DOI:
10.1039/D4TC01344A
(Paper)
J. Mater. Chem. C, 2024,
12, 11426-11432
Modulating organic functional groups in stimuli-responsive luminescent antimony chlorides†
Received
2nd April 2024
, Accepted 16th June 2024
First published on 2nd July 2024
Abstract
Stimuli-responsive inorganic–organic hybrid metal halides (IOMHs) have shown great potential in sensing, information encryption and anti-counterfeiting, etc. However, the stimuli-responsive behavior based on the regulation of the functional groups in organic species is still blank in IOMHs. Herein, three zero-dimensional (0-D) antimony-based IOMHs with different functional groups in the organic cations are reported, namely [AOEMIm]3SbCl6 (1, AOEMIm = 1-acetoxyethyl-3 methylimidazolium), [HOOCMMIm]3SbCl6·3(OOCMMIm) (2, HOOCMMIm = 1-carboxymethyl-3-methylimidazolium) and [HOOCMMIm]3SbCl6 (3). Photophysical characterizations show that 1, 2 and 3 exhibit typical self-trapped exciton triplet broadband emission, peaking at 610, 510 and 525 nm under excitation of 365 nm, 375 nm and 345 nm, respectively. Under the H2O stimulation, the organic groups in the crystal structures undergo a mutual transformation, that is, from acetoxyethyl or carboxyl to a mixture of carboxyl and deprotonated carboxyl. Accordingly, the organic group switching is accompanied by a solid–solid transformation from 1 or 2 to 3 crystals. The intrinsic mechanism for the phase transition is expounded as well. Due to the obvious luminescence change from yellow-green emission (3) to green emission (2), 3 is further applied in the detection of trace water in organic solvents. The water detection limit in tetrahydrofuran (THF) is as low as 0.3% v/v. This study provides a new avenue for the construction of stimuli-responsive IOMHs.
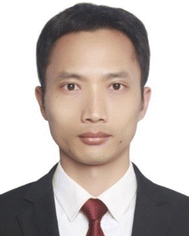
Ke-Zhao Du
| Ke-Zhao Du received a PhD degree in 2013 from Fujian Institute of Research on the Structure of Matter, Chinese Academy of Sciences, China under the supervision of Prof. Xiaoying Huang. After that, he worked as a postdoctoral researcher in Nanyang Technological University, Singapore and Duke University, USA. In 2018, he joined Fujian Normal University, China as a professor. His research interest focuses on the crystal structure–property relationship study of metal halides and their applications for energy transformation. |
Introduction
Inorganic–organic hybrid metal halides (IOMHs) have attracted much attention in the fields of lighting, imaging, anti-counterfeiting, ferroelectrics, and solar cells due to their simple synthesis methods, excellent luminescence properties, and variable structures.1–13 Typical IOMHs have the chemical formula AmBXn (A = organic cation, B = metal ion, X = halide anion).14 The composition and ionic structure characteristics of IOMHs dictate relatively weak interactions between cations and anions, leading to the formation of a soft lattice and chemical reactivity.15 Therefore, IOMHs are susceptible to structural transformations after regulating external factors such as temperature,16–20 humidity21–25 and pressure.26 The structural transformation can lead to strong fluorescence changes, such as fluorescence enhancement (on), diminution (off) or luminescence colour shift,22,23,27,28 which makes IOMHs an ideal class of stimuli-responsive luminescent materials.
However, most of the previously reported stimuli-responsive structural changes of IOMHs occur in inorganic components or lattices. That is, the external stimulation significantly changes the bond length and bond angle of the inorganic components,29,30 the coordination number of metal ions, the coordination/insertion of the guest molecules, or the dimensional change of BXnm−.31–42 For example, Xiao et al.43 reported that the bond length and bond angle of Sn2+-IOMHs C4N2H14SnBr4 change significantly with increasing pressure, resulting in a larger dipole moment and higher structural and excited state distortion. Peng et al. found that the hydrogen bond strength in [EtPPh3]2[SbCl5] can be influenced by diverse organic functional groups upon exposure to different organic gases. Consequently, the emission of the [SbCl5]2− unit is changed depending on their different distortion, resulting in solvatochromic and thermochromic photoluminescence (PL).4 Zang et al. reported the conversion of a green-emitting complex, C6N2H16MnBr4, into a non-emissive hydrated phase, C6N2H16MnBr4(H2O)2, by absorbing coordinated water molecules. The reversible crystal phase transition can switch the PL as well.33 As we know, most phase transition cases are involved with the isomerisation of organic cations in IOMHs.25,44,45 For example, Zhang et al. reported that chiral β-[Bmmim]2SbCl5 transforms into achiral α-[Bmmim]2SbCl5 by crystalline phase recognition, resulting in a domino phase transition accompanied by photoluminescence switching.46 However, the stimuli-responsive organic species reconstruction is still blank in OIMHs, which would broaden the research area in stimuli-responsive IOMHs.
