DOI:
10.1039/D0RA01907K
(Paper)
RSC Adv., 2020,
10, 13975-13983
Synthesis of 3-selanylbenzo[b]furans promoted by SelectFluor®†
Received
28th February 2020
, Accepted 30th March 2020
First published on 7th April 2020
Abstract
A simple and practical protocol for the synthesis of 3-selanyl-benzo[b]furans mediated by the SelectFluor® reagent was developed. This novel methodology provided a greener alternative to generate 3-substituted-benzo[b]furans via a metal-free procedure under mild conditions. The intramolecular cyclization reaction was carried out employing an electrophilic selenium species generated in situ through the reaction between SelectFluor® and organic diselenides. The formation of this electrophilic selenium species (RSe-F) was confirmed by heteronuclear NMR spectroscopy, and its reactivity was explored.
The benzo[b]furan scaffold is an important structural motif that is present in natural products and in synthetic compounds with therapeutic proprieties.1 Substituted benzo[b]furans have shown a broad range of biological activities,2 being found in a variety of pharmaceutical targets, such as Viibryd® and Ancoron® (Fig. 1).3 These drugs are used for treatment of depression and for cardiac arrhythmias, respectively. An efficient method to obtain substituted benzo[b]furans is the intramolecular cyclization reaction between 2-alkynylphenol or 2-alkynylanisole derivatives with different electrophilic species to generate a wide variety of 3-substituted-benzo[b]furans. This strategy is especially useful because of the atom-economic synthesis under mild conditions.4
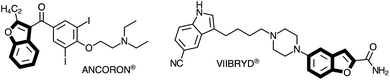 |
| Fig. 1 Substituted benzo[b]furans in commercial drugs. | |
Organoselenium compounds have attracted great interest due the large number of biological applications and their versatile reactivity.5 From a synthetic point of view, the ease cleavage of the Se–Se bond in diselenide compounds can generate species with different reactivity, as radical, electrophile, and nucleophile. This ample usefulness becomes the diselenides in key synthetic intermediates to introduce selenium moiety in organic compounds or to catalyse organic transformations.5,6
Despite the recent advances in the synthesis of 3-selanyl-benzo[b]furans, new electrophiles and reactional conditions were explored (Scheme 1).7–9,11–15 Initially, the establishing work by Larock and co-workers toward the synthesis of 3-selanyl-benzo[b]furans through the intramolecular cyclization of 2-(phenylethynyl)anisole with PhSeCl in CH2Cl2 at room temperature.7 In 2009, Zeni and co-workers demonstrated the synthesis of 3-selanyl-benzo[b]furans employing PhSeBr as an active electrophile.8 A pioneering protocol was reported by Zeni and co-workers, which employed FeCl3 (1.0 equiv.) and diorganyl diselenides in CH2Cl2 at 45 °C.9 Additionally, Lewis acids have been used as effective catalysts in Se–Se bonding cleavage to access functionalized selenium compounds.10 Afterward, alternative methods were developed, such as the synthesis of 3-selanyl-benzo[b]furans mediated by PdCl2/I2, I2/water, and CuI (1.5 equiv.).11–14 More recently, Liu and co-workers reported a radical cyclization reaction using selenium powder as selenium source and AgNO3 as catalyst in DMSO at 100 °C.15
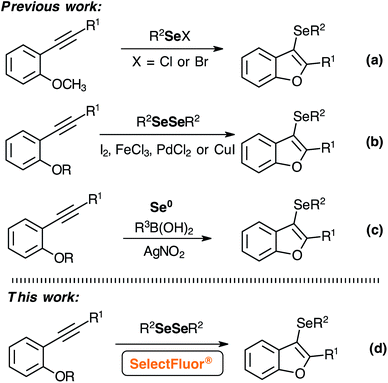 |
| Scheme 1 Methodologies to prepare 3-selanyl-benzo[b]furans. | |
Although, there are different methodologies to prepare 3-selanyl-benzo[b]furans and other functionalized selenium compounds through the reaction between diselenides compounds with oxidant reagents or Lewis acids, alternative electrophilic selenium species should be employed to avoid metals and/or toxic reagents.9–15 Furthermore, RSeCl and RSeBr,7,8 obtained from the reaction of diselenides with SO2Cl2 (or Cl2) and Br2 respectively, are commercially available and largely used as selenylating agent. However, these species present a low stability under moisture, and the high nucleophilicity of chloride and bromide leaving groups can lead to undesirable side reactions.
On the other hand, SelectFluor® is a versatile reagent used for different applications, such as fluorination reactions,16 C–H functionalization17 and organic function transfer.18 In addition, SelectFluor® has been used as an efficient method for intramolecular annulation reactions, due its higher reactivity.19 This ample application together with the desirable characteristics of the SelectFluor®, such as the higher stability, non-hydroscopic solid and hazard-free source of fluorine,20 promoted new possibilities to investigate fluorine chemistry. In 2004, Poleschner and Seppelt prepared PhSeF derivatives by the reaction between diorganyl diselenides and XeF2 in CH2Cl2 as a solvent at −40 °C.21 The products were characterized by low-temperature 19F and 77Se NMR, and it was the first confirmation of this type of electrophilic selenium compound. Although electrophilic selenium catalysis (ESC) with electrophilic fluoride reagents as oxidants has been demonstrated in the functionalization of alkenes,22 fewer knowledge about the reactivity of this selenium electrophilic species is available in the literature.23
Based on the development of new electrophilic selenium reagents,9–14,24 herein, we describe a metal-free synthesis of 3-selanyl-benzo[b]furans under mild conditions using this very reactive electrophilic selenium species (RSe-F), generated in situ at room temperature by the reaction of diorganyl diselenides with SelectFluor® reagent (Scheme 1). Moreover, the higher reactivity of RSe-F species could be explored for the insertion of selenium moiety in other building blocks because the environmentally friendly reactional condition, and the replacing chlorine and bromine by the non-nucleophilic fluorine counter ion, can partially circumvented some side reactions.
Results and discussion
We commenced optimization of the reaction conditions using 2-phenylalkynylanisole 1a and diphenyl diselenide 2a as standard substrates. The reaction conditions were investigated as outlined in Table 1.
