DOI:
10.1039/D2EA00041E
(Critical Review)
Environ. Sci.: Atmos., 2022,
2, 921-942
Ecological and human health risks of atmospheric microplastics (MPs): a review
Received
8th April 2022
, Accepted 29th July 2022
First published on 12th August 2022
Abstract
Microplastics (MPs) in the atmosphere are ubiquitous and persistent emerging pollutants which have been an environmental issue of global concern. This overview summarizes the sources, morphological size and compositional characteristics, spatial–temporal distributions, transport and fates, and both ecological and human health risks of MPs in the atmospheric environment. The results suggest that atmospheric MPs could be long-range transported and deposited into aquatic and terrestrial habitats, which pose ecological risks through bio-absorption, bio-concentration, bio-accumulation, ecotoxicity and associated chemical pollution. Since a large number of small-sized MPs are present in the atmosphere, exposure to them affects ingestion, metabolism and reproduction. Human exposure to atmospheric MPs by inhalation may induce health risks, including oxidative stress and inflammatory damage. Moreover, atmospheric MPs may also serve as carriers for other harmful chemical pollutants like heavy metals. Finally, further research studies on atmospheric MPs are recommended for future work.
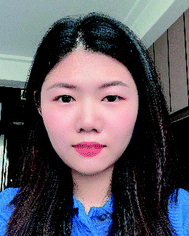 Xuewen Yao | Xuewen Yao is a PhD candidate at Nanjing University of Information Science and Technology, with a masters degree in Ecology, and she is currently studying for a doctorate in Environmental Ecology. She is interested in the sources and behavior of atmospheric microplastics and their risks to human health. |
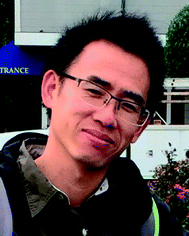 Xiao-San Luo | Xiao-San Luo is a Professor of Environmental Science at the Nanjing University of Information Science & Technology, where he leads a research group in Environmental Geoscience & Health. His research interests include human health risks of atmospheric particulate pollution and emerging contaminants such as microplastics, environmental persistent free radicals, etc. After earning his PhD from the Institute of Soil Science, Chinese Academy of Sciences (CAS), he worked in the Institute of Urban Environment, CAS, and completed a postdoctoral fellowship in the Department of Civil and Environmental Engineering, The Hong Kong Polytechnic University. Then as a scholar he also visited ETH Zürich, and Air Quality Research Center, Center for Health and the Environment, University of California, Davis. |
Environmental significance
Microplastics have been detected in the atmosphere of cities, suburbs, and remote areas (snowy mountains, sea), indicating that MPs can be transported over long distances in the atmosphere. MPs can cause pollution in the atmospheric environment. Therefore, it is necessary to illuminate the origin, distribution, environmental fate and risks of MPs in the atmosphere. As a global environmental pollution issue, atmospheric MPs impact both ecosystems and human beings. This review explores the ecological risks and human health risks posed by various MPs in the atmosphere.
|
1 Introduction
Plastic pollution has become a global threat. The global demand for plastics has grown exponentially, with the global production increasing to 368 million tons in 2019.1 Despite a growing number of strategies used to reduce plastic,2,3 55% of the world's plastic was discarded, followed by being incinerated (25%) and recycled (20%) in 2015.4 Nevertheless, large amounts of plastic still accumulate in the environment.5 Plastic particles are released from plastic products during usage and handling due to physical, chemical, and biological processes. Plastic particles of less than 5 mm in size are often referred to as microplastics (MPs)6 (Fig. 1).
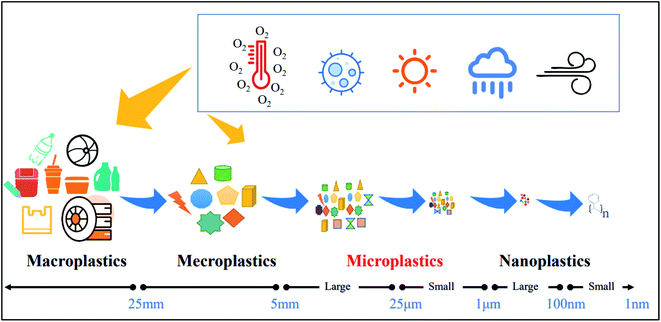 |
| Fig. 1 Definition of plastic size classes. | |
MPs enter ecosystems mainly through human activities. MPs are found in soil, air, and water environments.7 As an emerging pollutant, it has aroused widespread concern in the society. MPs can potentially migrate and physically transfer nutrients horizontally in the environment.8,9 MPs in the atmosphere are mostly transported by wind and enter the water and soil environments via dry and wet deposition.10 MPs can travel via wind, soil, and water streams all the way to the ocean.11,12 MPs in the soil environment can travel short distances through bioturbation and agricultural activities,13 re-enter the atmosphere through wind, and enter the water environment through rain.14 This illustrates that MPs can move in environments with different media.15,16 Several studies have been carried out on the sources of MPs and analytical methods for their detection.17–19 More attention is being paid to MPs present in the aquatic and terrestrial environments.15,20 MPs may be ingested and accumulated in organisms, affecting the survival, growth, reproduction, feeding and immune system of organisms.13,21,22 MPs enter the human body mainly through ingestion, inhalation and skin absorption. MPs have significant negative effects on human health.23 Atmospheric MPs are considered to be an important pathway for biological and human ingestion and inhalation, resulting in ecological risks and human health risks.24,25 Although there has been an increase in studies on atmospheric MPs in recent years, more studies on their sources, transport, and fates are needed.
The purpose of this review is to provide insight into the occurrence of MPs in the atmospheric environment, focusing on (a) using collected data to summarize the sources, morphological size and compositional characteristics, spatial–temporal distribution, transport and fates of MPs in the atmospheric environment; (b) discussing the ecological risks of atmospheric MPs; (c) exploring the toxicological effects of atmospheric MPs on human health.
2 Microplastics in the environment
2.1 Definition of MPs
Plastic is a very important organic synthetic polymer material. Plastics are processed into various colors, sizes, and shapes according to the different needs. In addition, plastics have wide applications owing to their unique physical and chemical properties, such as durable, water-proof, lightweight, and corrosion-resistant nature. Therefore, statistics show that about 6300 metric tons (Mt) of plastic waste had been produced by 2015. However, only around 9% of them have been recycled, and 12% have been incinerated. The remaining 79% of plastics were deposited in landfills or into the natural environment.26
MPs are synthetic polymers with diameters less than 5 mm.27 MPs have different colors, compositions, sizes, and shapes. They are found in diverse forms, including spheres, fragments, and fibers.28 According to sources, they can be divided into primary MPs and secondary MPs. Primary MPs refer to plastics smaller than 5 mm that are directly generated during production and eventually released into the environment. The primary sources of MPs mainly include cosmetics, personal skin care products, products related to the medical field and water-based coatings.1,11 Large plastic products or wastes exposed to the natural environment are broken into tiny plastic particles under the influence of physical, chemical and biological forces to form secondary microplastics,29 such as debris generated by mulch breakage, particles generated by tire wear, and so on. Plastics and MPs undergo degradation to form nanoplastics (NPs). The large and diverse MPs are found in aquatic,30 terrestrial31,32, and atmospheric environments.33,34 MPs have a long residence time in the environment because it is difficult to degrade them therefore this causes extensive and serious environmental pollution.
2.2 MPs in the atmosphere
MP pollution has gradually become an environmental issue that has attracted global attention. The number of publications on MPs has increased rapidly in the recent decade (Fig. 2). However, most published research focuses on their occurrence, transport, and ecological risks in the aquatic35,36 and terrestrial37–39 environments. There are few studies on MPs in the atmosphere, and the initial research began in 2015.40 MPs in the atmosphere are potentially important pollutants in urban and industrial environments, which receive rare attention. In the next few years, research on atmospheric MPs will increase.
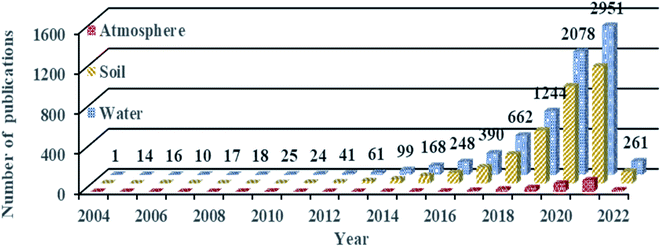 |
| Fig. 2 The number of papers containing MPs related to the atmosphere, soil and water, respectively. The statistics are the total number of publications containing MPs each year (Data from Web of Science). | |
2.3 Technologies for the collection and analysis of atmospheric MPs
The choice of sampling method affects the detection of MPs in the atmosphere. Passive atmospheric deposition or vacuum pump samplers are mainly used.41,42 The atmospheric deposition sampling method is suitable for continuous sample collection over a long period. Active vacuum pump samplers can be used to collect total suspended particulate matter (TSP) found in the atmosphere.43
Atmospheric MPs have been identified and quantified by macroscopic, microscopic, and spectroscopic techniques.44–46 The size of MPs detectable by physical characterization methods such as vision and microscopy is limited. Although physical characterization methods such as infrared spectroscopy and Raman spectroscopy are more conducive to identifying common MPs with smaller sizes (down to 50 nm), they cannot identify the color and shape of MPs.47 A growing body of research focuses on the identification and quantification of NPs in the environment.48,49 Several mass spectrometry (MS)-based methods have been proposed to quantify and characterize polymers commonly found in MPs and NPs.50 MS is well suited for the determination of MPs and NPs in air samples with small sample volumes that do not require complex digestion and separation,51 but the number of polymers that can be determined is still too small.52 MP samples can be destroyed during Raman spectroscopy identification. There are currently no well-established techniques for identifying smaller-sized MPs. There are also few studies that have measured NPs in actual samples.53 The presence of large amounts of NPs in the atmosphere has not been identified and quantified. There is an urgent need to understand the presence of NPs in the atmospheric environment and their toxicological effects on living organisms.
3 Atmospheric MP pollution
3.1 Occurrence and distribution of MPs in the atmospheric environment
Currently, the sample types of MPs in the atmospheric environment examined include total atmospheric fallout, indoor and outdoor air, snow, and street dust. Few studies investigated the occurrence and dispersion of airborne MPs in limited sites across three continents: Europe, North America, and Asia. Since 2015, most research has focused on the composition, properties, and abundance of atmospheric MPs40 (Tables 1 and 2). These studies suggest that sources and transport factors simultaneously influence the spatial distribution of atmospheric MPs.
Table 1 Available data reported on concentrations, physico-chemical characteristics and abundance of MPs in outdoor air worldwide
Environmental medium |
Continent |
Study area |
MP abundance |
MP composition |
MP size (μm) |
MP shape |
MP color |
Ref. |
Total atmospheric fallout (wet and dry deposition) |
Europe |
Paris, France |
29–280 particles per m2 per day |
N/A |
100–5000 |
Fiber: 90%, fragment: 10% |
N/A |
Dris et al.,40 2015 |
Paris, France |
2–355 particles per m2 per day (urban: 110 ± 96, sub-urban: 56 ± 38) |
PET, PUR, PA, RY |
50–600 |
Fiber |
N/A |
Dris et al.,57 2016 |
Pyrenees, France |
365 ± 69 particles per m2 per day |
PS, PE, PP, PVC, PET |
5–3000 |
Fragment, film, fiber |
Transparent, white, bright orange, blue, green, purple, black |
Allen et al.,86 2019 |
Hamburg, Germany |
136.5–512.0 particles per m2 per day |
PE, EVAC, PTFE, PVA, PET |
<63–5000 |
Fiber, fragment |
N/A |
Klein and Fischer,67 2019 |
London, England |
575–1008 microplastics per m2 per day |
PAN, PES, PA, PP, PVC, PE, PET, PS, PUR, AC, Pol. Petr. Res |
20–2899 |
Fiber, film, fragment, granule, foam |
N/A |
Wright et al.,61 2019 |
Asia |
Dongguan, China |
175–313 particles per m2 per day |
PE, PP, PS |
200–4200 |
Fiber, foam, fragment, film |
N/A |
Cai et al.,54 2017 |
Yantai, China |
1.46 × 105 particles per m2 per year |
PE, PVC, PS, PES |
50–5000 |
Fiber, foam, fragment, film |
White, black, red, transparent |
Zhou et al.,55 2017 |
Guangzhou, China |
51–178 plastics per m2 per day |
PET, PAN, PP, PA, PS, ALK, EP, ABS |
50–5000 |
Fiber, fragment, film, microbead |
Transparent/white, blue, yellow, red, orange, green, gray |
Huang et al.,87 2021 |
Snow |
Europe |
Swiss Alps and Bremen, Bavaria |
Arctic snow: 0–14.4 × 103 N per liter, European snow: 0.19 × 103 154 × 103 N per liter |
Varnish, nitrile rubber, PE, PA |
11–475 |
Fiber |
N/A |
Bergmann et al.,10 2019 |
Street dust |
Asia |
Tehran, Iran |
83–605 ± 10 particles per 30 g |
N/A |
100–5000 |
Fiber, granule, sphere, hexagonal, irregular polyhedron |
Transparent, red, green, blue, white, black, yellow, brown, pink, orange |
Dehghani et al.,59 2017 |
|
|
Asaluyeh County, Iran |
Industry: 60 particles per g, urban: 16 particles per g |
N/A |
≤5000 |
Spherule, fiber, fragment, film |
White-transparent, yellow-orange, black, red-pink, blue-green, black-grey |
Abbasi et al.,65 2019 |
|
|
Kusatsu, Japan |
2.0 ± 1.6 pieces per m2 |
PE, PP, PS, PET, PAK, PVS, EPC, SBR, EPDM, PU |
1400 ± 947 |
N/A |
N/A |
Yukioka et al.,76 2020 |
|
|
Da Nang, Vietnam |
19.7 ± 13.7 pieces per m2 |
PE, PP, PS, PET, PAK, PVS, EPC, SBR, EPDM, PU |
791 ± 530 |
Fragment, film/sheet, line/fiber, granule |
Black, gray, red, yellow, brown, white/transparent, blue, green |
Yukioka et al.,76 2020 |
|
|
Kathmandu, Nepal |
12.5 ± 10.1 pieces per m2 |
PE, PP, PS, PET, PAK, PVS, EPC, SBR, EPDM, PU |
907 ± 675 |
Fragment, film/sheet, line/fiber, granule |
Black, gray, red, yellow, brown, white/transparent, blue, green |
Yukioka et al.,76 2020 |
Total suspended particulates (TSP) |
Asia |
Shanghai, China |
0–4.18 items per m3 |
PET, PE, PES, PAN, PAA, RY, EVA, EP, ALK |
23.1–9555 |
Fiber, granule, fragment |
Black, blue, red, transparent, brown, green, yellow, grey |
Liu et al.,68 2019a |
West Pacific Ocean |
0–1.37 items per m3 |
PET, PE, PP, PE-PP, PES, PAN, PMA, EP, ALK, Phe, PS, PVA, PVC, PA |
20–2000 |
Fiber, granule, fragment, microbead |
Black, blue, gray, brown, yellow, red, orange, pink, purple, white, transparent, green |
Liu et al.,62 2019b |
Shanghai, China |
0.05–0.07 items per m3 |
PET, EP, PE, ALK, RY, PP, PA, PS |
12–2191 |
Fiber, fragment |
Black, blue, green, yellow, brown, transparent, white, pink, purple |
Liu et al.,69 2019c |
North Atlantic Ocean |
N/A |
PS, PE, PP, PDMS |
>5 |
N/A |
N/A |
Trainic et al.,71 2020 |
Karimata Strait |
0–0.8 items per 100 m3 |
PET |
382.2 |
Fiber |
Black |
Wang et al.,63 2020 |
Pearl River Estuary |
3–7.7 items per 100 m3 |
PET, PP, PA, PEP |
288.2–11182 |
Fiber |
Black, white, red, yellow, brown |
Wang et al.,63 2020 |
South China Sea |
0–3.1 items per 100 m3 |
PP, PET, PEVA |
286.1–1862 |
Fiber, fragment |
Black, red, yellow |
Wang et al.,63 2020 |
East India Ocean |
0–0.8 items per 100 m3 |
PET, PP, PAN-AA, PR |
58.6–988.4 |
Fiber, fragment |
Black, yellow, Blue |
Wang et al.,63 2020 |
|
|
Five megacities of Northern and Southeast China |
Northern cities: 358 ± 132 items per m3, South-east cities 230 ± 94 items per m3 |
PE, PES, PS, PP, PA, PVC |
5.9–1475 |
Fragment, fiber |
N/A |
Zhu et al.,72 2021 |
|
|
Sakarya, Turkey |
N/A |
PA, PUR, PE, PP, PES |
≤500 |
Fiber, fragment |
Dark blue, black, blue, brown, white, transparent |
Kaya et al.,60 2018 |
Table 2 Available data reported on concentrations, physico-chemical characteristics and abundance of MPs in indoor air worldwidea,b
Environmental medium |
Continent |
Study area |
MP abundance |
MP composition |
MP size (μm) |
MP shape |
MP color |
Ref. |
N/A: not available/reported.
