Treatment of mine water for the fast removal of zinc and lead by wood ash amended biochar†
Received
1st May 2022
, Accepted 5th August 2022
First published on 12th August 2022
Abstract
Lead and zinc mines are a primary source of environmental (post)-transition metal contamination resulting in major water pollution. In this study, the use of biochar amended with wood ash (WAS) was evaluated as a method to remediate zinc and lead contaminated mine water. Water from Nantymwyn lead mine, with zinc and lead concentrations as high as 12.1 mg L−1 and 1.7 mg L−1 respectively was used. The contact time for WAS to immobilise zinc and lead (1 min to 24 h), immobilisation mechanisms and maximum measured removal of lead and zinc were studied. FTIR spectroscopy and XPS was used to characterise WAS and the aqueous modelling program PHREEQC (pH redox equilibrium) was used to analyse mine water speciation. The fast removal performance of a biochar is a key indicator of its viability to be used as a green remediator. If the required contact time to remediate contaminated water is too long the sorbent becomes impractical. This study demonstrated that WAS removed 97% of zinc and 86% of lead within the first minute of contact with the mine water (0.5 g of WAS per 20 mL of mine water), with a maximum measured removal of 14.8 mg g−1 for zinc and 23.7 mg g−1 for lead (using 0.1–0.002 g of WAS per 40 mL of mine water). Fast removal was primarily a result of precipitation, and subsequent capture by WAS, and ion exchange. These findings show that WAS has the potential to be scaled up and deployed at mine sites to remediate contaminated water.
Environmental significance
The anthropogenic effect of mining on the aquatic environment is a global issue and has led to contamination of waters by up to 1000 times water quality thresholds for Zn and Pb. Larch biochar with the novel amendment of wood ash (WAS), was studied to determine if it was an effective remediator of contaminated mine water. Results demonstrated that WAS removed 97% of Zn and 86% of Pb within one minute of contact, with a maximum measured removal of 14.8 mg g−1 and 23.7 mg g−1, due to precipitation and ion exchange. This shows that WAS is a viable, sustainable and cost-effective option to remediate (post)-transition metals in mine water and other polluted waters such as motorway runoff and industrially polluted rivers.
|
1. Introduction
Active and abandoned mines are a major source of contamination for both terrestrial and aquatic environments.1 Water pollution from these mines can originate from point sources such as mine adits, or diffuse sources such as spoil heaps.2 In the UK, the potential contamination by mines is compounded by the fact that owners of mines abandoned before 1999 bear no responsibility for their impacts. In Wales, there are over 1300 such mines affecting over 700 km of river all of which were abandoned before 1999.3,4 Pb/Zn mining and smelting are seen as some of the primary sources of environmental (post)-transition metal contamination with Pb and Zn mines having resulted in major water contamination.5
Both Zn and Pb concentrations that are above European Union Water Framework Directive (WFD) standards have the potential to cause harm to the environment and to human health. Current WFD thresholds are 0.0129 mg L−1 for Zn and up to 0.014 mg L−1 for Pb.6 Metals such as Zn and Pb can enter the food chain harming plants, herbivores, carnivores and humans.7,8 High concentrations of Pb can have effects on the central nervous system and kidneys, interfere with brain development and adversely affect both male and female reproduction with blood concentrations above 60 μg dL−1.9 Exposure to Zn at concentrations ∼6.5 mg L−1 can cause nausea, vomiting, epigastric pain, lethargy, and fatigue.10,11
Where polluted mine water is present remediation is important not just to improve the quality of the water but also to improve the surrounding environment; mine impacted water can bioaccumulate in flora, fauna and soils adjacent to the mine impacted water. As a result improvements in water quality can also improve environmental quality in the area surrounding the watercourse.12 Indirectly this improvement in water quality can improve useable farmland and restrict the intake of metals into plants, animals, and eventually humans. Currently there are three basic methods of water remediation or improving water quality: (i) diversion of the water away from the mine so that metals are not leached into rivers and streams; (ii) active treatment of the polluted water e.g. with chemical dosing or electrical coagulation; and (iii) passive treatment e.g. with settling ponds or reed beds.13 As each site has different issues due to water chemistry, underground workings, physical space and access, remediation should be tailored on an individual site basis. Current remediation of metal mines in the UK has been limited due to the regulatory framework as no one holds direct responsibility for the mines and resultant pollution and where it does occur it is often expensive, requires significant maintenance and creates potentially toxic waste products.14,15
Water diversion is one of the remediation techniques that can be seen at Frongoch Mine, Wales, UK, an abandoned Pb–Zn mine with subterranean connections to Wemyss Pb–Zn mine. Frongoch has a large area of spoil mounds suitable for reprofiling and capping, and a single entry point for the majority of the water flowing into the mine. These site conditions make it possible for water in Frongoch to be diverted from entering the mine. The channel the diverted water follows has been lined with concrete cloth to reduce contact with spoil, and the largest spoil heaps have been recontoured and capped with either 300+ mm of clay or a geotechnical clay liner, covered with 100 mm of topsoil.16 While the mine continues to fail environmental quality standards, the Zn load in the stream was reduced from 23.0 t p.a. to 7.4 t p.a.16 This approach will however only be possible where site conditions allow stream diversion.
An example of a passive mine water treatment scheme can be seen at Force Crag Mine in Cumbria, a Zn/Pb mine that was abandoned in 1991, which adversely affects 60 km of river. Since 2014 the mine effluent has been captured by two parallel vertical flow pond compost bioreactors followed by a small wetland, removing 95–99% of the Zn.17 It is estimated however that the substrate will require replacing after 10 years of use, and the disposal alone of this is almost the same as the capital expenditure for building the system.17 A similar but larger system has been in use in West Fork Pb mine in Missouri, treating 76 L s−1 of water and reducing the Zn concentration by 75%, with a design life of 12 years.18
Active treatment systems, such as those employed at Wheal Jane in Cornwall, require less land area for the system, but ongoing dosing of chemicals; 2000 t of hydrated lime and 7 t of anionic flocculant, averaging £1.5 per M annually.14 The Wheal Jane active treatment system removes up to 99.2% of the metals, resulting in 600 t of metal contaminated sludge to dispose of annually.
