DOI:
10.1039/C9QM00659A
(Research Article)
Mater. Chem. Front., 2020,
4, 517-523
Microwave-assisted in situ large scale synthesis of a carbon dots@g-C3N4 composite phosphor for white light-emitting devices†
Received
25th October 2019
, Accepted 9th December 2019
First published on 24th December 2019
Abstract
Sustainable, environment-friendly phosphors produced from cheap and readily available precursor materials using green preparation processes are in high demand for lighting applications. Herein, a composite phosphor comprising carbon dots (CDs) and graphitic carbon nitride (g-C3N4) is prepared on a large scale from citric acid and urea in water, through a green microwave assisted in situ heating method. An optimized CDs@g-C3N4 composite with a 1 wt% loading of CDs shows a strong green emission under 410 nm excitation, with a photoluminescence quantum yield of 62% in the solid state. Using this composite as a phosphor in the color conversion layer, a white light emitting diode with a power efficiency of up to 42 lm W−1 is fabricated.
Introduction
Phosphors are highly demanded in the lighting industry, particularly for white light emitting diodes (WLEDs).1 Currently used commercial phosphors are mostly based on rare-earth materials, which experience issues of severe exploitation and less environmentally friendly refining, and may face a serious shortage in supply.2 It is thus of importance to look for alternative sustainable phosphors, which are to be produced using green preparation processes, and from readily available resources.
Carbon dots (CDs) have recently attracted attention in this respect owing to their tunable fluorescence emission, biocompatibility, low toxicity and facile preparation.3–13 Increasing efforts have been devoted towards overcoming the luminescence quenching of CDs in the solid state for WLED applications. Wang et al. mixed CDs with polystyrene to obtain a CD-based white emissive phosphor with a photoluminescence quantum yield (PLQY) of 25%, and made a WLED with a power efficiency of 14.8 lm W−1.14 Wang et al. designed a MOF material from CDs and Zr(IV) with white emission at 365 nm excitation with a PLQY of 37%, and fabricated a WLED with a power efficiency of 1.7 lm W−1.15 Shen et al. embedded CDs in trisodium citrate crystals to prepare a green emissive phosphor with a PLQY of 21%, and fabricated a WLED with a power efficiency of 6.9 lm W−1.16 We combined CDs with starch, polyvinylalcohol, BaSO4, NaCl, sodium silicate, silica and branched polyethylenimine to prepare phosphors with PLQYs ranging from 26% to 60%,17–23 and WLEDs with power efficiencies in the range of 8–28 lm W−1. Even though the PLQYs of CDs in the solid state have been gradually increased, the performances of CD-based WLEDs are still far from practical requirements (60–90 lm W−1) due to their low device power efficiency. Thus, there is still a need to develop new methods towards strongly emissive CD-based phosphors, and to improve the performance of CD-based WLEDs.
Graphitic carbon nitride (g-C3N4) is a stable material which has been proved to be an excellent catalyst for photodecomposition of water;24,25 it can be easily synthesized from low cost and environment-friendly urea.26 The band gap of bulk g-C3N4 is about 2.7 eV, while g-C3N4 nanosheets may have wider band gaps, which result in blue emission;27 they can be used as a matrix for preparing luminescent composites. Chen et al. reported a phenyl-modified g-C3N4-based green emissive phosphor with a PLQY of 38%.28
Herein, we have developed an in situ large scale synthetic procedure towards a strongly emissive CDs@g-C3N4 composite phosphor with its PLQY reaching 62%, via two-step microwave-assisted heating of citric acid and urea precursors. Using this sustainable and low cost synthesized CDs@g-C3N4 phosphor, WLEDs with power efficiencies up to 42 lm W−1 have been realized, which makes an important step towards practical lighting application of CDs.