Keeping this in mind, we selected organic cations with special functional groups to synthesize three IOMHs to study the organic reconstruction induced switching on structure and PL, namely [AOEMIm]3SbCl6 (1, AOEMIm = 1-acetoxyethyl-3-methylimidazolium) [HOOCMMIm]3SbCl6·3(OOCMMIm) (2,47 HOOCMMIm = 1-carboxymethyl-3-methylimidazolium), and [HOOCMMIm]3SbCl6 (3). Benefitting from the potential post-synthetic modification behaviour of cationic functional groups, external stimuli can be used to achieve the reconstruction of organic moieties, resulting in a series of controllable and stepwise structural transformations. Both 1 and 3 can respond to humidity stimulation and eventually change to 2, while 2 is reversibly converted to 3 under HCl and SbCl3 atmospheres. The apparent luminescence switching during the structural transformation makes 2 and 3 ideal candidates for fluorescent humidity sensors. Study shows that 3 can detect trace water in organic solvents with a detection limit as low as 0.3% v/v.
Results and discussion
Synthesis and crystal structural description
Single crystals of 1 were synthesized by an ionothermal reaction method. Using the same raw materials, 2 and 3 were synthesized by different reactant ratios and solvents (see ESI† for detailed information). The discrete anionic units [SbCl6]3− of the three compounds were surrounded by different cations (and neutral molecules in 2) to form a 0-D structure. The three compounds form a three-dimensional supramolecular framework based on hydrogen bonding forces (Fig. 1d–f). Detailed crystallographic and supramolecular interaction data for 1, 2, and 3 are listed in Tables S1–S3 (ESI†).
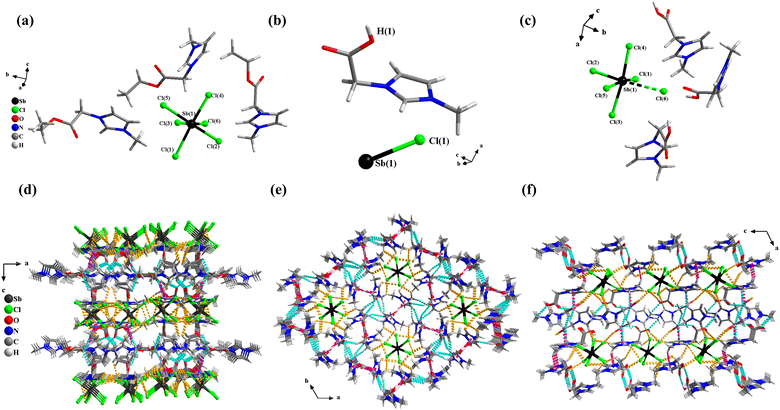 |
| Fig. 1 (a)–(c) Asymmetric units of crystals 1, 2 and 3, respectively. H (1) is half-occupied in 2. (d) The packing diagram of crystal 1 along the b-axis. (e) The packing diagram of crystal 2 along the c-axis. (f) The packing diagram of crystal 3 along the b-axis. The purple dashed lines represent cation⋯π interactions and π⋯ π interactions, the gold dashed lines represent C–H⋯Cl bonds, and the cyan dashed lines represent C–O⋯H bonds. | |
1 crystallizes in an orthorhombic crystal system with the space group of Pbca and the cell parameters are a = 20.4908(3) Å, b = 16.2409(3) Å, c = 21.6024(4) Å, V = 7189.0(2) Å3, Z = 8 (Table S1, ESI†). The crystallographic asymmetric unit of 1 contains three [AOEMIm]+ cations and one isolated [SbCl6]3− anion (Fig. 1a). It could be seen that the bond length of Sb–Cl in [SbCl6]3− ranges from 2.549(3) to 2.809(4) Å, forming a distorted octahedral geometry (Table S2, ESI†). In addition, there are abundant weak interactions in the structure, such as C–H⋯Cl, C–O⋯H, and C–H⋯π (Fig. S4a–c, ESI†). The anionic unit [SbCl6]3− formed a two-dimensional anionic layered structure through hydrogen bonding C–H⋯Cl (Fig. S4d, ESI†). The two-dimensional anionic layer formed a three-dimensional supramolecular framework by connecting the cationic layer through C–O⋯H and C–H⋯π (Fig. 1d, and Fig. S4e, ESI†).
2 crystallizes in a trigonal crystal system with the space group of R
and the cell parameters are a = 21.8818(4) Å, b = 21.8818(4) Å, c = 8.6170(3) Å, V = 3573.2(2) Å3, Z = 3 (Table S1, ESI†). Its crystallographic asymmetric unit contains one half-deprotonated [H0.5OOCMMIm]+0.5 and one-sixth of a [SbCl6]3− unit (Fig. 1b). That is, due to disorder, the organic moiety is composed of 0.5 [HOOCMMIm]+ and 0.5 neutral [OOCMMIm] (Fig. S5b, ESI†). The anion is close to that of an ortho-octahedron, and the lengths of the six Sb–Cl bonds are similar so that of the anionic unit located on a threefold rotation (Fig. S5a, ESI†). Each anionic unit [SbCl6]3− is surrounded by six imidazole rings with C–H⋯Cl h-bond interactions, forming a one-dimensional tubular structure (Fig. S5e, ESI†). Then the tubular structures are further interacted with each other via C–O⋯H and π⋯π interactions to form an overall three-dimensional supramolecular framework (Fig. 1e, and Fig. S5c, d, f, ESI†).