A cost-effective alternative to these remediation techniques is the use of biochar to remove environmental contaminants from aqueous media. The use of biochar has been highlighted as a cost effective remediation technique particularly in comparison to other removal techniques such as anionic liquid ion exchange (ALIX) process, activated carbon or packed bed filtration.19–22 Biochar is a porous, carbonaceous material produced by biomass pyrolysis at temperatures ranging from 350–1000 °C under limited oxygen conditions.23 In addition to the removal of contaminants from aqueous media studies have also highlighted several other benefits of biochar including carbon sequestration and enhancing soil fertility.19,24 Its appeal is heightened as a result of its relatively low cost, simple production process and the ability to use a vast number of locally sourced feedstocks including sustainable and waste materials.23,25–29
Biochar has six key immobilization mechanisms for inorganic contaminants in aqueous media namely: cation exchange, complexation, electrostatic attraction, cation π bonding, reduction and subsequent sorption, and precipitation30 (Fig. 1). Several mechanisms can be relevant in any given biochar and these mechanisms can remove contaminants at different rates. Initial fast stage adsorption can be attributed to mechanisms such as electrostatic attraction and ion exchange whereas rate limiting steps have been seen to mainly involve chemical processes such as inner sphere complexation and co-precipitation.31 The fast removal performance of biochar has been highlighted as a necessity for a successful adsorbent;32 if the required contact time between sorbent and sorbate to remediate a contaminated water is too long then the use of that sorbent becomes impractical. This is particularly true in systems with a continuous flow where contact time between biochar and contaminant is limited.
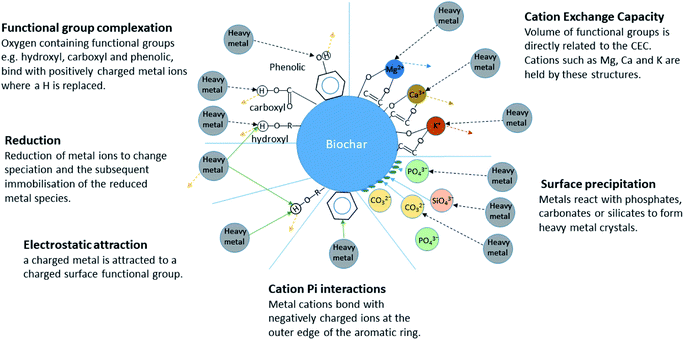 |
| Fig. 1 Immobilisation mechanisms of inorganic contaminants in aqueous media.30 | |
Wood ash, a waste product of biomass power plants, has been used as a novel amendment to biochar to improve immobilisation of metals.33 The use of a waste product rather than chemical activation to improve immobilisation not only increases the sustainability of biochar but prevents costs associated with chemical activation being incurred.34 The increased rates of immobilisation are as a result of the wood ash mineral fraction and pH buffering capacity.35 The alkali and alkaline earth metals added are central to ion exchange as well as increasing the pH of its environment inducing changes in metal speciation favourable to the immobilisation of Zn and Pb.36,37 Minerals including P and Si added to the biochar by the wood ash are also key in the formation of phosphates and silicates important in forming co-precipitates.
Research has demonstrated that biochar can be successful in the removal of key mine water contaminants such as Zn and Pb from aqueous solution.33 Most existing biochar studies do not focus on the scalability and practicality of the use of biochar which are essential considerations in moving to the end goal of industry-scale application. The required contact time for remediation is often overlooked. When considering practical use of biochar in a field setting the minimum contact time in which biochar can reach equilibrium is an important factor. This factor is particularly relevant in systems with continual flow, such as streams or rivers, where contact time between aqueous contaminant and sorbent is much shorter than in stagnant systems, such as ponds. Synthetic single contaminant solutions are often used rather than complex waters such as mine water. The adoption of single contaminant solutions neglects the fact that contaminants like Zn or Pb often co-exist with other pollutants, such as Fe, Al or SO42− causing competition for sorption sites effecting sorption.38,39 Furthermore, the biochars studied are often produced on a laboratory scale rather than on a large or industrial scale putting further steps in the process to move toward scaled up production.40,41
The overall aim of this study is to determine if the use of wood ash amended biochar is a viable option to remediate mine water contaminated with Zn and Pb. The following objectives have been devised to meet this aim using wood ash amended biochar produced by an industry scale pyrolysis gasification kiln: (i) to quantify the contact time required for WAS to immobilise Zn and Pb (ii) to quantify the maximum measured removal of Zn and Pb by WAS (iii) to identify the mechanisms key to Zn and Pb immobilisation.