Results and discussion
CDs were synthesized from citric acid and urea via a microwave-assisted heating method, following our previous work.29 Briefly, 6 g urea and 3 g citric acid were dissolved in 30 ml of deionized water, and heated for 3–4 min in a microwave oven to form a dark-brown CD solid, with a chemical yield of approximately 13.0%. This solid could be easily dispersed in water, providing a transparent solution with a yellow color under daylight, which showed green emission under UV light (insets in Fig. S1, ESI†). UV-vis absorption and PL spectra (405 nm excitation) of CDs dispersed in water are shown in Fig. S1 (ESI†); the PLQY was 15% under 405 nm excitation. g-C3N4 was prepared from urea as follows: 10 g urea was dissolved in 20 ml of deionized water, followed by microwave-assisted heating for 3–4 min.26
To produce composites of CDs with g-C3N4 (denoted CDs@g-C3N4 hereafter) from citric acid and urea, a two-step microwave-assisted heating method was developed, as illustrated in Fig. 1 and Fig. S2 (ESI†). First, appropriate amounts of citric acid (9–216 mg) and urea (18–432 mg) with a weight ratio of 1
:
2 were dissolved in 10 ml of water, followed by the first-step microwave heating for 3–4 min, which resulted in the formation of a non-emissive black powder comprising aggregated CDs. In the next step, 20 ml of 0.5 g ml−1 urea aqueous solution was added to dissolve this black powder and to form a transparent yellow solution. This solution was treated with the second-step microwave heating for 3–4 min, resulting in the formation of a green emissive powder. By controlling the ratio of the initial amount of citric acid and the total amount of urea, CDs@g-C3N4 composites with 0.5–12 wt% loading amounts of CDs were obtained, with chemical yields of approximately 7.0–7.4%. During the second step of the microwave heating process, the abundant urea forms g-C3N4, whereas CDs become embedded within the g-C3N4 network, which prevents their aggregation-induced luminescence quenching. Such a microwave-assisted method offers combined advantages of a low cost, fast and large-scale production of CD-based phosphors: more than 20 g of CDs@g-C3N4 composite material can be easily prepared as one batch, as presented in Fig. 2a. Under UV light, this composite exhibits strong green fluorescence, indicating the successful incorporation of CDs within g-C3N4 (Fig. 2b). Fig. 2c shows the fluorescence image of the CDs@g-C3N4 composite with 1 wt% CD loading concentration. The CDs@g-C3N4 composite with its particle size distribution ranging from 5 to 40 μm exhibits homogeneous intense green emission under blue light, demonstrating the uniform composition of CDs within g-C3N4. As expected, the color of the CDs@g-C3N4 composite becomes gradually darker upon increasing the CD loading concentration, while the emission was first enhanced and then decreased (Fig. 2d).
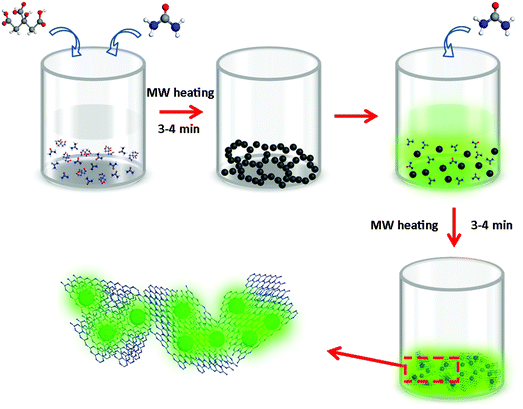 |
| Fig. 1 Schematic representation of the synthesis of CDs@g-C3N4 composites via a two-step microwave-assisted in situ heating method using citric acid and urea as precursors in water (MW: microwave). | |
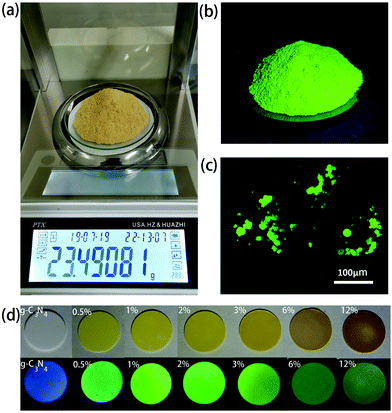 |
| Fig. 2 Photographs of a CDs@g-C3N4 composite (approximately 23.5 g) with 1 wt% CDs taken under daylight (a) and under UV light (b). (c) Fluorescence image of the CDs@g-C3N4 composite with 1 wt% CD loading concentration taken under a fluorescence microscope with blue excitation. (d) Photographs of the pressed tablets of bare g-C3N4 (far left) and a number of CDs@g-C3N4 composites with different weight loadings of CDs, taken under daylight (top row) and UV light (bottom row). | |
The scanning electron microscopy (SEM) image of g-C3N4 provided in Fig. 3a shows that it exhibits a layered morphology. The transmission electron microscopy (TEM) image further reveals that it consists of multiple nanosheets, with a lattice spacing of 0.32 nm as identified by a high resolution TEM (HRTEM) shown in the inset of Fig. 3b, corresponding to the (002) plane of graphitic carbon nitride.30 A TEM image of the CDs@g-C3N4 composite is shown in Fig. 3c. The layered structure related to g-C3N4 can be clearly seen alongside with dispersed dark dots with sizes of 2–8 nm. The HRTEM image shown in the inset of Fig. 3c shows that dots have a lattice spacing of 0.21 nm, corresponding to the (100) crystallographic facet of graphite-like carbon cores,31 which can be attributed to the CDs. XRD patterns of g-C3N4 and CDs@g-C3N4 are very similar (Fig. 3d), both exhibiting a dominating major peak at 27.8 degrees, which can be attributed to the (002) reflection of the graphite phase of g-C3N4.32 The g-C3N4 prepared by microwave heating is similar to that prepared from urea in a tube furnace (Fig. S3, ESI†).