ABS: acrylonitrile butadiene styrene, AC: acrylic, ALK: alkyl resin, EP: epoxy resin, EPC: ethylene/propylene copolymer, EPDM: ethylene/propylene/diene rubber, EVA/EVAC: ethylene-vinyl acetate copolymer, NY: nylon, PA: polyamide, PAA: polyacrylic acid, PAK: polyacrylate, PAN: polyacrylonitrile, PC: polycarbonate, PDMS: polydimethylsiloxane, PE: polyethylene, PEP: poly(ethylene-co-propylene), PES: polyester, PET: polyethylene terephthalate, Phe: phenoxy resin, PMA: poly(N-methyl acrylamide), Pol. Petr. Res: polymerized petroleum resin, PP: polypropylene, PS: polystyrene, PTFE: polytetrafluoroethylene, PU/PUR: polyurethane, PVA: poly(vinyl acetate), PVC: polyvinyl chloride, PVS: polyvinyl stearate, RY: rayon, SBR: styrene/butadiene rubber.
|
Indoor and outdoor air |
Europe |
Paris, France |
Indoor: 1.0–60.0 fibers per m3, outdoor: 0.3–1.5 fibers per m3 |
PP, PA, PET, RY |
Indoor: <3250, outdoor: <1650 |
Fiber |
N/A |
Dris et al.,58 2017 |
America |
California, America |
Indoor: 3.3 ± 2.9 fibers per m3, 12.6 ± 8.0 fragments per m3, outdoor: 0.6 ± 0.6 fibers per m3, 5.6 ± 3.2 fragments per m3 |
PE, PET, PS, PVA, AC |
Indoor: 58.6 ± 55, outdoor: 104.8 ± 64.9 |
Fiber, fragment |
N/A |
Gaston et al.,56 2020 |
Asia |
39 major cities in China |
Indoor fiber: 17–620 fibers per mg, indoor granule: 6–184 particles per mg, outdoor fiber: 7–431 fibers per mg, out-door granule: 0–100 particles per mg |
PET, PAN, NY, PE, PP, AC, PU, alkyd |
50–2000 |
Fiber, granule |
N/A |
Liu et al.,66 2019d |
Indoor airborne |
Europe |
Aarhus, Denmark |
1.7–16.2 particles/m3 |
PES, PE, NY, PP |
11–400 |
Fragment, fiber |
N/A |
Vianello et al.,70 2019 |
Asia |
Shanghai, China |
Dormitory: 9.9 × 103 MPs per m2 per day, office: 1.8 × 103 MPs per m2 per day, corridor: 1.5 × 103 MPs per m2 per day |
PES, RY, AC, cellophane, PP, PS, PA |
50–2000 |
Fiber |
Transparent, blue, red, black, yellow, purple, green |
Zhang et al.,64 2020 |
Oceania |
Metropolitan Sydney, Australia |
22–6169 fibres per m2 per day |
PE, PES, PET, PA, PVC |
50–5000 |
Fiber (99%), fragment, film |
Black, green, blue, red, grey, brown, transparent |
Soltani et al.,156 2021 |
3.1.1 Chemical compositions of atmospheric MPs.
MPs are composed of natural and synthetic polymers in the atmospheric environment.
Regarding the composition of fibers in atmospheric environments, the vast majority are made of natural materials, such as cotton, cellulose acetate, and a small amount of wool. The remaining few fibers contain plastic polymers, one of which is a blend of polyamide (nylon) and cotton and the other fully synthetic. Many types of synthetic polymers have been detected in atmospheric MPs. The most common types include polyethylene (PE), polyethylene terephthalate (PET), polypropylene (PP), polystyrene (PS), and polyamide (PA). These synthetic polymers are utilized for various items. For example, PE and PP are mainly used in plastic bags, containers and films, PET for beverage and drinking water bottles, PS for fast food boxes, and PA for engineering plastics.
The distribution of MP types in the air is influenced by the emission type, climatic conditions, and site topography, such as the compositions of MPs measured in different cities of China. The main types of atmospheric MPs measured in Dongguan city54 are PE, PP, and PS, while in the same year, the types of MPs measured in Yantai city55 mainly included PE, PVC, PS, and PES.
The current determination of the composition of MPs in the atmosphere is related to technology. Micro-Raman and FT-IR paint slightly different pictures of plastic compounds in the air.56 Micro-Raman shows that PVC dominates indoor air followed by PE, while FT-IR showed the predominance of PS followed by PE and PET. Therefore, the development of instrumental technology is crucial to the correct ongoing composition analysis of MPs in the atmosphere.
3.1.2 Morphological characteristics of atmospheric MPs.
(1) Size.
Plastics with a particle size of less than 5 mm are usually called MPs. The early study published regarding the size of MPs in the atmosphere40 provided knowledge on the 100–5000 μm range in the total atmospheric fallout. So far, the generally reported size of atmospheric MPs ranged widely from 4 to 5000 μm. Limited by the available methods, the 2015–2018 studies40,54,55,57–60 on the size measurements were not precise enough. With the development of qualitative and quantitative techniques for MPs, the research on the size of MPs has gradually narrowed to less than 2000 μm. MPs' size limits were often operationally defined by sampling and analysis methods. MPs down to 10
μm could be identified,61 expanding the range of detectable size reported by previous studies on air samples. Many fluorescent non-fibrous particles <20 μm and smaller fibers were observed in the total atmospheric deposition in central London,61 indicating the existence of smaller MPs in the atmosphere worthy of further study. These studies showed that MPs constitute a non-negligible fraction of atmospheric particulate matter, which can be inhaled and ingested. The studies also indicate that inhalation of MPs in the air is likely to have negative effects on human health.
There are also differences in the size of indoor and outdoor MPs in the same location, and different scholars hold different views on this topic. In a study of MPs in indoor and outdoor air in Paris,58 France, no fibers larger than 3250 μm were observed in indoor air, while in outdoor air, it was always smaller than 1650 μm. No significant difference in fiber length was found between indoor and outdoor air in California,56 but their data suggest that indoor fragments are half the size of outdoor ones. The size of MPs in indoor and outdoor air in Paris is larger than in California, as seen from data from the two cities, indicating that MPs in different cities may be different.
The study of total suspended MP particles in the West Pacific Ocean62 pointed out that the size of MPs was 20–2000 μm. The size of the MPs collected over the South China Sea and the Eastern Indian Ocean is less than 2000 μm.63 Therefore, MPs of larger size find it difficult to suspend into the air and even transmit to a longer distance.
(2) Color.
MPs are composed of various colors, including white, black, red, and transparent (Fig. 3b). Judging from the reported situation, black, blue, and white account for the main part of atmospheric MPs. Color is generally considered helpful in the initial visual assessment of atmospheric MP samples. Black MPs are the most abundant, accounting for 29.9% of all atmospheric MPs in the streets of the Tehran metropolitan area.59 We might infer from this that tire crumbs make up a large proportion of street dust.
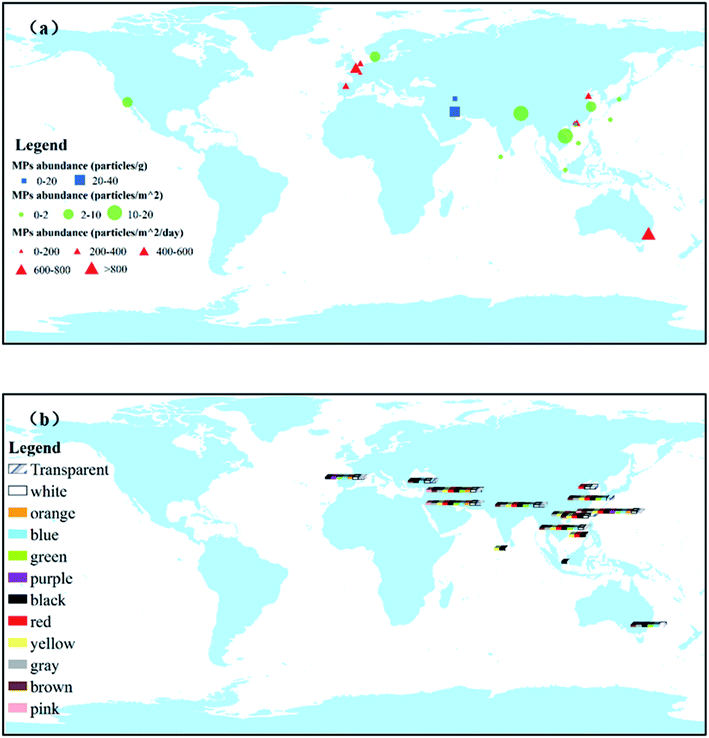 |
| Fig. 3 The global distribution of concentration and color characteristics of MPs in the atmosphere. Panel (a) represents the concentration of atmospheric MPs monitored in the published literature surveyed. Panel (b) represents the color of atmospheric MPs detected in the surveyed published literature. | |
(3) Shape.
Fibrous and fragmented shapes are the most abundant shapes in atmospheric MPs. In addition, irregular shapes such as fragments, films, and spheres also exist. The study conducted in Paris40 and in Yantai55 confirmed the highest proportion of fibrous MPs in atmospheric deposition samples. Fibers are also the most prevalent microplastic shape collected in different indoor environments.64 MPs in different shapes have different physical properties. Films tend to be flexible and thin, fibers tend to be very thin, and flakes tend to be thick and irregular in shape. Overall, the shape of MPs in the atmosphere depends on the original morphology of the primary MPs, the degradation and erosion processes on the surface of the plastic particles, and the residence time in the environment.43
In different functional areas of the same city, the shapes of atmospheric MPs are often different. For example, more film-like, spherical, and fragmented MPs were found in industrial areas while more fibrous MPs were found in urban areas.65 Most of the street dust samples were dominated by spherical and film-like particles, with MPs mainly composed of black debris and fibrous particles of varying sizes.66
3.1.3 Environmental abundance of atmospheric MPs.
Most available studies focus on atmospheric MP concentrations, which can be combined with size and composition to simulate their transport in the atmosphere.
Deposition fluxes of MPs with different morphologies in the atmospheric environment vary with seasons. For instance,55 by compiling data from the sampling points in Yantai city, atmospheric MP deposition flux in spring, summer and winter was relatively high, with a varied range of 4.84 × 102 to 6.24 × 102 particles per m2 per day, but in autumn, it was lower, only 1.30 × 102 particles per m2 per day. The daily deposition fluxes of different types of MPs vary widely, and there are large seasonal differences.
The average abundance of atmospheric MPs varies widely across investigated areas (Fig. 3a). In European cities, a median abundance of atmospheric MPs ranging from 136.5 to 512.0 particles per m2 per day was observed in the metropolitan area of Hamburg.67 In Asia, the atmospheric deposition in Dongguan city, China, comprises the concentrations of non-fibrous MPs and fibers ranging from 175 to 313 particles per m2 per day.54 For countries in the same continent, the concentration flux of atmospheric MPs in total atmospheric deposition in France is 29–280 particles per m2 per day.40 Atmospheric MPs had been measured at a deposition rate of between 575 and 1008 microplastics per m2 per day in central London, UK,61 and between 136.5 and 512.0 microplastics per m2 per day in Germany.67 These differences may be due to different detection methods and sampling heights.
