Microplastic distribution and ecological risks: investigating road dust and stormwater runoff across land uses†
Received
10th May 2023
, Accepted 10th November 2023
First published on 13th November 2023
Abstract
Road dust and stormwater runoff are significant pathways for transporting microplastics (MPs) from land-based sources to the surrounding ecological compartments. The aim of this study is to understand the occurrence of MPs in both road dust and stormwater samples collected under various land uses, including residential, commercial and industrial areas, within Melbourne metropolitan city, Australia. This study also evaluated the ecological risk indices of MP polymers under different land uses. MP emission characteristics and loads (number- and mass-based) were estimated to investigate pollution risks. Higher quantities of MPs were detected in road dust and stormwater in industrial areas (2410 items per kg and 35 items per L) than in commercial (2130 items per kg and 27 items per L) and residential (1970 items per kg and 24 items per L) areas. Mass loads of MPs were also higher in industrial regions in road dust and stormwater samples. It was found that 200 μm to 2450 μm sized MPs were abundant in road dust, which was higher than the sizes of stormwater MPs (125 μm to 960 μm). The water forces in the drainage system could be the reason for the breakdown of larger MPs. Fragments and fibers were the dominant shapes of MPs in all the selected areas. Fourier transform infrared spectroscopy of representative samples identified several types of polymers, predominantly polypropylene, polyethylene, polyethylene terephthalate/polyester, polyvinyl chloride and polyethylene. The hazard index indicates that the ecological risks of MPs are higher in industrial areas than in other areas. This study revealed that MP emission via road dust was significantly higher due to traffic and industrial and human activities. This study has demonstrated that stormwater runoff is the primary corridor for transporting MPs from road dust to the aquatic environment of wetlands.
Environmental significance
Microplastic (MP) pollution in road dust and stormwater runoff is a critical environmental concern, as it transports MPs from land-based sources to aquatic ecosystems. Addressing this issue is vital to preserve ecological health and mitigate contamination risks. The study found that industrial areas in Melbourne, Australia, exhibited higher MP quantities and ecological risks compared to commercial and residential areas. This increased pollution is attributed to traffic and industrial and human activities. The key finding highlights stormwater runoff as the main transport mechanism of MPs from road dust to wetlands. Understanding and addressing this issue has significant implications for developing targeted pollution control strategies, particularly in industrial areas, to protect the environment and maintain ecological balance.
|
1. Introduction
Plastics are generally light weight, inexpensive and easily molded but highly persistent in the environment. The current period of humankind can be coined as the beginning of the Plasticene Age due to plastic mineralization marks in rocks after we left the Holocene era.1 Plastics represent an unparalleled turning point in Earth's evolutionary history because virtually no living organism in the environment has evolved to readily consume them. Small plastic elements (<5 mm), which vary in shape, color, and size, are called microplastics (MPs).2 MPs are found in various environmental media, and approximately 2.7 million tons of MPs are released annually into water bodies worldwide.3 The number continually increases due to their thermal and bio-degradation resistances.4 MPs can become efficient carriers of environmental pollutants due to their small size, hydrophobicity, high specific surface area and weak photodegradation capability. MPs adhering to other organic pollutants can produce more complex secondary contaminants, generating advanced compound toxicity.5 The ingestion of MPs by marine species has been broadly reported, including fish, mussels, sea birds, turtles, lobsters and other marine mammals.6,7 Human health is severely threatened by the accumulation of MPs in the food chain.8
Primary MPs are intentionally formed solid products from manufacturing processes, while secondary MPs are fragments from larger plastics due to weathering actions and shear forces.9–12 Primary MPs are typically tiny spherical microbeads deliberately produced by the plastics industry for cosmetics, synthetic textiles, personal care products (PCPs), detergents and other cleaning agents. Secondary MPs are irregular plastics inadvertently produced due to the degradation of larger pieces of plastic, like plastic bags, crates, bottles, ropes and nets.13,14 Secondary MPs eventually form under constant exposure to ecological stresses.15 The fragmentation of plastics contributes more significantly to the abundance of MPs in the environment than the release of primary MPs. These primary MPs are predominantly found in PCPs, such as scrubs for cosmetics, skin cleansers, textile fibers, liquid baths and exfoliants in soap and toothpaste.16 Facial scrubs contain MPs of around 1000–19
000 particles per mL with various sizes, colors and shapes.17 Currently, various types of plastics are available in the market, such as polyvinyl chloride (PVC), polyethylene (PE), polyurethane (PUR), polypropylene (PP), polystyrene (PS), polyethylene terephthalate (PET) and synthetic rubber (SR).18,19 As such, one of the aims of this research study is to delineate the composition and distribution of these MPs in roadside kerbs.
Road dust is one of the forms of outdoor dust in a terrestrial environment. It comprises various elements created from organic and inorganic ingredients (such as rocks, metals and soil) by physical abrasion. These are also produced through several anthropogenic actions, including construction work and vehicular traffic.20,21 Road dust is known to contain non-depleted vehicle emissions from brake wear and tyres, plastic-enhanced road particles, polymer-modified bitumen, road marking paints and road pavement.22,23 It has been recognized as a rich source of MPs in urban areas, with 70–80% of marine MPs projected to originate from these land-based sources.24 The types and quantities of pollutants depend on the land use of the neighboring areas. One of the earliest reports in Australia investigated the presence of MPs in road dust25 and suggested that roads are the first point of release for MPs originating from wear and tear of tyres. Road dust and MPs from numerous anthropogenic actions are ultimately gathered in the open ocean. Wetlands and rivers are transitional water bodies that retain some MPs, and the remaining MPs are conveyed to oceans from varying terrestrial sources.26 MPs and road dust are transported into wetlands via stormwater and atmospheric winds. Hence, stormwater runoff is a significant source of contaminants for marine and aquatic habitats.27
Numerous research studies on major component analyses have suggested that rainfall and urbanization influence the accumulation of MPs.28–30 After a 3 day rainfall event in a Hong Kong river, the MP abundance was nearly twice that found on the coastal sea surface in the same area.31 An investigation of MP samples from three rivers in the Los Angeles area found a density in the range of of 33–4330 items per m3, with particle sizes of 1–4.75 mm. The average count of MPs in the sample was higher in the wet season than in the dry season.32 MP fragments in stormwater have also been examined in Mexico33 and Denmark34 to indicate the significance of stormwater runoff as a cause of MP accumulation in oceans.
MP pollution in the atmosphere is also a growing concern, but the understanding of its measurement to determine the amounts remains inadequate, as actual field-based measurements are limited. While urban runoff is a significant source of MPs in oceans, in most cases, the stormwater does not receive any treatment before reaching water bodies. Therefore, long-term monitoring of various stormwater runoffs is needed to assess the MPs accurately and recommend mitigation measures. However, recent reports on detecting MPs in stormwater and road dust are limited in detailed quantification, identification and classification. Few studies concerning MPs in limited industrial and residential areas have been published,35–37 but a comprehensive field survey is still lacking. Land use is critical in assessing MP loads in the aquatic environment. A unified sampling approach for MPs from multiple areas and applying different analytical methods to address different MP sizes are crucial for evaluating their impact on ecology. Therefore, the aims of this research are (i) to identify MPs in road dust and stormwater runoff across different land uses in Melbourne, Australia, (ii) to classify the morphology, mass loads, chemical composition and comparison MPs, and (iii) to evaluate the ecological risk indices of MP polymers.