2. Methods
2.1 Study site
Nantymwyn Pb mine is one of a number of mines accessing the mineral veins of Mid Wales which cross the site in a north easterly direction carrying galena (PbS) and sphalerite (ZnS).42 The mine (Fig. 2) (52°5′12′′ N; 3°46′20′′ W) was worked sporadically over centuries by a variety of methods as technologies progressed, leaving a complex hydrogeological system, scarcely mapped when in operation and now complicated further by 90 years of degradation since closure.43 Records detailing quantities of ore recovered are limited, but in the final four months of full scale operation in 1930, 168 t of Pb and 548 t of Zn concentrates were recovered.43 Nantymwyn causes the River Tywi to fail European Union Water Framework Directive (WFD) standards for Zn for up to 69 km.4 The River Tywi also provides drinking water to Swansea and Carmarthen from an intake at Nantgaredig. However, the substantially larger flow of the River Tywi means that metals from Nantymwyn are diluted.44,45 Much of the mine site is now a forestry plantation, but the main spoil heaps remain bare. The site is intersected by two streams; firstly, to the north the Nant y Bai flows through the main Nantymwyn spoil heaps and areas where the more recent surface operations were carried out.46 The Nant y Bai receives diffuse polluted water from spoil run off, including over 30% of its Zn load from tributary 1, which flows through spoil and tailings before joining the Nant y Bai, as well as subterranean sources.47 The second stream to the south is the smaller Nant y Mwyn, which does not flow through any visible surface spoil but receives point source input from the Pannau adit and the Deep Boat Level, both of which drain the mine workings. Zn and Pb WFD standards of 0.0129 mg L−1 and 0.014 mg L−1 respectively are both widely exceeded at Nantymwyn; this is apparent at both the Deep Boat Level and Tributary 1.48,49
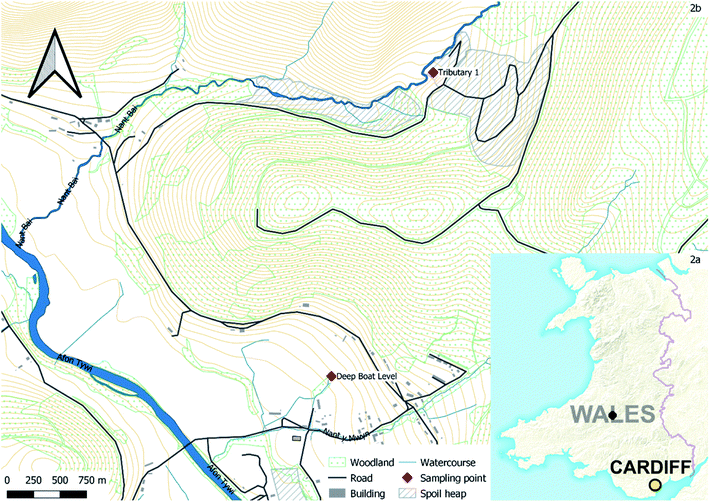 |
| Fig. 2 (a) Nantymwyn within Wales, (b) sample point locations (ordnance survey 2013, 2020). | |
2.2 Biochar production
European larch (Larix decidua (L.) Mill.) wood chips were pyrolyzed in a Pyrocal BigChar-1000 pyrolysis-gasification kiln at a temperature of 485–530 °C, with a retention time of ∼90 s. Wood ash, originating from a renewable energy plant in the UK, was added to the still hot biochar at a ratio of 1
:
1 and mixed for 15 min in a cement mixer to sinter the materials and then granulated to <3 mm with a Tria G1 granulator.35 Wood ash was selected as an amendment to the pristine biochar because of its mineral fraction (specifically Ca, K, Mg, S, P and Si) and pH buffering capacity. However, as a result of the mineral fraction the wood ash amended biochar (WAS) can potentially leach nutrients such as nitrates, phosphates and sulphates.50 Cairns et al.51 demonstrated that rinsing wood ash amended biochar with 200 mL of water per 5 g biochar removes these unbound nutrients and reduces leaching to below water framework directive thresholds. To remove these unbound nutrients every 15 g of biochar was rinsed with 600 mL of deionised water. To rinse, the biochar was added to an open-ended polypropylene column with an internal diameter of 31 mm, a wall thickness of 1 mm and a length of 500 mm. An 80 μm mesh was used to stabilise the column content and further prevent loss of biomass.33
2.3 Sample collection and mine water characterisation
Water was obtained from the Deep Boat Level adit and Tributary 1 at Nantymwyn lead mine (Fig. 2b) and sub-divided into five identical 1 L polyethylene terephthalate (PET) bottles. These bottles were each rinsed with sample water three times before filling, and stored at 4 °C, in accordance with national guidelines.52 The pH, electrical conductivity, temperature, redox and dissolved oxygen levels were taken concurrently at each site with a calibrated Hanna Instruments HI98194 Multiparameter meter following national guidelines.52
2.4 Sorption experiments
Batch sorption experiments were carried out to determine the effect of contact time on immobilisation using the Deep Boat Level adit and Tributary 1 water and to determine the maximum measured removal of Zn and Pb by biochar using Tributary 1 water. For both sorption batch experiments the sorbent was oven dried at 105 °C for 24 h. To determine the effect of contact time on immobilisation, biochar (0.5 g with a particle size of <3 mm) was added to 5 mL of deionised water in 50 mL polyethylene Falcon tubes and shaken for one hour to saturate the sorbent thus reducing the time needed for the mine water to saturate WAS. 0.5 g biochar was a larger quantity than previous studies to take into account the reduced immobilisation capacity of mine water compared to synthetic contaminated water.35 Mine water was then added (20 mL) to the biochar slurry and shaken for a known amount of time (1 min, 5 min, 15 min, 30 min, 1 h and 24 h). To determine the maximum measured removal of Zn and Pb by biochar a known amount of biochar (0.1 g, 0.05 g, 0.03 g, 0.02 g, 0.01 g, 0.006 g, 0.003 g and 0.002 g) was added to 40 mL of Tributary 1 mine water in 50 mL polyethylene Falcon tubes and shaken for 24 h. For both sorption batch experiments agitation was achieved on a Unitwist 400 Orbital Shaker at ∼280 rpm. The solution was subsequently separated from the sorbent using an MSE Centaur 2 centrifuge at 3000 rpm for 15 min.53 The supernatant was then acidified to <pH 2 with 1 mL of 70% HNO3 before being filtered with a 0.45 μm PTFE syringe filter for analysis. All experiments were performed in triplicates using a batch sorption equilibrium method with biochar without mine water and mine water without biochar used as controls.53
Zn and Pb concentrations of the acidified supernatants were measured using Microwave Plasma Atomic Emission Spectroscopy (MP-AES 4200, Agilent Technologies Inc., USA). For contact time sorption batch experiments the percentage of sorbent removal was calculated from the difference between initial metal concentration and final metal concentrations in the aqueous phase:
where
Ci is the initial concentration of metals in solution and
Caq is the final equilibrium concentration of metals in solution. For maximum measured removal experiments removal in mg g
−1 was calculated as the difference between initial metal concentration and the final metal concentration in the aqueous phase:
where
q is the maximum measured removal,
V is the volume of solution and
W is the weight of the biochar.