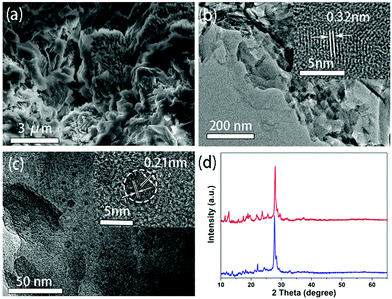 |
| Fig. 3 (a) SEM image of g-C3N4. (b) TEM image of g-C3N4, with a HRTEM image shown in the inset. (c) TEM image of a CDs@g-C3N4 composite with 1 wt% CDs, with a HRTEM image in the inset. (d) XRD patterns of bare g-C3N4 (red line) and the CDs@g-C3N4 composite with 1 wt% CDs (blue line). | |
g-C3N4 synthesized via microwave heating is slightly water soluble. The excitation–emission maps of g-C3N4 in aqueous solution and of the g-C3N4 powder are shown in Fig. 4a and Fig. S4 (ESI†), respectively. Both samples show a single emission band at around 400 nm. From the excitation–emission map of a dilute ethanol solution of the CDs (Fig. 4b), it can be seen that CDs exhibit excitation-dependent PL with two bands at 390 nm and 520 nm, as reported in our previous work.33 The strongest emission is observed at around 520 nm under 410 nm excitation. Fig. 4c shows that CDs@g-C3N4 composites mostly inherit the luminescence from the CDs, namely two bands at 390 nm and 510 nm, while the PL band from g-C3N4 at 400 nm cannot be observed. The effective excitation spectral region for the green emission band of the CDs@g-C3N4 composite is broader than that of the ethanol solution of CDs (Fig. 4d). Considering that the emission of g-C3N4 well overlaps with the PLE spectrum of CDs (Fig. 4d) and the emission band of g-C3N4 is observed in the PLE spectrum of CDs@g-C3N4 (Fig. 4d), it can be inferred that in the CDs@g-C3N4 composite an efficient resonant energy transfer occurs from g-C3N4 to the embedded CDs, and contributes to the enhancement of the green emission of the latter.
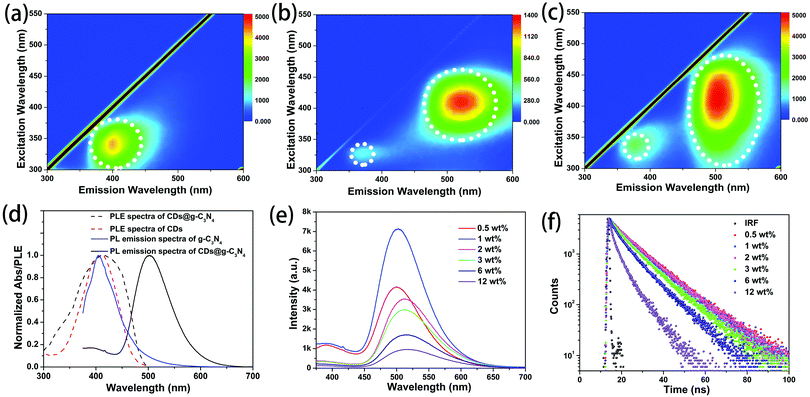 |
| Fig. 4 Excitation–emission maps of (a) g-C3N4 solid powder, (b) an ethanolic solution of CDs and (c) a solid powder of a CDs@g-C3N4 composite with 1 wt% CDs. (d) PLE spectra (monitored at 510 nm) of CDs (red dotted curve) and CDs@g-C3N4 (black dotted curve), and PL emission spectra (excited at 360 nm) of g-C3N4 (blue curve) and CDs@g-C3N4 (black curve). (e) PL spectra of CDs@g-C3N4 composites with different loadings of CDs, all excited at 360 nm. (f) PL decay curves of g-C3N4 and CDs@g-C3N4 powders with different loadings of CDs, monitored at 510 nm under 375 nm excitation. | |
Fig. 4e shows PL spectra of CDs@g-C3N4 composites with different loadings of CDs, all collected under 360 nm excitation. For the loading amounts of CDs lower than 1 wt%, both bands at 390 and 520 nm are present in the PL spectra. The strongest emission was observed for the CDs@g-C3N4 composite with 1 wt% of CDs. Upon further increase of the CD loading, the band at 390 nm vanished, while the band at 520 nm slightly red-shifted and gradually decreased in intensity. Both processes can be ascribed to the increasing re-absorption of the emitted light at higher loading amounts of CDs, while the decreased intensity of the green emission is probably related to the aggregation-induced luminescence quenching of CDs. A clear blue emission from the back of the pressed tablets of bare g-C3N4 can be seen when excited with UV light that passed through the tablets of bare g-C3N4 (Fig. S5, ESI†). Fig. 4f shows PL decay curves of CDs@g-C3N4 composites with different loading amounts of CDs, recorded under 375 nm excitation. At lower CD amounts (up to 1 wt%), the average PL lifetime derived from these curves is 11.6 ns. Upon increasing the CD loading the PL lifetime gradually decreased, which further confirms the assumption that the aggregation-induced luminescence quenching of CDs occurs in the composites. The highest PLQY of the CDs@g-C3N4 composite was measured to be 62% for 1 wt% CD loading (Fig. S6, ESI†).
