DOI:
10.1039/C5QO00391A
(Research Article)
Org. Chem. Front., 2016,
3, 309-313
Pd/norbornene-catalyzed sequential ortho-C–H alkylation and ipso-alkynylation: a 1,1-dimethyl-2-alkynol strategy†
Received
20th November 2015
, Accepted 26th December 2015
First published on 29th December 2015
Abstract
A palladium/norbornene-catalyzed ortho-C–H alkylation and ipso-alkynylation reaction for the synthesis of 2-alkyl-1-alkynyl arenes was reported. By the use of 1,1-dimethyl-2-alkynols as alkynyl reagents, the side O-alkylation reaction was mostly inhibited, and the reactivity of the three components matched well. As a result, A- and B-type side-products could be substantially inhibited and good to excellent yields were achieved.
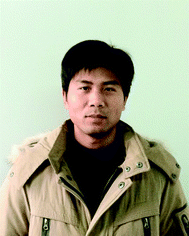 Zhenhua Gu | Zhenhua Gu studied chemistry at Nanjing University and received his PhD degree at the Shanghai Institute of Organic Chemistry in 2007 with Professor Shengming Ma. He conducted his postdoctoral research with Professor K. Peter C. Vollhardt (UC Berkeley) and Professor Armen Zakarian (UC Santa Barbara). In 2012, he began his independent academic career at the Department of Chemistry, the University of Science and Technology of China with the support from “Thousand Talents Plan”. Research in his group is mainly focused on the development of new methods and strategies for organic synthesis, including asymmetric catalysis, natural and un-natural bioactive molecule synthesis. |
Introduction
Multi-component reactions, including transition metal-catalyzed transformations, are one of the most attractive research areas in view of their step-economy.1 Among them, palladium/norbornene-catalyzed ortho C–H functionalization and ipso cross-coupling reaction is a unique transformation, which is particularly useful in the synthesis of poly-substituted aromatic compounds.2,3 Since its development in 1997, various polyfunctionalized arenes have been synthesized, particularly the contributions from the groups of Lautens and Catellani. During the past decade, numerous reagents, such as alkenes,4 cynane,5 amines/amides/imine,6 hydride,7 carbenes,8 and electron-rich arenes9 have been successfully applied to the final step ipso termination reactions and a number of interesting aromatic compounds have been synthesized.10 Recently Yu11 and Dong12 have developed Pd(II)-catalyzed efficiently selective meta-C–H functionalization reactions of arenes with ortho-directing groups, where norbornene worked as an efficient shuttle for selective meta-C–H functionalization. The utility of this reaction was further demonstrated by Lautens,13 Bach14 and our groups15 in the application of natural product synthesis, such as (+)-linoxepin, goniomitine, rhazinal etc. Alkynes are one of the most important functional groups in organic chemistry. They not only occur in many molecules, but are important building blocks or intermediates in organic syntheses.16 The ortho-substituted 1-alkynyl arenes are useful substrates for the synthesis of axially or helically chiral molecules.17 However, the Pd/norbornene-catalyzed ortho-C–H functionalization terminated by ipso-Sonogashira coupling has been rarely studied. In 2004 Catellani and co-workers18 disclosed a primary investigation on the ipso Sonogashira coupling termination, where a very slow addition of both alkyl bromides and terminal alkynes19 was necessary to avoid side-reactions and as a result a total of six days were required to get a reasonable conversion (80–89%). Recently we,20 later Chen and Wu et al.21, realized an efficient ipso-Sonogashira coupling for the synthesis of 2-alkynylanilines via protected alkynes by the release of small molecules, such as CO2etc., where the reactivity of different substrates matched well and the reaction was completed within 4 h with good yield.