2. Materials and methods
2.1 Study area and sample collection
The sample collection sites represent different industrial, commercial and residential land uses in Melbourne, Australia (Fig. S1†). Industrial zones have high traffic densities, while commercial and residential zones have moderate and low traffic densities, respectively. Traffic in industrial zones comprises predominantly large trucks, delivery vehicles and employee cars, resulting in consistently high traffic density across all chosen industrial areas. In contrast, commercial hubs such as shopping districts, malls and commercial centers witness heavy traffic, particularly on weekends and holidays, with traffic moderating during other times due to the presence of delivery trucks and shoppers. In contrast to industrial and commercial regions, the chosen residential areas display a lower level of traffic density. These specific neighborhoods typically accommodate a mix of local traffic from residents' vehicles and sporadic through traffic. To promote safety and control speed, residential areas frequently implement reduced speed limits and deploy traffic-calming measures. Road dust samples were collected from 30 locations, with 10 each for industrial, commercial and residential land use, as shown in Table S1.† Approximately 500 g of road dust samples were collected from the roadside kerbs using a small hand shovel and by using pig-bristle brushes to sweep the road gutter surface after a period of at least 136–144 consecutive hours of dry weather before sampling. Airtight polyethylene bags were temporarily used for sample collection, and the samples were then sent to the laboratory following previously reported protocols.38 In the laboratory, the samples were first air-dried at room temperature and then mechanically passed through a 4.7 mm sieve to remove larger debris such as large particles and plant leaves; which were predominantly from the leaves and tree barks from the nearby vegetation. Following this, all the samples were securely preserved in glass containers, ensuring no possibility of contamination, and they were earmarked for further MP analysis.
On the other hand, around 3 L of stormwater samples were collected from the wetland inlets using a sterile glass jar after rainfall. As shown in Table S2,† the wetland locations were also selected based on residential, commercial, and industrial land uses. These samples were stored at 4 °C for further analysis. The road dust and stormwater sample collection period spanned from March to July 2022.
2.2 Sample processing
To remove large elements (>5 mm), all samples were sieved using a 4.7 mm size sieve. The next step involved removing organic matter from the samples by oxidation using hydrogen peroxide (H2O2). In accordance with the methodology detailed in the research conducted by Ziajahromi et al.,39 the initial step in the identification of MPs entailed the removal of organic materials through this oxidation process. Around 50 g of road dust samples were placed in a glass beaker, and then 100 mL of ultrapure water and 50 mL of 30% (w/w) H2O2 solution were added. A magnetic stirrer was used to ensure consistent mixing of the solution. Afterward, the beaker was kept in a fume hood on a hot plate at 75 °C for digestion. The procedure was carried out until there were no longer any observable bubbles resulting from the oxidation of organic matter in the beaker. When bubbles were no longer visible in the solution, the hot plate was turned off, and the beaker was left to cool down. This step eliminated organic matter and opened the matrix for subsequent settling and filtration. De-ionized water was sprayed onto the inside wall of the beaker to dislodge the particles sticking to the walls. The suspension material was subsequently passed through filter paper, while the sediment material was isolated and collected separately. Grade 1 filter paper (90 mm diameter and 6 μm pore size) was used in a vacuum-supported arrangement. After filtration, the filter papers were dried in an oven (100 °C) for 24 h, and then placed under a microscope to identify MPs in these samples. The stormwater samples underwent the same preparation procedures.
2.3 Visual inspections and chemical analysis of the samples
A photo-optical digital microscope with 1600× magnification was used to identify and quantify all MPs. The shapes (films, fibers, beads, fragments, and foams) and color of MPs were examined. Fourier transform infrared spectroscopy (FTIR) analysis identified the chemical bonds in a particle by creating an electromagnetic absorption spectrum. FTIR spectra were recorded with an attenuated total reflection (ATR) fixture in the wavelength region of 500–4000 cm−1 at a 4 cm−1 resolution with 64 scans. The spectra were compared with numerous online spectral catalogs, and the spectra of the plastics standard in the library were set for polymer identification using PerkinElmer Spectrum version 10.5.2 software. However, FTIR may not be an ideal method for analyzing very small MPs due to the signal's sensitivity to particle size. When particles are extremely tiny, they might not produce a measurable signal. Additionally, successful FTIR analysis requires sample homogeneity, and in cases of poor particle distribution or high heterogeneity, obtaining accurate spectra becomes challenging. Overlapping absorption bands from various components can further complicate material identification, posing a substantial limitation for MP analysis.
A scanning electron microscope (SEM) was also employed to examine the surface morphology of the samples and was combined with an Oxford X-MaxN-20 energy-dispersive X-ray (EDX) detector for determining the composition of various elements.40 A conductive metal gold layer was created on the sample to prevent charging, reduce thermal damage, and improve the secondary electronic signals required for topographic examination in the SEM. The size distribution analysis of MPs was performed using the laser diffraction technique with a Malvern Mastersizer 3000. All samples were placed in 10 mL ultrapure water and stirred with a glass stirrer before analysis. The final result was expressed as a volume-weighted mean average of twenty-five measurements, and calculations were made from each size range mean.41
2.4 Microplastic load calculations/mass concentration
The mass concentration was calculated for the abundance of MPs in road dust/stormwater using each particle's shape, size, and polymer density. For calculation purposes, films and fragments were assumed to be cuboid, fibers, cylindrical, and microbeads and foams, spherical. The MP load was calculated using the following equations.42
Mass of a fragment and film = l × w × h × ρ |
Mass of a fiber = πr2l × ρ |
where l and w are the length (measured longest axis) and width (measured shortest axis), respectively; h is the height, where the minimum and maximum sizes were assumed to be 1/10th and 1/3rd of the longest axis, respectively; ρ is the density of polymers; r is the measured radius of the fiber and microbeads.
2.5 MP polymer risk indices: hazard index (Hi)
Plastics are composed of various additives and polymers. By considering MP abundance and chemical composition, the ecological risks of MPs can be evaluated.43 A MP polymer has various toxic impacts as it has a specific toxicity level, depending on its composition and chemical characteristics.44 By utilizing hazard scores, the polymeric risks were assessed.44 The chemical toxicity coefficients of the associated MP polymers and their abundances were used to calculate their pollution risks. The following formula was used for the assessment.45
where Hi is the summation of all MP polymeric risks/hazard indices at site i, j represents a polymer type, e.g., polyethylene (PE), m is the number of identified polymer types, Pji is the number of each MP polymer type identified at site i, and Ci is the MP's abundance at site i. Sj is the chemical toxicity coefficient or risk score obtained from the hazard scores assigned using ref. 44 for different polymers; some of the prominent Sj are PE = 11, PET = 4, PVC = 10
001, PP = 1, and PS = 30.
2.6 Quality control
During the experimental analysis, researchers placed a strong emphasis on minimizing the risk of cross-contamination of microplastic particles. As a preventive measure to reduce plastic contamination during the microplastic separation process, the choice has been made to wear cotton attire. Various precautions were taken to minimize contamination at every stage of the study, including thorough cleaning of the lab space with acetone, enveloping the glass apparatus in aluminum foil throughout the study period, and keeping the window frames and ventilators closed as a precautionary measure. These measures were implemented to maintain the integrity of the samples during transport, pretreatment and analysis. The pretreatment processes and filtration were conducted within a sterilized steel fume hood, and the personnel involved wore cotton lab coats and nitrile gloves. Subsequently, the filtered materials were preserved in sanitized glass containers shielded with aluminum foil. Prior to analysis, the filters were moved from the fume hood to the FTIR laboratory, ensuring that they remained covered with aluminum foil to prevent any potential external contamination. Field blanks were obtained during five separate sampling events for both categories to ensure quality control. These facilitated the identification and quantification of any contaminants introduced during the sampling and preparation procedures. These field blanks consisted of filtered water that underwent the same procedures as the actual samples in terms of collection, storage, pretreatment and analytical processes. MPs were detected in all blanks in minimal quantities, and there were no discernible distinctions between various types of blanks in terms of quantity, size, color, or morphological distribution. Nevertheless, sample concentrations were adjusted by subtracting the average number of particles within each size/morphology category in the blanks from the corresponding category in each sample.
The sampling efficiency test was performed to assess the loss of MP particles during sample processing. To determine the recovery rate, polystyrenes (PS) with a size of 1 mm were added to three separate glass dishes. The number of MPs was counted (40, 30 and 40, respectively) under a photo-optical digital microscope. The recovery samples went through the same processing method as the actual samples. After this process, the PSs were counted under a photo-optical digital microscope again with a proper light source.
3. Results and discussion
The identification, classification, and quantification of different MPs in road dust and stormwater under various land uses are described in the following section. FTIR and SEM-EDX analyses were used to identify the polymer types and for the identification of MPs.