2.5 Biochar properties
A range of biochar properties were determined to understand how they effected immobilisation. The release of base cations (Ca, K, Na and Mg) by the biochar were measured for biochar that had been agitated with deionised water and for biochar that had been agitated with mine water and compared to review possible cation exchange. The pH, EC and alkalinity of the biochar supernatant from the sorption experiments were measured prior to acidification using a calibrated Voltcraft pH Meter, a calibrated Whatman CDM 400 Conductivity Meter and a Hanna HI-3811 Alkalinity test kit respectively. Fourier transform infrared spectroscopy (FTIR) was undertaken using a PerkinElmer Spectrum Two FTIR Spectrometer. Measurements in the range 400–4000 cm−1 were determined to compare possible changes to functional groups, minerals capable of precipitation and aromatic structures. Comparisons of the spectra were made for biochar before sorbent loading and at each timed stage of the sorption experiment. X-ray photoelectron spectroscopy (XPS) analysis was carried out on a Kratos Axis Supra instrument using a monochromatic Al Kα X-ray source at 225 W, with a 15 mA emission current. Each sample had multiple wide scans at pass energy of 160 eV over the binding energy range of 1200–0 eV to identify all possible elements present, with a step size of 1 eV. High resolution spectra were scanned with a pass energy of 40 eV, a step size of 0.01 eV and a multi sweep dwell time of 2000 ms to improve the signal to noise ratios for the lower concentration elements of interest. The binding energy axis was charge correct to the C–C component of the carbon peak at 284.8 eV. Casa XPS (2.3.22PR1.0) was used to quantify the data using the Kratos sensitivity factor library, standard Shirley backgrounds and Gauss–Lorentz peak models. XPS analysis was conducted to identify the change in biochar characteristics, especially in composite compounds, before and after sorption.
2.6 Metal speciation
Speciation analysis of the mine water and biochar supernatants were carried out using the pH redox equilibrium (PHREEQC) program (version 3.7.1) and the MINTEQV4 database.54 The pH, redox, temperature and concentrations of base cations, metal contaminants, alkalinity and nutrients were used as model inputs. To confirm the PHREEQC modelling, the proportion of mobile Zn and Pb species in Tributary 1 were further investigated by comparing acidified and non-acidified Tributary 1 samples. One set of mine water samples were acidified to <pH 2 with 1 mL of 70% HNO3 before being filtered with a 0.22 μm PTFE syringe filter, a further set was not acidified and filtered with a 0.22 μm PTFE syringe filter. All experiments were performed in triplicates and measured using Microwave Plasma Atomic Emission Spectroscopy (MP-AES 4200, Agilent Technologies Inc., USA).
3. Results and discussion
3.1 Mine water chemistry
The Deep Boat Level was sampled 12 times during 2019–2020 and average metal concentration were calculated.47 It was characterised by a high Zn concentration of 12.7 mg L−1, a Pb concentration of 0.2 mg L−1, a pH of 7.0 and a flow of 24 L s−1.48 Tributary 1 had Zn concentrations of 9.8 mg L−1, Pb concentrations of 3.8 mg L−1, a pH of 6.4 and a flow of 3.4 L s−1, again from 12 samples taken 2019–2020.48 The Zn and Pb concentrations for both Deep Boat Level and Tributary 1 are substantially above the WFD standards for dissolved Zn and Pb in the River Tywi which are 0.0129 mg L−1 and 0.014 mg L−1 respectively.49
3.2 Contaminant removal time-scales
The time in which biochar can immobilise contaminants from aqueous media is an important aspect of remediation with flowing water, as contaminant contact time with any sorbent is limited.32,55 Mechanisms such as precipitation and ion exchange have been demonstrated to be important in early stage immobilisation whereas mechanisms such as co-precipitation can take longer and as such be seen as rate limiting factors.31
The immobilisation of Zn from both the Deep Boat Level and Tributary 1 was a relatively fast process with 97% of mine water Zn removed in the first minute and equilibrium and complete removal achieved around 1 h (Fig. 3A and B). The immobilisation of Pb from Tributary 1 was also a relatively fast process with 86% of mine water Pb removed in the first minute and an equilibrium of 92% removal again achieved around 1 h. Pb concentration in the Deep Boat Level mine water was below the limit of detection (<0.1 mg L−1) and as such removal was not quantified.
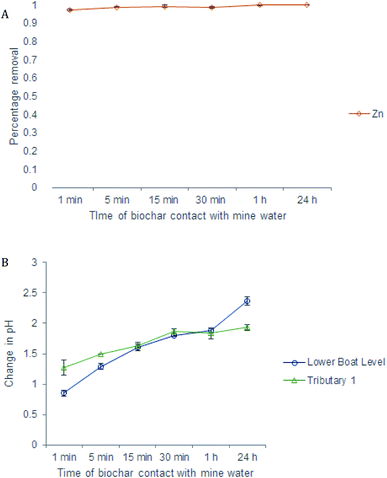 |
| Fig. 3 (A) Percentage of zinc removed from the Deep Boat Level mine water by contact time with wood ash amended biochar. Lead concentrations in the Deep Boat Level being below detection limits (<0.1 mg L−1); (B) percentage of zinc and lead removed from Tributary 1 mine water by contact time with wood ash amended biochar. | |
3.3 Immobilization mechanisms
The pH of both the Deep Boat Level and Tributary 1 mine water was increased as a result of contact with the wood ash amended biochar (WAS) (Fig. 4A) leading to early stage Zn and Pb immobilisation and capture via precipitation (Fig. 4B). An increase in media pH can be instrumental to changes in metal speciation that are favourable for the immobilisation of metals such as Zn and Pb.33 After a contact time of 1 min with WAS the Deep Boat Level mine water increased in pH to 8.2 and after 1 h increased to a pH of 9.2 where equilibrium was reached. After a contact time of 1 min with the WAS the Tributary 1 mine water pH increased to 8.9 and after 1 h it increased to a pH of 9.4 at which point equilibrium was reached.