Our continued studies found that the decarboxylative alkynylation strategy22 was only successful for the synthesis of 2-alkynylanilines. However, when alkyl iodide instead of O-benzoyl hydroxylamine was used, 99% of norbornene adduct A was isolated under identical reaction conditions. The use of a polar solvent, such as CH3CN in the reaction afforded a mixture of products with poor conversion (Scheme 1c). The desired ortho C–H alkylation and ipso-Sonogashira coupling product was curtailed to 10% of the isolated yield, and the major side reaction was the esterification of carboxylic acid with the alkyl iodides, such as the formation of B under basic conditions. We herein report our efforts on palladium/norbornene-catalyzed ortho-alkylation/ipso-Sonogashira coupling by the use of 1,1-dimethyl-2-alkynols23 as the alkynyl components.
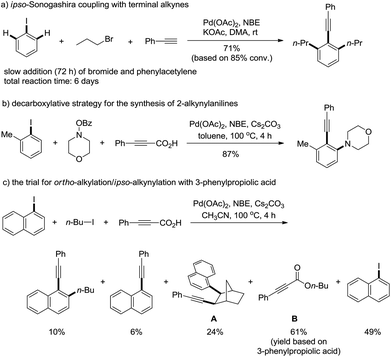 |
| Scheme 1 Sonogashira coupling termination for Catellani reaction. | |
Results and discussion
As mentioned above, the formation of propiolic esters is the critical problem for this transformation. We reasoned that the use of bulky 1,1-dimethyl-2-alkynols and tertiary alcohols would substantially lower the rate of O-alkylation reaction of alkynylation reagents, where terminal alkynes could be released via the loss of one molecule of acetone. Pleasingly, no O-alkylation product was detected when the reaction of 3a with 1-iodonaphthalene and 1-iodobutane was performed in dioxane, though only 20% of desired product 4a was isolated (Table 1, entry 1). Upon further optimization of the solvent, we found that a “non-polar” solvent, e.g. toluene was not suitable for this reaction (entry 2). The reactions had good performances in “polar” solvents, such as DME, DMF, MeCN etc., where MeCN was superior to others (entries 3–5). The reaction with K3PO4 as the base gave 43% yield of the desired product while the one with NaOAc was completely inert (entries 6 and 7). Other palladium sources, such as PdCl2, Pd(dba)2 were also screened, and no higher yield was obtained than Pd(OAc)2 (entries 8 and 9). The reaction proceeded well in the absence of phosphine ligands though a relatively low yield of 4a was achieved (entry 10). It should be noted that the reaction is sensitive to moisture and fresh distilled dry CH3CN from calcium hydride is necessary for a reproducible yield. The addition of water scavengers also endeavoured: MgSO4 showed good performance for this transformation and the yields became more reliable than the ones in the absence of MgSO4 (entries 11 and 12).24
Table 1 Reaction condition optimizationa
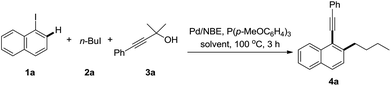
|
Entry |
Base |
Additive |
Solvent |
Yielda/% |
The reaction was conducted on 0.20 mmol of 1a, 0.40 mmol of 2a, 0.30 mmol of 3a, 0.60 mmol of norbornene, 0.60 mmol of Cs2CO3, 5 mol% of Pd(OAc)2, and 12.5 mol% of phosphine.
PdCl2 was used.
Pd(dba)2 was used.
No phosphine was used.