Table 1 Optimization of the reaction conditions for the synthesis of 3-phenylselanyl-benzo[b]furan 3aa
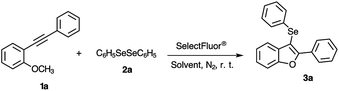
|
# |
2a (mmol) |
F® (mmol) |
Solvent (3.0 mL) |
Time (h) |
Yieldb (%) |
Reactions performed using 2-phenylalkynylanisole 1a (0.250 mmol) with diphenyl diselenide 2a and solvent under N2 atmosphere. Yields of isolated product. Reaction performed under air atmosphere. The reaction was performed using 1.0 mL of MeCN; N.R. = no reaction. |
1 |
0.125 |
0.250 |
MeCN |
2 |
97 |
2c |
0.125 |
0.250 |
MeCN |
2 |
67 |
3d |
0.125 |
0.250 |
MeCN |
2 |
70 |
4 |
0.125 |
0.125 |
MeCN |
2 |
90 |
5 |
0.150 |
0.125 |
MeCN |
2 |
92 |
6 |
0.125 |
0.062 |
MeCN |
2 |
40 |
7 |
0.125 |
0.125 |
DMSO |
24 |
N.R. |
8 |
0.125 |
0.250 |
DMF |
24 |
79 |
9 |
0.125 |
0.250 |
THF |
24 |
55 |
10 |
0.125 |
0.250 |
EtOH |
24 |
61 |
11 |
0.125 |
0.250 |
PEG-400 |
24 |
57 |
12 |
0.125 |
0.250 |
Glycerine |
24 |
45 |
Initially, the reaction was carried out using 0.250 mmol of 1a, 0.125 mmol of 2a and 0.250 mmol of SelectFluor® in MeCN at room temperature under N2 atmosphere. After 2.0 h, the yield of product 3a was 90% (Table 1, entry 1). When the reaction was performed under air atmosphere, product 3a was obtained in just 67% yield (Table 1, entry 2 vs. 1). The reduction of the amount of MeCN solvent was not beneficial for the reaction (Table 1, entry 3 vs. 1). When the amount of SelectFluor® was decreased to 0.125 mmol, the reaction performance was similar to entry 1 (Table 1). When the reaction was carried out with a small excess of diphenyl diselenide 2a, no effective improvement in the reaction condition was observed (Table 1, entry 5 vs. 1). These outcomes clearly demonstrate that the dependence on the amount of diorganyl diselenide with SelectFluor® reagent is not stoichiometric. A solvent evaluation was performed (Table 1, entries 7–12), with DMF demonstrating satisfactory yield but a longer reaction time (Table 1, entry 8 vs. 1). With DMSO solvent the formation of product 3a was not detected by TLC and GC (Table 1, entry 7). With THF, EtOH, PEG-400 and glycerine solvents the yields and reaction times were unsatisfactory (Table 1, entries 9–11). It is noticeable that this synthetic protocol is sensitive to water content, since the reaction using wet MeCN resulted in yield decrease and the formation of seleninic acid was observed by 77Se-{1H} NMR.
Next, we turned our attention to the reactional scope (Scheme 2), evaluating different 2-organylalkynylanisoles 1 with diverse diorganyl diselenides 2 under optimized reaction conditions (Table 1, entry 4).
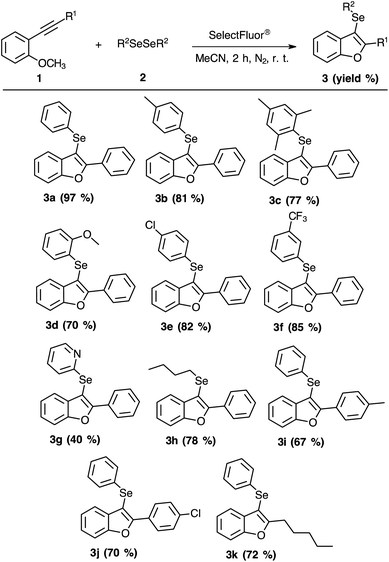 |
| Scheme 2 Substrate scope for the synthesis of 3-organylselanyl-benzo[b]furans 3a–k. | |
As summarized in Scheme 2, the substitution patterns on the phenyl moiety were satisfactory in all examples. The presence of methyl substituent, an electron-donating group, at para-position or ortho and para-positions afforded excellent yields (Scheme 2, 3b and 3c). The presence of methoxyl group at ortho-position also provided a satisfactory yield (Scheme 2, product 3d). When electron-withdrawing groups were evaluated, the conversion to the products 3e (para-chloride) and 3f (meta-CF3) yielded 82% and 85%, respectively (Scheme 2). When we examined the presence of pyridyl moiety on the aromatic diselenide, a moderate yield was obtained (Scheme 2, 3g). In an attempt to improve the performance of pyridyl moiety, a reaction to obtain the product 3g was carried out in 24 h at room temperature or employing heating (oil bath) of 50 °C for 2 h. However, both experimental changes were not affective to increase the yield of compound 3g. Furthermore, when the aromatic diselenides were switched for an aliphatic diselenide (Scheme 2, 3h) the performance of the reaction remained suitable.
Similarly, a substrate scope of the 2-organylalkynylanisoles 1 was also carried out (Scheme 2). These substrates were prepared by the Sonogashira coupling reaction between terminal alkynes with 2-bromoanisole.7 Once with the 2-organylalkynylanisoles 1 in hands, we started with evaluating the effect of electron-donating and electron-withdrawing groups. As can be seen in Scheme 2, para-methyl (3i) or para-chloride (3j) substituents gave a satisfactory efficiency, 67% and 70% of yield, respectively. When the aryl group was replaced by the alkyl group in the alkyne reagent the yield was similar (Scheme 2, 3k).
After determining the substrate scope regarding substituted diorganyl diselenides 2 and 2-organylalkynylanisoles 1, the reactivity of others diorganyl dichalcogenides (S and Te) were tested (Scheme 3). Notably, the reaction efficiency was reduced employing sulphur or tellurium elements. When diphenyl disulphide was used instead of diselenide, the yield has changed to 44% (Scheme 3, 3l). Considering the tellurium atom on the electrophilic intramolecular cyclization reaction, the yield was just 30% (Scheme 3, 3m). Based on the literature, it is possible to check the higher stability of the S–F bond,25 that could explain the lower effectivity of the 3-sulphuryl-benzo[b]furan 3l synthesis. However, there is not enough data describing the Te–F bonding stability and reactivity.21,26
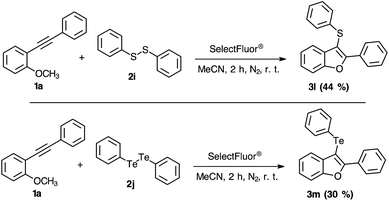 |
| Scheme 3 Chalcogenide scope for the synthesis of 3-chalcogenyl-benzo[b]furans 3l–m. | |
To further extend the practicability of this reaction, the reactivity of the 2-propagylanisole 1e was explored. Thus, the reaction was carried out using 0.250 mmol of 1e, 0.125 mmol of 2a and 0.250 mmol of SelectFluor® in MeCN at room temperature under N2 atmosphere. After 2.0 h, the yield was moderate (Scheme 4, 4a). To our surprise, the 1H and 13C NMR analyses have demonstrated altered standard spectra. These outcomes encouraged us to perform additional analyses to evaluate the product obtained. Consequently, HRMS (Fig. 2), infrared and NMR (ESI: 77Se-{1H}, COSY, HSQC and HMBC) analyses were carried out to check the structural assignment. All these findings support that a semi-pinacol rearrangement occurred, which an insertion of the C6H5Se group has occurred followed by a CH3 group shifting and providing the ketone 4a product (Scheme 4). Moreover, this reactivity of the 2-propagylanisole 1e is comparable with the literature, by isomerization27 or addition of others electrophilic species.28
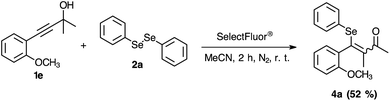 |
| Scheme 4 Reactivity of 2-propagylanisole 1e in the reaction with the electrophilic Se–F species. | |
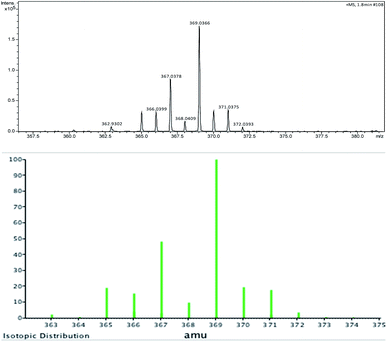 |
| Fig. 2 HRMS and isotopic distribution of product 4a (up: experimental analysis; and down: calculated MW + Na+ = 369.0369). | |
On evaluating the 2-organylalkynylanilines 1f and 1g to obtain 3-selanylindoles 5a–b, the results were less successful than the 3-selanyl-benzo[b]furans 3 (Scheme 5). This lower effectivity was established by a complex mixture of products in the TLC and 1H NMR analyses, obtaining the indoles 5a and 5b in just 25% and 20% of yield, respectively. The possible by-products can be suggested by the higher reactivity of the indoles with fluorine reagents.29 Additionally, a complete structural elucidation of the product 5b was performed to undoubtedly confirm the product 5b and obtain information about 15N NMR chemical shift profile (see ESI†).