To reveal the advantages of the microwave-assisted in situ synthesized CDs@g-C3N4 composite, composites of g-C3N4 and CDs were also prepared via physical mixing processing. g-C3N4 and CDs were separately synthesized via the above-mentioned microwave-assisted method, mixed in 30 ml of deionized water followed by stirring for 5 h, and freeze-dried to form composites (denoted CDs/g-C3N4) with CD loading amounts in the range of 0.5–6 wt%. From the TEM image of CDs/g-C3N4 (Fig. S7, ESI†), we can recognize that the CDs tend to form clusters at the edges of g-C3N4. It can be seen from Fig. 5a and Fig. S8, S9 (ESI†) that under UV light excitation the green emission from the CDs@g-C3N4 is much stronger than that of the CDs/g-C3N4 at the same CD loading. The values of the PLQYs and PL intensities of both CDs@g-C3N4 and CDs/g-C3N4 under 410 nm excitation are summarized in Fig. 5b. It can be seen that both the PLQY and PL intensity of CDs@g-C3N4 with 1 wt% CD loading concentration are the highest compared to CDs/g-C3N4, and the PLQY of CDs/g-C3N4 gradually decreases upon increasing the CD loading concentration. Thus, it can be inferred that the microwave-assisted in situ synthesis promotes the successful inclusion of CDs within the g-C3N4 network without evident agglomeration, while the synthesis of composites via physical mixing is not able to provide a homogeneous CD distribution upon increasing the CD loading concentration, which in turn results in CD aggregation-induced luminescence quenching.
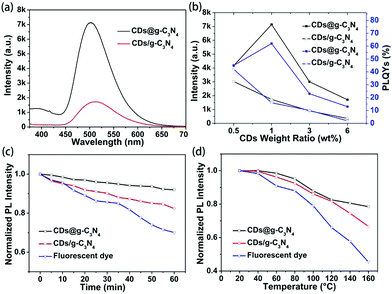 |
| Fig. 5 (a) PL spectra of CDs@g-C3N4 and CDs/g-C3N4 composites with 1 wt% CD loading concentration under 360 nm excitation. (b) Peak PL intensities under 360 nm excitation of CDs@g-C3N4 and CDs/g-C3N4 with different CD loading concentrations (black curves) and PLQYs of CDs@g-C3N4 and CDs/g-C3N4 composites with different CD loading concentrations under 410 nm excitation (blue curves). Evolution of the PL intensities of CDs@g-C3N4, CDs/g-C3N4 and organic dye (fluorescein sodium): (c) under UV light exposure (1.6 W cm−1) and (d) upon heating to 160 °C. | |
Previous reports pointed out the fact that CDs exhibit much better photostability than fluorescent organic dyes.18,34,35 We conducted a study of the photo- and thermal stability of the CDs@g-C3N4 composite, in terms of preserving its emission intensity. As shown in Fig. 5c and d, the PL intensity of the reference organic dye (fluorescein sodium) decreased to less than 70% and 50% of their initial PL intensity after continuous UV irradiation (1.6 W cm−1) for one hour and heating up to 160 °C, respectively, while the PL intensity of the CDs/g-C3N4 composite retained 85% and 65% of their initial PL intensity values. In contrast, the CDs@g-C3N4 composite maintained its emission stability, retaining 92% and 80% of the initial PL intensity after the same treatment, due to the beneficial protection of the homogeneously embedded CDs in the g-C3N4 matrix formed in the second-step of the microwave heating.