|
1 |
Cs2CO3 |
— |
Dioxane |
20 |
2 |
Cs2CO3 |
— |
Toluene |
Trace |
3 |
Cs2CO3 |
— |
DME |
67 |
4 |
Cs2CO3 |
— |
DMF |
50 |
5 |
Cs2CO3 |
— |
MeCN |
70 |
6 |
K3PO4 |
— |
MeCN |
43 |
7 |
NaOAc |
— |
MeCN |
NR |
8b |
Cs2CO3 |
— |
MeCN |
64 |
9c |
Cs2CO3 |
— |
MeCN |
48 |
10d |
Cs2CO3 |
— |
MeCN |
58 |
11 |
Cs2CO3 |
4A MS (200 mg) |
MeCN |
62 |
12 |
Cs2CO3 |
MgSO4 (150 mg) |
MeCN |
88 |
With the optimized reaction conditions in hand, a series of alkyl iodides 2 and 1,1-dimethyl-2-alkynols 3 were tested (Table 2). The reaction proceeded equally efficiently when iodoethane, iodopropane, and (3-iodopropyl)benzene were used as alkylation reagents (4b–d). The O-Bn, O-TBDPS and O-PMB functionalities in alkyl chains could be tolerated, and good to excellent yields were obtained (4e–g). 1-(Chloromethyl)-4-methoxybenzene (PMBCl) was also a compatible substrate, and the reaction afforded 58% yield of 4h in CH3CN while 80% in 1,2-dimethoxyethane. The electronic effect of 3-phenylprop-2-yn-1-ols was investigated. The reactions with substrates bearing electron-donating groups on the phenyl rings, such as p-methyl, p-(tert-butyl) and p-methoxyl groups gave the corresponding products in good yields (4i–k). In contrast to the decarboxylative synthesis of 2-alkynylanilines, this reaction worked well with the electron-withdrawing (CF3) or phenyl group on the para-position of phenyl rings, albeit relatively low yields were obtained (4l and 4m). The reaction proceeded uneventfully with other 3-arylprop-2-yn-1-ols (4n–p). Pleasingly it was found that the aliphatic substituted prop-2-yn-1-ols were compatible components, and the corresponding reactions gave decent yields of the desired products (4q and 4r).
The reaction was conducted on 0.20 mmol of 1a, 0.40 mmol of 2, 0.30 mmol of 3, 0.60 mmol of norbornene, 0.60 mmol of Cs2CO3, 5 mol% of Pd(OAc)2, and 12.5 mol% of P(p-MeOC6H4)3.
1-(Chloromethyl)-4-methoxybenzene was used.
|
|
The generality of this reaction was further explored by studies of various substituted iodoarenes, and a series of poly-substituted phenyl acetylenes could be synthesized easily (Table 3). Generally electron-rich iodoarenes worked better than the ones bearing electron-withdrawing groups. For example, the compounds bearing an ortho-CF3, chloro, or para-NO2 group (4s, 4A and 4C) were formed in around 60% yields while 4t–v, 4B, and 4D were isolated in 71–90% yields. The reaction with 2-iodo-(O-TBS)-hydroxymethyl benzene proceeded uneventfully to deliver the product 4w in 61% yield. The meta-chloro or fluoro-substitution has less electronic effect for this transformation, and both 4x and 4y were formed in 81% yields.
Table 3 Substrate scope for iodoarenesa
The reaction was conducted on 0.20 mmol of 1a, 0.40 mmol of 2, 0.30 mmol of 3, 0.60 mmol of norbornene, 0.60 mmol of Cs2CO3, 5 mol% of Pd(OAc)2, and 12.5 mol% of P(p-MeOC6H4)3.
|
|
The use of alkyl bromides instead of alkyl iodides dramatically decreased the efficiency of this reaction. However, the yield could be improved by the addition of NaI as the additive (Scheme 2).
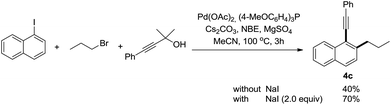 |
| Scheme 2 The reactions with 1-bromopropane. | |
A brief catalytic cycle for this transformation is proposed in Scheme 3 with 1-iodonaphthalene and 1-iodobutane and 3a as typical substrates. The oxidative addition of Pd(0) with 1a, followed by NBE insertion would give I. Due to the lack of cis-β-hydride, I would undergo carbopalladation to release one molecule HX and form II, which gave III after the oxidative addition with n-BuI and reductive elimination. Norbornene extrusion of III delivered the classic steric bulky aryl palladium species IV. Anion exchange of IV with 1,1-dimethyl-2-alkynols form alkoxypalladium V, which in situ generate alkynylpalladium VIvia the release of acetone. Finally, the reaction delivered the product via reductive elimination. The anion exchange of I with 3a would give VII, which was supposed to afford the A-type by-product via decarbonylation and reductive elimination. However, the relatively slow rate of decarbonylation in comparison with the formation of IIvia cyclopalladation led to the reaction disfavouring the formation of the A-type by-product.