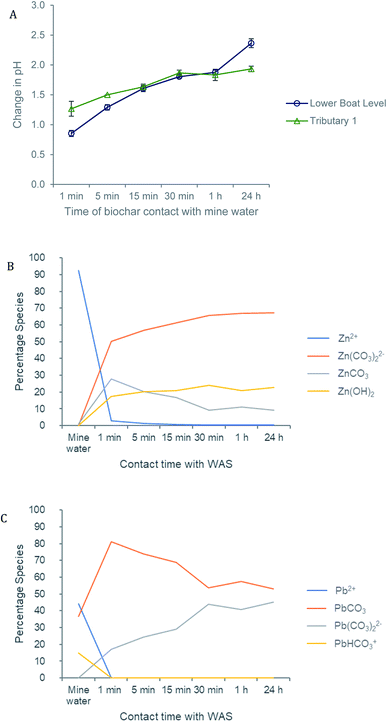 |
| Fig. 4 (A) Change in Deep Boat Level and Tributary 1 mine water pH by contact time with wood ash amended biochar; (B) speciation plots of Zn in Tributary 1 mine water across contact time with wood ash amended biochar; (C) speciation plots of Pb in Tributary 1 mine water across contact time with wood ash amended biochar. | |
Speciation modelling of Zn and Pb ions was conducted using PHREEQC software.56 According to the PHREEQC calculations with the environmental conditions and pH observed in the mine water of Tributary 1 before the addition of WAS, 92% of Zn would be in mobile divalent forms (Fig. 4B). This proportion of mobile Zn was confirmed by a comparison of Tributary 1 acidified (pH < 2) and non-acidified samples. Mobile species were captured in the filtered eluate whereas immobile species were filtered out of the eluate. Once WAS had been applied for 1 min, the PHREEQC modelling demonstrated that Zn mobile divalent forms only constitute 3% of Zn species present (Fig. 3B). This change was caused by WAS promoting the formation of immobile carbonates and hydroxides, such as ZnCO3. Divalent forms of Zn dropped to close to 0% when WAS had been added between 15 min and 24 h. The distribution shifts from divalent Zn to immobile zinc carbonate and hydroxide forms, forming mineral precipitates which were captured on the surface of WAS. The capture of ZnCO3 on the surface of the biochar was confirmed by XPS analyses where peaks attributed to ZnCO3 were observed only when WAS had been in contact with the Tributary 1 mine water (Fig. 5A and B).
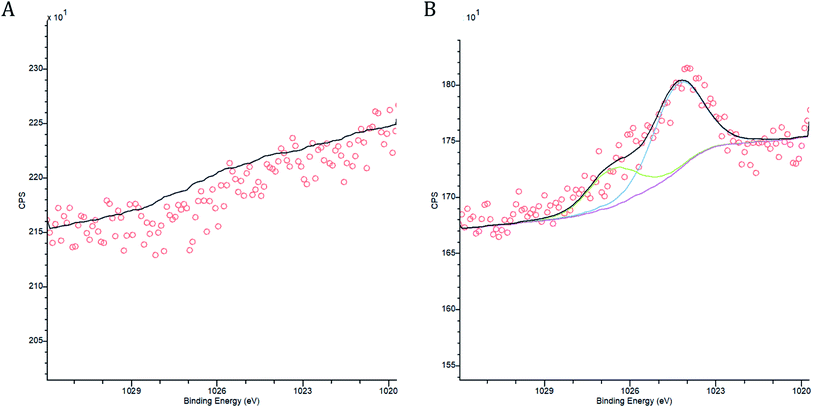 |
| Fig. 5 X-ray photoelectron spectroscopy (XPS) region scans and peak fitting for wood ash amended biochar (A) Zn peaks prior to contact with Tributary 1 mine water (B) Zn peaks after 24 hours contact time with Tributary 1 mine water. | |
PHREEQC results also showed that before the addition of WAS, 44% of the Pb in the mine water of Tributary 1 would be in mobile divalent forms (Fig. 4C). Again, that a proportion of Pb was mobile was confirmed by the comparison of filtered acidified and non-acidified Tributary 1 mine water. According to PHREEQC modelling, once WAS had been applied to the mine water for 1 min, the divalent Pb concentration was reduced to close to 0%; this proportion remained constant for the 24 h period of this study. Mobile divalent Pb species shift to immobile lead carbonate and hydroxide forms which dominate speciation causing Pb precipitation and capture by WAS. The formation of lead carbonates, such as Pb(CO3) modelled by PHREEQC, have been seen to be related to the high alkalinity of the biochar and soluble carbonate, sulphate and phosphate anions.57,58 Regular sampling of Nantymwyn has shown sulphate concentration to be as high as 11 mg L−1 adding substantially more anions into the system possibly playing a role in Zn and Pb speciation and further increasing the formation of precipitates.48,59 Given the right conditions, as the addition of WAS to the Nantymwyn system provides, precipitation is a rapid process. This, in part, explains the speed of Zn and Pb removal by WAS.
Ion exchange was also seen to play a role in early stage sorption of Zn and Pb by WAS. A rise in base cation release in the presence of metals can signify ion exchange on the surface of the biochar as the divalent contaminants replace the divalent cations such as Ca2+ and Mg2+.60 In this study concentrations of Ca and/or Mg in solution increased within the first minute of the experiment and remained broadly steady for the 24 h sampling period indicating that cation exchange played an early role in sorption of Zn and Pb and continued to be of importance for the duration of the study (Fig. 6A and B). Early stage sorption of Zn and Pb have been ascribed to ion exchange in several previous studies often alongside electrostatic attraction, with Ca and Mg being cited as the ions most important in the exchange, which is consistent with the findings of this study.31,61
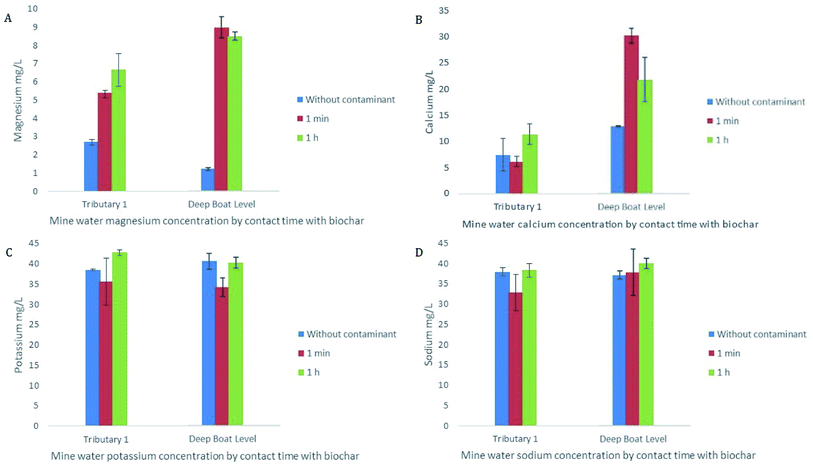 |
| Fig. 6 (A) Changes in magnesium concentrations by contact time with wood ash amended biochar for Tributary 1 and Deep Boat Level (B) changes in calcium concentrations by contact time with wood ash amended biochar for Tributary 1 and Deep Boat Level (C) changes in potassium concentrations by contact time with wood ash amended biochar for Tributary 1 and Deep Boat Level (D) changes in sodium concentrations by contact time with wood ash amended biochar for Tributary 1 and Deep Boat Level. | |
3.4 Maximum measured Zn and Pb removal and late stage immobilisation mechanisms
The maximum measured removal of Zn and Pb by wood ash amended biochar (WAS) was 14.8 mg g−1 and 23.7 mg g−1 respectively. Maximum immobilisation of Zn was seen with 0.5 mg of adsorbent per L of mine water and maximum immobilisation of Pb was seen with 0.05 mg of adsorbent per L of mine water. Immobilisation of Pb continued at 84% even as the proportion of Zn immobilised dropped to 3% demonstrating that WAS affinity with Pb is stronger than with Zn and that Pb removal can continue even once the capacity of WAS to remove Zn is exhausted. The key mechanisms that drive the removal of Zn and Pb through to maximum measured removal remained precipitation, and subsequent capture by WAS, and ion exchange.