A CDs@g-C3N4 composite with 1 wt% CDs, as the one with the most favorable light-emitting characteristics, was selected for WLED fabrication. InGaN LED chips (1 W) were covered with this phosphor dispersed in epoxy silicone resin (mass ratio 1
:
2), followed by heating in an oven at 80 °C for 1 h. A device which emitted cold white light with CIE color coordinates of (0.29, 0.33) and a color temperature of 7557 K was realized (Fig. 6), with a power efficiency of 42 lm W−1, which favorably compares with the performance of other CD-based downconversion WLEDs reported in the literature (Table S1, ESI†).
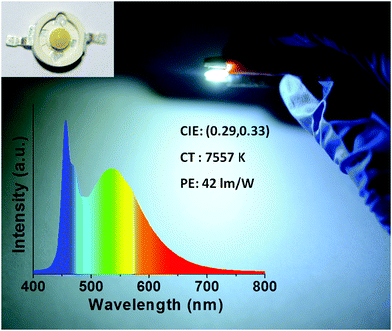 |
| Fig. 6 Photograph of a CD-based working LED prototype utilizing a CDs@g-C3N4 composite with 1 wt% CDs as a phosphor, and its emission spectra with the related characteristics. CIE: CIE color coordinates, CT: color temperature, PE: power efficiency. | |
Conclusions
CDs@g-C3N4 composite phosphors were conveniently fabricated on a large scale using a two-step microwave-assisted heating method from the cheap and environmentally friendly precursors citric acid and urea. CDs were in situ homogeneously embedded and bonded within the g-C3N4 network to prevent CD aggregation-induced luminescence quenching. Compared to a physical mixture of CDs and g-C3N4, composite CDs@g-C3N4 phosphors exhibited higher PLQYs with better photo- and thermal stability. The CDs@g-C3N4 composite with an optimal loading amount of CDs (1 wt%) emitted bright green light with a PLQY of 62% under 410 nm excitation, and retained 92% and 80% of its initial PL intensity after continuous UV irradiation (1.6 W cm−2) for one hour and heating up to 160 °C, respectively. Using this CDs@g-C3N4 composite as the color conversion layer, a WLED emitting cold white light with CIE color coordinates of (0.29, 0.33) and a color temperature of 7557 K was fabricated, with a high power efficiency of 42 lm W−1. The results of this work will further promote the development of environment-friendly CD-based phosphors for lighting applications.
Materials and methods
Citric acid (99.5%) was purchased from Aladdin. Urea (99%) was purchased from Macklin. All chemicals were used as received without further purification. The deionized water used in all the experiments was purified using a Millipore system.
Synthesis of CDs
Green-emissive CDs were synthesized using a microwave-assisted method, from citric acid as the carbon source and urea as the nitrogen source according to our previous work.32 6 g urea, 3 g citric acid and 20 ml of deionized water were mixed in a 100 ml beaker to form a colorless transparent solution. The beaker was transferred to a domestic 750 W microwave oven, and heated for 3–4 min. Upon heating, the water began to boil and gradually evaporated, resulting in the formation of a brownish solid.
Preparation of g-C3N4
10 g urea was dissolved in 20 ml of deionized water, followed by heating in a 750 W microwave oven for 3–4 min. Upon heating, the water gradually evaporated, resulting in the formation of a g-C3N4 powder, which was ground and used for further measurements.
Preparation of CDs@g-C3N4 composites
To prepare composite materials with different loading amounts of CDs, citric acid (9 mg, 18 mg, 36 mg, 54 mg, 108 mg, 216 mg) and urea (18 mg, 36 mg, 72 mg, 108 mg, 216 mg, 432 mg) were added into 20 ml of deionized water, and the mixtures were stirred for a period of time until both precursors were completely dissolved. Each solution was transferred to a 750 W microwave oven and heated for 3–4 min. Upon heating, the water began to boil and gradually evaporated, resulting in the formation of a brownish solid. Subsequently, addition of 20 ml of 0.5 g ml−1 urea to the solid made CDs fully soluble. This solution was placed into a 750 W microwave oven again and heated for 3–4 min until the water completely evaporated, which resulted in the formation of a CDs@g-C3N4 composite solid. The solid was ground and used for further measurements.