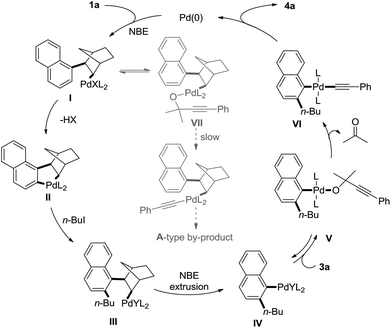 |
| Scheme 3 The plausible catalytic cycle. | |
Conclusions
We have developed a protocol for the efficient synthesis of poly-substituted 2-alkyl-1-alkynyl arenes in moderate to excellent yields via Pd/norbornene co-catalysis. The use of 1,1-dimethyl-2-alkynols as alkynyl reagents not only matched the reactivity with other components, but also substantially inhibited the side-reaction of O-alkylation.
Acknowledgements
This work was supported by the ‘973’ project from the MOST of China (2015CB856600), NSFC (21272221, 21472179), the Recruitment Program of Global Experts, and the Fundamental Research Funds for the Central Universities (WK 2060190028, 2060190026 and 3430000001).
Notes and references
-
(a) R. W. Armstrong, A. P. Combs, P. A. Tempest, S. D. Brown and T. A. Keating, Acc. Chem. Res., 1996, 29, 123 CrossRef CAS;
(b) I. Ugi, Pure Appl. Chem., 2001, 73, 187 CrossRef CAS;
(c) A. Dömling and I. Ugi, Angew. Chem., Int. Ed., 2000, 39, 3168 CrossRef;
(d)
Multicomponent Reactions, ed. J. Zhu and H. Bienaryrme, Wiley-VCH, Weinheim, 2005 Search PubMed.
-
(a) M. Catellani and L. Ferioli, Synthesis, 1996, 769 CrossRef CAS;
(b) M. Catellani, F. Frignani and A. Rangoni, Angew. Chem., Int. Ed. Engl., 1997, 36, 119 CrossRef CAS.
-
(a) M. Catellani, Synlett, 2003, 298 CrossRef CAS;
(b) M. Catellani, Top. Organomet. Chem., 2005, 14, 21 CAS;
(c) M. Lautens, D. Alberico, C. Bressy, Y.-Q. Fang, B. Mariampillai and T. Wilhelm, Pure Appl. Chem., 2006, 78, 351 CrossRef CAS;
(d) M. Catellani, E. Motti and N. Della Ca’, Acc. Chem. Res., 2008, 41, 1512 CrossRef CAS PubMed;
(e) A. Martins, B. Mariampillai and M. Lautens, Top. Curr. Chem., 2010, 292, 1 CrossRef CAS PubMed;
(f) P. Sehnal, R. J. K. Taylor and L. J. S. Fairlamb, Chem. Rev., 2010, 110, 824 CrossRef CAS PubMed;
(g) G. P. Chiusoli, M. Catellani, M. Costa, E. Motti, N. Della Ca’ and G. Maestri, Coord. Chem. Rev., 2010, 254, 456 CrossRef CAS;
(h) J.-T. Ye and M. Lautens, Nat. Chem., 2015, 7, 863 CrossRef CAS PubMed.