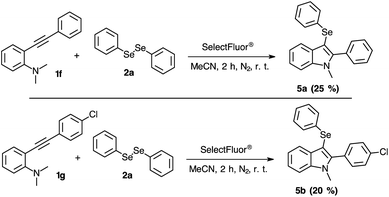 |
| Scheme 5 Substrate scope for the synthesis of 3-selanylindoles 5a–b. | |
The reactivity of the 2-phenylalkynylphenol 1d was also evaluated. According to Scheme 6, it is possible to observe that the phenol organic functional group is not sensitive to this higher reactive selenium electrophilic species, which the product 3a was obtained in 70% of yield.
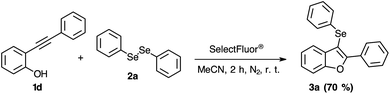 |
| Scheme 6 Synthesis of 3-phenylselanyl-benzo[b]furan 3a employing 2-phenylalkynylphenol 1d. | |
In order to gain insight into the mechanism, 1H, 13C-{1H}, 19F and 77Se-{1H} NMR analyses of a mixture between the diphenyl diselenide 2a and SelectFluor® were performed (ESI).30 For this purpose, 0.075 mmol of 2a and 0.150 mmol of SelectFluor® were solubilized in 1.0 mL of deuterated CD3CN and the NMR analyses were carried out at 25 °C. It was observed that a shielding has happened in the 1H and 13C-{1H} NMR chemical shifts of the SelectFluor® reagent, reflecting the leaving of fluorine atom. A deshielding was observed in the aromatic groups of the 2a compound around 1.0 ppm in the 1H NMR spectrum. Considering the 19F NMR experiment, the disappearance of 19F NMR chemical shift of the SelectFluor (ESI: 48.0 ppm) and the arising of a signal at −182.0 ppm, suggested the formation of a new fluorine compound. Finally, evaluating the 77Se-{1H} NMR analysis a new signal arisen at 1430.0 ppm, probably related to the Se–F bonding formation.21,31 When the 19F NMR experiment was performed employing 0.075 mmol of 2a with 0.075 mmol of SelectFluor®, similarly to the reaction conditions, a signal at −46.5 ppm was detected. But, the 77Se-{1H} NMR experiment did not show new peak, only regarding to the diphenyl diselenide compound.
Considering the NMR results21,31 and based on the literature,7–9 we have suggested a plausible mechanism for intramolecular cyclization reaction (Scheme 7). We have proposed two routes, based on the stoichiometric of the reagents, which both routes have the same intermediate detected by heteronuclear NMR spectroscopy. Initially, the formation of a higher reactive selenium electrophilic species A (77Se-{1H} = 1430.0 ppm and 19F = −182.0 ppm) is performed by the reaction between 1.0 equiv. of diselenide compound with 2.0 equiv. of SelectFluor® reagent (Scheme 7, route A). Next, the electrophilic selenium A reacts with the 2-organylalkynylanisole 1 to provides the intermediate B. Therefore, an intramolecular cyclization occurs by the oxygen attack on the activated triple bond and producing the 3-selanyl-benzo[b]furans. The CH3 or H leaving group of the methoxyl or phenol organic function, respectively, could be favoured by the nucleophilic attack of the nitrogen atom derived from the SelectFluor® residue. Considering the route B, a sub stoichiometric amount of SelectFluor® (1.0 equiv.) was employed. To explain the effectiveness of this experimental condition, the formation of an electrophilic selenium species C (19F = −46.5 ppm) was suggested, which the attack of 2-organylalkynylanisole 1 provides the intermediates A and B. Consequently, the intermediate B produces the desired product, and the intermediate A forms the species B, also resulting the 3-selanyl-benzo[b]furan compound.
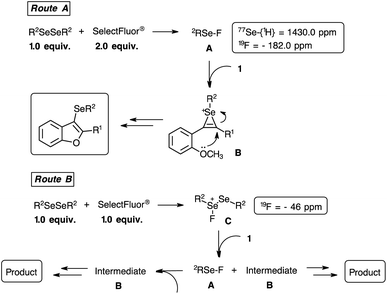 |
| Scheme 7 Plausible mechanism routes for the synthesis of 3-organylselanyl-benzo[b]furans 3. | |
Related to the cleavage of the Se–Se bonding for the formation of the species A, there are some studies that support an homolytic cleavage.32 Our tests to evaluate this type of cleavage have demonstrated a yield reduction. Under the standard reaction conditions, the reaction between 1a and 2a reagents was performed in the presence of 2,2,6,6-tetramethylpiperidin-1-oxyl (TEMPO) or benzene-1,4-diol, used as radical inhibitors (2.0 equiv.). The product yield in each test was 40% and 42%, respectively. Although, these findings support an homolytic cleavage of Se–Se, the higher reactivity of SelectFluor® against these radical inhibitors limit the conclusions of the reaction pathway. On the other hand, when we evaluate the mechanism involved in the ditelluride 2j, a different outcome was provided. At first, TEMPO as a radical inhibitor was added, and following our optimal experimental conditions, no product 3m was obtained. Considering the weak Te–Te bonding and the Te-oxidation facility,33 this result demonstrated that the reaction might occurred through a radical pathway.
Conclusions
In summary, a simple and efficient protocol for the synthesis of 3-selanyl-benzo[b]furans was developed. The methodology provided a greener alternative to generate 3-substituted-benzo[b]furans via a metal-free procedure under mild conditions. Additionally, we have confirmed the formation of the Se–F bonding, and its reactivity as an electrophilic selenium species was assessed. Compared with traditional methods, this methodology is a mild, metal-free and simple tool for the generation of selenium electrophile. These results demonstrate new possibilities of reaction application, since little information can be found in the literature about the reactivity of RSe-F electrophiles.