3 crystallizes in a monoclinic system with the space group of P21/c and the cell parameters are a = 20.7233(11) Å, b = 8.7393(3) Å, c = 17.6064(9) Å, β = 114.637(6) °, V = 2898.4(3) Å3, Z = 4 (Table S1, ESI†). The crystallographic asymmetric unit of 3 contains three [HOOCMMIm]+ cations and one isolated [SbCl6]3− anion (Fig. 1c). Notably, the Sb–Cl(6) bond with a bond length of 3.3245(7) Å, which is longer than the other Sb–Cl bonds, is seen as a secondary bond and results in an irregular octahedral anion (Table S2, ESI†). The anionic units form a two-dimensional anionic layer structure through hydrogen bonding C–H⋯Cl, and then the C–H⋯π and C–O⋯H interactions between the cations connected the two-dimensional anionic layer to form a three-dimensional supramolecular framework (Fig. 1f, and Fig. S6, ESI†).
Elemental analysis (EA) and PXRD results indicate that 1, 2, and 3 are pure phases (Fig. S7, ESI†). In addition, the 1H NMR spectra of 2 and 3 show a characteristic peak of COOH at the chemical shift of 12.50 ppm position. However, the COOH peak in 3 is much stronger than that in 2 (Fig. S8, ESI†), which is in good agreement with their structural components. Thermogravimetric (TG) analysis showed that 1, 2 and 3 are stable below 250 °C, 240 °C and 160 °C, respectively. This indicates that the title compounds have good thermal stability, which lays a foundation for the subsequent study of fluorescence properties (Fig. S9, ESI†).
Luminescence properties
The photoluminescence (PL) spectra, photoluminescence excitation (PLE) spectra, and time-resolved PL spectra have been performed to investigate the photoluminescent properties of compounds 1, 2 and 3. The PL spectrum of 1 shows a main peak at 610 nm, while the main PLE peak is located at 365 nm with a shoulder peak at 292 nm. Similarly, 2 has a main PL peak at 510 nm with PLE peaks at 286 nm and 375 nm, while 3 has a main PL peak at 525 nm with PLE peaks at 280 nm and 345 nm (Fig. 2a–c). The two peaks of their PLE spectra should correspond to the 1S0 → 1P1 and 1S0 → 3P1 electronic transitions of Sb3+ ions, respectively.48 The 1S0 → 1P1 electronic transition was allowed, while the 1S0 → 3P1 was partially allowed by the spin–orbit coupling.3,491, 2 and 3 exhibit broadband peaks at 610, 510 and 525 nm under the excitation of 365 nm, 375 nm and 345 nm, respectively. The corresponding PL lifetimes for 1, 2 and 3 are 3.168 μs, 2.093 μs and 3.106 μs (Fig. S10, ESI†), respectively, which suggests that their emissions might arise from the exciton relaxation of 3Pn → 1S0.5 The PLQYs for 1, 2 and 3 are 61.25%, 84.7% and 38.75%, respectively. The Commission Internationale del'Eclairage (CIE) chromaticity coordinates for 1, 2 and 3 are (0.510, 0.460), (0.200, 0.435) and (0.293, 0.542), respectively (Fig. 2d).
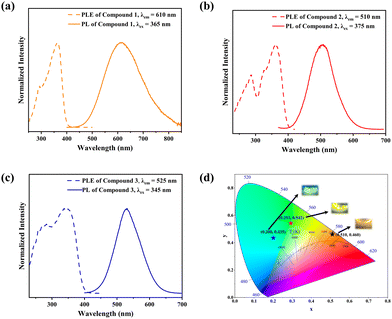 |
| Fig. 2
1 (a), 2 (b) and 3 (c) PLE and PL spectra at room temperature. The solid lines are the emission spectra at different excitation wavelengths. The dashed lines are the excitation spectra at different emission wavelengths. (d) CIE chromaticity coordinates corresponding to 1, 2 and 3. Inset: Photographs of the compounds under UV light. | |
The varied PLQYs of 1, 2 and 3 might have a correlation with the distortion of [SbCl6]3− in their crystal structure.50,51 The lower the degree of ground state structural distortion, the stronger the symmetry of its structure, and the smaller the non-radiative excitation energy loss during the excited state recombination, thus increasing the possibility of stronger luminescence.32 The distortions based on the Sb–Cl bond length and Cl–Sb–Cl angle variance are defined as follows.52
where
θn denotes the bond angle of each Cl–Sb–Cl,
dn is the average of the Sb–Cl bond distance, and
dn denotes individual Sb–Cl bond length.
σ2 and Δ
d represent the [SbCl
6]
3− distortion level. The
σ2 values of [SbCl
6]
3− in
1–3 were calculated as 6.50, 3.06 and 16.94, while the Δ
d ones are 13.07, 0 and 117.64, respectively (
Table 1). The [SbCl
6]
3− distortion level in
1–3 is inversely proportional to their PLQYs.