-
(a) A. Rudolph, N. Rackelmann, M.-O. Turcotte-Savard and M. Lautens, J. Org. Chem., 2009, 74, 289 CrossRef CAS PubMed;
(b) Z. Qureshi, W. Schlundt and M. Lautens, Synthesis, 2015, 2446 CAS;
(c) P.-X. Zhou, Y.-Y. Y. C. Liu, L.-B. Zhao, J.-Y. Hou, D.-Q. Chen, Q. Tang, A.-Q. Wang, J.-Y. Zhang, Q.-X. Huang, P.-F. Xu and Y.-M. Liang, ACS Catal., 2015, 5, 4927 CrossRef CAS;
(d) Y. Huang, R. Zhu, K. Zhao and Z. Gu, Angew. Chem., Int. Ed., 2015, 54, 12669 CrossRef CAS PubMed;
(e) F. Faccini, E. Motti and M. Catellani, J. Am. Chem. Soc., 2004, 126, 78 CrossRef CAS PubMed;
(f) A. Rudolph, N. Rackelmann and M. Lautens, Angew. Chem., Int. Ed., 2007, 46, 1485 CrossRef CAS PubMed;
(g) H. Zhang, P. Chen and G. Liu, Angew. Chem., Int. Ed., 2014, 53, 10174 CrossRef CAS PubMed;
(h) Z.-Y. Chen, C.-Q. Ye, X.-P. Zeng and J.-J. Yuan, Chem. – Eur. J., 2014, 20, 4237 CrossRef CAS PubMed.
-
(a) B. Mariampillai, D. Alberico, V. Bidau and M. Lautens, J. Am. Chem. Soc., 2006, 128, 14436 CrossRef CAS PubMed;
(b) B. Mariampillai, J. Alliot, M. Li and M. Lautens, J. Am. Chem. Soc., 2007, 129, 15372 CrossRef CAS PubMed.
-
(a) P. Thansandote, M. Raemy, A. Rudolph and M. Lautens, Org. Lett., 2007, 9, 5255 CrossRef CAS PubMed;
(b) P. Thansandote, E. Chong, K.-O. Feldmann and M. Lautens, J. Org. Chem., 2010, 75, 3495 CrossRef CAS PubMed;
(c) D. A. Candito and M. Lautens, Org. Lett., 2010, 12, 3312 CrossRef CAS PubMed;
(d) D. A. Candito and M. Lautens, Angew. Chem., Int. Ed., 2009, 48, 6713 CrossRef CAS PubMed;
(e) M. Blanchot, D. A. Candito, F. Larnaud and M. Lautens, Org. Lett., 2011, 13, 1486 CrossRef CAS PubMed;
(f) V. Narbonne, P. Retailleau, G. Maestri and M. Catellani, Org. Lett., 2014, 16, 628 CrossRef CAS PubMed.
-
(a) K. Mitsudo, P. Thansandote, T. Wilhelm, B. Mariampillai and M. Lautens, Org. Lett., 2006, 8, 3939 CrossRef CAS PubMed;
(b) A. Martins, D. A. Candito and M. Lautens, Org. Lett., 2010, 12, 5186 CrossRef CAS PubMed;
(c) Z. Dong and G. Dong, J. Am. Chem. Soc., 2013, 135, 18350 CrossRef CAS PubMed;
(d) Z. Dong, J. Wang, Z. Ren and G. Dong, Angew. Chem., Int. Ed., 2015, 54, 12664 CrossRef CAS PubMed.
-
(a) P.-X. Zhou, Y.-Y. Ye, J.-W. Ma, L. Zheng, Q. Tang, Y.-F. Qiu, B. Song, Z.-H. Qiu, P.-F. Xu and Y.-M. Liang, J. Org. Chem., 2014, 79, 6627 CrossRef CAS PubMed;
(b) P.-X. Zhou, L. Zheng, J.-W. Ma, Y.-Y. Ye, X.-Y. Liu, P.-F. Xu and Y.-M. Liang, Chem. – Eur. J., 2014, 20, 6745 CrossRef CAS PubMed.