Experimental section
All commercial reagents and solvents were used without additional purification. TLC was performed on silica gel plates (Merck silica gel 60, F254), and the spots were visualized with UV light (254 and 365 nm) or by charring the plate dipped in vanillin solution. For the FTIR (Fourier Transform Infrared) in the attenuated total reflection mode (FTIR-ATR), the samples were submitted to KI and placed on the crystal surface of a FTIR Bruker Alpha-P spectrometer, obtained at the range of 4000–1500 cm−1. 1H, 13C, 19F, 77Se-{1H}, COSY, HSQC and HMBC NMR spectra were recorded using an NMR spectrometer with 400 MHz (Bruker, Avance III HD model). The probe was a 5 mm direct F-BBO (fluoride broadband observed). Spectra were recorded in deuterated chloroform at 298 K (25 °C). The reported data include chemical shift (δ), multiplicity, coupling constant (J) in hertz, and integrated intensity. The following abbreviations were used to explain multiplicities: s = singlet, d = doublet, dd = doublet of doublet, dt = doublet of triplet, t = triplet, td = triplet of doublet, q = quartet, quint = quintet, sex = sextet and m = multiplet. The 19F NMR chemical shifts are reported in ppm relative to PhCF3 (δ −63 ppm). The 77Se-{1H} NMR chemical shifts are reported in ppm relative to the internal standard C6H5SeSeC6H5 (δ 463 ppm). The NMR pulse sequence employed for 77Se-{1H} NMR experiments was gated decoupling. HRMS (m/z) were measured by ESI technique.
General protocol for the preparation of 2-alkynylanisoles 1 via Sonogashira coupling reaction5
To a two-necked round bottom flask containing PdCl2(PPh3)2 (1 mol%) and Et3N (3.0 mL) was added 2-bromoanisole (1.0 mmol) and terminal alkyne (1.5 mmol). The resulting solution was stirred for 5 minutes at room temperature. After this time, it was added CuI (2 mol%) and the reaction mixture was allowed to stir at 75 °C for 12 hours. After, the mixture was diluted with ethyl acetate (20.0 mL) and washed with saturated brine (2 × 20.0 mL). The organic phase was separated, dried over MgSO4 and concentrated under vacuum. The residue was purified by flash chromatography and eluted with hexane.
General protocol for the preparation of 3-selanylalkynylanisoles 3
The corresponding diorganyl diselenides (0.125 mmol) was added to a round bottom flask flowed by addition of dry CH3CN solvent (2.0 mL). To this solution, SelectFluor® (0.250 mmol) was added under N2 atmosphere. As the SelectFluor® was dissolved the reaction colour changed from yellow to red-brown. After 5 minutes from the addition of SelectFluor®, a solution of 2-alkynylanisole 1 in dry CH3CN solvent (0.250 mmol in 1.0 mL) was added to the reaction mixture. The reaction colour usually changed from red-brown to a clear brown. The reaction progress was monitored by TLC. After reaction completion ethyl acetate (20.0 mL) and distilled water (20.0 mL) were added and the aqueous layer was washed with ethyl acetate (2 × 20.0 mL). Then, the combined organic layers were washed with distilled water (10.0 mL) to remove any remaining organic solvent. After removal of the solvent, column chromatography was performed using silica gel and either hexane or a mixture of hexane and ethyl acetate depending on the polarity of the product 3. The characterization data of synthesized products 3 are described in the ESI.†
2-Phenyl-3-(phenylselanyl)benzo[b]furan 3a. Yield: 0.084 g (97%). White solid, mp = 40–41 °C. 1H NMR (CDCl3, TMS, 400 MHz) δ (ppm) = 8.12 (d, J = 7.2 Hz, 2H), 7.47–7.42 (m, 2H), 7.37–7.19 (m, 6H), 7.14 (dt, J = 7.4 and 1.0 Hz, 1H), 7.09–7.02 (m, 3H). 13C NMR (100 MHz, CDCl3) δ (ppm) = 157.2, 154.1, 131.8, 131.3, 130.1, 129.3, 129.2, 129.1, 128.4, 127.7, 126.2, 125.2, 123.4, 121.1, 111.1, 99.6. MS: m/z (rel intensity) 350 (M+ 32.4); 270 (100.0), 255 (7.8), 241 (17.0), 165 (28.5), 134 (8.4), 115 (4.5), 77 (5.4).
2-Phenyl-3-[(4-methylphenyl)selanyl]benzo[b]furan 3b. Yield: 0.073 g (81%). Yellow solid, mp = 77 °C.2 1H NMR (400 MHz, CDCl3) δ (ppm) = 8.13 (d, J = 7.2 Hz, 2H), 7.44 (t, J = 8.2 Hz, 2H), 7.38–7.34 (m, 2H), 7.33–7.24 (m, 1H), 7.22 (td, J = 7.7 Hz and 1.4 Hz, 1H), 7.15–7.11 (m, 3H), 6.88 (d, J = 8.0 Hz, 2H), 2.14 (s, 3H). 13C NMR (100 MHz, CDCl3) δ (ppm) = 156.9, 154.0, 136.1, 131.9, 130.1, 130.0, 129.5, 129.1, 128.4, 127.7, 127.4, 125.1, 123.3, 121.2, 111.1, 100.1, 20.9.
2-Phenyl-3-(mesitylselanyl)benzo[b]furan 3c. Yield: 0.072 g (77%). Yellow solid, mp = 145–148 °C. 1H NMR (400 MHz, CDCl3) δ (ppm) = 8.04 (d, J = 7.3 Hz, 2H), 7.40–7.34 (m, 3H), 7.29 (m, 1H), 7.10 (dt, J = 1.4 and 8.0 Hz, 1H), 6.93 (dt, J = 7.5 and 1.0 Hz, 1H), 6.85 (m, 1H), 6.76 (s, 2H), 2.30 (s, 6H), 2.13 (s, 3H). 13C NMR (100 MHz, CDCl3) δ (ppm) = 153.9, 153.4, 142.2, 138.1, 131.6, 130.6, 128.9, 128.6, 128.3, 127.4, 126.4, 124.6, 122.8, 120.6, 110.9, 102.3, 24.1, 20.8. MS: m/z (rel intensity) 392 (M+ 37.9); 311 (2.3); 281 (4.8); 194 (100.0); 165 (33.3); 139 (5.4); 119 (14.8); 91 (16.7); 77 (10.4); 44 (12.2). HRMS calculated for C23H20OSe 392.0675, found: 392.0676.
3-(2-Methoxyphenylselanyl)-2-(phenyl)benzo[b]furan 3d. Yield: 0.063 g (70%). Yellow oil. 1H NMR (400 MHz, CDCl3) δ (ppm) = 8.19 (d, J = 7.8 Hz, 2H), 7.57–7.52 (m, 2H), 7.43–7.31 (m, 4H), 7.24–7.21 (m, 1H), 7.10 (t, J = 7.7 Hz, 1H), 6.84 (d, J = 8.1 Hz, 1H), 6.78 (d, J = 7.7 Hz), 6.66 (t, J = 7.5 Hz, 1H), 3.93 (s, 3H). 13C NMR (100 MHz, CDCl3) δ (ppm) = 158.0, 156.4, 154.2, 132.1, 130.1, 129.2, 128.4, 128.1, 127.8, 126.8, 125.2, 123.4, 121.8, 121.3, 120.6, 111.1, 110.2, 97.8, 55.8. EM: m/z (rel intensity) 380 (M+ 85.4), 300 (100.0), 268 (13.8), 257 (17.0), 207 (14.5), 194 (35.7), 165 (64.5), 91 (14.4), 77 (25.6), 63 (12.2). HRMS calculated for C21H16O2Se + Na = 403.02125, found: 403.0220.
