the sixth National Symposium on Mycology, Beijing China, 2003, http://kns.cnki.net/KCMS/detail/detail.aspx?FileName=ZGVL200309001001%26DbName=CPFD2003 Search PubMed.
- P. Rani, M. R. Lal, U. Maheshwari and S. Krishnan, Int. J. Med. Mushrooms, 2015, 17, 1171–1177 CrossRef CAS.
- J. L. Ćilerdžić, J. B. Vukojević, A. S. Klaus, Ž. S. Ivanović, J. D. Blagojević and M. M. Stajić, Acta Sci. Pol. Hortorum Cultus., 2018, 17, 13–22 CrossRef.
- K. Hapuarachchi, W. Elkhateeb, S. Karunarathna, C. Cheng, A. Bandara, P. Kakumyan, K. Hyde, G. Daba and T. Wen, Mycosphere, 2018, 9, 1025–1052 CrossRef.
- Z. B. Lin and B. X. Yang, Ganoderma and Health, Springer Singapore, 2019, DOI:10.1007/978-981-13-9867-4).
- G. S. Wu, J. J. Guo, J. L. Bao, X. W. Li, X. P. Chen, J. J. Lu and Y. T. Wang, Expert Opin. Invest. Drugs, 2013, 22, 981–992 CrossRef CAS.
- M. Al-Fatimi, M. Wurster and U. Lindequist, Medicines, 2016, 3, 10 CrossRef.
- J. L. Rios, Planta Med., 2011, 77, 681–691 CrossRef CAS.
- Y. H. Gao, G. Q. Wang, H. Y. Huang, H. Gao, X. S. Yao and D. Hu, Chinese J. Org. Chem., 2018, 38, 2335–2347 CrossRef CAS.
- Q. Xia, H. Z. Zhang, X. F. Sun, H. J. Zhao, L. F. Wu, D. Zhu, G. H. Yang, Y. Y. Shao, X. X. Zhang, X. Mao, L. Z. Zhang and G. M. She, Molecules, 2014, 19, 17478–17535 CrossRef.
- C. R. Zhang, S. P. Yang and J. M. Yue, Nat. Prod. Res., 2008, 22, 1137–1142 CrossRef CAS.
- H. S. Tan, X. Li, B. L. Gong and G. Li, Progress in Microbiology and Immunology, 2018, 46, 43–48 Search PubMed , https://kns.cnki.net/KCMS/detail/detail.aspx?FileName=WSMY201801012%26DbName=CJFQ2018.
- L. Ma, J. Yan, H. Lang, L. Jin, F. Qiu, Y. Wang, Z. Xi, W. Shan, Z. Zhan and Y. Ying, Phytochem. Lett., 2019, 29, 154–159 CrossRef CAS.
- R. M. Liu, Y. B. Li, X. F. Liang, H. Z. Liu, J. H. Xiao and J. J. Zhong, Chem.-Biol. Interact., 2015, 240, 134–144 CrossRef CAS.
- G. Zhang, Ocean University of China, 2011, http://kns.cnki.net/KCMS/detail/detail.aspx?FileName=1011231430.nh%26DbName=CDFD2012.
- X. R. Peng and M. H. Qiu, Nat. Prod. Bioprospect., 2018, 8, 137–149 CrossRef CAS.
- X. R. Peng, L. Li, X. Wang, G. L. Zhu, Z. R. Li and M. H. Qiu, Fitoterapia, 2016, 111, 18–23 CrossRef CAS.
- R. A. A. Mothana, R. Jansen, W. Jülich and U. Lindequist, J. Nat. Prod., 2000, 63, 416–418 CrossRef CAS.
- M. Wang, F. Wang, F. Xu, L. Ding, Q. Zhang, H. Li, F. Zhao, L. Wang, L. Zhu, L. Chen and F. Qiu, Bioorg. Med. Chem. Lett., 2016, 26, 3342–3345 CrossRef CAS.
- X. Niu, S. Li, H. Sun and C. Che, J. Nat. Prod., 2006, 69, 1364–1365 CrossRef CAS.
- X. L. Wang, Z. H. Wu, L. Di, F. J. Zhou, Y. M. Yan and Y. X. Cheng, Fitoterapia, 2019, 132, 88–93 CrossRef CAS.