PHREEQC modelling demonstrated that as the proportion of immobile Zn species reduced so too did the proportion of Zn immobilised by WAS with a strong correlation (r = 0.91; p < 0.01) illustrating the importance of precipitation to Zn removal (ESI 2†). The modelling also shows that immobile Pb species remains as high as 92% even when Zn immobile species reduces to 20%. This partly explains why WAS continues to remove Pb beyond Zn exhaustion.
Cation exchange with Ca and Mg is also a relevant mechanism in the ongoing removal of the metals of concern by WAS. As the removal of Zn decreases the concentration of Ca, and to a lesser extent Mg, also decrease indicating that these base cations have been replaced by Zn and reducing the sites left available for Zn (Fig. 7A). There is a strong correlation between Ca concentration and Zn removal (r = 0.85; p < 0.01) as well as a strong correlation between Mg concentration and Zn removal (r = 0.77; p < 0.01). FTIR analysis further demonstrates the importance of ion exchange in the removal of metals of concern (Fig. 7B). The FTIR peak at 1418 is assigned to the carboxyl surface functional group;35,62 when immobilisation of Zn by WAS is as high as 99% this peak is strong. However, as immobilisation of Zn decreases to exhaustion this peak diminishes considerably. This, in conjunction with the correlation between Zn immobilisation and Ca and Mg concentrations, shows that ion exchange plays an important role in the immobilisation of metals of concern from the mine water from initial immobilisation to exhaustion.
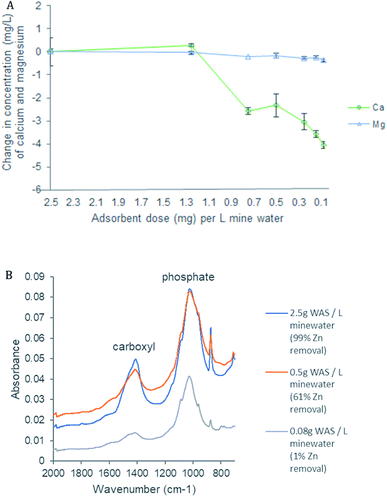 |
| Fig. 7 (A) The change in concentration (mg L−1) of calcium and magnesium in the presence of different quantities of wood ash amended biochar (WAS) in a litre of mine water. (B) FTIR spectra of wood ash amended biochar (WAS) before maximum measured removal of Zn (2.5 mg WAS per L mine water), maximum measured removal of Zn (0.5 g WAS per L mine water) and at exhaustion of Zn (0.08 g WAS per L mine water). | |
4. Conclusion
On a global scale, mine sites are a major source of contamination of aqueous environments. This study investigated if wood ash amended biochar (WAS) could immobilise Zn and Pb with a short enough contact time to be viable as a green remediator using mine impacted waters from the Deep Boat Level and Tributary 1 areas of the Nantymwyn. These waterways displayed Zn concentrations as high as 12.1 mg L−1 and Pb concentrations as high as 1.7 mg L−1, both considerably above WFD standards for the River Tywi (0.0129 mg L−1 for Zn and 0.014 mg L−1 for Pb). WAS removed 97% of Zn and 86% of Pb within the first minute of contact time with the mine water, with a maximum measured removal of 14.8 mg g−1 for Zn and 23.7 mg g−1 for Pb. The removal of these metals of concern was primarily caused by precipitation, and subsequent capture by WAS, and ion exchange; both processes were key to early stage immobilisation. Elevated pH and the presence of minerals shifted metal species to carbonates, such as Pb(CO3) and Zn(CO3) and hydroxides such as Zn(OH)2. Under such conditions, these metal species are more susceptible to precipitation. The resultant capture of the species modelled by PHREEQC on the surface of the biochar was observed by XPS. Ion exchange was demonstrated between the metal contaminants and base cations specifically Ca and Mg. The fast removal performance due to these mechanisms demonstrates the potential for WAS to remove pollutants from aqueous environments.
Conflicts of interest
There are no conflicts to declare.
Acknowledgements
Knowledge Economy Skills Scholarships (KESS) is a pan-Wales higher level skills initiative led by Bangor University on behalf of the HE sector in Wales. It is part funded by the Welsh Government's European Social Fund (ESF) convergence programme for West Wales and the Valleys. This work is part funded by the Welsh Government's European Social Fund (ESF) convergence programme for West Wales and the Valleys. The authors thank Richard Haine (Frog Environmental), Sion Brackenbury (TerrAffix Soil Solutions), Peter Lanfear (TerrAffix Soil Solutions) and Dr Ian Mabbett (Swansea University) for their continued support.
References
- S. J. Beane, S. D. W. Comber, J. Rieuwerts and P. Long, Abandoned metal mines and their impact on receiving waters: A case study from Southwest England, Chemosphere, 2016, 153, 294–306 CrossRef CAS PubMed.