3-[(4-Chlorophenyl)selanyl]-2-phenylbenzo[b]furan 3e. Yield: 0.078 g (82%). Yellow solid, mp = 87 °C. 1H NMR (400 MHz, CDCl3) δ (ppm) = 8.08 (d, J = 7.0 Hz, 2H), 7.46 (d, J = 8.2 Hz, 1H), 7.40–7.27 (m, 4H), 7.24 (td, 7.7 Hz, 1.4 Hz, 1H), 7.16–7.09 (m, 3H), 7.03–7.00 (m, 2H). 13C NMR (100 MHz, CDCl3) δ (ppm) = 157.3, 154.0, 132.3, 131.5, 130.4, 129.9, 129.5, 129.4, 129.3, 128.4, 127.7, 125.3, 123.5, 120.9, 111.2, 99.3. MS: m/z (rel intensity) 384 (M+ 37.2); 304 (100.0), 268 (22.1), 241 (18.6), 165 (38.8), 134 (13.5), 63 (3.7).
2-Phenyl-3-[(3-trifluormethylphenyl)selanyl]benzo[b]furan 3f. Yield: 0.088 g (85%). Yellow solid, mp = 80 °C. 1H NMR (400 MHz, CDCl3) δ (ppm) = 8.17 (d, J = 7.3 Hz, 2H), 7.61–7.49 (m, 2H), 7.49–7.32 (m, 7H), 7.26–7.18 (m, 2H). 13C NMR (100 MHz, CDCl3) δ (ppm) = 157.7, 154.2, 132.7, 132.0, 131.5 (q, J = 34.4 Hz), 131.4, 129.8, 129.6, 129.5, 128.5, 127.8, 125.6 (q, J = 3.6 Hz), 125.5, 123.0 (q, J = 272.9), 123.6, 122.9 (q, J = 3.6 Hz), 120.9, 111.3, 98.7. MS: m/z (rel intensity) 418 (M+ 35.6), 338 (100.0), 309 (7.3), 268 (4.3), 241 (6.7), 165 (29.8), 139 (6.1), 115 (3.9).
2-Phenyl-3-(2-pyridylselanyl)benzo[b]furan 3g. Yield: 0.024 g (40%). Yellow solid, mp = 46 °C. 1H NMR (400 MHz, CDCl3) δ (ppm) = 8.45 (ddd, J = 4.8, 1.8, 0.8 Hz, 1H), 8.23–8.20 (m, 2H); 7.60–7.55 (m, 2H), 7.47–7.35 (m, 4H), 7.32–7.29 (m, 2H), 7.01 (ddd, J = 7.4, 4.9, 1 Hz, 1H), 6.9 (dt, J = 8.0, 1.0 Hz). 13C NMR (100 MHz, CDCl3) δ (ppm) = 157.6, 157.4, 154.2, 150.0, 136.9, 131.7, 129.9, 129.5, 128.5, 127.8, 125.4, 123.6, 122.9, 121.1, 120.5, 111.3, 99.0. HRMS calculated for C18H13NOSe + Na = 374.0059, found: 374.0055.
3-(Butylselanyl)-2-phenylbenzo[b]furan 3h. Yield: 0.066 g (78%). Yellow oil. 1H NMR (400 MHz, DMSO-d6) δ (ppm) = 8.19 (d, J = 7.8 Hz, 2H), 7.64 (d, J = 7.8 Hz, 2H), 7.52–7.55 (m, 2H), 7.47–7.43 (m, 1H), 7.40–7.32 (m, 1H), 2.80 (t, J = 7.2 Hz, 2H), 1.43 (quint, J = 7.1 Hz, 2H), 1.24 (sext, J = 7.3 Hz, 2H), 0.68 (t, J = 7.3 Hz, 2H). 13C NMR (100 MHz, DMSO-d6) δ (ppm) = 155.33, 153.26, 131.88, 129.76, 129.29, 128.65, 127.30, 125.44, 123.58, 120.73, 111.31, 99.90, 31.76, 27.65, 21.91, 13.22. EM: m/z (rel intensity) 330 (M+ 35.3), 274 (11.7), 245 (12.5), 194 (100.0), 165 (31.8), 41 (5.9).
3-(Phenylselanyl)-2-(4-methylphenyl)benzo[b]furan 3i. Yield: 0.060 g (67%). White solid, mp = 62 °C. 1H NMR (400 MHz, CDCl3) δ (ppm) = 8.10 (d, J = 8.2 Hz, 2H), 7.55–7.49 (m, 2H), 7.34–7.20 (m, 6H), 7.18–7.10 (m, 3H), 2.39 (s, 3H). 13C NMR (100 MHz, CDCl3) δ (ppm) = 157.5, 154.0, 139.4, 131.9, 131.5, 129.0, 127.6, 127.3, 126.1, 124.9, 123.3, 121.0, 111.0, 98.8, 21.4. MS: m/z (rel intensity) 364 (31), 363 (4), 284 (100), 269 (11), 255 (9), 241 (13), 178 (33), 165 (4), 15 (1), 77 (22).
2-(4-Chlorophenyl)-3-(phenylselanyl)benzo[b]furan 3j. Yield: 0.069 g (70%). Yellow solid, mp = 71–73 °C. 1H NMR (400 MHz, CDCl3) δ (ppm) = 8.07 (d, J = 8.7 Hz, 2H), 7.44–7.41 (m, 2H), 7.30 (d, J = 8.7 Hz, 2H), 7.23 (t, J = 7.7 Hz, 1H), 7.19–7.11 (m, 3H), 7.08–7.01 (m, 3H). 13C NMR (100 MHz, CDCl3) δ (ppm) = 155.9, 154.0, 135.2, 131.8, 131.1, 129.3, 129.2, 128.9, 128.7, 128.5, 126.4, 125.4, 123.5, 121.2, 111.2, 100.2. MS: m/z (rel intensity) 384 (M+ 51.34), 304 (100.0), 281 (7.35), 268 (25.64), 241 (19.30), 207 (17.43), 199 (16.27), 163 (25.01), 134 (7.98), 73 (10.12).
2-(nPentyl)-3-(phenylselanyl)benzo[b]furan 3k. Yield: 0.067 g (72%). Clear oil. 1H NMR (400 MHz, CDCl3) δ (ppm) = 7.47–7.42 (m, 2H), 7.28–7.22 (m, 4H), 7.18–7.10 (m, 3H), 2.97 (t, J = 7.5 Hz, 2H), 1.73 (p, J = 6.8 Hz, 1H), 1.32–1.29 (m, 4H), 0.84 (t, J = 6.5 Hz, 3H). 13C NMR (100 MHz, CDCl3) δ (ppm) = 163.8, 154.4, 131.8, 130.7, 129.13, 129.10, 126.0, 124.0, 123.0, 120.3, 110.9, 100.0, 31.3, 27.9, 27.3, 22.3, 13.9. EM: m/z (rel intensity) 344 (M+ 80.1), 287 (36.2), 264 (41.0), 207 (100.0), 178 (33.6), 131 (37.1), 115 (8.5), 102 (8.7), 77 (10.0). HRMS calculated for C19H20OSe + Na = 367.05764, found: 367.0549.