In addition, climate, population density, level of industrialization, and human activities may also affect the spatial distribution of MPs in the atmosphere. The same sampling and detection method was used to assess the intensity of exposure to MPs in the atmosphere of five megacities in China.72 The results showed that the concentrations of MPs in the air in northern cities (358 ± 132 items per m3) were higher than those in southeast cities (230 ± 94 items per m3). Significant differences in the distribution of atmospheric MPs in different functional areas of the same city show that the above guesses are well-founded. According to one year of atmospheric MP monitoring in Paris, France,57 the deposition rate of MPs in the urban atmosphere was 110 ± 96 plastics per m2 per day, while that in the sub-urban was 56 ± 38 plastics per m2 per day.
In small areas, human activities are an important factor affecting the distribution of MPs in the air. There are also obvious flux differences between indoor and outdoor air MPs collected in the same functional area. For example, fibers and fragments in indoor air are twice as high as outdoors in coastal buildings in California, USA.56 Measurements in Paris, France58 ranged from 1.0 to 60.0 fibers per m3 for indoor MPs and 0.3 to 1.5 fibers per m3 for outdoor MPs. Data from different regions show that the concentration of MPs in indoor air is significantly higher than in outdoor air.
The factors that influence the distribution of MPs in the air are complex. The spatial distribution of MPs in an atmospheric deposition is related to wind speed, wind direction, and precipitation.56 More emphasis should be paid to the exact links between these factors in future studies. Human activities have been shown to have a significant impact on microplastic pollution. Whether indoor64 or outdoor60 environments, more MPs would be found in larger population densities. According to the results, we can intuitively see the occurrence and distribution of MPs and infer the source and transport in the atmospheric environment.
3.2 Sources of atmospheric MPs
3.2.1 The use, cleaning and drying process of fiber products.
The source of atmospheric MPs is difficult to trace, but potential sources can be explored through the characteristics and polymer types of atmospheric MPs. The main source of airborne MPs is synthetic fibers.72,73 Globally, more than 90 million tons of textile fibers were produced in 2016. Two-thirds of them were synthetic and plastic fibers, and the production rate has grown at around 6.6% per year over the past decade.74 The concentration of MPs in indoor air is much greater than that in outdoor air, which may be related to the high abundance of synthetic textiles in indoor air.56–58 The global demand for synthetic textiles (e.g., clothes, blankets, and curtains) is growing. In the production process of synthetic fibers, the friction and cutting of the fibers will produce many fine fibers, and the smaller fibers can easily float into the air. In daily use, textile products are easily broken into fine particles and enter the atmosphere when subjected to mechanical wear and ultraviolet radiation.75 MP fragments can originate from the decomposition, wear, and weathering of packaging and reusable products.
3.2.2 Dust resuspension.
Dust is often seen as the fate of atmospheric MPs, but MPs in dust can be re-transported into the atmosphere by wind, so dust is a secondary source of atmospheric MPs. Smaller MPs (<100 microns), including clothing fibers and car tires, can significantly increase road dust.76 An important source of MPs in street dust is road traffic emissions. From a quantitative study on the emission of MPs in the atmosphere from urban mining bases, tire micro-rubber is emitted the most,77 which also confirms this view. Global per capita tire plastic emissions are about 0.81 kg per year. In the air, tire wear accounts for about 3–7% of particulate matter (PM2.5), suggesting that it may contribute to the global health burden of air pollution.78
3.2.3 Landfill or incineration.
Much of the plastic waste is disposed of by dumping it into landfills, significantly exposing the plastic to the atmosphere.79 Other sources of airborne MPs may come from emissions from the recycling of plastics. Plastic waste does not decompose in landfills,80 but degrades into MP particles over time through physical, chemical, and biological processes6 that not only affect the environment, but also emit MPs into the air.81 The accumulation and release of MPs in landfills is a long-term process, and landfills might not be the final sink of plastic but a potential source of MPs.82 Large amounts of MPs are present in atmospheric suspended particulates from landfills. However, by studying MPs in lichen samples at different distances from landfills, the spatial impact of landfill emissions is limited.83 The number of MPs decreased exponentially with distance, from 79 MP g−1 dw at a close range to 13 MP g−1 dw at 200 m and 7 MP g−1 dw at 1500 m from the landfill.
3.2.4 Other sources.
Industry and agriculture can also generate MPs and release them into the environment.35 Other human activities, such as the process of using building materials (paint, wallpaper) and kitchen plastic utensils (brushes, rags), release MPs during use and friction.84 It was suggested that some MP particles in the ocean may enter the atmosphere through bubble burst jets and wave action.85
The source and transmission of atmospheric MPs are new contents and hot issues in the study of MPs. We can infer the type and sources of MPs from their color and shape.
3.3 Transport and fate of MPs in the atmospheric environment
The ubiquity of MPs is due to the movement of MPs in the atmosphere. Transport, diffusion, and deposition mechanisms are the driving forces for the movement of atmospheric MPs (Fig. 4).
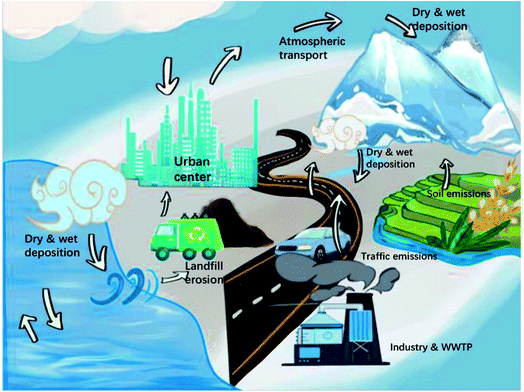 |
| Fig. 4 Sources, transport and fates of MPs in the atmosphere. | |
3.3.1 Transport of atmospheric MPs.
In outdoor environments, it had been demonstrated that MPs could be transported to great distances under the action of airflow. This was reflected in the detection of MPs in Arctic snow,1 in atmospheric deposition in the pristine mountains of the remote French Pyrenees,86 and in the suspended atmospheric particulates in the Western Pacific.62 In indoor environments, human activity induces airflow in the room, leading to the resuspension of settled particles and increasing the concentration of MPs in indoor air.64
Transport is affected by the size and shape of atmospheric MPs.43 MPs that can be transported in the atmosphere tend to be smaller. There are still many unknowns about the transport mechanism of atmospheric MPs. From the MPs detected in snow samples and in the air suspended over the sea surface, it can be assumed that fibers and debris are more easily transported.1,63 The transport is related to the concentration of MPs in the atmosphere.
The transport of atmospheric MPs is affected by climate change. Wind provides an efficient route for the transport of atmospheric MPs. The monsoon affects the transport flux of atmospheric MPs, increasing the possibility of long-distance transport, which in turn affects their fate.87 Atmospheric transport may lead to preferential accumulation of MPs in certain regions.88 This affects the ecosystems in some areas, increasing the risk of organisms being exposed to MPs.
3.3.2 Fate of atmospheric MPs.
MPs carried into the atmosphere by airflow have different fates depending on their size, composition, and shape. Particle size is a major factor in determining the environmental fate of atmospheric MPs. MPs enter the atmosphere and quickly combine with water vapor in the air to form aerosols. For common aerosol particles, the smaller the particle size, the longer they remain suspended in the air. While larger MPs sink under the influence of gravity, smaller ones tend to be suspended in the atmosphere for a longer duration, transported to greater distances, or enter terrestrial or marine systems by wet deposition. This also explains the particle size <50 μm observed in the remotely transported atmosphere.86 In an experiment,62 the proportion of microfibers in the TSP decreased the most, and it was speculated that microfibers were more likely to sink. Meanwhile, the amount of PE collected at night was four times higher than that during the day, speculating that this may be related to the density and water absorption of the polymer. Airborne MPs with lower densities are more likely to be transported farther.89
Atmospheric MPs deposited into aquatic and terrestrial ecosystems may be ingested by animals, undergoing nutrient level transfer and bioaccumulation. Plant leaf surfaces can serve as potential temporary sinks for atmospheric MPs.90 Some atmospheric MPs are temporarily stored on the surface of plant leaves and resuspended into the atmosphere under the action of wind.91 Some nano-scale plastics may enter plants through leaves, thereby affecting the growth of plants.
Physical, chemical and biological actions further break down MPs in the atmosphere. For example, weathering can lead to the release of additives and oligomers in MPs, causing greater harm to the environment.92 The fate of atmospheric MPs depends not only on the type and concentration of emissions, but also meteorology, topography and human activities. As the number of rainy days increases, the content of fibrous MPs collected in atmospheric deposition increases significantly.93 Precipitation promotes the deposition of MPs in the air and is one of the important factors affecting the content of MPs in atmospheric deposition samples. Low temperature will lead to the emergence of a strong inversion layer and a low atmospheric boundary layer,94 resulting in poor dispersion of suspended particles in the atmosphere and high concentrations of atmospheric particles over cities. The wind is a positive driver of MP deposition and increases resuspension. High wind speeds favor the atmospheric deposition of MPs. MP deposition rates and mean wind-speed high-consistence abundances in atmospheric fallout also increased with wind speed.61 The analysis of MPs in different indoor areas showed the dynamic changes of human activities affecting MPs in the indoor environment.64
4 Ecological risks of atmospheric MPs
4.1 Effects of atmospheric MPs on the aquatic ecosystem
Atmospheric MPs enter aquatic ecosystems through dry and wet deposition (Fig. 5a), thereby, atmospheric deposition is a major source of MPs in the aquatic environment. MPs are persistent in aqueous environments because of their durability. Some MPs, denser than seawater, may sink to the seabed. Other MPs, lighter than seawater, frequently float on the water surface.96 Both marine and freshwater organisms can ingest MPs.
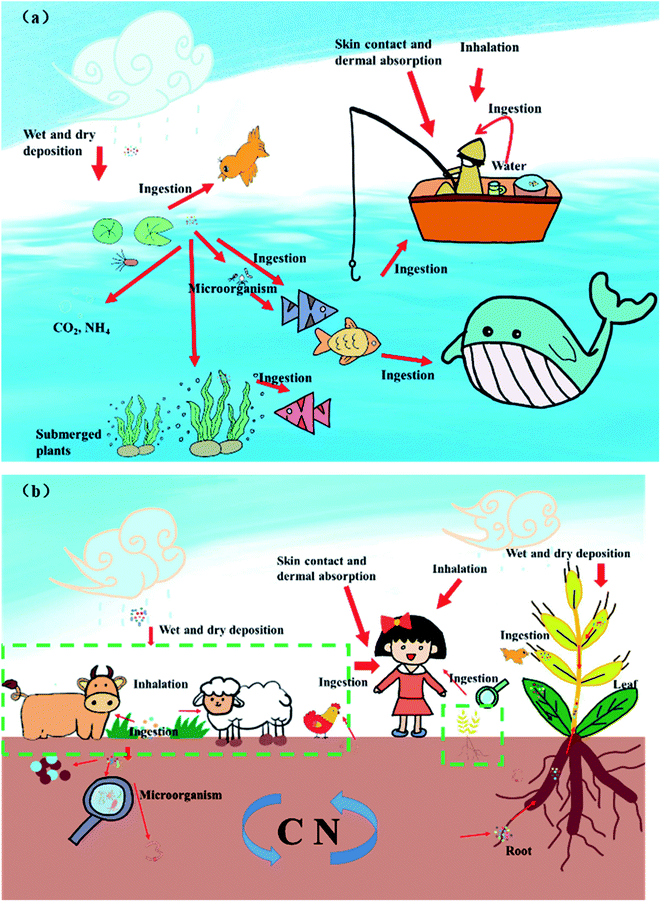 |
| Fig. 5 Transport and exposure pathways, ecological risks and human health risks of atmospheric MPs. Panel (a) represents the terrestrial environment, and panel (b) represents the aquatic environment. | |
4.1.1 Effects on aquatic animals.
There are increasing research studies on the effects of MPs on aquatic animals. MPs entering the aquatic environment from the atmosphere are ingested by a variety of aquatic animals, such as invertebrates, mammals,97 fish,98 and shellfish.99 Mussels effectively reflect environmental pollution. Judging from the fact that MPs in the water environment pose a great threat to survival, microplastic pollution in the North Pacific is already serious.100 The ecological risks caused by MPs need to be taken seriously. Mammals can be exposed to MPs through direct ingestion of seawater or indirect ingestion of prey.101 MPs can affect the gut microbiota of aquatic animals because of the associated chemicals.95 They may accumulate in the digestive tracts of aquatic animals, clogging their guts and limiting the ability of these organisms to ingest and digest food.102 This can cause toxicity and even death to aquatic animals.
Ingestion of low doses of MPs often does not cause the immediate death of organisms, and natural dietary habits103 and histological changes104 can serve as potential indicators of susceptibility to ingested MPs. For fish, the consumption of MPs can cause significant toxicological damage, including induction of inflammation and oxidative stress in the gut.105 In addition to ingestion, considering the mode of adhesion increases the estimation of the bioavailability of MPs to aquatic organisms. The attachment of MPs to animals is also a way for them to move through the food web.106 Non-aquatic animals such as seagulls107 and penguins108 may mistakenly ingest MPs floating on the sea surface. Because the MPs floating on the water are similar in size and color to their prey, they are vulnerable to active or accidental feeding by zooplankton.109 In addition to the direct adverse effects on biota, MPs in aquatic environments may also play a potential role as carriers of hydrophobic organic chemicals and microorganisms.110 The effects of MPs on organisms in the aquatic environment are chronic, cumulative and persistent.97 They can accumulate in organisms, enter the food web, reach higher trophic levels, and even endanger human health.
4.1.2 Effects on aquatic microorganisms.
MPs bring more organic and inorganic carbon to the aquatic environment, which stimulates the activity of microorganisms.111 This could lead to the release of more CO2 from the water environment, as well as to alter the CH4 cycle. Moreover, MPs affect the structure and function of microbial communities. The invasion of MPs changed the composition and structure of bacterial communities and decreased biofilm richness and diversity.112 On the other hand, microbes may influence the fate of MPs.113 Changes in aquatic microbiome function affect carbon metabolism and food webs in aquatic environments. The buoyancy, hydrophobic surface and ability to transport over long distances of MPs make them novel substrates of choice for microorganisms.114 The potential toxicity of MPs to aquatic organisms may be related to microbial pathogens.115 MPs will act as a transfer carrier of harmful microorganisms in the water environment, affecting the colonization of microbial pathogens on the surface of MPs.116
4.1.3 Effects on hydrophytes.
MPs floating on the water surface hinder the absorption of light by phytoplankton, which in turn prevents them from providing food and oxygen to aquatic life.96 This view was also illustrated by the fact that MPs reduce the photosynthetic activity of Chlorella by causing physical damage and oxidative stress.117 But it is very likely that after the algae have adapted to the presence of MPs in the water environment, they are stimulated to grow more, creating ecological risks. High concentrations of MPs can significantly alter the structure of phytoplankton communities.118 There could be an increase in the abundance of algae in parts of the phytoplankton community. Changes in phytoplankton communities will affect food webs and ecological functions in the aquatic environment.