3.1 Mapping of MPs in road dust under different land uses
3.1.1 Quantification of MPs in road dust.
MPs abundances in road dust samples were estimated for 30 selected industrial, commercial, and residential zones; the ranges were 1280 to 2410 items per kg, 620 to 2130 items per kg and 540 to 1270 items per kg, respectively. The highest number of MPs was in the industrial area because the selected industrial sites contain manufacturing precincts, high traffic density, workshops, parking lots, and business hubs. It was found that MP concentration varied with the traffic volume, population, and industrial activities.46 The highest MP abundance, 2410 items per kg, was obtained from Grieve Parade in the industrial site. Patchaiyappan et al.47 reported a comparable level of MP contamination in a heavily industrialized region located in the southern part of Chennai city, India, with an average of 2650 items per kg of the road dust sample. Liu et al.48 also discovered the highest concentration of MPs, 127
986 items per kg MPs in a stormwater retention pond within an industrial zone. In general, the second highest range was found in a commercial area. The lowest 540 items per kg MPs were detected from Fulton Road, which is entirely residential; there were no shops or other commercial activities. Most of the residential areas had low MPs due to general residential activities. Studies in Iran also found fewer MPs in road dust in the residential area than in the industrial area.20 MPs were found in different colors. Fibers discovered in road dust predominantly displayed red, black, white and green hues; while fragments exhibited a spectrum of colors including red, blue, black, brown, yellow, and transparent; films were primarily characterized based on shades of yellow and transparency, while microbeads presented a wide array of colors.49
3.1.2 Classification of road dust MPs based on shapes.
All possible common shapes, like fragments, fibers, microbeads and films, were identified in the studied road dust samples. The distribution of these shapes is presented in Fig. 1. The fragments at 60% were the dominant shape of MPs in industrial zones, followed by fibers at 21%, microbeads at 11% and films at 8%. Spherical fragments were detected mainly in the industrial road dust. Spherical elements are considered primary MPs, purposely manufactured for personal care products and medical and industrial goods.50 Fragments also originate from the epoxy powder coating process, commonly used in chemical, electronic and mechanical engineering production.51 The domination of fragments in industrial areas of the current study is conceivably due to all these activities. Synthetic textile products mainly release fibers13 through the manufacturing activity of the textile materials to the surrounding atmosphere, like in all the industrial areas. Plastic microbeads are widely utilized in the pharmaceutical industries to produce personal care products,52 which is why 11% of MPs in the microbead form were released in currently studied industrial zones. There were relatively fewer microbeads detected in the residential areas. The fiber percentage led in residential areas at 68%, followed by 19% fragments and 13% films. The domination of fiber in the residential areas is probably due to the synthetic clothes and furniture (e.g., carpets, bedding and curtains) used by the residents of those areas.53 There was no significant difference between the fragment and fiber percentages in commercial areas; fiber accounted for 46% of the MPs, and fragments for 30%, followed by 15% film and 8% microbeads. Several articles have confirmed the link between the high fiber and fragmented plastic dissemination in road dust with human events,47,54 where commercial areas are an excellent example of areas crowded with people with numerous activities. Films are usually derived from packaging materials, and they have the characteristic of reflecting light. Abbasi and Keshavarzi also found shiny MP films in road dust samples from Bushehr City, Iran, and stated that they originated from packaging materials.20
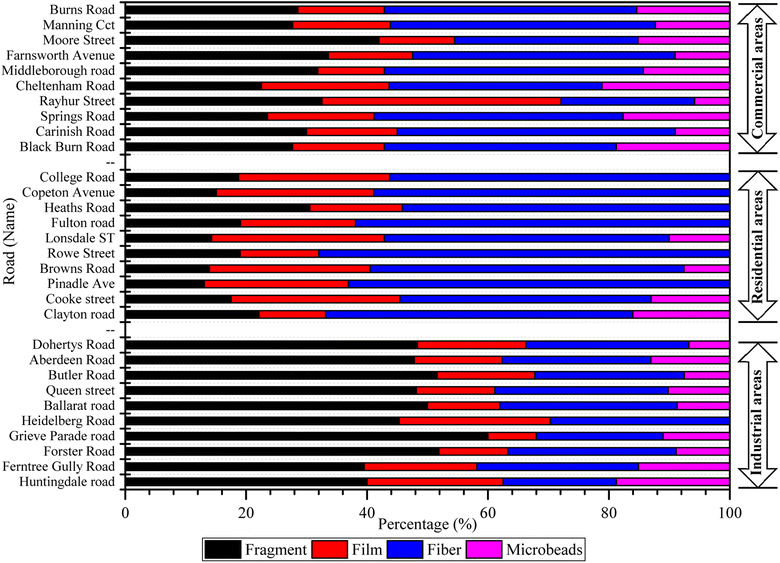 |
| Fig. 1 Distribution of total MPs in road dust based on their shapes, such as fibers, fragments, microbeads, and films. | |
3.1.3 FTIR analysis of road dust MPs.
FTIR analysis was performed on the uniquely appearing plastics identified through visual examination. Fig. 2 presents the major polymer types and additives observed in road dust samples collected from residential, industrial, and commercial roads. Overall, the spectral findings revealed various MP types in the road dust, including polyethylene (PE), polyethylene terephthalate/polyester (PET/PES), polypropylene (PP), polystyrene (PS), polyvinyl chloride (PVC), and macro rubber (Ma-R). Cuihe et al.,55 identified the prevalent polymers PP, PE and PET in the form of fibers or fragments of MPs in road dust samples, and their research highlighted the significant impact of traffic on MP pollution in urban areas, with MP abundance correlating with higher traffic density. PP and PE are major polymer types, constituting more than 50% of the total MPs. The breakdown of packaging and non-packaging materials (e.g., plastic containers, bottles, bags and toys) was one reason for the environmental contribution of PP and PE materials. The MP polymeric distribution in the industrial areas was PP (42%) > PE (18%) > PET/PES (15%) > PVC (10%) > Ma-R (8%) > PS (7%). A higher percentage of PP in industrial areas compared to residential and commercial areas was because PP is used for making plastic parts for machinery and equipment, as well as in the textile industry.56 According to Ken-Ichi and Haruhiko,46 PP was also the dominant MP in heavy traffic road dust in Tokyo, Japan. The composition of MPs in residential areas was found to be PET/PES (55%) > PP (22%) > PE (15%) > PS (8%). PES is used to produce motorized textiles and synthetics,57 significantly altering total MPs in residential sites. On the other hand, in commercial areas, PE was the most abundant (34%), followed by PP 25%, PET/PES (20%), PVC (8%), Ma-R (7%), and PS (5%). PVC is used for road markings and paints.56