- J. S. Rieuwerts, S. Austin and E. A. Harris, Contamination from historic metal mines and the need for non-invasive remediation techniques: A case study from Southwest England, Environ. Monit. Assess., 2009, 148(1–4), 149–158 CrossRef CAS PubMed.
-
Environment Agency Wales, Metal Mines Strategy for Wales, Cardiff, 2002, available from: https://naturalresources.wales/media/680181/metal-mines-strategy-for-wales-2.pdf Search PubMed.
-
Coal Authority, Metal Mine Failing Waterbodies Assessment - Overview Report, 2020;(February) Search PubMed.
- X. Zhang, L. Yang, Y. Li, H. Li, W. Wang and B. Ye,
et al., Impacts of lead/zinc mining and smelting on the environment and human health in China, Environ. Monit. Assess., 2012, 184, 2261–2273 CrossRef CAS PubMed . https://publications.environment-agency.gov.uk/pdf/.
-
Water Framework Directive, The Water Framework Directive (Standards and Classification) Directions (England and Wales), Water Framework Directive, 2015, vol. 40(2), p. 66, available from: https://www.legislation.gov.uk/uksi/2015/1623/pdfs/uksiod_20151623_en_auto.pdf Search PubMed.
- A. Kumar, A. Kumar, M. Cabral-Pinto, A. K. Chaturvedi, A. A. Shabnam and G. Subrahmanyam,
et al., Lead Toxicity: Health Hazards, Influence on Food Chain, and Sustainable Remediation Approaches, Int. J. Environ. Res. Public Health, 2020, 17, 2179 CrossRef CAS PubMed . https://www.mdpi.com/1660-4601/17/7/2179/htm.
- Z. Shi, S. Wang, B. Pan, Y. Liu, Y. Li and S. Wang,
et al., Effects of zinc acquired through the plant-aphid-ladybug food chain on the growth, development and fertility of Harmonia axyridis, Chemosphere, 2020, 259, 127497 CrossRef CAS PubMed.
- H. Needleman, Lead poisoning, Annu. Rev. Med., 2004, 55(1), 209–222 Search PubMed.
- G. J. Fosmire, Zinc toxicity, Am. J. Clin. Nutr., 1990, 51(2), 225–227 CrossRef CAS PubMed.
- R. A. Bozym, F. Chimienti, L. J. Giblin, G. W. Gross, I. Korichneva and Y. Li,
et al., Free zinc ions outside a narrow concentration range are toxic to a variety of cells in vitro, Exp. Biol. Med., 2010, 235(6), 741–750, DOI:10.1258/ebm.2010.009258.
- A. Sartorius, M. Johnson, S. Young, M. Bennett, K. Baiker and P. Edwards,
et al., Human health implications from consuming eggs produced near a derelict metalliferous mine: a case study, Food Addit. Contam., Part A, 2022, 39(6), 1074–1085, DOI:10.1080/19440049.2022.2062059.
- S. K. Thisani, D. V. von Kallon and P. Byrne, Review of remediation solutions for acid mine drainage using the modified hill framework, Sustainability, 2021, 13(15), 8118 CrossRef CAS.
-
L. Wyatt, I. Watson, S. Kershaw and A. Moorhouse, An adaptable and dynamic management strategy for the treatment of polluted mine water from the abandoned Wheal Jane Mine, Cornwall, UK, Proceedings of the Eighth International Seminar on Mine Closure, 2013, pp. 69–78, Fig. 1 Search PubMed.
-
S. A. Rose, Z. N. Matthews, G. Morgan, C. Bullen and P. Stanley, Sono-electrochemistry (Electrolysis with assisted Power Ultrasound) Treatment Trials of discharges from Cwm Rheidol – Ystumtuen mines, Ceredigion, Mid Wales, UK, IMWA “Mine Water: Technological and Ecological Challenges, 2019, pp. 262–268 Search PubMed.
-
P. Edwards, T. Williams and P. Stanley, Surface water management and encapsulation of mine waste to reduce water pollution from Frongoch Mine, Mid Wales, IMWA 2016 – Mining Meets Water – Conflicts and Solutions, 2016, pp. 546–553, Bick 1996 Search PubMed.
-
M. T. Bailey, C. J. Gandy and A. P. Jarvis, Reducing life-cycle costs of passive mine water treatment by recovery of metals from treatment wastes, IMWA 2016 – Mining Meets Water – Conflicts and Solutions, 2016;(January), pp. 1255–1262 Search PubMed.
-
J. Gusek and K. Clarke-Whistler, Where does the recovery of metal resources from passive treatment systems fit in sustainable development initiatives
associated with large mining projects?, 22nd American Society of Mining and Reclamation Annual National Conference 2005, 2005;1(October), pp. 454–469 Search PubMed.
- M. I. Inyang, B. Gao, Y. Yao, Y. Xue, A. Zimmerman and A. Mosa,
et al., A review of biochar as a low-cost adsorbent for aqueous heavy metal removal, Crit. Rev. Environ. Sci. Technol., 2016, 46(4), 406–433 CrossRef CAS.
- M. Inyang and E. Dickenson, The potential role of biochar in the removal of organic and microbial contaminants from potable and reuse water: A review, Chemosphere, 2015, 134, 232–240, DOI:10.1016/j.chemosphere.2015.03.072.
- D. Mohan, S. Rajput, V. K. Singh, P. H. Steele and C. U. Pittman, Modeling and evaluation of chromium remediation from water using low cost bio-char, a green adsorbent, J. Hazard. Mater., 2011, 188(1–3), 319–333, DOI:10.1016/j.jhazmat.2011.01.127.
- R. T. Bachmann, D. Wiemken, A. B. Tengkiat and M. Wilichowski, Feasibility study on the recovery of hexavalent chromium from a simulated electroplating effluent using Alamine 336 and refined palm oil, Sep. Purif. Technol., 2010, 75(3), 303–309 CrossRef CAS.
-
European Biochar Foundation, Guidelines for a Sustainable Production of Biochar, European Biochar Foundation, 2016;(December), pp. 1–22 Search PubMed.
- T. Kätterer, D. Roobroeck, O. Andrén, G. Kimutai, E. Karltun and H. Kirchmann,
et al., Biochar addition persistently increased soil fertility and yields in maize-soybean rotations over 10 years in sub-humid regions of Kenya, Field Crop. Res., 2019, 235(February), 18–26 CrossRef.