Many common MPs are denser than water, so they settle in aquatic environments. A large number of microfibrils were detected in Cladophora in the Great Lakes of the United States.119 These MPs can interact with submerged aquatic plants. MPs in the aquatic environment will pose growth stress on submerged plants, including reducing plant height and chlorophyll content.120 MPs may enter plants through the leaves or roots of submerged plants. Microfibrils were detected from the cell wall, confirming that MPs entered the plant. MPs entering plants can affect oxidative stress, antioxidant responses, and fluorescence parameters of chlorophyll in submerged plants.121 On the other hand, submerged plants also affect the distribution of MPs in the water environment. These submerged plants that absorb MPs may be eaten by other fish, causing more serious ecological risks through the the food chain and web.
4.2 Effects of atmospheric MPs on the terrestrial ecosystem
Atmospheric transport and deposition provide the possibility for MPs to enter the terrestrial environment. Many studies have confirmed that atmospheric MPs enter the terrestrial ecosystem through dry and wet deposition.55,57,67 Tire plastic can be released into the soil close to the road and beyond.122 MPs that enter the terrestrial environment from the atmosphere either persist in the soil layer for a long time or degrade slowly. MPs accumulate in the soil in large quantities, posing a potential threat to terrestrial ecosystems.123 Different types of MPs have contrasting properties and induce different impacts on terrestrial ecosystems (Fig. 5b).
4.2.1 Effects of MPs on the soil biophysical environment.
The different types, shapes and sizes of MPs pose different negative effects on the soil environment. MPs, which are similar in shape and size to soil particles, have less impact on the soil structure.124 The shape of fibrous MPs is much different from that of soil non-linear particles, which may mean that it can wrap around soil particles more efficiently. The shape of MPs has the potential to alter soil biophysical properties. A study has shown that MPs entering the soil can reduce soil bulk density and water-holding capacity and change the soil structure.125 MP types influence the interaction of MPs and soil pore space. Polyacrylic fibers and PE fragments did not significantly reduce soil bulk density as did PES fibers. However, research has shown that PES microfibers cannot change the soil bulk density.126 This may be caused by the differences in the experimental soils. Further research is needed to fully understand how MPs affect the soil physical environment, as well as the effects of microplastic shape, size, and composition.
4.2.2 Effects of MPs on soil organisms.
The effects of MPs on soil organisms have been documented. Soil organisms include microorganisms, animals, and plants that live in the soil. MP contamination alters the physical properties of the soil, affecting soil hydrodynamics and microbial activity.127 This may inevitably alter the composition of soil microbial communities, with implications for soil fauna and plants.
MPs influence soil microbial community structure. It was found that PE significantly changed the structure of bacterial communities and affected the succession of bacterial communities.128 MPs affect the evolution of soil microbes by changing the soil environment.129 MPs can serve as a source of nutrients and organic carbon in the soil, and microbiota utilizing this resource has a survival advantage. Their shape, size and concentration limit the effects of MPs on microbes. Fiber, foam and film MPs significantly affect soil bacterial composition compared to particles.130 The change in soil microbes could lead to a change in their interaction with soil animals.
Soil animals can ingest MPs. Many studies have focused on the harmful effects of MPs on soil-dwelling animals, such as earthworms,131 springtails,132 and nematodes.133 Ingestion of MPs may lead to insufficient nutrient supply, oxidative stress and intestinal damage to soil animals, thereby inhibiting their growth and reproduction.135 Although tissue and immune system damage were evident, lower MP concentrations clearly impacted the mortality outcome of soil animals.136 More research is needed in the future to identify histopathology and the immune system as potential indicators of susceptibility to ingested MPs. The size and concentration of MPs can determine how toxic they are to soil animals.131,137 Through experiments, it was found that the addition of MPs could significantly affect the composition and abundance of microarthropod and nematode communities.133 Likewise, exposure to MPs significantly enhanced bacterial diversity in collembola's gut.132 The response of soil fauna to the addition of MPs can cascade through the soil food web. Changes in soil fauna and trophic structure caused by the presence of MPs can significantly affect the activity of microorganisms.133 This indirect effect may be stronger than the direct effect of MPs on microbes. Research has provided new insights that extractable additives in MPs are the main toxic pathway for soil fauna.134 While some specific types of MPs and the extractable additives both reduced worm growth and survival, the effects of both were not significant.
MPs in the atmosphere may enter the soil environment to affect the growth of plants and may also have an impact on plant leaves through dry and wet deposition. MPs in the atmosphere can adhere to the foliar of leaves and even enter plants through stomata. Most of the MPs that adhered to and entered the leaf were fibrous,138 which may be related to the shape of the leaf stomata. Plant leaf stomata absorb NPs and transfer them down to the roots. Foliar absorption of MPs may affect photosynthesis in plants.139 Foliar exposure to MPs can have a considerable impact on growth, lowering crop dry weight, plant height, and leaf area.140 MPs deposited from the atmosphere into the soil are absorbed by plant roots and transferred to shoots.141 MPs present in the soil can alter the rooting ability of plants. PES and PS in soil resulted in a significant increase in root biomass.127 MPs can significantly increase the root length of plants and reduce the average root diameter. Thin, long roots increase the absorption of MPs. In the case of the aerial parts of plants, MPs also affect plant leaf shape and total biomass. Smaller size MPs may have more severe chemical toxicity in addition to their physical impact on the biota. It was thought that NPs may enter plant roots and may cause damage to plants, such as oxidative stress.142
4.2.3 Effects of MPs on the biogeochemical cycles of soil carbon and nitrogen.
There are important links between carbon and nitrogen cycles and soil microbes that are related to greenhouse gases (GHGs) and climate change. Some studies have confirmed that MPs deposited from the atmosphere into the soil can affect carbon and nitrogen cycles in the soil by altering the composition and function of microbial communities.143,144 A study has shown that adding MPs can significantly promote soil CO2 emissions.145 This could exacerbate global warming and cause even more serious environmental problems. However, no effect of adding MPs on soil NO emissions was found. MPs with different characteristics affect the diversity of bacterial communities in the soil. MPs have different effects on different soil microbes. The composition of dissolved organic carbon in the soil is affected by the size of MPs.146 It was reported that143 PUF and PLA promoted sediment nitrification and denitrification, while PVC inhibited it. This indicates that different MPs affect soil carbon and nitrogen cycles differently. Nitrogen availability in the soil was reduced in MP-treated soils. It is possible that MPs affect the nitrogen cycle by altering soil porosity.144 There is also a view that MPs may alter soil nutrient cycle by altering soil bacteria or genes and enzymes associated with carbon, nitrogen and phosphorus.147 The biological mechanism requires further studies to explain.
5 Human health risks of atmospheric MPs
Growing research studies have shown that humans are generally exposed to environmental MPs through ingestion, inhalation, and skin contact.148
5.1 Inhalation by respiration
The particulate toxicity of atmospheric MPs after inhalation is related to the physiological mechanisms of deposition and clearance involved in human inhalation of MPs.149 MPs settle in various parts of the human respiratory system by gravity. The clearance mechanism can reduce the concentration of MPs in the body. Microplastic particle toxicity induced by dust overload, oxidative stress, cytotoxicity, and particulate matter translocation can all decrease the clearance mechanism,150 thereby affecting human health.
The size of the MPs determines their respiratory potential, as only MPs smaller than 10 μm can be inhaled. Both indoors and outdoors, MPs smaller than 10 μm were detected, indicating that MPs in the air can be directly inhaled.151 Plastic fibers were observed in human lung samples, confirming that they can be inhaled.152 Although most of the coarse inhalable particles undergo mucociliary clearance in the upper airway, some fine particles can escape this mechanism and deposit deep in the lungs.153 These particles (especially longer fibers) tend to avoid gaps and exhibit extreme durability in physiological fluids, where they may persist and accumulate upon inhalation. Although airborne fibers are more abundant than fragments, the smaller size consists almost entirely of fragments. Therefore, finer-sized fragments are more likely to enter the human lungs. Fragments rather than fibers are primarily transported in the air. For instance, 31 synthetic polymer particles and fibers were observed in the lung tissue of 13 of the 20 autopsies of the deceased, of which 87.5% were fragments and 12.5% were fibers,154 confirming this idea. Most of the inhaled MPs are retained in the upper respiratory tract.
The effects of MPs on human health also depend on exposure time and concentration. Elevated concentrations of MPs in the atmosphere may significantly increase human health risks.155 Simulating through a breathing thermal manikin, 272 MPs could be inhaled by a mannequin within 24 hours.153 Young children tend to be at a greater risk than adults from exposure to airborne MPs.66,156 The general population is generally exposed to low concentrations of atmospheric MPs. However, occupationally exposed practitioners may be exposed to high levels of MPs, which may lead to the accumulation of higher concentrations of MPs and occupational diseases.149,157 Cytotoxicity is also highly influenced by the size and shape of MPs.
NPs and MPs have potential toxicological effects on human lung cells. Exposure of lung cells to MPs inhibited cell proliferation, as well as caused significant changes in cell morphology.158 NPs may affect the internalization, cell viability, cell cycle, and apoptosis of human lung epithelial cells.159,160 It was demonstrated that exposure of normal human lung epithelial cells BEAS-2B to MPs resulted in inflammation and oxidative damage, as well as disruption of intercellular junctional proteins in the lung.161 This can lead to acute and chronic respiratory diseases. Airborne MPs were also suspected of causing chemical toxicity as potential PAH carriers, plastic additives, and metals, among others.160,162,163 Microplastic with additives tended to pose higher inflammatory potential than those without additives.157 Airborne MPs may be toxic to organs other than the respiratory system. For example, ultrafine particles (UFPs) may reach the gut via inhalation and diffusion from the lungs into the systemic circulation or ingestion of inhaled particles after airway mucociliary clearance.155
Ingested or inhaled particles may further enter the bloodstream and may be toxic to other organs (such as liver, embryo, brain, etc.) after translocation.155,164–168 Different types of MPs have varied toxic effects on human organs.169 But no studies have clearly explained how MPs enter the bloodstream and are transported to other organs after being inhaled or ingested.
5.2 Oral ingestion
Plastics are an inevitable part of our daily lives. MPs are frequently present in human daily water and food. In addition, large amounts of MPs are found in everyday drinking bottled beverages, tap water, and alcohol.
The concentrations of MPs in different water sources vary widely. MP particles were detected in different brands of bottled water and tap water samples from around the world.170,171 The most common MP shapes in tap water were fibers (99%).171 In some case studies, 95% of MP particles were detected in bottled water with a size of 6 to 100 μm,170 or 80% of MP particles were detected with a size of 5 to 20 μm.172 This suggests that humans easily ingest MPs in drinking water. Moreover, human-caused MP pollution is also present in beer, salt, and seafood.96,171,194 These MPs have many sources, with atmospheric emissions being a potential source.173
MPs transfer nutrients through the food chain. MPs can be transferred from the environment into living organisms and then, through the food web, posing a risk to human health.174,175 Some of the MPs entering the human digestive system are excreted through feces,176,177 and some remain in the human body.178 The risk of intestinal exposure to MPs in infants is higher than in adults.177 The toxicity of MPs to humans varies depending on their sources and properties.
Researchers disagreed on the health risks of MPs to the human gut. Ingestion of MPs by the human body can cause abrasions, perforations and even blockages in the digestive tract.179 According to the effects of artificial in vitro digestion on 5 types of MPs, all plastic particles had high resistance to artificial digestive juices,180 and the major stages of the human gastrointestinal tract do not break down particles. However, in a study of the digestion process of seal pups,181 MPs break down into smaller particles in the stomach.
In vitro simulation experiments on human cells Caco-2 and intestinal flora showed that PE could weaken the cell activities and change the composition of intestinal flora, which would pose a threat to human health.182 However, when PS of different sizes was used on Caco-2 cells, the particles did not cause damage and inflammation to the cells.183 Oral ingestion of environmental level MPs is unlikely to represent a serious health risk to people. Most current studies on MPs in the human digestive system rely on epidemiological studies, in vivo animal tests,185 and in vitro cell culture approaches. The consequences of MP exposure on the human digestive system are mostly unknown and necessitate detailed and more profound assessment.
Through oral ingestion, MPs that exist in the human body can accumulate in the human digestive system or translocate to other organs (e.g., liver, kidney).184 In addition to the toxicity of MPs themselves to the human digestive system, they may also act as a carrier for other toxic substances.185–188 Most of the MP samples detected in human digestion were clear filaments,178 probably because MPs are decolorized in the digestive tract. It is still unknown whether the MP pigments remain in the human body and cause harm to the human body. There are many more ingested routes that require our attention, such as ingesting vegetables and poultry exposed to MPs.
5.3 Skin contact and dermal absorption
Human skin is the largest organ of the human body and performs a strict barrier function to the external environment.189 Particulate pollutants are associated with skin aging. Traffic-related particles can increase freckles on the forehead and cheeks, as well as wrinkles in the nasolabial folds.190 Skin contact with MPs is considered a non-critical route of exposure. However, fine particulate matter is often considered a major environmental issue that causes respiratory problems and skin diseases in humans.191 Various particulate matter in air pollution, which induce oxidative stress by producing reactive oxygen species (ROS) and secreting pro-inflammatory cytokines, can affect the development and exacerbation of skin diseases.192 There is no available direct evidence that atmospheric MPs can penetrate the skin, and further research in this field is needed.
6 Conclusion and perspectives
Environmental risk research of MPs, particularly atmospheric MP research, is still in its early stages. MPs have been detected in atmospheric fallout,40,54,67 indoor and outdoor air,56,58 snow samples,10 and street dust.65,76 MPs in the atmosphere have various shapes, colors, sizes and compositions. The monitoring of MPs in the atmosphere is related to collection and measurement methods. Currently, there is no uniform and accurate technique to support the study of MPs in the atmosphere. Therefore, more research in the future should focus on the development of related technologies and instruments.195 When analyzing the chemical composition of atmospheric MPs, the main focus is on the concentration, characteristics and composition of atmospheric MPs. The coexistence of MPs with other pollutants requires more in-depth research. Understanding the complex composition of atmospheric MPs enables better management and reduction of pollution caused by atmospheric MPs. Most detected airborne MPs ranged in size from 200 to 600 μm. Possibly affected by the method adopted, more and smaller MPs were not detected. MPs smaller than 10 μm have been found in the atmospheric environment. Smaller atmospheric MPs can be ingested and inhaled by organisms, causing more serious toxicological damage.