-
(a) C. Bressy, D. Alberico and M. Lautens, J. Am. Chem. Soc., 2005, 127, 13148 CrossRef CAS PubMed;
(b) C. Blaszykowski, E. Aktoudianakis, C. Bressy, D. Alberico and M. Lautens, Org. Lett., 2006, 8, 2043 CrossRef CAS PubMed;
(c) A. Martins, D. Alberico and M. Lautens, Org. Lett., 2006, 8, 4827 CrossRef CAS PubMed;
(d) C. Blaszykowski, E. Aktoudianakis, D. Alberico, C. Bressy, D. G. Hulcoop, F. Jafarpour, A. Joushaghani, B. Laleu and M. Lautens, J. Org. Chem., 2008, 73, 1888 CrossRef CAS PubMed;
(e) N. Della Ca’, G. Maestri and M. Catellani, Chem. – Eur. J., 2009, 15, 7850 CrossRef PubMed;
(f) C. Lei, X. Jin and J. S. Zhou, Angew. Chem., Int. Ed., 2015, 54, 13397 CrossRef CAS PubMed.
- For other related typical examples, see:
(a) L. Jiao and T. Bach, J. Am. Chem. Soc., 2011, 133, 12990 CrossRef CAS PubMed;
(b) M. Cheng, J. Yan, F. Hu, H. Chen and Y. Hu, Chem. Sci., 2013, 4, 526 RSC;
(c) K. M. Gericke, D. I. Chai, N. Bieler and M. Lautens, Angew. Chem., Int. Ed., 2009, 48, 1447 CrossRef CAS PubMed;
(d) H. Liu;, M. El-Salfiti and M. Lautens, Angew. Chem., Int. Ed., 2012, 51, 9846 CrossRef PubMed.
-
(a) X.-C. Wang, W. Gong, L.-Z. Fang, R.-Y. Zhu, S. Li, K. M. Engle and J.-Q. Yu, Nature, 2015, 519, 334 CrossRef CAS PubMed;
(b) P.-X. Shen, X.-C. Wang, P. Wang, R.-Y. Zhu and J.-Q. Yu, J. Am. Chem. Soc., 2015, 137, 11574 CrossRef CAS PubMed.
- Z. Dong, J. Wang and G.-B. Dong, J. Am. Chem. Soc., 2015, 137, 5887 CrossRef CAS PubMed.
- H. Weinstabl, M. Suhartono, Z. Qureshi and M. Lautens, Angew. Chem., Int. Ed., 2013, 52, 5305 CrossRef CAS PubMed.
- L. Jiao, E. Herdtweck and T. Bach, J. Am. Chem. Soc., 2012, 134, 14563 CrossRef CAS PubMed.
- X. Sui, R. Zhu;, G. Li, X. Ma and Z. Gu, J. Am. Chem. Soc., 2013, 135, 9318 CrossRef CAS PubMed.
-
(a)
Modern Acetylene Chemistry, ed. P. J. Stang and F. Diederich, VCH, Weinheim, 1995 Search PubMed;
(b)
Acetylene Chemistry: Chemistry, Biology and Material Science, ed. F. Diederich, P. J. Stang and R. R. Tykwinski, Wiley-VCH, Weinheim, 2005 Search PubMed;
(c) R. Chinchilla and C. Nájera, Chem. Rev., 2014, 114, 1783 CrossRef PubMed;
(d) R. K. Kumar and X. Bi, Chem. Commun., 2016, 52, 853 RSC.
-
Transition-Metal-Mediated Aromatic Ring Construction, ed. K. Tanaka, John Wiley & Sons, Inc., Hoboken, New Jersey, 2013 Search PubMed.
- E. Motti, M. Rossetti, G. Bocelli and M. Catellani, J. Organomet. Chem., 2004, 689, 3741 CrossRef CAS.