Table 1 Summary of the photophysical properties, σ2 and Δd for the title compounds at 300 K
Compound |
λ
ex (nm) |
λ
em (nm) |
FWHM (nm) |
Φ (%)a |
τ
av (μs)b |
σ
2
|
Δd (×10−4) |
Ref. |
Φ is the PLQY.
τav is the average PL lifetime.
|
[AOEMIm]3SbCl6 (1) |
292, 365 |
610 |
170 |
61.25 |
3.168 |
6.50 |
13.07 |
This work |
[HOOCMMIm]3SbCl6·3(OOCMMIm) (2) |
286, 375 |
510 |
89 |
84.7 |
2.093 |
3.06 |
0 |
This work |
[HOOCMMIm]3SbCl6 (3) |
280, 345 |
525 |
90 |
38.75 |
3.106 |
16.94 |
117.61 |
This work |
Structural transformation
It was noteworthy that the orange-emitting 1 and the yellow-green-emitting 3 can gradually convert into the green-emitting 2 when exposed to air, which is confirmed by the PXRD results (Fig. 3a and c). The transition time is determined by the relative humidity of the environment. The higher humidity will induce faster structural transformation from 3 to 2 (Fig. S11, ESI†). The ester group portion of [AOEMIm]+ in 1 can be hydrolysed in air, resulting in irreversible conversion from 1 to 2 (eqn (1)). The conversion from 3 to 2 might be triggered by the H2O-induced deprotonation from the carboxylate group to H2O (Fig. 3b). To verify our speculation, we immersed 3 in acetonitrile and tested the pH before and after the structure transformation from 3 to 2 (Fig. S12, ESI†). The pH value changes from 5 to 2 (Fig. S13, ESI†), confirming the proton transfer in the transformation. During the transformation from 3 to 2, SbCl3 as a by-product is found in PXRD as shown in Fig. S14, ESI,† confirming the release of SbCl3 during the transformation process (eqn (2)). Furthermore, based on eqn (3), 3 can be yielded by fumigating 2 with a mixture of SbCl3 and HCl, which is confirmed by our experiments as shown in Fig. 3a, and d and Fig. S15 (ESI†). The structure transformations in this work are given as eqn (1) to eqn (3): | 2[AOEMIm]3SbCl6 (1) + 9H2O → [HOOCMMIm]3SbCl6·3(OOCMMIm) (2) + 3H3O+ + SbCl3 + 3Cl− + 6CH3CH2OH | (1) |
| 2[HOOCMMIm]3SbCl6 (3) + 3H2O → [HOOCMMIm]3SbCl6·3(OOCMMIm) (2) + 3H3O+ + SbCl3 + 3Cl− | (2) |
| [HOOCMMIm]3SbCl6·3(OOCMMIm) (2) + SbCl3 + 3HCl → 2[HOOCMMIm]3SbCl6 (3) | (3) |
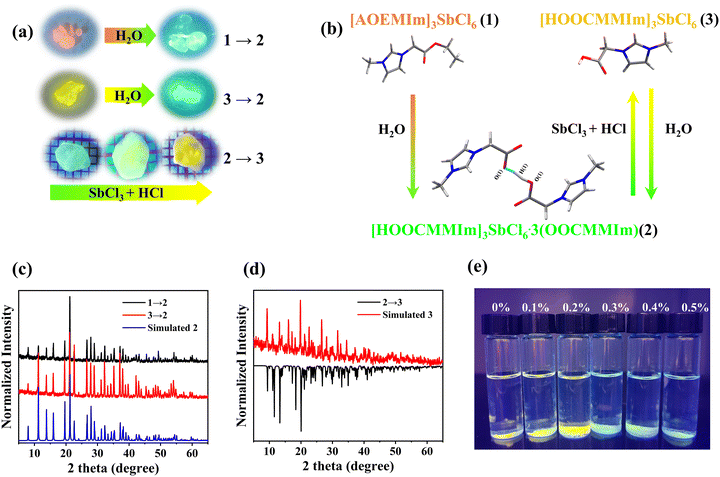 |
| Fig. 3 (a) Plot of the transformations of the title compounds over time under UV irradiation. (b) Simple schematic of three-mode conversion. (c) Comparison of the experimental PXRD of 1 and 3 converted to 2 with the SCXRD simulation data of 2, respectively. (d) Comparison of the experimental PXRD of 2 converted to 3 with the SCXRD simulated data of 3. (e) Dehydration of 3 in tetrahydrofuran solvent containing different amounts of water (0–0.5% v/v). | |
As far as we know, this is the first report about regulating the structure transition in IOMHs based on post-synthetic modification of the organic component.