Atmospheric MPs are from a wide range of sources, including synthetic textiles, dust, and landfills. Most studies mainly use models or speculate on the origin of atmospheric MPs based on the characteristics of MPs. No available data can specify the detailed sources of atmospheric MPs and the contribution rate of each source to predict the future global atmospheric MP pollution. The effects of weathering and biodegradation on MP transport in the atmospheric environment need to be elucidated. Will MPs continue to break down into NPs during transport? What is the ultimate fate of NPs?
The driving forces for the movement of atmospheric MPs are migration, diffusion and deposition mechanisms. Large amounts of MPs in the atmosphere enter aquatic and terrestrial ecosystems mainly through wet and dry deposition. MPs can move in environments with different media.196 There is value in distinguishing MPs that enter aquatic and terrestrial ecosystems from the atmosphere from those that are native to aquatic and terrestrial ecosystems. However, in practice, it is currently difficult to directly explain the ecological risks posed by MPs from the atmosphere entering other ecosystems. Few studies have been able to accurately account for the ecological risks posed by atmospheric MPs, for which there are also no guidelines, thus requiring more exploration. Possibly this is based on an accurate understanding of the source–pathway–sink relationship of atmospheric MPs in various environmental media.
The published literature investigated the human health risks posed by atmospheric MPs through in vitro cell cultures and models.193 Researchers hold different opinions on the risks MPs pose to human health. Ingestion or inhalation of MPs (especially NPs) is widely believed to be toxic to human organs. There is currently no research on how micro–nano plastics enter the human body and what damage they do to various human body organs. The toxicological effects of various types, sizes and shapes of micro–nano plastics on human cells also need attention. Knowledge gaps remain on the environmental exposures, bio–nano interactions, and potential risks of MPs.197 More scientifically reliable data are needed to explain the presence of atmospheric MPs and the resulting ecological and human health risks.
After understanding the pollution of MPs in the atmospheric environment, policies to help reducing MP pollution need to be implemented in the future. Strictly controlling the way MPs enter the environment and selecting MPs that pose less ecological and human health risks have become an urgent goal.
Conflicts of interest
There are no conflicts to declare.
Acknowledgements
This research was funded by the National Natural Science Foundation of China (NSFC 41977349), and the National Key Research and Development Project of China (2019YFC1804704). We would like to thank Dr Tariq Mehmood from the College of Ecology and Environment, Hainan University for his revisions and linguistic polish to this manuscript.
References
-
PlasticsEurope, Available on: https://www.plasticseurope.org/application/files/8016/1125/2189/AF_Plastics_the_facts-WEB-2020-ING_FINAL.pdf, 2020 Search PubMed.
- S. B. Borrelle, J. Ringma, K. L. Law, C. C. Monnahan, L. Lebreton, A. McGivern, E. Murphy, J. Jambeck, G. H. Leonard, M. A. Hilleary, M. Eriksen, H. P. Possingham, H. D. Frond, L. R. Gerber, B. Polidoro, A. Tahir, M. Bernard, N. Mallos, M. Barnes and C. M. Rochman, Predicted growth in plastic waste exceeds efforts to mitigate plastic pollution, Science, 2020, 369, 1515–1518 CrossRef CAS PubMed.
- D. E. MacArthur, Beyond plastic waste, Science, 2017, 358(6365), 843 CrossRef CAS PubMed.
-
H. Ritchie, M. Roser, Plastic pollution, 2018, https://ourworldindata.org/plastic-pollution Search PubMed.
- L. Lebreton and A. Andrady, Future scenarios of global plastic waste generation and disposal, Palgrave Commun., 2019, 5, 6 CrossRef.
- D. Sol, A. Laca, A. Laca and M. Diaz, Approaching the environmental problem of microplastics: importance of WWTP treatments, Sci. Total Environ., 2020, 740, 140016 CrossRef CAS PubMed.
- A. K. Priya, A. A. Jalil, K. Dutta, S. Rajendran, Y. Vasseghian, J. Q. Qin and M. Soto-Moscoso, Microplastics in the environment: recent developments in characteristic, occurrence, identification and ecological risk, Chemosphere, 2022, 298, 134161 CrossRef CAS PubMed.
- B. B. Xi, B. Wang, M. Chen, X. Q. Lee, X. Y. Zhang, S. S. Wang, Z. B. Yu and P. F. Wu, Environmental behaviors and degradation methods of microplastics in different environmental media, Chemosphere, 2022, 299, 134354 CrossRef CAS PubMed.
- J. Qian, S. J. Tang, P. F. Wang, B. H. Lu, K. Li, W. Jin and X. X. He, From source to sink: review and prospects of microplastics in wetland ecosystems, Sci. Total Environ., 2021, 758, 143633 CrossRef CAS PubMed.
- M. Bergmann, S. Mutzel, S. Primpke, M. B. Tekman, J. Trachsel and G. Gerdts, White and wonderful? Microplastics prevail in snow from the Alps to the Arctic, Sci. Adv., 2019, 5(8), eaax1157 CrossRef CAS PubMed.
- J. Jeyavani, A. Sibiya, S. Shanthini, C. Ravi, S. Vijayakumar, D. K. Rajan and B. Vaseeharan, A Review on Aquatic Impacts of Microplastics and Its Bioremediation Aspects, Curr. Pollut. Rep., 2021, 7, 286–299 CrossRef CAS.
- S. Y. Tang, L. Gao, H. Z. Gao, Z. S. Chen and D. L. Zou, Microplastics pollution in China water ecosystems: a review of the abundance, characteristics, fate, risk and removal, Water Sci. Technol., 2020, 82(8), 1495–1508 CrossRef CAS PubMed.
- F. X. Zhu, C. Y. Zhu, C. Wang and C. Gu, Occurrence and Ecological Impacts of Microplastics in Soil Systems: A Review, Bull. Environ. Contam. Toxicol., 2019, 102, 741–749 CrossRef CAS PubMed.
- M. C. Rillig and A. Lehmann, Microplastic in terrestrial ecosystems, Science, 2020, 368(6498), 1430–1431 CrossRef CAS PubMed.
- J. Gong and P. Xie, Research progress in sources, analytical methods, eco-environmental effects, and control measures of microplastics, Chemosphere, 2020, 254, 126790 CrossRef CAS PubMed.
- X. Long, T. Fu, X. Yang, Y. Y. Tang, Y. Zheng, L. Zhu, H. Z. Shen, J. H. Ye, C. Wang, T. Wang and B. J. Li, Efficient Atmospheric Transport of Microplastics over Asia and Adjacent Oceans, Environ. Sci. Technol., 2020, 56(10), 6243–6252 CrossRef PubMed.
- N. L. Fahrenfeld, G. Arbuckle-Keil, N. N. Beni and S. L. Bartelt-Hunt, Source tracking microplastics in the freshwater environment, TrAC, Trends Anal. Chem., 2019, 112, 248–254 CrossRef CAS.
- C. M. Rochman, Microplastics research—from sink to source, Sicence, 2018, 360(3684), 28–29 CrossRef CAS PubMed.
- W. J. Shim, S. H. Hong and S. E. Eo, Identification methods in microplastic analysis: a review, Anal. Methods, 2017, 9, 1384–1391 RSC.
- A. A. D. S. Machado, W. Kloas, C. Zarfl, S. Hempel and M. C. Rillig, Microplastics as an emerging threat to terrestrial ecosystems, Global Change Biol., 2017, 24(4), 1405–1416 CrossRef PubMed.
- J. C. Anderson, B. J. Park and V. P. Palace, Microplastics in aquatic environments: implications for Canadian ecosystems, Environ. Pollut., 2016, 218, 269–280 CrossRef CAS PubMed.
- A. Sarker, D. M. Deepo, R. Nandi, J. Rana, S. Islam, S. Rahman, M. N. Hossain, Md. S. Islam, A. Baroi and J. Kim, A review of microplastics pollution in the soil and terrestrial ecosystems: a global and Bangladesh perspective, Sci. Total Environ., 2020, 733, 139296 CrossRef CAS PubMed.
- M. S. Bhuyan, S. Venkatramanan, S. Selvam, S. Sylvia, M. M. Hossain, M. Rashed-Un-Nabi, C. R. Paramasivam, M. P. Jonathan and M. S. Islam, Plastics in marine ecosystem: a review of their sources and pollution conduits, Reg. Stud. Mar. Sci., 2021, 41, 101539 Search PubMed.
- Y. Zhang, S. Y. Pu, X. Lv, Y. Gao and L. Ge, Global trends and prospects in microplastics research: a bibliometric analysis, J. Hazard. Mater., 2020, 400, 123110 CrossRef CAS PubMed.
- G. L. Chen, Q. Y. Feng and J. Wang, Mini-review of microplastics in the atmosphere and their risks to humans, Sci. Total Environ., 2020, 703, 135504 CrossRef CAS PubMed.
- R. Geyer, J. R. Jambeck and K. L. Law, Production, use, and fate of all plastics ever made, Sci. Adv., 2017, 3(7), e1700782 CrossRef PubMed.
- R. C. Thompson, Y. Olsen, R. P. Mitchell, A. Davis, S. J. Rowland, A. W. G. John, D. McGonigle and A. E. Russell, Lost at sea: where is all the plastic?, Science, 2004, 304(5672), 838 CrossRef CAS PubMed.
- R. C. Hale, M. E. Seeley, M. J. L. Guardia, L. Mai and E. Y. Zeng, A global perspective on microplastics, J. Geophys. Res.: Oceans, 2020, 125(1), e2018JC014719 Search PubMed.
- M. A. Browne, T. Galloway and R. Thompson, Microplastic-an emerging contaminant of potential concern?, Integr. Environ. Assess. Manage., 2007, 3(4), 559–561 CrossRef PubMed.
- S. Xu, J. Ma, R. Ji, K. Pan and A. J. Miao, Microplastics in aquatic environments: occurrence, accumulation, and biological effects, Sci. Total Environ., 2020, 703, 134699 CrossRef CAS PubMed.
- K. Duis and A. Coors, Microplastics in the aquatic and terrestrial environment: sources (with a specific focus on personal care products), fate and effects, Environ. Sci. Eur., 2016, 28, 2 CrossRef PubMed.
- J. J. Duan, N. Bolan, Y. Li, S. Y. Ding, T. Atugoda, M. Vithanage, B. Sarkar, D. C. W. Tsang and M. B. Kirkham, Weathering of microplastics and interaction with other coexisting constituents in terrestrial and aquatic environments, Water Res., 2021, 196, 117011 CrossRef CAS PubMed.
- A. A. Horton and S. J. Dixon, Microplastics: an introduction to environmental transport processes, WIREs Water, 2018, 5(2), e1268 Search PubMed.
- I. Batool, A. Qadir, J. M. Levermore and F. J. Kelly, Dynamics of airborne microplastics, appraisal and distributional behaviour in atmosphere; a review, Sci. Total Environ., 2022, 806, 150745 CrossRef CAS PubMed.
- H. S. Auta, C. U. Emenike and S. H. Fauziah, Distribution and importance of microplastics in the marine environment: a review of the sources, fate, effects, and potential solutions, Environ. Int., 2017, 102, 165–176 CrossRef CAS PubMed.
- O. S. Alimi, J. F. Budarz, L. M. Hernandez and N. Tufenkji, Microplastics and nanoplastics in aquatic environments: aggregation, deposition, and enhanced contaminant transport, Environ. Sci. Technol., 2018, 52(4), 1704–1724 CrossRef CAS PubMed.
- W. F. Wang, J. Ge, X. Y. Yu and H. Li, Environmental fate and impacts of microplastics in soil ecosystems: progress and perspective, Sci. Total Environ., 2020, 708, 134841 CrossRef CAS PubMed.
- D. F. He, Y. M. Luo, S. B. Lu, M. T. Liu, Y. Song and L. L. Lei, Microplastics in soils: analytical methods, pollution characteristics and ecological risks, TrAC, Trends Anal. Chem., 2018, 109, 163–172 CrossRef CAS.
- B. L. Xu, F. Liu, Z. Cryder, D. Huang, Z. J. Lu, Y. He, H. Z. Wang, Z. M. Lu, P. C. Brookes, C. X. Tang, J. Gan and J. M. Xu, Microplastics in the soil environment: occurrence, risks, interactions and fate – a review, Crit. Rev. Environ. Sci. Technol., 2019, 50(21), 2175–2222 CrossRef.
- R. Dris, J. Gasperi, V. Rocher, M. Saad, N. Renault and B. Tassin, Microplastic contamination in an urban area: a case study in Greater Paris, Environ. Chem., 2015, 12, 592–599 CrossRef CAS.
- G. L. Chen, Z. L. Fu, H. R. Yang and J. Wang, An overview of analytical methods for detecting microplastics in the atmosphere, TrAC, Trends Anal. Chem., 2020, 130, 115981 CrossRef CAS.
- N. Habibi, S. Uddin, S. W. Fowler and M. Behbehani, Microplastics in the atmosphere: a review, J. Environ. Expo. Assess., 2022, 1, 6 Search PubMed.
- Y. L. Zhang, S. C. Kang, S. Allen, D. Allen, T. G. Gao and M. Sillanpaa, Atmospheric microplastics: a review on current status and perspectives, Earth-Sci. Rev., 2020, 203, 103118 CrossRef CAS.
- L. D. S. Galvão, E. M. SartiFernandes, R. R. Ferreira, D. D. S. Rosa and H. Wiebeck, Critical steps for microplastics characterization from the atmosphere, J. Hazard. Mater., 2022, 424, 127668 CrossRef PubMed.
- O. Mbachu, G. Jenkins, C. Pratt and P. Kaparaju, A new contaminant superhighway? a review of sources, measurement techniques and fate of atmospheric microplastics, Water, Air, Soil Pollut., 2020, 231, 85 CrossRef CAS.