Preparation of CDs/g-C3N4 composites by means of physical mixing
Separately synthesized CDs (5 mg, 10 mg, 30 mg, 60 mg) were mixed with g-C3N4 (1 g) under continuous stirring in deionized water for 2 h, and then placed into a lyophilizer for vacuum freezing. The resulting powders were ground and used for further measurements.
Fabrication of WLEDs based on CDs@g-C3N4 phosphors
InGaN LED chips (emission peak at 450 nm, operating under a voltage of 3.0 V) were purchased from Shenzhen Xinhongxian Optoelectronics Technology Co., Ltd. For the preparation of the color conversion layer, the CDs@g-C3N4 composites were mixed with the epoxy silicone resin precursors with a mass ratio of 1
:
2. The mixtures were deposited on the LED chip followed by heating at 80 °C for 1 h.
Characterization
UV-visible absorption spectra were measured on a Shimadzu UV-3101PC spectrophotometer. PL and PL excitation spectra were measured on a Hitachi F-7000 spectrophotometer. Absolute PLQYs were measured in a calibrated integrating sphere using an FLS920 spectrofluorimeter (Edinburgh Instruments Ltd). PL lifetimes were measured via time-correlated single-photon counting using an FLS920 spectrometer under 405 nm excitation. Fluorescence microscopy images were obtained on a C2+ confocal microscope system (Nikon confocal instruments). X-ray powder diffraction (XRD) data were collected on a Siemens D5005 diffractometer. Transmission electron microscopy (TEM) was performed on an FEI Tecnai-G2-F20 microscope at 200 kV. Scanning electron microscopy (SEM) was performed on a JEOL FESEM 6700F microscope with a primary electron energy of 3 kV. The emission spectra of WLEDs were measured on a Fu Xiang NOVA-EX fiber optic spectrometer.
Conflicts of interest
There are no conflicts of interest to declare.
Acknowledgements
This work was funded by the Science and Technology Development Fund, Macau SAR (0040/2019/A1), the National Natural Science Foundation of China (No. 61975200), the Youth Innovation Promotion Association of CAS (2018252), Jilin Province Science and Technology Research Projects (No. 20170101191JC, 20180101190JC and 20170101042JC), and the Ministry of Science and Higher Education of the Russian Federation (Grant No. 14. Y26.31.0028).
References
- E. F. Schubert and J. K. Kim, Solid-state light sources getting smart, Science, 2005, 308, 1274–1278 CrossRef CAS PubMed.
- S.-H. Lim, Y.-H. Ko, C. Rodriguez, S.-H. Gong and Y.-H. Cho, Electrically driven, phosphor-free, white light-emitting diodes using gallium nitride-based double concentric truncated pyramid structures, Light: Sci. Appl., 2016, 5, e16030 CrossRef CAS PubMed.
- J. B. Essner, C. H. Laber, S. Ravula, L. Polo-Parada and G. A. Baker, Pee-dots: biocompatible fluorescent carbon dots derived from the upcycling of urine, Green Chem., 2016, 18, 243–250 RSC.
- D. Li, D. Han, S. N. Qu, L. Liu, P. T. Jing, D. Zhou, W. Y. Ji, X. Y. Wang, T. F. Zhang and D. Z. Shen, Supra-(carbon nanodots) with a strong visible to near-infrared absorption band and efficient photothermal conversion, Light: Sci. Appl., 2016, 5, e16120 CrossRef CAS PubMed.
- B. Andreiuk, A. Reisch, V. G. Pivovarenko and A. S. Klymchenko, An aluminium-based fluorinated counterion for enhanced encapsulation and emission of dyes in biodegradable polymer nanoparticles, Mater. Chem. Front., 2017, 1, 2309–2316 RSC.
- Y. Huang, Y. L. Liang, Y. F. Rao, D. D. Zhu, J. J. Cao, Z. X. Shen, W. K. Ho and S. C. Lee, Environment-Friendly Carbon Quantum Dots/ZnFe2O4 Photocatalysts: Characterization, Biocompatibility, and Mechanisms for NO Removal, Environ. Sci. Technol., 2017, 51, 2924–2933 CrossRef CAS PubMed.
- L. L. Yu, X. Yue, R. Yang, S. S. Jing and L. B. Qu, A sensitive and low toxicity electrochemical sensor for 2,4-dichlorophenol based on the nanocomposite of carbon dots, hexadecyltrimethyl ammonium bromide and chitosan, Sens. Actuators, B, 2016, 224, 241–247 CrossRef CAS.
- Y. Zhan, B. Shang, M. Chen and L. M. Wu, One-Step Synthesis of Silica-Coated Carbon Dots with Controllable Solid-State Fluorescence for White Light-Emitting Diodes, Small, 2019, 15, 9 Search PubMed.