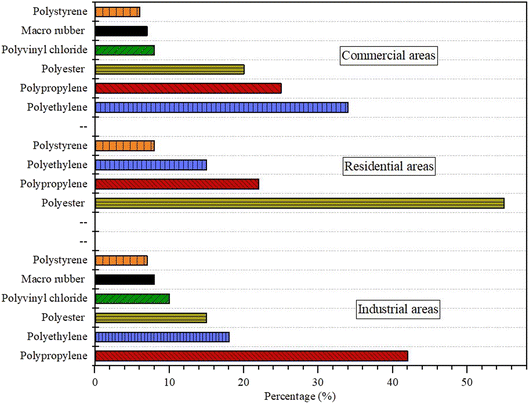 |
| Fig. 2 Major polymer types and additives in road dust samples from residential, industrial, and commercial roads. | |
The major peaks in the FTIR spectra of the red fragment, as shown in Fig. 3(a), were observed near 2916 cm−1 to 2849 cm−1, 1468 cm−1 and 724 cm−1. The sample spectra matched the reference polymer spectra, confirming the presence of a polyethylene group; FTIR analysis confirmed that the sample is a fragment of low-density polyethylene (LDPE). The peak at 1468–2849 cm−1 can be attributed to the stretching of the aromatic C–C bonds.58 The major peaks for the black fragment in Fig. 3(b) were located close to 2916 cm−1, 2845 cm−1, 1464 cm−1 and 714 cm−1, and indicated the presence of high-density polyethylene (HDPE). FTIR analysis confirmed that the microbeads in Fig. 3(c) are polystyrene, with peaks observed near 3025 cm−1, 2924 cm−1, 1601 cm−1, 1491 cm−1, 1448 cm−1 and 1030 cm−1. The spectral range between 1030 and 1448 cm−1 is indicative of the stretching vibrations associated with the C–O group in alcohol compounds.59 The red fiber in Fig. 3(d) was classified under the PE group, as confirmed by FTIR analysis. Previous studies have reported the presence of characteristic peaks in the polyethylene (PE) spectrum at 1466 cm⁻1, associated with carbonyl groups, and at 2913 cm⁻1, suggesting the presence of hydroxyl groups.60
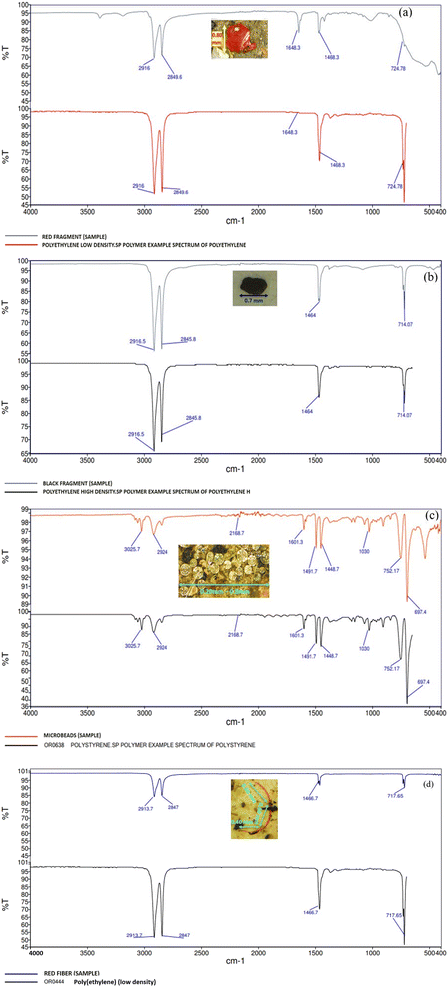 |
| Fig. 3 FTIR spectra of (a) low-density polyethylene (LDPE), (b) high-density polyethylene (HDPE), (c) polystyrene (PS), and (d) polyethylene (PE) detected in road dust samples. The upper line represents the spectra of MP samples in road dust, and the lower line represents the standard spectra for the reference polymer obtained from the library database. | |
3.1.4 SEM-EDX analysis of road dust MPs.
SEM-EDX analyses of road dust MPs were carried out to validate the identification of MPs. These analyses revealed the chemical composition of the MPs. Fig. S2(a)–(c)† present the SEM image of microbead, fiber and film particles, as well as their surface nature. The elemental composition can confirm the nature of the analyzed MP particles.61 Plastics are composed of higher percentages of carbon (C) and oxygen (O) and have minor percentages of additional elements.61,62 Therefore, the particles with strong carbon peaks are considered as MPs. The EDX analysis in Fig. S2(a)† showed the presence of basic elements such as magnesium (Mg), silicon (Si), sulphur (S), aluminium (Al), and chlorine (Cl) with calcium (Ca), C, O and sodium (Na) in the microbeads. Elements such as Cl, Ca, Mg, Si, Na and Al are typically used in plastic production and are derived from additives.63 Hence, the result established that the components are MP particles. Trace elements (e.g., Cr, Fe, Cu and Ti) are also commonly used as additives in plastic items to achieve the required properties, such as stiffness and color.64 The EDX analysis of the components in Fig. S2(b) and (c)† revealed the presence of different elements with strong C signals.
The major road dust associated MP polymers were found to be PE, PET/PES and PP. Stormwater runoff is a significant pathway through rain events for the introduction of road dust associated MPs into water environments. Hence, the consequence of road dust associated MPs in stormwater is evaluated in the following section.
3.2 Mapping of MP in stormwater under different land uses
3.2.1 Quantification of MPs in stormwater.
The colors of MPs are predominantly black, yellow, red, green, orange, brown, blue, and transparent. The MP concentrations in stormwater samples are calculated based on the number of particles per liter. The MP abundances in selected stormwater samples from industrial, commercial and residential areas averaged 35 items per L, 27 items per L and 24 items per L, respectively. The findings closely resemble those of a prior investigation in Denmark conducted by Liu et al.,63 wherein they reported the presence of MPs at around 23 items per L in water samples collected from urban and highway stormwater ponds.
The highest number of MPs, 43 items per L, was found in the Frogs Hollow Wetland, located near an industrial area. The lowest number of MPs, 17 items per L, was found in the Talbot Park wetland in a residential area. MPs were less abundant in residential areas due to lower levels of human activities and low traffic volume. Teresita et al.,65 also observed the highest MP concentration in industrial land use (191 items per L) compared to residential land use (158 items per L) in Tijuana, Mexico. Many studies have stated that MP abundance in stormwater is correlated with the vicinity of densely populated areas.66,67 MPs were found in red, blue, black, yellow, brown, and transparent colors.
3.2.2 Classification based on shape and FTIR analysis of stormwater MPs.
Four prevalent shapes of MPs, fragments, fibers, foams, and films, were identified from stormwater samples, as shown in Fig. 4. Fibers were the dominant MPs in most residential site samples, while fragments were prominent in industrial areas.
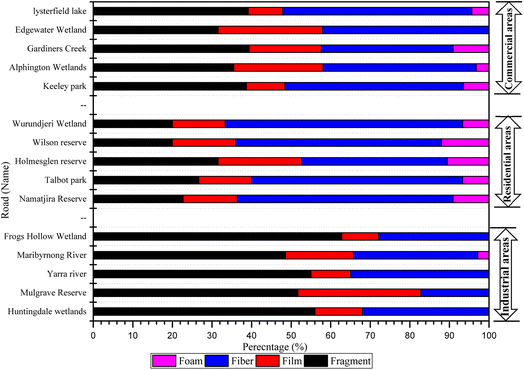 |
| Fig. 4 Distribution of total MPs in stormwater based on their shapes, such as fibers, fragments, foams, and films. | |
Fibers comprised 60% (highest) of the MPs in the Wurundjeri Wetland, near a densely populated residential area. In contrast, in the Holmesglen Reserve Wetland, a green, less populated, light-traffic residential area, fibers comprised 36% (lowest) of the total MPs. Synthetic fibers are torn and removed from indoor resources, including clothing and other soft indoor furnishings, such as carpets and curtains.68 The film-type MPs appear in packaging, cleaning products and containers.5,61 The samples from commercial areas showed fibers and fragments in close percentages (around 40%), and the films and foams were 15% and 4%, respectively. Fragments were higher in all industrial samples and highest at about 63% from the Frogs Hollow Wetland. Wenjiao et al.,69 also evaluated stormwater samples under different land uses in Wuhan, China, and found that fragments (45%) were more prevalent in high-traffic sites compared to fibre-shaped MPs (29%). Fragments can be generated from road markings, tyre abrasions, breakdown of larger plastics and various urban activities.70,71