- Q. Luo, Z. Wang, J. Luo, Z. Tu and Y. Cheng, RSC Adv., 2017, 7, 38037–38043 RSC.
- Z. Tan, J. L. Zhao, J. M. Liu, M. Zhang, R. D. Chen, K. B. Xie and J. G. Dai, Fitoterapia, 2017, 118, 73–79 CrossRef CAS.
- S. Y. Lee, M. Kim, S. H. Kim, C. Y. Hong, S. H. Ryu and I. G. Choi, Microbiol. Res., 2016, 182, 141–149 CrossRef CAS.
- H. Kuang, Chemistry of Chinese materia medica, China Press of Traditional Chinese Medicine, Beijing, 2003 Search PubMed.
- J. G. Yu, R. Y. Chen, Z. X. Yao, S. L. Yang and J. L. Ma, Acta Pharm. Sin. B, 1990, 25, 612–616 CAS.
- X. F. Bao, C. P. Liu, J. N. Fang and X. Y. Li, Carbohydr. Res., 2001, 332, 67–74 CrossRef CAS.
- Q. Ying, X. C. Dong and M. H. Qiu, J Yunnan Nationalities University, 2005, 15, 134–137 Search PubMed.
- Y. Zheng, Yanbian University, 2017, http://kns.cnki.net/KCMS/detail/detail.aspx?FileName=1017109872.nh%26DbName=CMFD2018.
- T. Q. Chen, J. Z. Wu, F. H. Mao, L. Y. Zhong and J. Z. Wu, Acta Edulis Fungi, 2018, 25, 47–52 Search PubMed.
- T. T. Li, P. P. Hua, Z. R. Huang, Y. Y. Pan, R. B. Jia, H. P. Chen and B. Liu, Farm Products Processing, 2017, 37–40 CAS.
- L. Zhao, G. H. Zhao, Z. D. Zhao, P. Chen, J. Y. Tong and X. S. Hu, J. Agric. Food Chem., 2004, 52, 3954–3959 CrossRef CAS.
- P. Rzymski, M. Mleczek, P. Niedzielski, M. Siwulski and M. Gąsecka, J. Food Sci., 2016, 81, C587–C592 CrossRef CAS.
- S. Q. Huang, South China University of Technology, 2010, http://kns.cnki.net/KCMS/detail/detail.aspx?FileName=2010226838.nh%26DbName=CDFD2010.
- K. X. Zhu, S. P. Nie, C. Li, S. Lin, M. M. Xing, W. J. Li, D. M. Gong and M. Y. Xie, Int. J. Biol. Macromol., 2013, 57, 142–150 CrossRef CAS.
- C. Chen, P. Li, Y. Li, G. Yao and J. H. Xu, Oncol. Lett., 2016, 12, 3571–3578 CrossRef CAS.
- T. S. Wu, L. S. Shi and S. C. Kuo, J. Nat. Prod., 2001, 64, 1121–1122 CrossRef CAS.
- H. G. Su, Chengdu University of Traditional Chinese Medicine, 2018, http://kns.cnki.net/KCMS/detail/detail.aspx?FileName=1018890211.nh%26DbName=CMFD2019.
- H. G. Su, Q. M. Zhou, L. Guo, Y. J. Huang, C. Peng and L. Xiong, Phytochemistry, 2018, 156, 89–95 CrossRef CAS.
- V. T. Nguyen, N. T. Tung, T. D. Cuong, T. M. Hung, J. A. Kim, M. H. Woo, J. S. Choi, J. H. Lee and B. S. Min, Phytochem. Lett., 2015, 12, 69–74 CrossRef CAS.
- Y. S. Chen and P. Lan, ACS Omega, 2018, 3, 3471–3481 CrossRef CAS.
- F. H. Huang, M. H. Shang, Y. T. Xiong, J. Zhang and L. Y. Zhang, Chin. J. Nat. Med., 2010, 3, 114–118 Search PubMed.
- Z. B. Lin, Basic Medical Sciences and Clinics, 2000, 20, 7–9 Search PubMed.
- Q. Z. Cao and Z. B. Lin, Acta Pharmacol. Sin., 2004, 25, 833–838 CAS.
- C. Zhao, J. L. Fan, Y. Y. Liu, W. L. Guo, H. Cao, J. B. Xiao, Y. Wang and B. Liu, Food Chem., 2019, 271, 148–156 CrossRef CAS.