2-(4-Chlorophenyl)-3-(phenylselanyl)benzo[b]furan 3l. Yield: 0.035 g (44%). White solid, mp = 76 °C. 1H NMR (400 MHz, CDCl3) δ (ppm) = 8.23 (d, J = 7.4 Hz, 2H), 7.56 (d, J = 8.2 Hz, 1H), 7.49–7.37 (m, 5H), 7.36–7.28 (m, 2H), 7.24–7.19 (m, 4H). 13C NMR (100 MHz, CDCl3) δ (ppm) = 157.5, 153.9, 136.1, 130.8, 129.7, 129.4, 129.0, 128.5, 127.4, 126.5, 125.5, 125.2, 123.4, 120.4, 111.3, 104.6. MS: m/z (rel intensity) 302 (M+ 100.0), 273 (10.5), 241 (17.1), 225 (36.0), 197 (32.1), 165 (34.9), 152 (10.8), 139 (9.5), 105 (32.1), 77 (13.0), 51 (7.6).
2-(Phenyl)-3-(phenylteluryl)benzo[b]furan 3m. Yield: 0.031 g (30%). Orange solid, mp = 80 °C.2 1H NMR (400 MHz, CDCl3) δ (ppm) = 8.12 (d, J = 7.1 Hz, 2H), 7.54–7.53 (m, 2H), 7.47–7.44 (m, 4H), 7.42–7.38 (m, 1H), 7.34 (dt, J = 7.5 and 1.4 Hz, 1H), 7.25–7.23 (m, 1H), 7.18–7.08 (m, 3H). 13C NMR (100 MHz, CDCl3) δ (ppm) = 159.2, 154.6, 134.9, 134.3, 130.6, 129.5, 129.3, 128.5, 128.3, 127.2, 125.2, 123.3, 123.1, 114.8, 111.0, 82.6. EM: m/z (rel intensity) 400 (M+ 19.7), 270 (100.0), 241 (19.2), 207 (5.7), 193 (5.9), 165 (63.1), 139 (12.4), 115 (8.8), 77 (21.7), 51 (8.4).
4-(2-Methoxyphenyl)-3-methyl-4-(phenylselanyl)but-3-en-2-one 4a. Yield: 0.037 g (42%). Yellow oil. 1H NMR (400 MHz, CDCl3) δ (ppm) = 7.47 (dd, J = 7.7, 1.8 Hz, 1H11), 7.40 (ddd, J = 8.4 Hz, 7.4 Hz and 1.8 Hz, 1H, H9), 7.28–7.25 (m, 2H14,14′), 7.19–7.13 (m, 3H15,15′,16), 6.92–6.86 (m, 2H, H8,10), 3.77 (s, 3H, H12), 2.17 (s, 3H1), 2.04 (s, 3H, H4). 13C NMR (100 MHz, CDCl3) δ (ppm): 194.2 (C2), 158.4 (C7), 146.2 (C6), 133.3 (C9), 131.2 (C11), 131.1 (C14,14′), 128.9 (C15,15′), 127.6 (C5), 127 (C3), 126.3 (C16), 120.3 (C10), 111.4 (C8), 55.7 (C12), 24.9 (C1), 22.6 (C4). MS: m/z (rel intensity) 346 (19.6), 331 (3.4), 315 (2.9), 265 (3.7), 189 (21.9), 174 (17.8), 158 (20.1), 135 (100.0), 129 (14.1), 105 (3.5), 92 (11.2), 77 (34.4), 51 (4.9). IR (cm−1) 2936, 1636, 1479, 1248, 1016, 734. HRMS calculated for C18H19O2Se + Na = 369.0360, found: 369.0366.
1-Methyl-2-phenyl-3-(phenylselanyl)indole 5a. Yield: 0.023 g (25%). Pale yellow oil. 1H NMR (500 MHz, CDCl3) δ (ppm) = 7.66 (d, J = 6.5 Hz, 1H), 7.35–7.38 (m, 6H), 7.28–7.31 (m, 1H), 7.18–7.19 (m, 1H), 7.13–7.14 (m, 2H), 7.02–7.07 (m, 3H), 3.67 (s, 3H). 13C NMR (125 MHz, CDCl3) δ (ppm) = 145.8, 137.7, 134.6, 131.2, 130.7, 130.6, 128.8, 128.6, 128.3, 128.1, 125.2, 122.6, 120.8, 120.6, 109.7, 96.3, 31.7. EM: m/z (rel intensity) 363 (M+ 21.0), 283 (100.0), 267 (11.7), 204 (10.8), 190 (3.8), 165 (4.7), 141 (6.3), 77 (5.2).
2-(4-Chlorophenyl)-2-(phenylselanyl)-1-methylindol 5b. Yield: 0.020 g (20%). Yellow solid, mp = 103–104 °C. 1H NMR (400 MHz, CDCl3) δ (ppm) = 7.60 (d, J = 7.9 Hz, 1H, H5), 7.35–7.32 (m, 3H, H8,11,11′), 7.28–7.22 (m, 3H, H7,12,12′), 7.13 (ddd, J = 7.5 Hz, 7.0 Hz and 1.0 Hz, 1H, H6), 7.08–6.99 (m, 5H, H15–17), 3.65 (s, 3H, H1). 13C NMR (100 MHz, CDCl3) δ (ppm) = 144.4 (C2), 137.7 (C9), 134.8 (C13), 134.3 (C14), 132.0 (C11,11′), 130.5 (C4), 129.6 (C10), 128.9 (C12,12′), 128.4 (C15,15′,16), 128.3 (C15,15′,16), 125.3 (C17), 122.9 (C7), 121.0 (C6), 120.7 (C5), 109.7 (C8), 96.9 (C3), 31.7 (C1). 15N NMR (40 MHz, CDCl3) δ (ppm) = 130 ppm. EM: m/z (rel intensity) 397 (M+ 23.4), 317 (100.0), 281 (16.7), 267 (8.8), 204 (9.7), 141 (15.1), 77 (3.7).
Conflicts of interest
There are no conflicts to declare.
Acknowledgements
The authors thank FAPERGS, CNPq and FINEP for their financial support. This study was financed in part by the Coordenação de Aperfeiçoamento de Pessoal de Nível Superior – Brasil (CAPES) – Finance Code 001. The publication of this paper was partially supported by PRPPGI/UFPel and CAPES.
Notes and references
- F. M. Dean, The Total Synthesis of Natural Products, Wiley, New York, 1973, vol. 1 Search PubMed; P. Cagniant and D. Cagniant, Advances in Heterocyclic Chemistry, Academic Press, New York, 1975 Search PubMed; X.-F. Wu and Y. Li, Transition Metal-Catalysed Benzofuran Synthesis, Elsevier Inc., Amsterdam, 2017 Search PubMed; B. R. Thorat, R. Yamgar and K. More, Review: Synthesis and Biological Importance of Benzofuran Derivatives, Lambert Academic Pub., India, 2016 Search PubMed; B. A. Keay, J. H. Hopkins and P. W. Dibble, Furans and their Benzo Derivatives: Applications, Pergamon Press, Oxford, 2008, pp. 587–616 Search PubMed.