- X. Luo, Z. Q. Wang, L. Yang, T. G. Gao and Y. L. Zhang, A review of analytical methods and models used in atmospheric microplastic research, Sci. Total Environ., 2020, 828, 154487 CrossRef PubMed.
- S. Huppertsberg and T. P. Knepper, Instrumental analysis of microplastics – benefits and challenges, Anal. Bioanal. Chem., 2018, 410, 6343–6352 CrossRef CAS PubMed.
- C. E. Enyoh, Q. Y. Wang, T. Chowdhury, W. Q. Wang, S. L. Lu, K. Xiao and Md. A. H. Chowdhury, New Analytical Approaches for Effective Quantification and Identification of Nanoplastics in Environmental Samples, Processes, 2021, 9(11), 2086 CrossRef CAS.
- S. Adhikari, V. Kelkar, R. Kumar and R. U. Halden, Methods and challenges in the detection of microplastics and nanoplastics: a mini-review, Polym. Int., 2021, 71(5), 543–551 CrossRef.
- N. P. Ivleva, Chemical Analysis of Microplastics and Nanoplastics: Challenges, Advanced Methods, and Perspectives, Chem. Rev., 2021, 121, 11886–11936 CrossRef CAS PubMed.
- M. Velimirovic, K. Tirez, S. Verstraelen, E. Frijns, S. Remy, G. Koppen, A. Rotander, E. Bolea-Fernandez and F. Vanhaecke, Mass spectrometry as a powerful analytical tool for the characterization of indoor airborne microplastics and nanoplastics, J. Anal. At. Spectrom., 2021, 36, 695–705 RSC.
- P. Li, Q. C. Li, Z. N. Hao, S. J. Yu and J. F. Liu, Analytical methods and environmental processes of nanoplastics, J. Environ. Sci., 2020, 94, 88–99 CrossRef PubMed.
- H. W. Cai, E. G. Xu, F. N. Du, R. L. Li, J. F. Liu and H. H. Shi, Analysis of environmental nanoplastics: progress and challenges, Chem. Eng. J., 2021, 410, 128208 CrossRef CAS.
- L. Q. Cai, J. D. Wang, J. P. Peng, T. Zhi, Z. W. Zhan, X. L. Tan and Q. Q. Chen, Characteristic of microplastics in the atmospheric fallout from Dongguan city, China: preliminary research and first evidence, Environ. Sci. Pollut. Res., 2017, 24, 24928–24935 CrossRef PubMed.
- Q. Zhou, C. G. Tian and Y. M. Luo, Various forms and deposition fluxes of microplastics identified in the coastal urban atmosphere (in Chinese), Chin. Sci. Bull., 2017, 62(33), 3902–3909 CrossRef.
- E. Gaston, M. Woo, C. Steele, S. Sukumaran and S. Anderson, Microplastics differ between indoor and outdoor air masses: insights from multiple microscopy methodologies, Appl. Spectrosc., 2020, 74(9), 1079–1098 CrossRef CAS PubMed.
- R. Dris, J. Gasperi, M. Saad, C. Mirande and B. Tassin, Synthetic fibers in atmospheric fallout: a source of microplastics in the environment?, Mar. Pollut. Bull., 2016, 102(1–2), 290–293 CrossRef PubMed.
- R. Dris, J. Gasperi, C. Mirande, C. Mandin, M. Guerrouache, V. Langlois and B. Tassin, A first overview of textile fibers, including microplastics, in indoor and outdoor environments, Environ. Pollut., 2017, 221, 453–458 CrossRef CAS PubMed.
- S. Dehghani, F. Moore and R. Akhbarizadeh, Microplastic pollution in deposited urban dust, Tehran metropolis, Iran, Environ. Sci. Pollut. Res., 2017, 24, 20360–20371 CrossRef CAS PubMed.
- A. T. Kaya, M. Yurtserver and S. C. Bayraktar, Ubiquitous exposure to microfiber pollution in the air, Eur. Phys. J. Plus, 2018, 133, 488 CrossRef.
- S. L. Wright, J. Ulke, A. Font, K. L. A. Chan and F. J. Kelly, Atmospheric microplastic deposition in an urban environment and an evaluation of transport, Environ. Int., 2020, 136, 105411 CrossRef CAS PubMed.
- K. Liu, T. N. Wu, X. H. Wang, Z. Y. Song, C. X. Zong, N. Wei and D. J. Li, Consistent transport of terrestrial microplastics to the ocean through atmosphere, Environ. Sci. Technol., 2019b, 53, 10612–10619 Search PubMed.
- X. H. Wang, C. J. Li, K. Liu, L. X. Zhu, Z. Y. Song and D. J. Li, Atmospheric microplastic over the South China Sea and East Indian Ocean: abundance, distribution and source, J. Hazard. Mater., 2020, 389, 121846 CrossRef CAS PubMed.
- Q. Zhang, Y. P. Zhao, F. N. Du, H. W. Cai, G. H. Wang and H. H. Shi, Microplastic fallout in different indoor environments, Environ. Sci. Technol., 2020, 54(11), 6530–6539 CrossRef CAS PubMed.
- S. Abbasi, B. Keshavarzi, F. Moore, A. Turner, F. J. Kelly, A. O. Dominguez and N. Jaafarzadeh, Distribution and potential health impacts of microplastics and microrubbers in air and street dusts from Asaluyeh County, Iran, Environ. Pollut., 2019, 244, 153–164 CrossRef CAS PubMed.
- C. G. Liu, J. Li, Y. L. Zhang, L. Wang, J. Deng, Y. Gao, L. Yu, J. J. Zhang and H. W. Sun, Widespread distribution of PET and PC microplastics in dust in urban China and their estimated human exposure, Environ. Int., 2019, 128, 116–124 CrossRef CAS PubMed.
- M. Klein and E. K. Fischer, Microplastic abundance in atmospheric deposition within the Metropolitan area of Hamburg, Germany, Sci. Total Environ., 2019, 685, 96–103 CrossRef CAS PubMed.
- K. Liu, X. H. Wang, T. Feng, P. Xu, L. X. Zhu and D. J. Li, Source and potential risk assessment of suspended atmospheric microplastics in Shanghai, Sci. Total Environ., 2019a, 675, 462–471 Search PubMed.
- K. Liu, X. H. Wang, N. Wei, Z. Y. Song and D. J. Li, Accurate quantification and transport estimation of suspended atmospheric microplastics in megacities: implications for human health, Environ. Int., 2019c, 132, 105127 Search PubMed.
- A. Vianello, R. L. Jensen, L. Liu and J. Vollertsen, Simulating human exposure to indoor airborne microplastics using a Breathing Thermal Manikin, Sci. Rep., 2019, 9, 8670 CrossRef PubMed.
- M. Trainic, J. M. Flores, I. Pinkas, M. L. Pedrotti, F. Lombard, G. Bourdin, G. Gorsky, E. Boss, Y. Rudich, A. Vardi and I. Koren, Airborne microplastic particles detected in the remote marine atmosphere, Commun. Earth Environ., 2020, 1, 64 CrossRef.
- X. Zhu, W. Huang, M. Z. Fang, Z. L. Liao, Y. Q. Wang, L. S. Xu, C. W. Shi, C. J. Lu, H. H. Deng, R. Dahlgren and X. Shang, Airborne Microplastic concentrations in five megacities of northern and southeast China, Environ. Sci. Technol., 2021, 55(19), 12871–12881 CAS.
- M. Beaurepaire, R. Dris, J. Gasperi and B. Tassin, Microplastics in the atmospheric compartment: a comprehensive review on methods, results on their occurrence and determining factors, Curr. Opin. Food Sci., 2021, 4, 159–168 CrossRef.
- J. Gasperi, S. L. Wright, R. Dris, F. Collard, C. Mandin, M. Guerrouache, V. Langlois, F. J. Kelly and B. Tassin, Microplastics in air: Are we breathing it in?, Curr. Opin. Environ. Sci. Health, 2018, 2, 1–5 CrossRef.
- C. Jonsson, O. L. Arturin, A. C. Hanning, R. Landin, E. Holimstrom and S. Roos, Microplastics shedding from textiles—Developing analytical method for measurement of shed material representing release during domestic washing, Sustainability, 2018, 10, 2457 CrossRef.
- S. Yukioka, S. Tanaka, Y. Nabetani, Y. Suzuki, T. Ushijima, S. Fujii, H. Takada, Q. V. Tran and S. Singh, Occurrence and characteristics of microplastics in surface road dust in Kusatsu (Japan), Da Nang (Vietnam), and Kathmandu (Nepal), Environ. Pollut., 2017, 256, 113447 CrossRef PubMed.
-
Q. Zhang, R. Wang, Y. Q. Shen, L. Zhan and Z. M. Xu, Characteristics of unorganized emissions of microplastics from road fugitive dust in urban mining bases, 2022, available at SSRN, DOI:10.2139/ssrn.4034523.
- P. J. Kole, A. J. Lohr, F. G. A. J. V. Belleghem and A. M. J. Ragas, Wear and tear of tyres: a stealthy source of microplastics in the environment, Int. J. Environ. Res. Public Health, 2017, 14(10), 1265 CrossRef PubMed.
-
R. Qiu, Y. Song, X. T. Zhang, B. Xie and D. F. He, Microplastics in urban environments: sources, pathways, and distribution, in Microplastics in Terrestrial Environments – Emerging Contaminants and Major Challenges, ed. D. He and Y. Luo, Springer Press, 2020, pp. 41–61 Search PubMed.
- T. Q. Thinh, T. T. N. Sang, T. Q. Viet, L. T. M. Tam, N. P. Dan, E. Strady and K. L. T. Chung, Preliminary assessment on the microplastic contamination in the atmospheric fallout in the Phuoc Hiep landfill, Cu Chi, Ho Chi Minh city, Vietnam J. Sci. Technol., 2020, 62(3), 83–89 CrossRef.
- K. Waldschlager, S. Lechthaler, G. Stauch and H. Schuttrumpf, The way of microplastic through the environment-Application of the source-pathway-receptor model (review), Sci. Total Environ., 2020, 713, 136584 CrossRef PubMed.
- P. J. He, L. Y. Chen, L. M. Shao, H. Shao, H. Zhang and F. Lu, Municipal solid waste (MSW) landfill: A source of microplastics? – evidence of microplastics in landfill leachate, Water Res., 2019, 159, 38–45 CrossRef CAS PubMed.
- S. Loppi, B. Roblin, L. Paoli and J. Aherne, Accumulation of airborne microplastics in lichens from a landfill dumping site (Italy), Sci. Rep., 2021, 11, 4564 CrossRef CAS PubMed.
- S. Kacprzak and L. D. Tijing, Microplastics in indoor environment: sources, mitigation and fate, J. Environ. Chem. Eng., 2022, 10(2), 107359 CrossRef CAS.
- S. Allen, D. Allen, K. Moss, G. L. Roux, V. R. Phoenix and J. E. Sonke, Examination of the ocean as a source for atmospheric microplastics, PLoS One, 2020, 15(5), e0232746 CrossRef CAS PubMed.
- S. Allen, D. Allen, V. R. Phoenix, G. L. Roux, P. D. Jimenez, A. Simonneau, S. Binet and D. Galop, Atmospheric transport and deposition of microplastics in a remote mountain catchment, Nat. Geosci., 2019, 12, 339–344 CrossRef CAS.
- X. H. Wang, K. Liu, L. X. Zhu, C. J. Li, Z. Y. Song and D. J. Li, Efficient transport of atmospheric microplastics onto the continent via the East Asian summer monsoon, J. Hazard. Mater., 2021, 414, 125477 CrossRef CAS PubMed.
- F. Petersen and J. A. Hubbart, The occurrence and transport of microplastics: the state of the science, Sci. Total Environ., 2021, 758, 143936 CrossRef CAS PubMed.
- R. Ahmed, A. K. Hamid, S. A. Krebsbach, J. Z. He and D. J. Wang, Critical review of microplastics removal from the environment, Chemosphere, 2020, 293, 133557 CrossRef PubMed.
- X. H. Huang, Y. Chen, Y. C. Meng, G. D. Liu and M. X. Yang, Are we ignoring the role of urban forests in intercepting atmospheric microplastics?, J. Hazard. Mater., 2022, 436, 129096 CrossRef CAS PubMed.
- M. Velimirovic, K. Tirez, S. Verstraelen, E. Frijns, S. Remy, G. Koppen, A. Rotander, E. Bolea-Fernandez and F. Vanhaecke, Mass spectrometry as a powerful analytical tool for the characterization of indoor airborne microplastics and nanoplastics, J. Anal. At. Spectrom., 2021, 36, 695–705 RSC.
- P. Liu, X. Zhan, X. W. Wu, J. L. Li, H. Y. Wang and S. X. Gao, Effect of weathering on environmental behavior of microplastics: Properties, sorption and potential risks, Chemosphere, 2020, 242, 125193 CrossRef CAS PubMed.
- Y. M. Huang, T. He, M. T. Yan, L. Yang, H. Gong, W. J. Wang, X. Qing and J. Wang, Atmospheric transport and deposition of microplastics in a subtropical urban environment, J. Hazard. Mater., 2021, 416(15), 126168 CrossRef CAS PubMed.
- L. F. Chen, S. L. Peng, J. G. Liu and Q. Q. Hou, Dry deposition velocity of total suspended particles and meteorological influence in four locations in Guangzhou, China, J. Environ. Sci., 2012, 24(4), 632–639 CrossRef.
- L. Lu, T. Luo, Y. Zhao, C. H. Cai, Z. W. Fu and Y. X. Jin, Interaction between microplastics and microorganism as well as gut microbiota: a consideration on environmental animal and human health, Sci. Total Environ., 2019, 667, 94–100 CrossRef CAS PubMed.
- M. Smith, D. C. Love, C. M. Rochman and R. A. Neff, Microplastics in seafood and the implications for human health, Curr. Environ. Health Rep., 2018, 5, 375–386 CrossRef CAS PubMed.
- S. E. Nelms, J. Barnett, A. Brownlow, N. J. Davison, R. Deaville, T. S. Galloway, P. K. Lindeque, D. Santillo and B. J. Godley, Microplastics in marine mammals stranded around the British coast: ubiquitous but transitory?, Sci. Rep., 2019, 9, 1075 CrossRef CAS PubMed.