Luminescent water-sensing
Various types of sensors have been used for water detection,19,23,53,54 including fibre optic sensors, fluorescent sensors, capacitive sensors, and resistive sensors. The water triggers structural transformation and accordingly luminescence colour and/or intensity changes allow IOMHs to be used as fluorescence sensors.22,23,27,28 Therefore, it can be applied to the detection of trace water in organic solvents,55–57 which has the advantages of low cost, high sensitivity and so on. The powder of 3 was immersed in THF with different water contents (0–0.5% v/v). When the water content was above 0.3% v/v, the yellow-green emission of compound 3 turned into the green emission of compound 2 within 10 min. The detection limit of [HOOCMMIm]3SbCl6 was about 0.3% v/v (Fig. 3e), which was much lower than that of (PPZ)2SbCl7·5H2O (1.5% v/v).58
Conclusion
In summary, three 0-D antimony (III) chloride-based IOHMs with photoluminescence from Sb(III) were synthesized by ionothermal and solventothermal methods. The single-crystal structures of these compounds are analysed in detail. The PLQYs of 1, 2, and 3 are 61.25%, 84.7%, and 38.75%, respectively, in correlation with the distortion of [SbCl6]3− in their crystal structure. Under H2O treatment, the ester group portion of [AOEMIm]+ in 1 will be hydrolysed, while the carboxyl in 3 will be deprotonated. As a result, H2O stimulated organic cation reconstruction can lead to the gradual and controllable transformation from 1 or 3 to 2. In addition, stimulated by the vapour from HCl and SbCl3, 2 is reversibly converted to 3. The mechanism for the structural transformations among the title compounds is verified by experiments including PXRD and pH test. The H2O triggered PL switching from 3 to 2 is adopted to detect the trace water in organic solvents. The water detection limit in THF is as low as 0.3% v/v, better than the previous homogeneous materials. This study opens a new avenue for synthesizing stimuli-responsive IOMHs through organic group reconstruction.
Experimental
Materials and methods
1-Acetoxyethyl-3 methylimidazolium chloride ([AOEMIm]Cl, 99%) and 1-carboxymethyl-3-methylimidazolium chloride ([HOOCMMIm]Cl, 99%) were purchased from Lanzhou Greenchem ILs (Lanzhou China); antimony (III) chloride (SbCl3, 99%) was purchased from Adamas Reagent Co., Ltd. Methanol (CH3OH, AR) was purchased from Sinopharm Chemical Reagent Co., Ltd (China). Tetrahydrofuran (THF, water ≤ 30 ppm (by K. F.), 99.9%, stabilized with BHT, SafeDry, Safeseal) was purchased from Adamas Reagent Co., Ltd. All reagents were used without further purification.
Characterization
Powder X-ray diffraction (PXRD) patterns were measured on a Rigaku Miniflex-II diffractometer by utilizing CuKα radiation (λ = 1.54178 Å) in the angular range of 2θ = 5–65°.59 Thermogravimetric (TG) analyses were performed on a NETZSCH STA 449F3 unit at a heating rate of 10 K min−1 under a N2 atmosphere. Photoluminescence excitation (PLE) and photoluminescence (PL) spectra and time-resolved PL spectra and quantum yields of compound 1–3 were recorded on an Edinburgh FLS1000 UV/V/NIR fluorescence spectrometer. Elemental analysis (EA) was conducted on a German Elementary Vario MICRO instrument. Solid-state NMR spectra were obtained on a Bruker AVANCE III 500 M spectrometer.
Syntheses [AOEMIm]3SbCl6 (1)
The pure phase 1 was formed by the reaction of SbCl3 and [AOEMIm]Cl with a molecular ratio of 1
:
3. The mixture was heated and dissolved to form a clear melt, which was then cooled to room temperature (RT) for 1 week to form colourless, transparent crystals. The yield was calculated to be nearly 52.7% based on the Sb atom. EA: calcd (%): C, 34.23%; H, 4.67%; N, 9.98%; found: C, 34.97%; H, 4.77%; N, 11.09%.
[HOOCMMIm]3SbCl6·3(OOCMMIm) (2)
The pure phase 2 was formed by the reaction of SbCl3 and [HOOCMMIm]Cl in a molecular ratio of 1
:
6 and using 1 mL of methanol as a solvent. After reacting at RT for 10 hours, crystals of 2 would be formed. The yield was calculated to be nearly 63.0% based on the Sb atom. EA: calcd (%): C, 36.69%; H, 4.36%; N, 14.26%; found: C, 36.23%; H, 4.52%; N, 13.94%. 1H NMR (500 MHz, δ): 3.81(s, 5H), 5.13(s, 5H), 6.79(s, 3H), 7.52(s, 4H), 8.93(s, 2H).
[HOOCMMIm]3SbCl6 (3)
3 was synthesized in a similar way to 2 with the mole ratio changed to 1
:
3. The mixture was heated to 80 °C for 10 hours so that it was clearly molten, and then crystals of 3 were generated after cooling the molten mixture to room temperature (RT) for 1 day. The yield was calculated to be nearly 96.0% based on the Sb atom. EA: calcd (%): C, 28.52%; H, 3.59%; N, 11.09%; found: C, 28.70%; H, 3.59%; N, 11.26%. 1H NMR (500 MHz, δ): 3.81 (s, 1H), 5.13 (s, 5H), 6.20 (s, 3H), 7.47 (s, 4H), 8.89 (s, 2H), 12.5 (s, 6H).