- J. D. Lambert, R. O. Meyers, B. N. Timmermann and R. T. Dorr, Cancer Lett., 2001, 171, 47 CrossRef CAS PubMed; R. Ikeda, T. Nagao, H. Okabe, Y. Nakano, H. Matsunaga, M. Katano and M. Mori, Chem. Pharm. Bull., 1998, 46, 871 CrossRef PubMed; C. Leung, J. L. Charlton and C. Cow, Can. J. Chem., 2000, 78, 553 CrossRef; S. Zacchino, G. Rodriguez, G. Pezzenati, G. Orellana, R. Enriz and S. M. Gonzalez, J. Nat. Prod., 1997, 60, 659 CrossRef PubMed; D. M. Schultz, J. A. Prescher, S. Kidd, D. Marona-Lewicka, D. E. Nichols and A. Monte, Bioorg. Med. Chem., 2008, 16, 6242 CrossRef PubMed; K. Takahata, H. Tsunekawa, C. Hirami, K. Yoshimi, S. Shimazu, F. Yoneda, H. Katsuki and A. Akaike, Neurosci. Res., 2006, 55, S117 Search PubMed; Y. Boukharsa, J. Taoufik, B. Meddah, Y. Cherrah, R. Y. Tiendrebeogo, A. Benomar, M. E. A. Faozi, A. Ibrahimi and M. Ansar, Med. Chem. Res., 2016, 25, 494 CrossRef; P. Yadav, P. Singh and A. K. Tewari, Bioorg. Med. Chem., 2014, 24, 2251 CrossRef PubMed; R. Romagnoli, C. Lopez-Cara, I. Castagliuolo, G. Basso, P. G. Baraldi, S. S. Ortega, S. Mitola, G. Viola, M. K. Salvador, A. Bancale, R. Ronca, F. Prencipe, E. Hamel, R. Bortolozzi and E. Porcu, J. Med. Chem., 2015, 58, 3209 CrossRef PubMed; L. F. Duncan, G. Wang, O. V. Ilyichova, M. J. Scanlon, B. Heras and B. M. Abbott, Molecules, 2019, 24, 3756 CrossRef PubMed.
- P. L. McCormack, Drugs, 2015, 75, 1915 CrossRef CAS PubMed; A. Rickli, S. Kopf, M. C. Hoener and M. E. B. Liechti, J. Pharmacol., 2015, 75, 1915 CrossRef PubMed.
- B. Godoi, R. F. Schumacher and G. Zeni, Chem. Rev., 2011, 111, 2937 CrossRef CAS PubMed.
- I. Beletskaya, Chem. Rev., 2011, 111, 1596 CrossRef CAS PubMed; A. M. Barcellos, A. L. Abenante and M. T. Sarro, Curr. Org. Chem., 2017, 21, 2044 CrossRef; G. Mugesh, W.-W. Du Mont and H. Sies, Chem. Rev., 2001, 101, 2125 CrossRef PubMed; J. J. Comasseto and R. A. Gariani, Tetrahedron, 2009, 65, 8447 CrossRef; C. W. Nogueira and J. B. T. Rocha, Arch. Toxicol., 2011, 85, 1313 CrossRef PubMed; G. Perin, E. J. Lenardão, R. G. Jacob and R. B. Panatieri, Chem. Rev., 2009, 109, 1277 CrossRef PubMed; A. J. Mukeherjee, S. S. Zade, H. B. Singh and R. B. Sunoj, Chem. Rev., 2010, 110, 4357 CrossRef PubMed; E. J. Lenardão, C. Santi and L. Sancineto, New Frontiers in Organoselenium Compounds, Springer, Switzerland, 2018 Search PubMed; H. Cao, Y. Yang, X. Chen, J. Liu, C. Chen, S. Yuan and L. Yu, Chin. Chem. Lett., 2020 DOI:10.1016/j.cclet.2020.01.027; T. A. C. Goulart, J. A. G. Kazmirski, D. F. Back and G. Zeni, J. Org. Chem., 2019, 84, 14113 CrossRef PubMed; T. Prochnow, A. Maroneze, D. F. Back and G. Zeni, J. Org. Chem., 2019, 84, 2891 CrossRef PubMed; C. Chen, Y. Cao, X. Wu, Y. Cai, J. Liu, L. Xu, K. Ding and L. Yu, Chin. Chem. Lett., 2020 DOI:10.1016/j.cclet.2019.12.019.
- T. Wirth, Organoselenium Chemistry. Synthesis and Reactions, Wiely-VCH Verlag & Co. KGaA, Weiheim, 2012, p. 462 CrossRef CAS; D. M. Freudendahl, S. A. Shahzad and T. Wirth, Eur. J. Org. Chem., 2009, 1649 CrossRef CAS; C. Santi, Organoselenium Chemistry: Between Synthesis and Biochemistry, Bentham Science, Sharjah, e-book, 2014, DOI:10.2174/97816080583891140101; A. D. Sonawane, Y. Kubota and M. Koketsu, J. Org. Chem., 2019, 84, 8602 CrossRef PubMed; G. Perin, P. C. Nobre, D. H. Mailahn, M. S. Silva, T. Barcellos, R. G. Jacob, E. J. Lenardão, C. Santi and J. A. Roehrs, Synthesis, 2019, 51, 2293–2304 CrossRef.
- D. Yue, T. Yao and R. C. Larock, J. Org. Chem., 2005, 70, 10292 CrossRef CAS PubMed.
- F. Manarin, J. A. Roehrs, R. M. Gay, R. Brandão, P. H. Menezes, C. W. Nogueira and G. Zeni, J. Org. Chem., 2009, 74, 2153 CrossRef CAS PubMed.
- R. M. Gay, F. Manarin, C. C. Shneider, D. A. Barancelli, M. D. Costa and G. Zeni, J. Org. Chem., 2010, 75, 5701 CrossRef CAS PubMed.
- L. Yu, J. Meng, L. Xia and R. Guo, J. Org. Chem., 2009, 74, 5087 CrossRef CAS PubMed; L. Yu, L. Ren, R. Guo and T. Chen, Synth. Commun., 2011, 41, 1958 CrossRef; L. Yu, L. Ren, R. Yi, Y. Wu, T. Chen and R. Guo, J. Organomet. Chem., 2011, 696, 2228 CrossRef.
- H.-A. Du, X.-G. Zhang, R.-Y. Tang and J.-H. Li, J. Org. Chem., 2009, 74, 7844 CrossRef CAS PubMed.
- M. Xu, X.-H. Zhang and P. Zhong, Tetrahedron Lett., 2011, 52, 6800 CrossRef CAS.
- J.-S. Han, Y.-L. Shao, X.-H. Zhang and P. Zhong, Phosphorus, Sulfur Silicon Relat. Elem., 2013, 188, 1599 CrossRef CAS.
- J. C. Kazmierczak, A. M. S. Recchi, F. Gritzenco, E. B. Balbom, T. Barcellos, A. Sperança and B. Godoi, Eur. J. Org. Chem., 2017, 6382 CrossRef CAS.
- C. An, C.-Y. Li, X.-B. Huang, W.-X. Gao, Y.-B. Zhou, M.-C. Liu and H.-Y. Wu, Org. Lett., 2019, 21, 6710 CrossRef CAS PubMed.
- J. Bi, Z. Zhang, Q. Liu and G. Zhang, Green Chem., 2012, 14, 1159 RSC; J. W. Kee, H. Shao, C. W. Kee, Y. Lu, H. S. Soo and C. H. Tan, Catal. Sci. Technol., 2017, 7, 848 RSC; G. S. Lal, G. P. Pez and R. G. Syvret, Chem. Rev., 1996, 96, 1737 CrossRef CAS PubMed; B. Lantano and A. Postigo, Org. Biomol. Chem., 2017, 15, 9954 RSC; T. Liang, C. N. Neumann and T. Ritter, Angew. Chem., Int. Ed., 2013, 52, 8214 CrossRef PubMed; A. Lin, C. B. Huehls and J. Yang, Org. Chem. Front., 2014, 1, 434 RSC; N. Naveen and R. Balamurugan, Org. Biomol. Chem., 2017, 15, 2063 RSC; P. Xu, S. Guo, L. Wang and P. Tang, Synlett, 2015, 26, 36 CrossRef; C. Chatalova-Sazepin, R. Hemelaere, J.-F. Paquin and G. M. Sammls, Synthesis, 2015, 47, 2554 CrossRef; D. D'Alonzo, M. D. Fenza, G. Palumbo, V. Romanucci, A. Zarrelli, G. D. Fabio and A. Guaragna, Synthesis, 2017, 49, 998 CrossRef.