- X. R. Peng, J. Q. Liu, C. F. Wang, X. Y. Li, Y. Shu, L. Zhou and M. H. Qiu, J. Nat. Prod., 2014, 77, 737–743 CrossRef CAS.
- G. Lippi, F. Schena, M. Montagnana, G. L. Salvagno, G. Banfi and G. C. Guidi, Eur. J. Intern. Med., 2011, 22, e36–e38 CrossRef CAS.
- Y. J. Liu, J. L. Du, L. P. Cao, R. Jia, Y. J. Shen, C. Y. Zhao, P. Xu and G. J. Yin, Int. Immunopharmacol., 2015, 25, 112–120 CrossRef CAS.
- Y. L. Wu, F. Han, S. S. Luan, R. Ai, P. Zhang, H. Li and L. X. Chen, J. Agric. Food Chem., 2019, 67, 5147–5158 CrossRef CAS.
- T. Q. Li, H. R. Yu, Y. Y. Song, R. L. Zhang and M. Ge, J. Trace Elem. Med. Biol., 2019, 52, 118–125 CrossRef CAS.
- A. Smania, F. D. Monache, E. D. F. A. Smania and R. S. Cuneo, Int. J. Med. Mushrooms, 1999, 1, 325–330 CrossRef.
- R. A. A. Mothana, R. Jansen, W. Jülich and U. Lindequist, J. Nat. Prod., 2000, 63, 416–418 CrossRef CAS.
- K. Skalicka-Woźniak, J. Szypowski, R. Łoś, M. Siwulski, K. Sobieralski, K. Głowniak and A. Malm, Acta Soc. Bot. Pol., 2012, 81, 17–21 CrossRef.
- Z. T. Xu, X. P. Chen, Z. F. Zhong, L. D. Chen and Y. T. Wang, Am. J. Chin. Med., 2012, 39, 15–27 CrossRef.
- S. Y. Wang, M. L. Hsu, H. C. Hsu, C. H. Tzeng, S. S. Lee, M. S. Shiao and C. K. Ho, Int. J. Cancer, 1997, 70, 699–705 CrossRef CAS.
- Q. Yu, S. P. Nie, W. J. Li, W. Y. Zheng, P. F. Yin, D. M. Gong and M. Y. Xie, Phytother. Res., 2013, 27, 186–191 CrossRef CAS.
- Y. H. You and Z. B. Lin, Acta Pharmacol. Sin., 2002, 23, 787–791 CAS.
- X. L. Zhu, A. F. Chen and Z. B. Lin, J. Ethnopharmacol., 2007, 111, 219–226 CrossRef CAS.
- F. Nouroz, F. Bibi, S. Noreen and N. Masood, Egypt. J. Med. Hum. Genet., 2016, 17, 149–154 CrossRef.
- O. Demaria, S. Cornen, M. Daëron, Y. Morel, R. Medzhitov and E. Vivier, Nature, 2019, 574, 45–56 CrossRef CAS.
- H. Wang, S. Q. Wang, Q. Yu, S. P. Nie, Q. D. Xiang, M. M. Zhao, S. Y. Liu and M. Y. Xie, Food Chem. Toxicol., 2017, 108, 478–485 CrossRef CAS.
- S. L. Zhang, G. B. Pang, C. Chen, J. Z. Qin, H. Yu, Y. M. Liu, X. H. Zhang, Z. T. Song, J. Zhao, F. J. Wang, Y. Y. Wang and L. W. Zhang, Carbohydr. Polym., 2019, 205, 192–202 CrossRef CAS.
- Q. D. Xiang, Q. Yu, H. Wang, M. M. Zhao, S. Y. Liu, S. P. Nie and M. Y. Xie, J. Agric. Food Chem., 2017, 65, 5306–5315 CrossRef CAS.
- J. S. Zhang, Q. J. Tang, M. Zimmerman-Kordmann, W. Reutter and H. Fan, Life Sci., 2002, 71, 623–638 CrossRef CAS.
- X. R. Peng, J. Q. Liu, C. F. Wang, Z. H. Han, Y. Shu, X. Y. Li, L. Zhou and M. H. Qiu, Food Chem., 2015, 171, 251–257 CrossRef CAS.