Single crystal X-ray diffraction (SCXRD)
A suitable single crystal was selected under an optical microscope for SCXRD measurement. Intensity data for single crystals 1 and 3 were collected at 100 K using graphite monochromatic MoKα radiation (λ = 0.71073 Å) on a Supernova CCD diffractometer. Single-crystal intensity data for 2 were collected at 100 K using graphite monochromatic CuKα radiation (λ = 1.54178 Å) on a Supernova CCD diffractometer. The structure was solved by direct methods and refined by full-matrix least-squares on F2 using the SHELX-2018 program package.60 The hydrogen atoms connected to all C atoms were located at geometrically calculated positions. The non-hydrogen atoms were refined anisotropically.
Data availability
CCDC No. 2341218, 2344297, 2341219 contain the supplementary crystallographic data for this paper. The data can be obtained free of charge from The Cambridge Crystallographic Data Centre viahttps://www.ccdc.cam.ac.uk/data_request/cif.
Author contributions
Z. P. W., Y. L. and D. D. H. conceived the project. Y. L. and D. D. H. designed and performed most of the experiments. Y. L. and D. D. H. carried out PL, PLE, PL attenuation, TG, UV, XRD, and SCXRD experiments. H. W. L. and Z. Z. Z. helped with the experiments. Y. L. and D. D. H. wrote the manuscript. Z. P. W., X. Y. H. and K. Z. D. revised the manuscript. All authors discussed the results and commented on the manuscript; Z. P. W., X. Y. H. and K. Z. D. supervised the project.
Conflicts of interest
There are no conflicts to declare.
Acknowledgements
This work was supported by the National Natural Science Foundation of China (No. 92261115, 22205236 and 22373014) and the Natural Science Foundation of Fujian Province (No. 2022J05089).
Notes and references
- T.-H. Zhuang, Y.-M. Lin, H.-W. Lin, Y.-L. Guo, Z.-W. Li, K.-Z. Du, Z.-P. Wang and X.-Y. Huang, Molecules, 2023, 28, 2380 CrossRef CAS PubMed.
- Y. Jing, Y. Liu, M. Li and Z. Xia, Adv. Opt. Mater., 2021, 9, 2002213 CrossRef CAS.
- Y.-C. Peng, S.-H. Zhou, J.-C. Jin, T.-H. Zhuang, L.-K. Gong, H.-W. Lin, Z.-P. Wang, K.-Z. Du and X.-Y. Huang, J. Phys. Chem. C, 2022, 126, 17381–17389 CrossRef CAS.
- Y.-C. Peng, J.-C. Jin, Q. Gu, Y. Dong, Z.-Z. Zhang, T.-H. Zhuang, L.-K. Gong, W. Ma, Z.-P. Wang, K.-Z. Du and X.-Y. Huang, Inorg. Chem., 2021, 60, 17837–17845 CrossRef CAS PubMed.
- Y.-C. Peng, H.-W. Lin, S.-H. Zhou, J.-C. Jin, T.-H. Zhuang, A. Ablez, Z.-P. Wang, K.-Z. Du and X.-Y. Huang, Molecules, 2023, 28, 1978 CrossRef CAS PubMed.
- K. M. McCall, V. Morad, B. M. Benin and M. V. Kovalenko, ACS Mater. Lett., 2020, 2, 1218–1232 CrossRef CAS PubMed.
- L. Zhou, J. F. Liao and D. B. Kuang, Adv. Opt. Mater., 2021, 9, 2100544 CrossRef CAS.
- J.-C. Jin, N.-N. Shen, Y.-P. Lin, L.-K. Gong, H.-Y. Tong, K.-Z. Du and X.-Y. Huang, Inorg. Chem., 2020, 59, 13465–13472 CrossRef CAS PubMed.
- J.-Q. Zhao, Y.-Y. Ma, X.-J. Zhao, Y.-J. Gao, Z.-Y. Xu, P.-C. Xiao, C.-Y. Yue and X.-W. Lei, Research, 2023, 6, 0094 CrossRef CAS PubMed.
- S. Yu, H. Peng, Q. Wei, T. Li, W. Huang, X. He, Z. Du, J. Zhao and B. Zou, Mater. Horiz., 2024, 11, 2230–2241 RSC.
- C. Sun, Y. H. Guo, S. S. Han, J. Z. Li, K. Jiang, L. F. Dong, Q. L. Liu, C. Y. Yue and X. W. Lei, Angew. Chem., Int. Ed., 2020, 59, 16465–16469 CrossRef CAS PubMed.
- C. Sun, J. Zang, Y. Liu, Q. Zhong, X. Xing, J. Li, C. Yue and X. Lei, CCS Chem., 2022, 4, 3106–3121 CrossRef CAS.
- J. Q. Zhao, M. F. Han, X. J. Zhao, Y. Y. Ma, C. Q. Jing, H. M. Pan, D. Y. Li, C. Y. Yue and X. W. Lei, Adv. Opt. Mater., 2021, 9, 2100556 CrossRef CAS.