FTIR analysis detected various polymers in stormwater samples, such as polyester, PE, PP, LDPE, HDPE and nylon. As shown in Fig. 5(a), the major peaks were near 2918 cm−1 and 2848 cm−1, and the cluster peaks were observed at 1463 cm−1 and 721 cm−1. This result confirmed that the film was made of LDPE, showing the same chemical bonds as in the reference polymer with functional groups. A navy-blue fragment (Fig. 5(b)) was found in the Alphington Wetland, and its FTIR analysis showed major peaks at 2967 cm−1, 1711 cm−1 and 1409 cm−1, and cluster peaks at 1240 cm−1 and 1094 cm−1, which confirms the sample to be PE. Fig. 5(c) shows the fiber sample found in the Namatjira Reserve Wetland, where major FTIR peaks were found at 3295 cm−1, 2920 cm−1, and 2849 cm−1 and cluster peaks at 1636 cm−1 to 720 cm−1, confirming that the fiber was polyamide nylon. The results shown in Fig. 6(a)–(c) are for all areas, where the polymer distribution of MPs in the industrial areas was PP (40%) > PE (27%) > PVC (15%) > PET/PES (10%) > Ma-R (8%). The PE was highest in both residential and commercial areas. The prevalence of these polymers matched the market's demand, with PP, PVC, PET, PE and PS collectively constituting over 50% of the total plastic production.72 The dominance of PE and PVC polymer types could be due to their application in packaging and manufacturing industries; PP and PET are used in manufacturing food containers, polyester fabric, textiles and reusable products.26,73
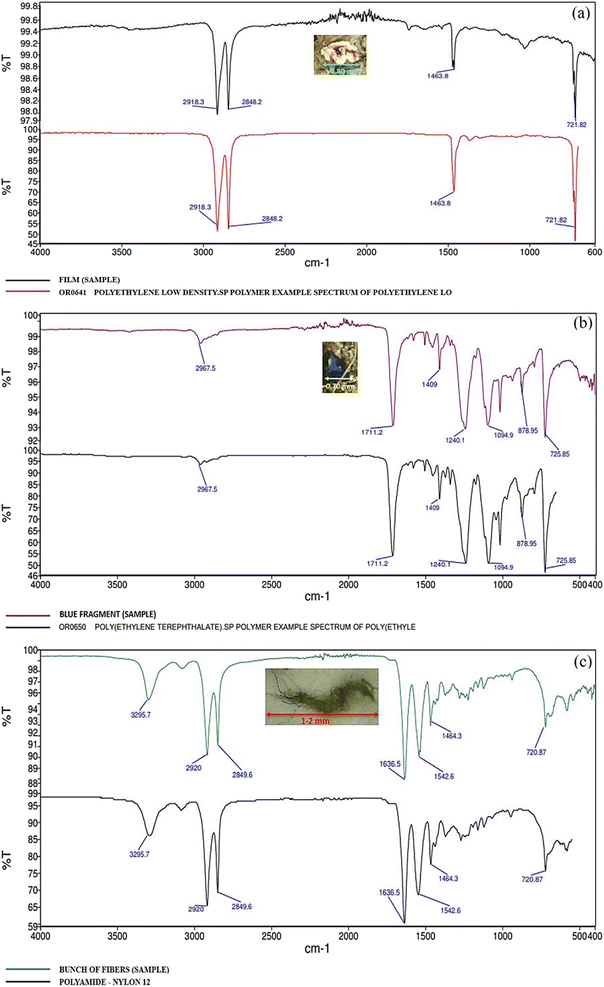 |
| Fig. 5 FTIR spectra of (a) low-density polyethylene (LDPE), (b) polyethylene (PE) and (c) polyamide detected in stormwater samples. The upper line represents the spectra of MP samples in stormwater, and the lower line represents the standard spectra for the reference polymer obtained from the library database. | |
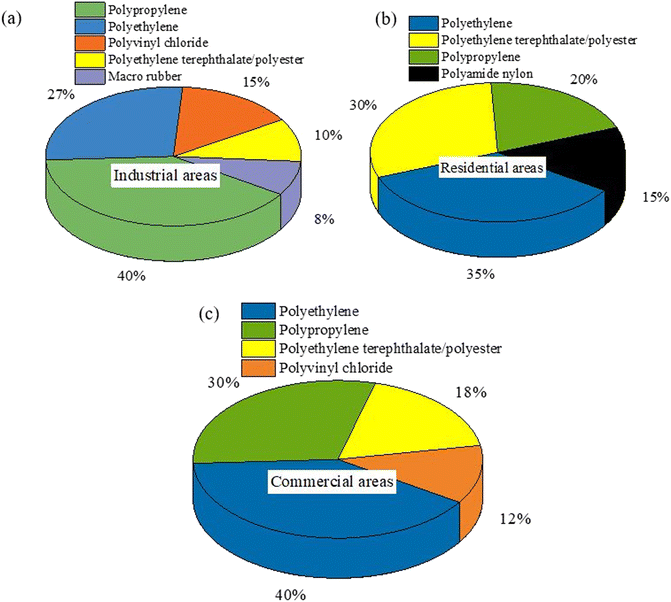 |
| Fig. 6 Major polymer types in stormwater samples from different wetlands in (a) industrial, (b) residential and (c) commercial areas. | |
3.2.3 SEM-EDX analysis of stormwater MPs.
SEM-EDX analysis results of fragments, fibers, and films are presented in Fig. S3(a)–(c).† SEM images revealed various surface roughnesses in different MPs, suggesting that the MPs have complex surface topography characteristics. Fragment and film SEM images, shown in Fig. S3(a) and (c)†, indicated that the surfaces are rough, cracked and damaged. Most fragment and film-type MPs had sharp edges.74 The fiber SEM image showed sharp and regular edges with a smooth morphology. EDX analysis revealed that fiber and film MPs were composed a high percentage of C and O, respectively, with minor percentages of other elements. Many plastics contain C and O, with the greatest percentage of other elements.62 EDX analysis of the fragment elements discovered in stormwater confirmed the presence of several elements such as Si, Ca, S, Fe, Mg, Cl and P, and these substances are used for plastic manufacture.63
3.3 Particle size analysis, MP mass concentrations, and the hazard index (Hi)
3.3.1 Particle size analysis and recovery rates of MPs in road dust and stormwater.
The particle size analysis results of MPs for both road dust and stormwater are given in Table 1 and Fig. S4.† The analysis of road dust MPs showed that 80% were within the range of 200 μm (D10) to 2450 μm (D90). A slightly different result was seen under the different land use and climate conditions in the Tehran metropolis, Iran, where the range was 250 μm to 500 μm for street road dust.24 In contrast, 80% of stormwater MPs were 125 μm (D10) to 960 μm (D90). This result is closer to the result reported by Lei et al.,75 in Taihu Lake, China, which was between 10 and 1000 μm. This result indicated that road dust contains larger-sized MPs than stormwater due to water forces in the drainage system, which could break down the large MPs and make their sizes smaller.35,76
Table 1 Particle size analysis of MPs identified in industrial, commercial, and residential road dust and stormwater samples
Sample |
MPs particle size, μm |
D (10) |
D (50) |
D (90) |
Road dust |
200 |
1120 |
2450 |
Stormwater |
125 |
430 |
960 |
The recovery rate of microplastics was investigated to assess the loss of microplastic particles during sample processing. The average recovery rate for the three processed samples was 90 ± 2.5. Pankkonen77 also found a comparable recovery rate of MPs across the three processed samples, with an average of 81 ± 2.2%, and deemed this result to be satisfactory. Recovery rates predominantly gauge the effectiveness of the extraction method, given that the attributes of the microplastic particles under examination do not encompass the complete range of diversity present in the sample, which includes variances in size, material composition and particle shape.78
3.3.2 Mass concentration of MPs.
Road dust loads of MPs from the industrial areas were higher than those from commercial and residential areas. Fragments were the most abundant shape, and PP was the highest polymer composition in the industrial areas. The mass-based loadings were calculated by converting the MP numbers to weights according to the shapes, sizes, and polymer densities.79 The mass-based MP load from the industrial area ranged from 2.46–6.90 g kg−1. The MP load in the residential area was 2.14–5.38 g kg−1, which was lower than that in the industrial and commercial (2.23–6.12 g kg−1) areas. The MP numbers, and sizes, percentages and densities of polymers in stormwater samples were also considered for mass calculation. The stormwater MP mass load from the industrial area was higher than that from residential and commercial areas. The highest mass was 0.1 g L−1 from industrial wetland samples, and the lowest was 0.03 g L−1 from residential wetland samples. Liu et al.72 found 182 ± 52 μg kL−1 MPs in residential stormwater retention ponds in Denmark, which was lower than the that in industrial ponds (593 ± 101 μg kL−1). Youna et al.42 also reported that MP loads were higher in industrial sites compared with residential sites in Gumi, South Korea.