- C. M. Boerger, G. L. Lattin, S. L. Moore and C. J. Moore, Plastic ingestion by planktivorous fishes in the North Pacific Central Gyre, Mar. Pollut. Bull., 2010, 60(12), 2275–2278 CrossRef CAS PubMed.
- S. Gundogdu, C. Cevik and N. T. Atas, Stuffed with microplastics: Microplastic occurrence in traditional stuffed mussels sold in the Turkish market, Food Biosci., 2020, 37, 100715 CrossRef CAS.
- C. Y. Chen, T. H. Lu, Y. F. Yang and C. M. Liao, Marine mussel-based biomarkers as risk indicators to assess oceanic region-specific microplastics impact potential, Ecol. Indic., 2021, 120, 106915 CrossRef CAS.
- L. J. Zantis, E. L. Carroll, S. E. Nelms and T. Bosker, Marine mammals and microplastics: a systematic review and call for standardization, Environ. Pollut., 2021, 269, 116142 CrossRef CAS PubMed.
- M. Cole, P. Lindeque, E. Fileman, C. Hhalsband, R. Goodhead, J. Moger and T. S. Galloway, Microplastic ingestion by zooplankton, Environ. Sci. Technol., 2013, 47(12), 6646–6655 CrossRef CAS PubMed.
- C. C. Suckling, Responses to environmentally relevant microplastics are species-specific with dietary habit as a potential sensitivity indicator, Sci. Total Environ., 2021, 751, 142341 CrossRef CAS PubMed.
-
N. F. Rahim and K. Yaqin, Histological Alteration of Green Mussel Perna viridis Organs Exposed to Microplastics, Squalen Bulletin of Marine and Fisheries Postharvest and Biotechnology, 2022, vol. 17(1) Search PubMed.
- R. Qiao, C. Sheng, Y. F. Lu, Y. Zhang, H. Q. Ren and B. Lemos, Microplastics induce intestinal inflammation, oxidative stress, and disorders of metabolome and microbiome in zebrafish, Sci. Total Environ., 2019, 662, 246–253 CrossRef CAS PubMed.
- P. Kolandhasamy, L. Su, J. N. Li, X. Y. Qu, K. Jabeen and H. H. Shi, Adherence of microplastics to soft tissue of mussels: A novel way to uptake microplastics beyond ingestion, Sci. Total Environ., 2018, 610–611, 635–640 CrossRef CAS PubMed.
- V. A. Lindborg, J. F. Ledbetter, J. M. Walat and C. Moffett, Plastic consumption and diet of Glaucous-winged Gulls (Larus glaucescens), Mar. Pollut. Bull., 2012, 64(11), 2351–2356 CrossRef CAS PubMed.
- M. L. Brandao, K. M. Braga and J. L. Luque, Marine debris ingestion by Magellanic penguins, Spheniscus magellanicus (Aves: Sphenisciformes), from the Brazilian coastal zone, Mar. Pollut. Bull., 2011, 62(10), 2246–2249 CrossRef CAS PubMed.
- M. T. He, M. T. Yan, X. F. Chen, X. K. Wang, H. Hong, W. J. Wang and J. Wang, Bioavailability and toxicity of microplastics to zooplankton, Gondwana Res., 2022, 108, 120–126 CrossRef CAS.
- N. B. Hartmann, S. Rist, J. Bodin, L. H. Jensen, S. N. Schmidt, P. Mayer, A. Meibom and A. Baun, Microplastics as vectors for environmental contaminants: exploring sorption, desorption, and transfer to biot, Integr. Environ. Assess. Manage., 2017, 13(3), 488–493 CrossRef PubMed.
- C. Romera-Castillo, M. Pinto, T. M. Langer, X. A. Alvarez-Salgado and G. J. Herndl, Dissolved organic carbon leaching from plastics stimulates microbial activity in the ocean, Nat. Commun., 2018, 9, 1430 CrossRef PubMed.
- L. Z. Miao, P. F. Wang, J. Hou, Y. Yao, Z. L. Liu, S. Q. Liu and T. F. Li, Distinct community structure and microbial functions of biofilms colonizing microplastics, Sci. Total Environ., 2019, 650(2), 2395–2402 CrossRef CAS PubMed.
- M. Andres, K. Rojas-Jimenez and H. Grossart, Collateral effects of microplastic pollution on aquatic microorganisms: an ecological perspective, TrAC, Trends Anal. Chem., 2019, 112, 234–240 CrossRef.
- A. R. McCormick, T. J. Hoellein, M. G. London, J. Hittie, J. W. Scott and J. J. Kelly, Microplastic in surface waters of urban rivers: concentration, sources, and associated bacterial assemblages, Ecosphere, 2016, 7(11), e01556 CrossRef.
- Y. Y. Yang, W. Z. Liu, Z. L. Zhang, H. Grossart and G. M. Gadd, Microplastics provide new microbial niches in aquatic environments, Appl. Microbiol. Biotechnol., 2020, 104, 6501–6511 CrossRef CAS PubMed.
- M. T. Gong, G. Q. Yang, L. Zhuang and E. Y. Zeng, Microbial biofilm formation and community structure on low-density polyethylene microparticles in lake water microcosms, Environ. Pollut., 2019, 252, 94–102 CrossRef CAS PubMed.
- Y. F. Mao, H. N. Ai, Y. Chen, Z. Y. Zhang, P. Zeng, L. Kang, W. Li, W. K. Gu, Q. He and H. Li, Phytoplankton response to polystyrene microplastics: Perspective from an entire growth period, Chemosphere, 2018, 208, 59–68 CrossRef CAS PubMed.
- J. N. Hitchcock, Microplastics can alter phytoplankton community composition, Sci. Total Environ., 2022, 819, 153074 CrossRef CAS PubMed.
- J. Peller, M. B. Nevers, M. Byappanahalli, C. Nelson, B. G. Babu, M. A. Evans, E. Kostelnik, M. Keller, J. Johnston and S. Shidler, Sequestration of microfibers and other microplastics by green algae, Cladophora, in the US Great Lakes, Environ. Pollut., 2021, 276, 116695 CrossRef CAS PubMed.
- C. Zhang, M. F. Jian, Y. M. Chen, Q. Q. Chen, X. F. He, M. Y. Cong and W. J. Yang, Effects of polystyrene microplastics (PS-MPs) on the growth, physiology, and biochemical characteristics of Hydrilla verticillata, Chin. J. Appl. Ecol., 2021, 32(1), 317–325 Search PubMed.
- M. D. H. J. Senavirathna, Z. Z. Liu and T. Fujino, Root adsorption of microplastic particles affects the submerged freshwater macrophyte Egeria densa, Water, Air, Soil Pollut., 2022, 233, 80 CrossRef CAS.
- B. Baensch-Baltruschat, B. Kocher, F. Stock and G. Reifferschid, Tyre and road wear particles (TRWP) – a review of generation, properties, emissions, human health risk, ecotoxicity, and fate in the environment, Sci. Total Environ., 2020, 733, 137823 CrossRef CAS PubMed.
- L. Z. Li, Y. M. Luo, R. J. Li, Q. Zhou, W. J. G. M. Peijnenburg, N. Yin, J. Yang, C. Tu and Y. C. Zhang, Nat. Sustain., 2020, 3, 929–937 CrossRef.
- D. L. Huang, X. Y. Wang, L. S. Yin, S. Chen, J. X. Tao, W. Zhou, H. J. Chen, G. X. Zhang and R. H. Xiao, Research progress of microplastics in soil-plant system: ecological effects and potential risks, Sci. Total Environ., 2022, 812, 151487 CrossRef CAS PubMed.
- A. A. D. S. Machado, C. W. Lau, J. Till, W. Kloas, A. Lehmann, R. Becher and M. C. Rillig, Impacts of microplastics on the soil biophysical environment, Environ. Sci. Technol., 2018, 52(17), 9656–9665 CrossRef PubMed.
- D. L. Huang, X. Y. Wang, L. S. Yin, S. Chen, J. X. Tao, W. Zhou, H. J. Chen, G. X. Zhang and R. H. Xiao, Research progress of microplastics in soil-plant system: ecological effects and potential risks, Sci. Total Environ., 2022, 812, 151487 CrossRef CAS PubMed.
- A. A. D. S. Machado, C. W. Lau, W. Kloas, J. B. J. B. Bachelier, E. Faltin, R. Recker, A. S. Gorlich and M. C. Rillig, Microplastics can change soil properties and affect plant performance, Environ. Sci. Technol., 2019, 53(10), 6044–6052 CrossRef PubMed.
- J. Wang, M. K. Huang, Q. Wang, Y. Z. Sun, Y. R. Zhao and Y. Huang, LDPE microplastics significantly alter the temporal turnover of soil microbial communities, Sci. Total Environ., 2020, 726, 138682 CrossRef CAS PubMed.
- M. C. Rillig, A. A. D. S. Machado, A. Lehmann and U. Klumper, Evolutionary implications of microplastics for soil biota, Environ. Chem., 2018, 16(1), 3–7 CrossRef PubMed.
- Y. Z. Sun, C. X. Duan, N. Cao, X. F. Li, X. M. Li, Y. M. Chen, Y. Huang and J. Wang, Effects of microplastics on soil microbiome: The impacts of polymer type, shape, and concentration, Sci. Total Environ., 2022, 806, 150516 CrossRef CAS PubMed.
- X. F. Jiang, Y. Q. Chang, T. Zhang, Y. Qiao, G. Klobucar and M. Li, Toxicological effects of polystyrene microplastics on earthworm (Eisenia fetida), Environ. Pollut., 2020, 259, 113896 CrossRef CAS PubMed.
- D. Zhu, Q. L. Chen, X. L. An, X. R. Yang, P. Christie, X. Ke, L. H. Wu and Y. G. Zhu, Exposure of soil collembolans to microplastics perturbs their gut microbiota and alters their isotopic composition, Soil Biol. Biochem., 2018, 116, 302–310 CrossRef CAS.
- D. Lin, G. R. Yang, P. P. Dou, S. H. Qian, L. Zhao, Y. C. Yang and N. Fanin, Microplastics negatively affect soil fauna but stimulate microbial activity: insights from a field-based microplastic addition experiment, Proc. R. Soc. B, 2020, 287, 20201268 CrossRef CAS PubMed.
- J. Ding, C. X. Liu, Q. F. Chen, Z. Y. Zhang, J. L. Han, A. Liang, D. Zhu, H. T. Wang, M. Lv and L. X. Chen, Extractable additives in microplastics: a hidden threat to soil fauna, Environ. Pollut., 2022, 294, 118647 CrossRef CAS PubMed.
- Y. L. Zhang, X. T. Zhang, X. Y. Li and D. F. He, Interaction of microplastics and soil animals in agricultural ecosystems, Curr. Opin. Environ. Sci. Health, 2022, 26, 100327 CrossRef.
- J. Li, Y. Song and Y. B. Cai, Focus topics on microplastics in soil: Analytical methods, occurrence, transport, and ecological risks, Environ. Pollut., 2020, 257, 113570 CrossRef CAS PubMed.
- D. D. Cao, X. Wang, X. X. Luo, G. C. Liu and H. Zheng, Effects of polystyrene microplastics on the fitness of earthworms in an agricultural soil, IOP Conf. Ser.: Earth Environ. Sci., 2017, 61, 012148 CrossRef.
- K. Liu, X. H. Wang, Z. Y. Song, N. Wei and D. J. Li, Terrestrial plants as a potential temporary sink of atmospheric microplastics during transport, Sci. Total Environ., 2020, 742, 140523 CrossRef CAS PubMed.
- X. S. Luo, H. J. Bing, Z. X. Luo, Y. J. Wang and L. Jin, Impacts of atmospheric particulate matter pollution on environmental biogeochemistry of trace metals in soil-plant system: a review, Environ. Pollut., 2019, 255, 113138 CrossRef CAS PubMed.
- J. P. Lian, W. T. Liu, L. Z. Meng, J. N. Wu, L. Chao, A. Zeb and Y. B. Sun, Foliar-applied polystyrene nanoplastics (PSNPs) reduce the growth and nutritional quality of lettuce (Lactuca sativa L.), Environ. Pollut., 2021, 280, 116978 CrossRef CAS PubMed.
- C. Spano, S. Muccifora, M. R. Castiglione, L. Bellani, S. Bottega and L. Giorgetti, Polystyrene nanoplastics affect seed germination, cell biology and physiology of rice seedlings in-short term treatments: evidence of their internalization and translocation, Plant Physiol. Biochem., 2022, 172, 158–166 CrossRef CAS PubMed.
- M. C. Rillig, A. Lehmann, A. A. D. S. Machado and G. W. Yang, Microplastic effects on plants, New Phytol., 2019, 223(3), 1066–1070 CrossRef PubMed.
- M. E. Seeley, B. Song, R. Passie and R. C. Hale, Microplastics affect sedimentary microbial communities and nitrogen cycling, Nat. Commun., 2020, 11, 2372 CrossRef CAS PubMed.
- F. R. Meng, X. M. Yang, M. Riksen and V. Geissen, Effect of different polymers of microplastics on soil organic carbon and nitrogen – a mesocosm experiment, Environ. Res., 2022, 204, 111938 CrossRef CAS PubMed.
- B. Gao, H. Y. Yao, Y. Y. Li and Y. Z. Zhu, Microplastic Addition Alters the Microbial Community Structure and Stimulates Soil Carbon Dioxide Emissions in Vegetable-Growing Soil, Environ. Toxicol. Chem., 2021, 40(2), 352–365 CrossRef CAS PubMed.
- X. W. Ren, J. C. Tang, X. M. Liu and Q. L. Liu, Effects of microplastics on greenhouse gas emissions and the microbial community in fertilized soil, Environ. Pollut., 2020, 256, 113347 CrossRef CAS PubMed.
- D. L. Huang, X. Y. Wang, L. S. Yin, S. Chen, J. X. Tao, W. Zhou, H. J. Chen, G. X. Zhang and R. H. Xiao, Research progress of microplastics in soil-plant system: ecological effects and potential risks, Sci. Total Environ., 2022, 812, 151487 CrossRef CAS PubMed.
- A. D. Vethaak and J. Legler, Microplastics and human health, Science, 2021, 371(6530), 672–674 CrossRef CAS PubMed.
- J. C. Prata, Airborne microplastics: consequences to human health?, Environ. Pollut., 2018, 234, 115–126 CrossRef CAS PubMed.