- C. Zhou, L. J. Xu, S. Lee, H. Lin and B. Ma, Adv. Opt. Mater., 2020, 9, 2001766 CrossRef.
- Y. Zhou and N. P. Padture, ACS Energy Lett., 2017, 2, 2166–2176 CrossRef CAS.
- N. X. Wu, C. Chen, S. T. Lin, H. H. Li, P. P. He and H. D. Zheng, Inorg. Nano-Met. Chem., 2023, 53, 428–436 CrossRef CAS.
- N. Leblanc, N. Mercier, M. Allain, O. Toma, P. Auban-Senzier and C. Pasquier, J. Solid State Chem., 2012, 195, 140–148 CrossRef CAS.
- W. C. Zhang, Z. H. Sun, J. Zhang, S. G. Han, C. M. Ji, L. N. Li, M. C. Hong and J. H. Luo, J. Mater. Chem. C, 2017, 5, 9967–9971 RSC.
- D. Y. Li, J. H. Song, Y. Cheng, X. M. Wu, Y. Y. Wang, C. J. Sun, C. Y. Yue and X. W. Lei, Angew. Chem., Int. Ed., 2022, 61, e202206437 CrossRef CAS PubMed.
- J. Q. Zhao, H. S. Shi, L. R. Zeng, H. Ge, Y. H. Hou, X. M. Wu, C. Y. Yue and X. W. Lei, Chem. Eng. J., 2022, 431, 134336 CrossRef CAS.
- Q. Kong, X. Meng, S. Ji, Q. Wang, B. Yang, T. Bai, X. Wang, Z. Wang, R. Zhang, D. Zheng, F. Liu and K.-L. Han, ACS Mater. Lett., 2022, 4, 1734–1741 CrossRef CAS.
- Z. Gong, W. Zheng, P. Huang, X. Cheng, W. Zhang, M. Zhang, S. Han and X. Chen, Nano Today, 2022, 44, 101460 CrossRef CAS.
- W. Gao, M. Leng, Z. Hu, J. Li, D. Li, H. Liu, L. Gao, G. Niu and J. Tang, Dalton Trans., 2020, 49, 5662–5668 RSC.
- Z. Wang and X. Huang, Chem. – Eur. J., 2022, 28, e202200609 CrossRef CAS PubMed.
- D.-Y. Li, J.-H. Song, Z.-Y. Xu, Y.-J. Gao, X. Yin, Y.-H. Hou, L.-J. Feng, C.-Y. Yue, H. Fei and X.-W. Lei, Chem. Mater., 2022, 34, 6985–6995 CrossRef CAS.
- S. Guo, Y. Zhao, K. Bu, Y. Fu, H. Luo, M. Chen, M. P. Hautzinger, Y. Wang, S. Jin, W. Yang and X. Lü, Angew. Chem., Int. Ed., 2020, 59, 17533–17539 CrossRef CAS PubMed.
- G. Song, M. Li, S. Zhang, N. Wang, P. Gong, Z. Xia and Z. Lin, Adv. Funct. Mater., 2020, 30, 2002468 CrossRef CAS.
- L. Zhou, J. F. Liao, Z. G. Huang, J. H. Wei, X. D. Wang, W. G. Li, H. Y. Chen, D. B. Kuang and C. Y. Su, Angew. Chem., Int. Ed., 2019, 58, 5277–5281 CrossRef CAS PubMed.
- O. Toma, M. Allain, F. Meinardi, A. Forni, C. Botta and N. Mercier, Angew. Chem., Int. Ed., 2016, 55, 7998–8002 CrossRef CAS PubMed.
- M.-M. Lun, H.-F. Ni, Z.-X. Zhang, J.-Y. Li, Q.-Q. Jia, Y. Zhang, Y. Zhang and D.-W. Fu, Angew. Chem., Int. Ed., 2023, 63, e202313590 CrossRef PubMed.
- L. Gong, F. Huang, Z. Zhang, Y. Zhong, J. Jin, K.-Z. Du and X. Huang, Chem. Eng. J., 2021, 424, 130544 CrossRef CAS.
- M.-E. Sun, Y. Li, X.-Y. Dong and S.-Q. Zang, Chem. Sci., 2019, 10, 3836–3839 RSC.
- H. L. Liu, H. Y. Ru, M. E. Sun, Z. Y. Wang and S. Q. Zang, Adv. Opt. Mater., 2021, 10, 2101700 CrossRef.
- Y. Tian, H. Peng, Q. L. Wei, Y. X. Chen, J. J. Xia, W. C. Lin, C. Y. Peng, X. F. He and B. S. Zou, Chem. Eng. J., 2023, 458, 141436 CrossRef CAS.
- Z. An, R. M. Liao, H. Q. Yao, Y. Cheng, B. J. Zhu, J. R. Li and H. Y. Ye, Eur. J. Inorg. Chem., 2023, 26, e202200740 CrossRef CAS.