3.3.3 Hazard index (Hi).
According to Kabir et al.45 the classification for the polymeric risk hazard indices (Hi) is specified as low when <150, medium when 150 to <300, considerable when 300 to <600, high from 600 to 1200, and very high when >1200. When considering the Hi values, as shown in Fig. 7, they were observed to not differ significantly in road dust and stormwater in all sampling sites. The results revealed that polymeric risk varied from classification considerable to high levels in Melbourne, Australia. For instance, the Hi values of the industrial and commercial areas are high (>600) due to MPs with high hazard scores for PVC and the numbers of PE and PP. PVC contains dangerous chemical additives, including phthalates, lead, cadmium and organotin, which can be toxic to human health. PVC is extensively utilized in industrial processes.80 The Hi is also substantially induced by PE and PP frequency and distribution at industrial and commercial sites in this study. These polymer plastics are commonly used in food packaging and reusable bags, including bottles, jars and films.81 The residential sampling sites with MP abundance reveal classification considerable Hi level values. These polymeric risks showed that the existence of toxic polymers causes higher hazard scores. Even though all the highly toxic polymers detected appeared in lower percentages than the other polymers, they still posed high risks due to their higher polymeric hazard scores. In contrast, the higher proportions of polymers with low hazard scores have developed lower polymeric risks.
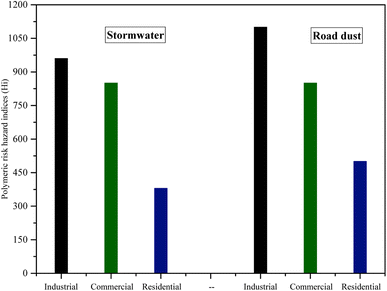 |
| Fig. 7 Polymeric risk hazard indices (Hi) of microplastics (road dust and stormwater) under different land uses. | |
4. Conclusions
Road dust and stormwater contaminated with MPs are causes for significant environmental and health concerns. MPs from the road dust and stormwater samples were evaluated in this study for their prevalence, characteristics, and health hazards. FTIR analysis facilitated the identification of several major polymers, such as PP, PE, PVC, PS and PES, comprising the MPs detected in the industrial, residential, and commercial road dust and stormwater samples. Moreover, using SEM-EDX to analyze the elements in the MP samples proved the existence of petroleum-based hydrocarbon polymers such as PE and PP. The concentration of MPs was higher in industrial site samples than in residential and commercial sites samples. This trend is due to high traffic density, expanding population, and various workshops, parking lots and business hubs in industrial areas. Fibers and fragments were identified as the two dominant shapes of MPs in all road dust and stormwater sample sites. The pollution risk assessment of MPs showed classification ranging from considerable to high levels in this study. A higher abundance of MPs and the presence of highly toxic polymers were the reason for the high pollution risks in the environment. The detected MP concentration, distribution, physicochemical properties, and estimated pollution risks posed eco-toxicological threats to the environment and living inhabitants. The evaluation of MP pollution risks in this research provided insights into pollution and a basis for further comprehensive environmental and human health risk assessments.
Future studies are essential for improving MP removal techniques before they enter open water. The cost-effectiveness of the MP removal processes compared with the conventional treatment method should be assessed in future research. There is also scope to research the mechanism of organic contaminant sorption to MPs under actual environmental conditions. Further investigation also requires understanding the fluxes and pathways of the MP cycle associated with road dust, and changeable characteristics of from the source to wetlands.
Author contributions
S. M. Alamgir Kabir: conceptualization, sample collection, formal analysis, investigation, methodology, validation, writing – original draft. Muhammed A. Bhuiyan: supervision, writing – review & editing. Guomin Zhang: supervision. Biplob Kumar Pramanik: supervision, writing – review & editing.
Conflicts of interest
The authors declare that there is no conflict of interest regarding the publication of this manuscript.
Acknowledgements
The authors entirely acknowledge the technical support provided by Analytical Chemistry facility, the Microscopy & microanalysis facility, and the X-ray facility at RMIT University. Dr Biplob Pramanik acknowledges the Australian Research Council for his Discovery Early Career Research 2023 Award (DE230101306).
References
-
D. D. Post, Holocene era to Plasticence? Pressreader, 2018 Search PubMed.
- C. M. Rochman, C. Brookson, J. Bikker, N. Djuric, A. Earn and K. Bucci,
et al., Rethinking microplastics as a diverse contaminant suite, Environ. Toxicol. Chem., 2019, 38(4), 703–711 CrossRef CAS PubMed.
- M. Ali, C.-P. Elena, H. Carla and B. Anne-Marie, Transport mechanisms and fate of microplastics in estuarine compartments: A review, Mar. Pollut. Bull., 2022, 177, 113553 CrossRef PubMed.
-
W. C. Li, The occurrence, fate, and effects of microplastics in the marine environment, Microplastic Contamination in Aquatic Environments, Elsevier, 2018, p. 133–173 Search PubMed.
- F. Wang, C. S. Wong, D. Chen, X. Lu, F. Wang and E. Y. Zeng, Interaction of toxic chemicals with microplastics: a critical review, Water Res., 2018, 139, 208–219 CrossRef CAS PubMed.
- E. Guzzetti, A. Sureda, S. Tejada and C. Faggio, Microplastic in marine organism: Environmental and toxicological effects, Environ. Toxicol. Pharmacol., 2018, 64, 164–171 CrossRef CAS PubMed.
- N. P. Ivleva, A. C. Wiesheu and R. Niessner, Microplastic in aquatic ecosystems, Angew. Chem., Int. Ed., 2017, 56(7), 1720–1739 CrossRef CAS PubMed.
- M. Eriksen, L. C. Lebreton, H. S. Carson, M. Thiel, C. J. Moore and J. C. Borerro,
et al., Plastic pollution in the world's oceans: more than 5 trillion plastic pieces weighing over 250,000 tons afloat at sea, PLoS One, 2014, 9(12), e111913 CrossRef PubMed.
-
A. A. K. Max Siegfried and E. Besseling, Carolien Kroeze Export of microplastics from land to sea. A modelling approach, Elsevier, Water Res., 2017, vol. 127, p. 249e257 Search PubMed.
-
J. Boucher, Primary Microplastics in the Oceans: A Global Evaluation of Sources, IUCN Gland, Switzerland, 2017, p. 46 Search PubMed.
-
P.-E. Sundt PS, F. Syversen, et al., Sources of Microplastics-Pollution to the Marine Environment, Mepex Consult AS, Asker, Norway, 2014, vol. 108, p. 108 Search PubMed.
- B. R. Kiran, H. Kopperi and S. Venkata Mohan, Micro/nano-plastics occurrence, identification, risk analysis and mitigation: challenges and perspectives, Rev. Environ. Sci. Biotechnol., 2022, 21(1), 169–203 CrossRef PubMed.
- R. Roychand and B. K. Pramanik, Identification of micro-plastics in Australian road dust, J. Environ. Chem. Eng., 2020, 8(1), 103647 CrossRef CAS.
-
C. Christopher Blair and Q. Brian, 5 - Microplastics, Standardisation and Spatial Distribution, 2017, pp. 101–130 Search PubMed.
- M. Cole, P. Lindeque, C. Halsband and T. S. Galloway, Microplastics as contaminants in the marine environment: a review, Mar. Pollut. Bull., 2011, 62(12), 2588–2597 CrossRef CAS PubMed.
- S. B. Kurniawan, N. S. M. Said, M. F. Imron and S. R. S. Abdullah, Microplastic pollution in the environment: insights into emerging sources and potential threats, Environ. Technol. Innov., 2021, 23, 101790 CrossRef CAS.
- I. E. Napper, A. Bakir, S. J. Rowland and R. C. Thompson, Characterisation, quantity and sorptive properties of microplastics extracted from cosmetics, Mar. Pollut. Bull., 2015, 99(1–2), 178–185 CrossRef CAS PubMed.
-
A. J. L. Pieter Jan Kole, F. G. A. J. Van Belleghem and A. M. J. Ragas, Wear and Tear of Tyres: A Stealthy Source of Microplastics in the Environment, Environmental Research and Public Health, 2017, p. 31 Search PubMed.