- The slow addition was necessary for some terminal alkyne-involved reactions to avoid side reactions, see:
(a) T. Hamada, X. Ye and S. S. Stahl, J. Am. Chem. Soc., 2008, 130, 833 CrossRef CAS PubMed;
(b) L. Chu; and F.-L. Qing, J. Am. Chem. Soc., 2010, 132, 7262 CrossRef PubMed;
(c) X. Jiang, L. Chu and F.-L. Qing, J. Org. Chem., 2012, 77, 1251 CrossRef CAS PubMed.
- F. Sun and Z. Gu, Org. Lett., 2015, 17, 2222 CrossRef CAS PubMed.
- S.-F. Pan, X.-J. Ma, D.-N. Zhong, W.-Z. Chen, M.-C. Liu and H.-Y. Wu, Adv. Synth. Catal., 2015, 357, 3052 CrossRef CAS.
-
(a) A. Fromm, C. Wüllen, D. Hackenberger and L. J. Gooßen, J. Am. Chem. Soc., 2014, 136, 10007 CrossRef CAS PubMed;
(b) C. K. Haley, C. D. Gilmore and B. M. Stoltz, Tetrahedron, 2013, 69, 5732 CrossRef CAS PubMed;
(c) P. Hu, M. Zhang, X. Jie and W. Su, Angew. Chem., Int. Ed., 2012, 51, 227 CrossRef PubMed;
(d) K. Xie, Z. Yang, X. Zhou, X. Li, S. Wang, Z. Tan, X. An and C.-C. Guo, Org. Lett., 2010, 12, 1564 CrossRef CAS PubMed;
(e) L. J. Goossen, G. Deng and L. M. Levy, Science, 2006, 313, 662 CrossRef CAS PubMed;
(f) L. J. Goossen, N. Rodríguez, B. Melzer, C. Linder, G. Deng and L. M. Levy, J. Am. Chem. Soc., 2007, 129, 4824 CrossRef CAS PubMed;
(g) C.-C. Hu and Y.-Y. Chen, Org. Chem. Front., 2015, 2, 1352 RSC;
(h) J. M. Crawford, K. E. Shelton, E. K. Reeves, B. K. Sadarananda and D. Kalyani, Org. Chem. Front., 2015, 2, 726 RSC.
-
(a) A. Dermenci, J. W. Coe and G. Dong, Org. Chem. Front., 2014, 1, 567 RSC;
(b) P. Nguyen, S. Todd, D. Van den Biggelaar, N. J. Taylor, T. B. Marder, F. Wittmann and R. H. Friend, Synlett, 1994, 299 CrossRef CAS;
(c) C. Huynh and G. Linstrumelle, Tetrahedron, 1988, 44, 6337 CrossRef;
(d) C.-K. Choi, I. Tomita and T. Endo, Chem. Lett., 1999, 28, 1253 CrossRef;
(e) H. F. Chow, C. W. Wan, K. H. Low and Y. Y. Yeung, J. Org. Chem., 2001, 66, 1910 CrossRef CAS PubMed;
(f) H. Hu, F. Yang and Y.-J. Wu, J. Org. Chem., 2013, 78, 10506 CrossRef CAS PubMed;
(g) T. Nishimura, H. Araki, Y. Maeda and S. Uemura, Org. Lett., 2003, 5, 2997 CrossRef CAS PubMed.
- The corresponding reactions with other phosphine ligands under the optimized conditions, such as PPh3, P(2-fury)3, Xphos, bis-[2-(diphenylphosphino]phenyl]ether, P(2-MeOC6H4)3 gave 4a in 45%, 29%, 21%, 53% and 32% yields, respectively.
Footnote |
† Electronic supplementary information (ESI) available: Experimental procedures, characterization data, 1H and 13C NMR spectra for all new compounds. See DOI: 10.1039/c5qo00391a |
|
This journal is © the Partner Organisations 2016 |