- X. Bao, Y. Yuan, J. Chen, B. Zhang, D. Li, D. Zhou, P. Jing, G. Xu, Y. Wang, K. Holá, D. Shen, C. Wu, L. Song, C. Liu, R. Zbořil and S. Qu, In vivo theranostics with near-infrared-emitting carbon dots—highly efficient photothermal therapy based on passive targeting after intravenous administration, Light: Sci. Appl., 2018, 7, 91 CrossRef PubMed.
- R. X. Wang, X. F. Wang and Y. M. Sun, One-step synthesis of self-doped carbon dots with highly photoluminescence as multifunctional biosensors for detection of iron ions and pH, Sens. Actuators, B, 2017, 241, 73–79 CrossRef CAS.
- X. Li, M. Rui, J. Song, Z. Shen and H. Zeng, Carbon and Graphene Quantum Dots for Optoelectronic and Energy Devices: A Review, Adv. Funct. Mater., 2015, 25, 4929–4947 CrossRef CAS.
- S. Tao, T. Feng, C. Zheng, S. Zhu and B. Yang, Carbonized Polymer Dots: A Brand New Perspective to Recognize Luminescent Carbon-Based Nanomaterials, J. Phys. Chem. Lett., 2019, 10, 5182–5188 CrossRef CAS PubMed.
- D. Qu, D. Yang, Y. Sun, X. Wang and Z. Sun, White Emissive Carbon Dots Actuated by the H-/J-Aggregates and Forster Resonance Energy Transfer, J. Phys. Chem. Lett., 2019, 10, 3849–3857 CrossRef CAS PubMed.
- Y. Wang, S. Kalytchuk, L. Wang, O. Zhovtiuk, K. Cepe, R. Zboril and A. L. Rogach, Carbon dot hybrids with oligomeric silsesquioxane: solid-state luminophores with high photoluminescence quantum yield and applicability in white light emitting devices, Chem. Commun., 2015, 51, 2950–2953 RSC.
- A. Wang, Y.-L. Hou, F. Kang, F. Lyu, Y. Xiong, W.-C. Chen, C.-S. Lee, Z. Xu, A. L. Rogach, J. Lu and Y. Y. Li, Rare earth-free composites of carbon dots/metal–organic frameworks as white light emitting phosphors, J. Mater. Chem. C, 2019, 7, 2207–2211 RSC.
- C.-L. Shen, J.-H. Zang, Q. Lou, L.-X. Su, Z. Li, Z.-Y. Liu, L. Dong and C.-X. Shan, In-situ embedding of carbon dots in a trisodium citrate crystal matrix for tunable solid-state fluorescence, Carbon, 2018, 136, 359–368 CrossRef CAS.
- M. Sun, S. Qu, Z. Hao, W. Ji, P. Jing, H. Zhang, L. Zhang, J. Zhao and D. Shen, Towards efficient solid-state photoluminescence based on carbon-nanodots and starch composites, Nanoscale, 2014, 6, 13076–13081 RSC.
- Z. Tian, D. Li, E. V. Ushakova, V. G. Maslov, D. Zhou, P. Jing, D. Shen, S. Qu and A. L. Rogach, Multilevel Data Encryption Using Thermal-Treatment Controlled Room Temperature Phosphorescence of Carbon Dot/Polyvinylalcohol Composites, Adv. Sci., 2018, 5, 1800795 CrossRef PubMed.
- D. Zhou, Y. Zhai, S. Qu, D. Li, P. Jing, W. Ji, D. Shen and A. L. Rogach, Electrostatic Assembly Guided Synthesis of Highly Luminescent Carbon-Nanodots@BaSO4 Hybrid Phosphors with Improved Stability, Small, 2017, 13, 1602055 CrossRef PubMed.
- Y. Zhai, D. Zhou, P. Jing, D. Li, H. Zeng and S. Qu, Preparation and application of carbon-nanodot@NaCl composite phosphors with strong green emission, J. Colloid Interface Sci., 2017, 497, 165–171 CrossRef CAS PubMed.
- Z. Tian, X. Zhang, D. Li, D. Zhou, P. Jing, D. Shen, S. Qu, R. Zboril and A. L. Rogach, Full-Color Inorganic Carbon Dot Phosphors for White-Light-Emitting Diodes, Adv. Opt. Mater., 2017, 5, 1700416 CrossRef.