- A. L. Andrady, Microplastics in the marine environment, Mar. Pollut. Bull., 2011, 62(8), 1596–1605 CrossRef CAS PubMed.
- S. Abbasi, B. Keshavarzi, F. Moore, H. Delshab, N. Soltani and A. Sorooshian, Investigation of microrubbers, microplastics and heavy metals in street dust: a study in Bushehr city, Iran, Environ. Earth Sci., 2017, 76(23), 1–19 CAS.
- R. K. Khan and M. A. Strand, Road dust and its effect on human health: a literature
review, Epidemiol. Health, 2018, 40, 1–11 Search PubMed.
-
C. Vogelsang, A. Lusher, M. E. Dadkhah, I. Sundvor, M. Umar, S. B. Ranneklev, et al., Microplastics in road dust–characteristics, pathways and measures, NIVA-rapport, 2019 Search PubMed.
- M. Siegfried, A. A. Koelmans, E. Besseling and C. Kroeze, Export of microplastics from land to sea. A modelling approach, Water Res., 2017, 127, 249–257 CrossRef CAS PubMed.
- S. Dehghani, F. Moore and R. Akhbarizadeh, Microplastic pollution in deposited urban dust, Tehran metropolis, Iran, Environ. Sci. Pollut. Res., 2017, 24(25), 20360–20371 CrossRef CAS PubMed.
- R. Roychand and B. K. Pramanik, Identification of micro-plastics in Australian road dust, J. Environ. Chem. Eng., 2019, 8(1), 103647 CrossRef.
- S. Allen, D. Allen, V. R. Phoenix, G. Le Roux, P. Durántez Jiménez and A. Simonneau,
et al., Atmospheric transport and deposition of microplastics in a remote mountain catchment, Nat. Geosci., 2019, 12(5), 339–344 CrossRef CAS.
-
S. Monira, M. A. Bhuiyan, N. Haque and B. K. Pramanik, Assess the Performance of Chemical Coagulation Process for Microplastics Removal from Stormwater, Process Safety and Environmental Protection, 2021 Search PubMed.
- L. Su, B. Nan, N. J. Craig and V. Pettigrove, Temporal and spatial variations of microplastics in roadside dust from rural and urban Victoria, Australia: Implications for diffuse pollution, Chemosphere, 2020, 252, 126567 CrossRef CAS PubMed.
- Y. Zhen, P. Chenglei, L. Hengxiang, L. Lang, L. Shan and H. Rui,
et al., Atmospheric microplastics at a southern China metropolis: occurrence, deposition flux, exposure risk and washout effect of rainfall, Sci. Total Environ., 2023, 869, 161839 CrossRef PubMed.
- W. Yiting, D. Peng, X. Dongyu, Z. Yanliang and G. Bo, Microplastic reorganization in urban river before and after rainfall, Environ. Pollut., 2022, 314, 120326 CrossRef PubMed.
- P. K. Cheung, P. L. Hung and L. Fok, River microplastic contamination and dynamics upon a rainfall event in Hong Kong, China, Environ. Processes, 2019, 6(1), 253–264 CrossRef.
- C. J. Moore, G. Lattin and A. Zellers, Quantity and type of plastic debris flowing from two urban rivers to coastal waters and beaches of Southern California, J. Integr. Coast. Zone Manag., 2011, 11(1), 65–73 Search PubMed.
-
T. Exchange, Preferred Fiber & Materials Market Report 2019, Textile Exchange, 2019 Search PubMed.
- K. B. Olesen, D. A. Stephansen, N. van Alst and J. Vollertsen, Microplastics in a stormwater pond, Water, 2019, 11(7), 1466 CrossRef CAS.
- S. Monira, R. Roychand, M. A. Bhuiyan, F. I. Hai and B. K. Pramanik, Identification, classification and quantification of microplastics in road dust and stormwater, Chemosphere, 2022, 299, 134389 CrossRef CAS PubMed.
- H. Deng, R. Wei, W. Luo, L. Hu, B. Li and Y. Di,
et al., Microplastic pollution in water and sediment in a textile industrial area, Environ. Pollut., 2020, 258, 113658 CrossRef CAS PubMed.
- Y. Fang, L. Dandan, Z. Zixian, W. Ling, L. Siwan and H. En,
et al., Characteristics and the potential impact factors of microplastics in wastewater originated from different human activity, Process Saf. Environ. Prot., 2022, 166, 78–85 CrossRef.
- S. Abbasi, B. Keshavarzi, F. Moore, H. Delshab, N. Soltani and A. Sorooshian, Investigation of microrubbers, microplastics and heavy metals in street dust: a study in Bushehr city, Iran, Environ. Earth Sci., 2017, 76(23), 798 CrossRef.
- S. Ziajahromi, D. Drapper, A. Hornbuckle, L. Rintoul and F. D. Leusch, Microplastic pollution in a stormwater
floating treatment wetland: Detection of tyre particles in sediment, Sci. Total Environ., 2020, 713, 136356 CrossRef CAS PubMed.
- P. Biplob Kumar, R. Rajeev, M. Sirajum, B. Muhammed and J. Veeriah, Fate of road-dust associated microplastics and per- and polyfluorinated substances in stormwater, Process Saf. Environ. Prot., 2020, 144, 236–241 CrossRef.
- P. Fisher, C. Aumann, K. Chia, N. O'Halloran and S. Chandra, Adequacy of laser diffraction for soil particle size analysis, PLoS One, 2017, 12(5), e0176510 CrossRef PubMed.
- C. Youna, S. Won Joon, H. Sung Yong, H. Gi Myung, J. Mi and H. Sang Hee, Microplastic emission characteristics of stormwater runoff in an urban area: intra-event variability and influencing factors, Sci. Total Environ., 2023, 866, 161318 CrossRef PubMed.
- M. Ranjani, S. Veerasingam, R. Venkatachalapathy, M. Mugilarasan, B. Andrei and M. Vladimir,
et al., Assessment of potential ecological risk of microplastics in the coastal sediments of India: a meta-analysis, Mar. Pollut. Bull., 2021, 163, 111969 CrossRef CAS PubMed.
- L. Delilah, L. Åke and D. Göran, Environmental and health hazard ranking and assessment of plastic polymers based on chemical composition, Sci. Total Environ., 2011, 409(18), 3309–3324 CrossRef PubMed.
- A. H. M. E. Kabir, Assessing small-scale freshwater microplastics pollution, land-use, source-to-sink conduits, and pollution risks: Perspectives from Japanese rivers polluted with microplastics, Sci. Total Environ., 2021, 768, 144655 CrossRef CAS PubMed.
- K. Ken-Ichi and N. Haruhiko, Plastic additives as tracers of microplastic sources in Japanese road dusts, Sci. Total Environ., 2020, 736, 139694 CrossRef PubMed.
- A. Patchaiyappan, K. Dowarah, S. Z. Ahmed, M. Prabakaran, S. Jayakumar and C. Thirunavukkarasu,
et al., Prevalence and characteristics of microplastics present in the street dust collected from Chennai metropolitan city, India, Chemosphere, 2021, 269, 128757 CrossRef CAS PubMed.
- F. Liu, A. Vianello and J. Vollertsen, Retention of microplastics in sediments of urban and highway stormwater retention ponds, Environ. Pollut., 2019, 255, 113335 CrossRef CAS PubMed.
- M. Sirajum, A. B. Muhammed, H. Nawshad, S. Kalpit, R. Rajeev and I. H. Faisal,
et al., Understanding the fate and control of road dust-associated microplastics in stormwater, Process Saf. Environ. Prot., 2021, 152, 47–57 CrossRef.
- Y. Meral and Y. Ulaş, Use of a convolutional neural network for the classification of microbeads in urban wastewater, Chemosphere, 2019, 216, 271–280 CrossRef PubMed.
- Z. Du, S. Wen, J. Wang, C. Yin, D. Yu and J. Luo, The review of powder coatings, J. Mater. Sci. Chem. Eng., 2016, 4(3), 54–59 Search PubMed.
-
P. Sundt, P.-E. Schulze and F. Syversen, Sources of Microplastic-Pollution to the Marine Environment, Mepex for the Norwegian Environment Agency, 2014, vol. 86, p. 20 Search PubMed.