- S. El-Mekkawy, M. R. Meselhy, N. Nakamura, Y. Tezuka, M. Hattori, N. Kakiuchi, K. Shimotohno, T. Kawahata and T. Otake, Phytochemistry, 1998, 49, 1651–1657 CrossRef CAS.
- D. Kang, M. Mutakin and J. Levita, Int. J. Chem., 2015, 7, 62–68 CAS.
- Z. Tan, J. L. Zhao, J. M. Liu, M. Zhang, R. D. Chen, K. B. Xie, D. W. Chen and J. G. Dai, J. Asian Nat. Prod. Res., 2018, 20, 844–851 CrossRef CAS.
- R. S. El Dine, A. M. El Halawany, C. Ma and M. Hattori, J. Nat. Prod., 2008, 71, 1022–1026 CrossRef CAS.
- M. R. Liu, East China University of Science and Technology, 2012, http://kns.cnki.net/KCMS/detail/detail.aspx?FileName=1012516399.nh%26DbName=CDFD2013.
- N. Sato, Q. Zhang, C. Ma and M. Hattori, Chem. Pharm. Bull., 2009, 57, 1076–1080 CrossRef CAS.
- W. J. Zhang, J. Y. Tao, X. P. Yang, Z. L. Yang, L. Zhang, H. S. Liu, K. L. Wu and J. G. Wu, Biochem. Biophys. Res. Commun., 2014, 449, 307–312 CrossRef CAS.
- W. Z. Lim, P. G. Cheng, A. Y. Abdulrahman and T. C. Teoh, J. Biomol. Struct. Dyn., 2020, 38, 4273–4288 CrossRef CAS.
- Q. C. Zhu, Y. M. Amen, K. Ohnuki and K. Shimizu, J. Funct. Foods, 2017, 34, 224–228 CrossRef.
- M. M. R. Sarker, M. A. T. R. Zihad, M. Islam, M. Nahar, M. M. Islam, H. Imam, A. Ghosh, M. S. Mustapha and N. E. Ismail, Funct. Foods Health Dis., 2015, 5, 450–466 CrossRef CAS.
- Q. Q. Zhang, University of Science and Technology of China, 2020, http://kns.cnki.net/KCMS/detail/detail.aspx?FileName=1020092405.nh%26DbName=CMFDTEMP.
- S. Fatmawati, R. Kondo and K. Shimizu, Bioorg. Med. Chem. Lett., 2013, 23, 5900–5903 CrossRef CAS.
- N. Singh, D. G. Armstrong and B. A. Lipsky, JAMA, J. Am. Med. Assoc., 2005, 293, 217–228 CrossRef CAS.
- P. G. Cheng, C. W. Phan, V. Sabaratnam, N. Abdullah, M. A. Abdulla and U. R. Kuppusamy, J. Evidence-Based Complementary Altern. Med., 2013, 2013, 671252 Search PubMed.
- B. S. Chen, J. Tian, J. J. Zhang, K. Wang, L. Liu, B. Yang, L. Bao and H. W. Liu, Fitoterapia, 2017, 120, 6–16 CrossRef CAS.
- K. Wang, L. Bao, K. Ma, J. Zhang, B. Chen, J. Han, J. Ren, H. Luo and H. Liu, Eur. J. Med. Chem., 2017, 127, 1035–1046 CrossRef CAS.
- R. K. Hu, W. L. Guo, Z. R. Huang, L. Li, B. Liu and X. C. Lv, J. Funct. Foods, 2018, 46, 403–412 CrossRef CAS.
- Y. Xu, X. Zhang, X. H. Yan, J. L. Zhang, L. Y. Wang, H. Xue, G. C. Jiang, X. T. Ma and X. J. Liu, Int. J. Biol. Macromol., 2019, 135, 706–716 CrossRef CAS.
- C. Xiao, Q. P. Wu, J. M. Zhang, Y. Z. Xie, W. Cai and J. B. Tan, J. Ethnopharmacol., 2017, 196, 47–57 CrossRef CAS.
Footnote |
† Electronic supplementary information (ESI) available: Fig. S1: various mushrooms peddled in the market (Ganoderma is marked in red), Table. S1: the main chemical components of Ganoderma, Fig. S2: metabolites isolated from various Ganoderma species. See DOI: 10.1039/d0ra07219b |
|
This journal is © The Royal Society of Chemistry 2020 |