- B. Huang, Y. Zhao, C. Yang, Y. Gao and W. Xia, Org. Lett., 2017, 19, 3799 CrossRef CAS PubMed; S. Sathyamoorthi, Y.-H. Lai, R. M. Bain and R. N. Zare, J. Org. Chem., 2018, 83, 5681 CrossRef PubMed; J. D. Galloway, D. N. Mai and R. D. Baxter, Org. Lett., 2017, 19, 5772 CrossRef PubMed; L. Niu, J. Liu, X.-A. Liang, S. Wang and A. Lei, Nat. Commun., 2019, 10, 1 CrossRef PubMed.
- Q. Tian, B. Chen and G. Zhang, Green Chem., 2016, 18, 6236 RSC; H. Wang, L.-N. Guo and X.-H. Duan, Chem. Commun., 2014, 50, 7382 RSC; L. Liu, J. Wang and H. Zhou, J. Org. Chem., 2015, 80, 4749 CrossRef CAS PubMed; X. Jiang, J. Yang, F. Zhang, P. Yu, P. Yi, Y. Sun and Y. Wang, Org. Lett., 2016, 18, 3154 CrossRef PubMed; X. Yuan, J.-F. Yao and Z.-Y. Tang, Org. Lett., 2017, 19, 1410 CrossRef PubMed; J. Q. Huang, Z. Nairoukh and I. Marek, Org. Biomol. Chem., 2018, 16, 1079 RSC; Z. Jin, R. S. Hidinger, B. Xu and G. B. Hammond, J. Org. Chem., 2012, 77, 7725 CrossRef PubMed; Y. Liu, X. Chen, J. Zhang and Z. Xu, Synlett, 2013, 24, 1371 CrossRef.
- B. Tréguier and S. P. Roche, Org. Lett., 2014, 16, 278 CrossRef CAS PubMed; Q. Zhao, H. Xiang, J.-A. Xiao, P.-J. Xia, J.-J. Wang, X. Chen and H. Yang, J. Org. Chem., 2017, 82, 9837 CrossRef PubMed; J. Zhang, H. Wang, S. Ren, W. Zhang and Y. Liu, Org. Lett., 2015, 17, 2920 CrossRef PubMed; D. Parmar and M. Rueping, Chem. Commun., 2014, 50, 13928 RSC.
- P. T. Nyffeler, S. G. Durón, M. D. Burkart, S. P. Vincent and C.-H. Wong, Angew. Chem., Int. Ed., 2005, 44, 192 CrossRef CAS PubMed.
- H. Poleschner and K. Seppelt, Chem.–Eur. J., 2004, 10, 6565 CrossRef CAS PubMed.
- R. Guo, L. Liao and X. Zhao, Molecules, 2017, 22, 835 CrossRef PubMed; Z. Tian, Z. Liu, P. Yu and W. Zhang, Chin. J. Appl. Chem., 2016, 33, 10 Search PubMed; L. Shao, Y. Li, J. Lu and X. Jiang, Org. Chem. Front., 2019, 6, 2999 RSC.
- Y. Usuki, M. Iwaoka and S. Tomoda, Chem. Lett., 1992, 8, 1507 CrossRef.
- Selenium and Tellurium Chemistry, Chapter 2: New Selenium Electrophiles and Their Reactivity, ed. J. D. Woollins and R. S. Laitinen, Springer-Verlag, Berlin, Heidelberg, 2011 RSC; H. Sahoo, G. S. Grandhi, I. Ramakrishna and M. Baidya, Org. Biomol. Chem., 2019, 17, 10163 RSC; V. Kumar, K. Banert, D. Ray and B. Saha, Org. Biomol. Chem., 2019 10.1039/C9OB02235J; G. Perin, L. K. Soares, P. S. Hellwig, M. S. Silva, J. S. S. Neto, J. A. Roehrs, T. Barcellos and E. J. Lenardão, New J. Chem., 2019, 43, 6323 RSC; H. A. Goular, J. S. S. Neto, A. M. Barcellos, T. Barcellos, M. S. Silva, D. Alges, R. G. Jacob, E. J. Lenardão and G. Perin, Adv. Synth. Catal., 2019, 361, 1 CrossRef CAS.
- B. Gao, L. Zhang, Q. Zheng, F. Zhou, L. M. Klivansky, J. Lu, Y. Liu, J. Dong, P. Wu and K. B. Sharpless, Nat. Chem., 2017, 9, 1083 CrossRef CAS PubMed; J. Dong, L. Krasnova, M. G. Finn and K. B. Sharpless, Angew. Chem., Int. Ed., 2014, 53, 9430 CrossRef PubMed.
- G. W. Fraser and J. B. Millar, J. Chem. Soc., Dalton Trans., 1974, 2029 RSC; J. V. Comasseto, R. L. O. R. Cunha and G. C. Clososki, Comprehensive Organometallic Chemistry III, Elsevier Ltd, Netherlands, 2007, vol. 9, pp. 587–648 Search PubMed.
- X. Gan, Z. Fu, L. Liu, Y. Yan, C. Chen, Y. Zhou and J. Dong, Tetrahedron Lett., 2019, 60, 150906 CrossRef CAS.
- W. Ye, W. Li and J. Zhang, Chem. Commun., 2014, 50, 9879 RSC; Q. Tian, B. Chen and G. Zhang, Green Chem., 2016, 18, 6236 RSC.
- R. Lin, S. Ding, Z. Shi and N. Jiao, Org. Lett., 2011, 13, 4498 CrossRef CAS PubMed; N. Liu, Q.-P. Tian, Q. Yang and S.-D. Yang, Synlett, 2016, 27, 2621 CrossRef; J.-W. Yuan, J.-L. Zhu, B. Li, L.-Y. Yang, P. Mao, S.-R. Zhang, Y.-C. Li and L.-B. Qu, Org. Biomol. Chem., 2019, 17, 10178 RSC.
- M. S. Chauhan, G. D. Yadav, F. Hussain and S. Singh, Catal. Sci. Technol., 2014, 4, 3945 RSC.
- H. Dueck, Sulfur, Selenium and Tellurium NMR, John Wiley & Sons Ltd, Oxford, UK, 2007 Search PubMed.
- C. Geng, L. Du, F. Liu, R. Zhu and C. Liu, RSC Adv., 2015, 5, 33385 RSC.
- X. Deng, H. Cao, C. Chen, H. Zhou and L. Yu, Sci. Bull., 2019, 64, 1280 CrossRef CAS.
Footnote |
† Electronic supplementary information (ESI) available. See DOI: 10.1039/d0ra01907k |
|
This journal is © The Royal Society of Chemistry 2020 |
Click here to see how this site uses Cookies. View our privacy policy here.