- J. Q. Zhao, Y. Y. Ma, X. J. Zhao, Y. J. Gao, Z. Y. Xu, P. C. Xiao, C. Y. Yue and X. W. Lei, Research, 2023, 6, 0094 CrossRef CAS PubMed.
- X. He, H. Peng, Q. Wei, Z. Zhou, Z. Du, J. Zhao and B. Zou, Aggregate, 2024, 5, e407 CrossRef CAS.
- W. Ma, Q. Qian, S. M. H. Qaid, S. Zhao, D. Liang, W. Cai and Z. Zang, Nano Lett., 2023, 23, 8932–8939 CrossRef CAS PubMed.
- K. J. Liu, K. Liu, S. Q. Hao, A. Hou, J. D. Cao, M. Z. Quan, Y. G. Wang, C. Wolverton, J. Zhao and Q. L. Liu, Adv. Funct. Mater., 2024, 34, 2309296 CrossRef CAS.
- S. H. Xue, C. M. Shi, L. J. Xu and Z. N. Chen, Adv. Opt. Mater., 2024, 2302854 CrossRef CAS.
- Y. R. Xie, J. T. Peng, Q. Y. Qin and B. B. Luo, ACS Appl. Nano Mater., 2024, 7, 1319–1326 CrossRef CAS.
- B. Lian, J. D. Yao, M. Ren, B. S. Zou, B. B. Luo and R. S. Zeng, J. Mater. Chem. C, 2024, 12, 2944–2952 RSC.
- Y. Shi, Z. Ma, D. Zhao, Y. Chen, Y. Cao, K. Wang, G. Xiao and B. Zou, J. Am. Chem. Soc., 2019, 141, 6504–6508 CrossRef CAS PubMed.
- N. Shen, J. Li, G. Li, B. Hu, J. Li, T. Liu, L. Gong, F. Huang, Z. Wang and X. Huang, Inorg. Chem., 2019, 58, 8079–8085 CrossRef CAS PubMed.
- B. Yang, W. Ming, M. H. Du, J. K. Keum, A. A. Puretzky, C. M. Rouleau, J. Huang, D. B. Geohegan, X. Wang and K. Xiao, Adv. Mater., 2018, 30, 1705801 CrossRef PubMed.
- Z. Zhang, Y. Lin, J. Jin, L. Gong, Y. Peng, Y. Song, N. Shen, Z. Wang, K. Du and X. Huang, Angew. Chem., Int. Ed., 2021, 60, 23373–23379 CrossRef CAS PubMed.
- F. Lin, H. Wang, H. Lin, W. Liu and J. Li, Chem. Commun., 2021, 57, 1754–1757 RSC.
- A. Vogler and H. Nikol, Comments Inorg. Chem., 1993, 14, 245–261 CrossRef CAS.
- Z. Li, Y. Li, P. Liang, T. Zhou, L. Wang and R.-J. Xie, Chem. Mater., 2019, 31, 9363–9371 CrossRef CAS.
- D. Chen, F. Dai, S. Hao, G. Zhou, Q. Liu, C. Wolverton, J. Zhao and Z. Xia, J. Mater. Chem. C, 2020, 8, 7322–7329 RSC.
- A. Biswas, R. Bakthavatsalam, B. P. Mali, V. Bahadur, C. Biswas, S. S. K. Raavi, R. G. Gonnade and J. Kundu, J. Mater. Chem. C, 2021, 9, 348–358 RSC.
- G. V. G. K. Robinson and P. H. Ribbe, Science, 1971, 172, 567–570 CrossRef PubMed.
- J. B. Luo, J. H. Wei, Z. Z. Zhang and D. B. Kuang, Inorg. Chem., 2022, 61, 338–345 CrossRef CAS PubMed.
- M. Li, J. Zhou, M. S. Molokeev, X. Jiang, Z. Lin, J. Zhao and Z. Xia, Inorg. Chem., 2019, 58, 13464–13470 CrossRef CAS PubMed.
- H. S. Jung, P. Verwilst, W. Y. Kim and J. S. Kim, Chem. Soc. Rev., 2016, 45, 1242–1256 RSC.
- P. Kumar, A. Ghosh and D. A. Jose, ChemistrySelect, 2021, 6, 820–842 CrossRef CAS.
- D. Wang, H. Zhao, H. Li, S. Sun and Y. Xu, J. Mater. Chem. C, 2016, 4, 11050–11054 RSC.
- J.-B. Luo, J.-H. Wei, Z.-Z. Zhang and D.-B. Kuang, Inorg. Chem., 2022, 61, 338–345 CrossRef CAS PubMed.
- C. F. Macrae, I. Sovago, S. J. Cottrell, P. T. A. Galek, P. McCabe, E. Pidcock, M. Platings, G. P. Shields, J. S. Stevens, M. Towler and P. A. Wood, J. Appl. Crystallogr., 2020, 53, 226–235 CrossRef CAS PubMed.
- G. M. Sheldrick, Acta Crystallogr., Sect. C: Struct. Chem., 2015, 71, 3–8 Search PubMed.
|
This journal is © The Royal Society of Chemistry 2024 |