- R. Dris, J. Gasperi, V. Rocher, M. Saad, N. Renault and B. Tassin, Microplastic contamination in an urban area: a case study in Greater Paris, Environ. Chem., 2015, 12(5), 592–599 CrossRef CAS.
- H. Fakour, S.-L. Lo, N. T. Yoashi, A. M. Massao, N. N. Lema and F. B. Mkhontfo,
et al., Quantification and analysis of microplastics in farmland soils: characterization, sources, and pathways, Agriculture, 2021, 11(4), 330 CrossRef CAS.
- Y. Cuihe, N. Siping, X. Yanrong and W. Jing, Microplastics in urban road dust: Sampling, analysis, characterization, pollution level, and influencing factors, Trac. Trends Anal. Chem., 2023, 168, 117348 CrossRef.
- S. Yukioka, S. Tanaka, Y. Nabetani, Y. Suzuki, T. Ushijima and S. Fujii,
et al., Occurrence and characteristics of microplastics in surface road dust in Kusatsu (Japan), Da Nang (Vietnam), and Kathmandu (Nepal), Environ. Pollut., 2020, 256, 113447 CrossRef CAS PubMed.
-
C. Saricam and N. Okur, Polyester usage for automotive applications, Polyester-Production, Characterization and Innovative Applications London: IntechOpen, 2018, pp. 69–85 Search PubMed.
- S. M. Kharrazi, M. Soleimani, M. Jokar, T. Richards, A. Pettersson and N. Mirghaffari, Pretreatment of lignocellulosic waste as a precursor for synthesis of high porous activated carbon and its application for Pb (II) and Cr (VI) adsorption from aqueous solutions, Int. J. Biol. Macromol., 2021, 180, 299–310 CrossRef CAS PubMed.
- H. N. Tran, S.-J. You and H.-P. Chao, Fast and efficient adsorption of methylene green 5 on activated carbon prepared from new chemical activation method, J. Environ. Manag., 2017, 188, 322–336 CrossRef CAS PubMed.
- M. Zbyszewski and P. L. Corcoran, Distribution and degradation of fresh water plastic particles along the beaches of Lake Huron, Canada. Water, Air, & Soil Pollution, 2011, 220, 365–372 Search PubMed.
- M. J. Nematollahi, F. Zarei, B. Keshavarzi, M. Zarei, F. Moore and R. Busquets,
et al., Microplastic occurrence in settled indoor dust in schools, Sci. Total Environ., 2022, 807, 150984 CrossRef CAS PubMed.
- R. M. Blair, S. Waldron, V. R. Phoenix and C. Gauchotte-Lindsay, Microscopy and elemental analysis characterisation of microplastics in sediment of a freshwater urban river in Scotland, UK, Environ. Sci. Pollut. Res., 2019, 26, 12491–12504 CrossRef PubMed.
- S. Liu, M. Jian, L. Zhou and W. Li, Distribution and characteristics of microplastics in the sediments of Poyang Lake, China, Water Sci. Technol., 2019, 79(10), 1868–1877 CrossRef CAS PubMed.
- M. J. Nematollahi, F. Moore, B. Keshavarzi, R. D. Vogt, H. N. Saravi and R. Busquets, Microplastic particles in sediments and waters, south of Caspian Sea: frequency, distribution, characteristics, and chemical composition, Ecotoxicol. Environ. Saf., 2020, 206, 111137 CrossRef CAS PubMed.
- P.-C. Teresita de Jesus, R.-J. Ruben, R.-H. Eduardo, A.-A. Adriana and W. Fernando Toyohiko, Microplastics in stormwater runoff in a semiarid region, Tijuana, Mexico, Sci. Total Environ., 2020, 704, 135411 CrossRef PubMed.
- A. R. A. Lima, M. Barletta and M. F. Costa, Seasonal distribution and interactions between plankton and microplastics in a tropical estuary, Estuar. Coast Shelf Sci., 2015, 165, 213–225 CrossRef.
- H. A. Nel, J. W. Hean, X. S. Noundou and P. W. Froneman, Do microplastic loads reflect the population demographics along the southern African coastline?, Mar. Pollut. Bull., 2017, 115(1–2), 115–119 CrossRef CAS PubMed.
- Q. Zhang, Y. Zhao, F. Du, H. Cai, G. Wang and H. Shi, Microplastic fallout in different indoor environments, Environ. Sci. Technol., 2020, 54(11), 6530–6539 CrossRef CAS PubMed.
- S. Wenjiao, C. Zhiyi, M. Longjie, H. Shiwen, Z. Cheng and Z. Wen bin,
et al., The abundance and characteristics of microplastics in rainwater pipelines in Wuhan, China, Sci. Total Environ., 2021, 755, 142606 CrossRef PubMed.
- K. Unice, M. Weeber, M. Abramson, R. Reid, J. van Gils and A. Markus,
et al., Characterizing export of land-based microplastics to the estuary-Part I: application of integrated geospatial microplastic transport models to assess tire and road wear particles in the Seine watershed, Sci. Total Environ., 2019, 646, 1639–1649 CrossRef CAS PubMed.
- V. Shruti, F. Pérez-Guevara, I. Elizalde-Martínez and G. Kutralam-Muniasamy, Current trends and analytical methods for evaluation of microplastics in stormwater, Trends Environ. Anal. Chem., 2021, 30, e00123 CrossRef CAS.
- L. Fan, O. Kristina Borg, B. Amelia Reimer and V. Jes, Microplastics in urban and highway stormwater retention ponds, Sci. Total Environ., 2019, 671, 992–1000 CrossRef.
- G. Erni-Cassola, V. Zadjelovic, M. I. Gibson and J. A. Christie-Oleza, Distribution of plastic polymer types in the marine environment; a meta-analysis, J. Hazard Mater., 2019, 369, 691–698 CrossRef CAS PubMed.
- V. Hidalgo-Ruz, L. Gutow, R. C. Thompson and M. Thiel, Microplastics in the marine environment: a review of the methods used for identification and quantification, Environ. Sci. Technol., 2012, 46(6), 3060–3075 CrossRef CAS PubMed.
- S. Lei, X. Yingang, L. Lingyun, Y. Dongqi, K. Prabhu and L. Daoji,
et al., Microplastics in Taihu Lake, China, Environ. Pollut., 2016, 216, 711–719 CrossRef PubMed.
- B. K. Pramanik, S. K. Pramanik and S. Monira, Understanding the fragmentation of microplastics into nano-plastics and removal of nano/microplastics from wastewater using membrane, air flotation and nano-ferrofluid processes, Chemosphere, 2021, 282, 131053 CrossRef CAS PubMed.
-
P. Pankkonen, Urban Stormwater Microplastics–Characteristics and Removal Using a Developed Filtration System, 2020 Search PubMed.
- M. Simon, N. van Alst and J. Vollertsen, Quantification of microplastic mass and removal rates at wastewater treatment plants applying Focal Plane Array (FPA)-based Fourier Transform Infrared (FT-IR) imaging, Water Res., 2018, 142, 1–9 CrossRef CAS PubMed.
- S. Eo, S. H. Hong, Y. K. Song, G. M. Han and W. J. Shim, Spatiotemporal distribution and annual load of microplastics in the Nakdong River, South Korea, Water Res., 2019, 160, 228–237 CrossRef CAS PubMed.
- A. L. Andrady and M. A. Neal, Applications and societal benefits of plastics, Philos. Trans. R. Soc. Lond. B Biol. Sci., 2009, 364(1526), 1977–1984 CrossRef CAS PubMed.
- M. K. Nguyen, C. Lin, N. T. Q. Hung, D.-V. N. Vo, K. N. Nguyen and B. T. P. Thuy,
et al., Occurrence and distribution of microplastics in peatland areas: a case study in Long An province of the Mekong Delta, Vietnam, Sci. Total Environ., 2022, 844, 157066 CrossRef CAS PubMed.
|
This journal is © The Royal Society of Chemistry 2024 |
Click here to see how this site uses Cookies. View our privacy policy here.