- D. Zhou, D. Li, P. Jing, Y. Zhai, D. Shen, S. Qu and A. L. Rogach, Conquering Aggregation-Induced Solid-State Luminescence Quenching of Carbon Dots through a Carbon Dots-Triggered Silica Gelation Process, Chem. Mater., 2017, 29, 1779–1787 CrossRef CAS.
- E. Liu, D. Li, X. Zhou, G. Zhou, H. Xiao, D. Zhou, P. Tian, R. Guo and S. Qu, Highly Emissive Carbon Dots in Solid State and Their Applications in Light-Emitting Devices and Visible Light Communication, ACS Sustainable Chem. Eng., 2019, 7, 9301–9308 CrossRef CAS.
- D. Qu, J. Liu, X. Miao, M. Han, H. Zhang, Z. Cui, S. Sun, Z. Kang, H. Fan and Z. Zai, Peering into water splitting mechanism of g-C3 N4-carbon dots metal-free photocatalyst, Appl. Catal., B, 2018, 227, 418–424 CrossRef CAS.
- J. Liu, Y. Liu, N. Liu, Y. Han, X. Zhang, H. Huang, Y. Lifshitz, S.-T. Lee, J. Zhong and Z. Kang, Metal-free efficient photocatalyst for stable visible water splitting via a two-electron pathway, Science, 2015, 347, 970–974 CrossRef CAS PubMed.
- W. Liu, S. Xu, S. Guan, R. Liang, M. Wei, D. G. Evans and X. Duan, Confined Synthesis of Carbon Nitride in a Layered Host Matrix with Unprecedented Solid-State Quantum Yield and Stability, Adv. Mater., 2018, 30, 1704376 CrossRef PubMed.
- P. Niu, L. Zhang, G. Liu and H.-M. Cheng, Graphene-Like Carbon Nitride Nanosheets for Improved Photocatalytic Activities, Adv. Funct. Mater., 2012, 22, 4763–4770 CrossRef CAS.
- T. X. Chen, C. C. Chen, Q. Liu, Z. G. Zhang and X. M. Fang, A one-step process for preparing a phenyl-modified g-C3N4 green phosphor with a high quantum yield, RSC Adv., 2017, 7, 51702–51710 RSC.
- S. Qu, X. Liu, X. Guo, M. Chu, L. Zhang and D. Shen, Amplified Spontaneous Green Emission and Lasing Emission From Carbon Nanoparticles, Adv. Funct. Mater., 2014, 24, 2689–2695 CrossRef CAS.
- C. Lu, Y. Yang and X. Chen, Ultra-Thin Conductive Graphitic Carbon Nitride Assembly through van der Waals Epitaxy toward High-Energy-Density Flexible Supercapacitors, Nano Lett., 2019, 19, 4103–4111 CrossRef CAS PubMed.
- Z. Wang, Y. Zhao, K. Tait, X. Liao, D. Schiferl, C. Zha, R. T. Downs, J. Qian, Y. Zhu and T. Shen, A quenchable superhard carbon phase synthesized by cold compression of carbon nanotubes, Proc. Natl. Acad. Sci. U. S. A., 2004, 101, 13699–13702 CrossRef CAS PubMed.
- A. Shahzeydi, M. Ghiaci, H. Farrokhpour, A. Shahvar, M. Sun and M. Saraji, Facile and green synthesis of copper nanoparticles loaded on the amorphous carbon nitride for the oxidation of cyclohexane, Chem. Eng. J., 2019, 370, 1310–1321 CrossRef CAS.
- P. T. Jing, D. Han, D. Li, D. Zhou, L. G. Zhang, H. Zhang, D. Z. Shen and S. N. Qu, Origin of Anisotropic Photoluminescence in Heteroatom-Doped Carbon Nanodots, Adv. Opt. Mater., 2017, 5, 7 Search PubMed.
- Y. Zhai, Y. Wang, D. Li, D. Zhou, P. Jing, D. Shen and S. Qu, Red carbon dots-based phosphors for white light-emitting diodes with color rendering index of 92, J. Colloid Interface Sci., 2018, 528, 281–288 CrossRef CAS.
- Y. Xiong, J. Schneider, C. J. Reckmeier, H. Huang, P. Kasak and A. L. Rogach, Carbonization conditions influence the emission characteristics and the stability against photobleaching of nitrogen doped carbon dots, Nanoscale, 2017, 9, 11730–11738 RSC.
Footnote |
† Electronic supplementary information (ESI) available. See DOI: 10.1039/c9qm00659a |
|
This journal is © the Partner Organisations 2020 |
Click here to see how this site uses Cookies. View our privacy policy here.