- Y. Wang, J. Huang, F. X. Zhu and S. D. Zhou, Airborne microplastics: a review on the occurrence, migration and risks to humans, Bull. Environ. Contam. Toxicol., 2021, 107, 657–664 CrossRef CAS PubMed.
- Z. L. Liao, X. L. Ji, Y. Ma, B. Q. Lv, W. Huang, X. Zhu, M. Z. Fang, Q. Wang, X. D. Wang, R. Dahlgren and X. Shang, Airborne microplastics in indoor and outdoor environments of a coastal city in Eastern China, J. Hazard. Mater., 2021, 417, 126007 CrossRef CAS PubMed.
- J. L. Pauly, S. J. Stegmeier, H. A. Allaart, R. T. Cheney, P. J. Zhang, A. G. Mayer and R. J. Streck, Inhaled cellulosic and plastic fibers found in human lung tissue, Cancer Epidemiol., Biomarkers Prev., 1998, 7(5), 419–428 CAS.
- A. Vianello, R. L. Jensen and J. Vollertsen, Simulating human exposure to indoor airborne microplastics using a Breathing Thermal Manikin, Sci. Rep., 2019, 9, 8670 CrossRef PubMed.
- L. F. Amato-Lourenco, R. Carvalho-Oliveira, G. R. Junior, L. d. S. Galvao, R. A. Ando and T. Mauad, Presence of airborne microplastics in human lung tissue, J. Hazard. Mater., 2021, 416, 126124 CrossRef CAS PubMed.
- T. L. Alderete, R. B. Jones, Z. H. Chen, J. S. Kim, R. HAbre, F. Lurmann, F. D. Gilliland and M. I. Goran, Exposure to traffic-related air pollution and the composition of the gut microbiota in overweight and obese adolescents, Environ. Res., 2018, 161, 472–478 CrossRef CAS PubMed.
- N. S. Soltani, M. P. Taylor and S. P. Wilson, Quantification and exposure assessment of microplastics in Australian indoor house dust, Environ. Pollut., 2021, 283, 117064 CrossRef CAS PubMed.
- W. Shi, Y. Cao, X. L. Chai, Q. Zhao, Y. X. Geng, D. Liu and S. L. Tian, Potential health risks of the interaction of microplastics and lung surfactant, J. Hazard. Mater., 2022, 429, 128109 CrossRef CAS PubMed.
- K. E. Goodman, J. T. Hare, Z. I. Khamis, T. Hua and Q. A. Sang, Exposure of human lung cells to polystyrene microplastics significantly retards cell proliferation and triggers morphological changes, Chem. Res. Toxicol., 2021, 34(4), 1069–1081 Search PubMed.
- M. K. Xu, G. Halimu, Q. R. Zhang, Y. B. Song, X. H. Fu, Y. Q. Li, Y. S. Li and H. W. Zhang, Internalization and toxicity: a preliminary study of effects of nanoplastic particles on human lung epithelial cell, Sci. Total Environ., 2019, 694, 133794 CrossRef CAS PubMed.
- M. S. Yee, L. Hii, C. K. Looi, W. Lim, S. Wong, Y. Kok, B. Tan, C. Wong and C. Leong, Impact of microplastics and nanoplastics on human health, Nanomaterials, 2021, 11(2), 496 CrossRef CAS PubMed.
- C. D. Dong, C. W. Chen, Y. C. Chen, H. H. Chen, J. S. Lee and C. H. Lin, Polystyrene microplastic particles: In vitro pulmonary toxicity assessment, J. Hazard. Mater., 2020, 385, 121575 CrossRef CAS PubMed.
- K. L. Sun, Y. Song, F. L. He, M. Y. Jing, J. C. Tang and R. T. Liu, A review of human and animals exposure to polycyclic aromatic hydrocarbons: health risk and adverse effects, photo-induced toxicity and regulating effect of microplastics, Sci. Total Environ., 2021, 773, 145403 CrossRef CAS PubMed.
- V. Godoy, G. Blazquez, M. Calero, L. Quesada and M. A. Martin-Lara, The potential of microplastics as carriers of metals, Environ. Pollut., 2019, 255(3), 113363 CrossRef CAS PubMed.
- A. Ragusa, A. Svelato, C. Santacroce, P. Catalano, V. Notarstefano, O. Carnevali, F. Papa, M. C. A. Rongioletti, F. Baiocco, S. Draghi, E. D'Amore, D. Rinaldo, M. Matta and E. Giorgini, Plasticenta: first evidence of microplastics in human placenta, Environ. Int., 2021, 146, 106274 CrossRef CAS PubMed.
- J. C. Prata, Airborne microplastics: consequences to human health?, Environ. Pollut., 2018, 234, 115–126 CrossRef CAS PubMed.
- A. F. R. M. Ramsperger, V. K. B. Narayana, W. Gross, J. Mohanraj, M. Thelakkat, A. Greiner, H. Schmalz, H. Kress and C. Laforsch, Environmental exposure enhances the internalization of microplastic particles into cells, Sci. Adv., 2020, 6(50), eabd1211 CrossRef CAS PubMed.
- A. J. Thorley, P. Ruenraroengsak, T. E. Potter and T. D. Tetley, Critical determinants of uptake and translocation of nanoparticles by the human pulmonary alveolar epithelium, ACS Nano, 2014, 8(11), 11778–11789 CrossRef CAS PubMed.
- A. Peters, B. Veronesi, L. Calderon-Garciduenas, P. Gehr, L. C. Chen, M. Geiser, W. Reed, B. Rothen-Rutishauser, S. Schurch and H. Schulz, Translocation and potential neurological effects of fine and ultrafine particles a critical update, Part. Fibre Toxicol., 2006, 3, 13 CrossRef PubMed.
- G. F. Schirinzi, I. Perez-Pomeda, J. Sanchis, C. Rossini, M. Farre and D. Barcelo, Cytotoxic effects of commonly used nanomaterials and microplastics on cerebral and epithelial human cells, Environ. Res., 2017, 159, 579–587 CrossRef CAS PubMed.
- S. A. Mason, V. G. Welch and J. Neratko, Synthetic polymer contamination in bottled water, Front. Chem., 2018, 6, 407 CrossRef PubMed.
- M. Kosuth, S. A. Mason and E. V. Wattenberg, Anthropogenic contamination of tap water, beer, and sea salt, PLoS One, 2018, 13(4), e0194970 CrossRef PubMed.
- D. Schymanski, C. Goldbeck, H. U. Humpf and P. Furst, Analysis of microplastics in water by micro-Raman spectroscopy: release of plastic particles from different packaging into mineral water, Water Res., 2018, 129, 154–162 CrossRef CAS PubMed.
- G. Liebezeit and E. Liebezeit, Synthetic particles as contaminants in German beers, Food Addit. Contam., Part A: Chem., Anal., Control, Exposure Risk Assess., 2014, 31(9), 1574–1578 CrossRef CAS PubMed.
- I. Hantoro, A. J. Lohr, F. G. A. J. V. Belleghrm, B. Widianarko and A. M. J. Ragas, Microplastics in coastal areas and seafood: implications for food safety, Food Addit. Contam., Part A: Chem., Anal., Control, Exposure Risk Assess., 2015, 36(5), 674–711 CrossRef PubMed.
- C. Walkinshaw, P. K. Lindeque, R. Thompson, T. Tolhurst and M. Cole, Microplastics and seafood: lower trophic organisms at highest risk of contamination, Ecotoxicol. Environ. Saf., 2020, 190, 110066 CrossRef CAS PubMed.
- A. Luqman, H. Nugrahapraja, R. A. Wahyuono, I. Islami, M. H. Haekal, Y. Fardiansyah, B. Q. Putri, F. I. Amalludin, E. A. Rofiqa, F. Gotz and A. T. Wibowo, Microplastic Contamination in Human Stools, Foods, and Drinking Water Associated with Indonesian Coastal Population, Environments, 2021, 8(12), 138 CrossRef.
- J. J. Zhang, L. Wang, L. Trasande and K. Kannan, Occurrence of polyethylene terephthalate and polycarbonate microplastics in infant and adult feces, Environ. Sci. Technol., 2021, 8(11), 989–994 CAS.
- Y. S. Ibrahim, S. T. Anuar, A. A. Azmi, W. M. A. W. M. Khalik, S. Lehata, S. R. Hamzah, D. Ismail, Z. F. Ma, A. Dzulkarnaen, Z. Zakaria, N. Mustaffa, S. E. T. Sharif and Y. Y. Lee, Detection of microplastics in human colectomy specimens, J. Gastroenterol. Hepatol., 2021, 5(1), 116–121 Search PubMed.
- G. Fackelmann and S. Sommer, Microplastics and the gut microbiome: how chronically exposed species may suffer from gut dysbiosis, Mar. Pollut. Bull., 2019, 143, 193–203 CrossRef CAS PubMed.
- V. Stock, C. Fahrenson, A. Thuenemann, M. H. Donmez, L. Voss, L. Bohmert, A. Braeuning, A. Lampen and H. Sieg, Impact of artificial digestion on the sizes and shapes of microplastic particles, Food Chem. Toxicol., 2020, 135, 111010 CrossRef CAS PubMed.
- F. F. Wang, Y. Y. Yu, H. W. Wu, W. N. Wu, L. Wang, L. H. An and W. Q. Cai, Microplastics in spotted seal cubs (Phoca largha): Digestion after ingestion?, Sci. Total Environ., 2021, 785(1), 147426 CrossRef CAS.
- W. T. Huang, H. Yin, Y. Y. Yang, L. Z. Jin, G. N. Lu and Z. Dang, Influence of the co-exposure of microplastics and tetrabromobisphenol A on human gut: Simulation in vitro with human cell Caco-2 and gut microbiota, Sci. Total Environ., 2021, 778, 146264 CrossRef CAS PubMed.
- V. Stock, L. Böhmert, E. Lisicki, R. Block, J. Cara-Carmona, L. K. Pack, R. Selb, D. Lichtenstein, L. Voss, C. J. Henderson, E. Zabinsky, H. Sieg, A. Braeuning and A. Lampen, Uptake and effects of orally ingested polystyrene microplastic particles in vitro and in vivo, Arch. Toxicol., 2019, 93, 1817–1833 CrossRef CAS PubMed.
- Y. F. Deng, Y. Zhang, B. Lemos and H. Q. Ren, Tissue accumulation of microplastics in mice and biomarker responses suggest widespread health risks of exposure, Sci. Rep., 2017, 7, 46687 CrossRef PubMed.
- J. Liu, M. Lv, A. Sun, J. Ding, Y. Q. Wang, X. B. Chang and L. X. Chen, Exposure to microplastics reduces the bioaccumulation of sulfamethoxazole but enhances its effects on gut microbiota and the antibiotic resistome of mice, Chemosphere, 2022, 294, 133810 CrossRef CAS PubMed.
- V. Godoy, A. Martínez-Férez, M. Á. Martín-Lara, J. A. Vellido-Pérez, M. Calero and G. Blázquez, Microplastics as Vectors of chromium and lead during dynamic simulation of the human gastrointestinal tract, Sustainability, 2020, 12(11), 4792 CrossRef CAS.
- E. Fournier, L. Etienne-Mesmin, C. Grootaert, L. Jelsbak, K. Syberg, S. Blanquet-Diot and M. Mercier-Bonin, Microplastics in the human digestive environment: a focus on the potential and challenges facing in vitro gut model development, J. Hazard. Mater., 2021, 778, 146264 Search PubMed.
- Y. L. Liao and J. Y. Yang, Microplastic serves as a potential vector for Cr in an in-vitro human digestive model, Sci. Total Environ., 2020, 703, 134805 CrossRef CAS PubMed.
- R. Chen, B. Hu, Y. Liu, J. X. Xu, G. S. Yang, D. D. Xu and C. Y. Chen, Beyond PM2.5: the role of ultrafine particles on adverse health effects of air pollution, Biochim. Biophys. Acta, Gen. Subj., 2016, 1860(12), 2844–2855 CrossRef CAS PubMed.
- E. Araviiskaia, E. Berardesca, T. Bieber, G. Gontijo, M. S. Viera, L. Marrot, B. Chuberre and B. Dreno, The impact of airborne pollution on skin, J. Eur. Acad. Dermatol. Venereol., 2019, 33(8), 1496–1505 CrossRef CAS PubMed.
- L. T. Ngoc, D. Park, Y. Lee and Y. Lee, Systematic review and meta-analysis of human skin diseases due to particulate matter, Int. J. Environ. Res. Public Health, 2017, 14(12), 1458 CrossRef PubMed.
- K. E. Kim, D. Cho and H. J. Park, Air pollution and skin diseases: adverse effects of airborne particulate matter on various skin diseases, Life Sci., 2016, 152, 126–134 CrossRef CAS PubMed.
- A. Branchner, D. Fragouli, I. F. Duarte, P. M. A. Farias, S. Dembski, M. Ghosh, I. Barisic, D. Zdzieblo, J. Vanoirbeek, P. Schwabl and W. Neuhaus, Assessment of human health risks posed by nano-and microplastics is currently not feasible, Int. J. Environ. Res. Public Health, 2020, 17(23), 8832 CrossRef PubMed.
- K. D. Cox, G. A. Covernyon, H. L. Davies, J. F. Dower, F. Juanes and S. E. Dudas, Human consumption of microplastics, Environ. Sci. Technol., 2019, 53(12), 7068–7074 CrossRef CAS PubMed.
- Y. M. Huang, X. Qing, W. J. Wang, G. Han and J. Wang, Mini-review on current studies of airborne microplastics: analytical methods, occurrence, sources, fate and potential risk to human beings, TrAC, Trends Anal. Chem., 2020, 125, 115821 CrossRef CAS.
- C. E. Enyoh, A. W. Verla, E. N. Verla, F. Chizoruolbe and C. E. Amaobi, Airborne microplastics: a review study on method for analysis, occurrence, movement and risks, Environ. Monit. Assess., 2019, 191, 668 CrossRef CAS PubMed.
-
F. Dang, Q. Y. Wang, Y. N. Huang, Y. J. Wang and B. S. Xing, Key knowledge gaps for One Health approach to mitigate nanoplastic risks, Eco-Environment & Health, 2022, DOI:10.1016/j.eehl.2022.02.001.
|
This journal is © The Royal Society of Chemistry 2022 |
Click here to see how this site uses Cookies. View our privacy policy here.