- M. Ahmad, A. Upamali, J. Eun, M. Zhang, N. Bolan and D. Mohan,
et al., Biochar as a sorbent for contaminant management in soil and water : A review, Chemosphere, 2014, 99, 19–33, DOI:10.1016/j.chemosphere.2013.10.071.
- F. Zhang, X. Wang, D. Yin, B. Peng, C. Tan and Y. Liu,
et al., Efficiency and mechanisms of Cd removal from aqueous solution by biochar derived from water hyacinth (Eichornia crassipes), J. Environ. Manage., 2015, 153, 68–73, DOI:10.1016/j.jenvman.2015.01.043.
- R. Z. Wang, D. L. Huang, Y. G. Liu, C. Zhang, C. Lai and G. M. Zeng,
et al., Investigating the adsorption behavior and the relative distribution of Cd2+ sorption mechanisms on biochars by different feedstock, Bioresour. Technol., 2018, 261(April), 265–271, DOI:10.1016/j.biortech.2018.04.032.
- X. Xiao, B. Chen, Z. Chen, L. Zhu and J. L. Schnoor, Insight into Multiple and Multilevel Structures of Biochars and Their Potential Environmental Applications: A Critical Review, Environ. Sci. Technol., 2018, 52(9), 5027–5047 CrossRef CAS PubMed.
- E. Fosso-Kankeu, R. Weideman, D. Moyakhe, F. B. Waanders, M. le Roux and Q. P. Campbell, Hydrothermal preparation of biochar from spent coffee grounds, and its application for the removal of cadmium from coal tailings leachate, J. South. Afr. Inst. Min. Metall., 2019, 119(7), 607–612 CAS . https://www.scielo.org.za/scielo.php?script=sci_arttext%26pid=S2225-62532019000700004%26lng=en%26nrm=iso%26tlng=en.
-
S. Cairns, G. Sigmund, I. Robertson and R. Haine, Engineered biochar as adsorbent for the removal of contaminants from aqueous medium, in Engineered Biochar: Fundamentals, Preparation, Characterization and Applications, ed. S. Ramola, O. Masek, A. Mendez and T. Tsubota, Springer Nature, 2022 Search PubMed.
- J. Ifthikar, T. Wang, A. Khan, A. Jawad, T. Sun and X. Jiao,
et al., Highly Efficient Lead Distribution by Magnetic Sewage Sludge Biochar: Sorption Mechanisms and Bench Applications, Bioresour. Technol., 2017, 238, 399–406, DOI:10.1016/j.biortech.2017.03.133.
- Z. Zhou, Z. Xu, Q. Feng, D. Yao, J. Yu and D. Wang,
et al., Effect of pyrolysis condition on the adsorption mechanism of lead, cadmium and copper on tobacco stem biochar, J. Cleaner Prod., 2018, 187, 996–1005, DOI:10.1016/j.jclepro.2018.03.268.
- S. Cairns, I. Robertson, G. Sigmund and A. Street-Perrott, The removal of lead, copper, zinc and cadmium from aqueous solution by biochar and amended biochars, Environ. Sci. Pollut. Res., 2020, 27(17), 21702–21715, DOI:10.1007/s11356-020-08706-3.
- P. Chakraborty, S. Show, W. Ur Rahman and G. Halder, Linearity and non-linearity analysis of isotherms and kinetics for ibuprofen remotion using superheated steam and acid modified biochar, Process Saf. Environ. Prot., 2019, 126, 193–204, DOI:10.1016/j.psep.2019.04.011.
- S. Cairns, S. Chaudhuri, G. Sigmund, I. Robertson, N. Hawkins and T. Dunlop,
et al., Wood ash amended biochar for the removal of lead, copper, zinc and cadmium from aqueous solution, Environ. Technol. Innovation, 2021, 101961 CrossRef CAS . https://linkinghub.elsevier.com/retrieve/pii/S235218642100609X.
- J. M. Cerrato, J. M. Blake, C. Hirani, A. L. Clark, A. M. S. Ali and K. Artyushkova,
et al., Wildfires and water chemistry: Effect of metals associated with wood ash, Environ. Sci.: Processes Impacts, 2016, 18(8), 1078–1089, 10.1039/C6EM00123H.
- R. B. Fidel, D. A. Laird, M. L. Thompson and M. Lawrinenko, Characterization and quantification of biochar alkalinity, Chemosphere, 2017, 167, 367–373 CrossRef CAS PubMed.
- H. Li, X. Dong, E. B. da Silva, L. M. de Oliveira, Y. Chen and L. Q. Ma, Mechanisms of metal sorption by biochars: Biochar characteristics and modifications, Chemosphere, 2017, 178, 466–478 CrossRef CAS PubMed.
- T. Bandara, J. Xu, I. D. Potter, A. Franks, J. B. A. J. Chathurika and C. Tang, Mechanisms for the removal of Cd(II) and Cu(II) from aqueous solution and mine water by biochars derived from agricultural wastes, Chemosphere, 2020, 254, 126745 CrossRef CAS PubMed.
- M. B. Ahmed, J. L. Zhou, H. H. Ngo, W. Guo and M. Chen, Progress in the preparation and application of modified biochar for improved contaminant removal from water and wastewater, Bioresour. Technol., 2016, 214, 836–851, DOI:10.1016/j.biortech.2016.05.057.
- D. Zhang, M. Yan, Y. Niu, X. Liu, L. van Zwieten and D. Chen,
et al., Is current biochar research addressing global soil constraints for sustainable agriculture?, Agric., Ecosyst. Environ., 2016, 226, 25–32 CrossRef.
-
M. J. Al-Atia and J. W. Barnes, Rubidium: a Primary Dispersion Pathfinder at Ogofau Gold Mine, Southern Wales, in Geochemical Exploration 1974: Proceedings of the Fifth International Geochemical Exploration Symposium. Vancouver: Association of Exploration Geochemists, ed. I. L. Elliot and W. K. Fletcher, 1975, pp. 341–352 Search PubMed.
- S. J. S. Hughes, Nant y Mwyn Mine, Llandovery, Dyfed, Br. Min., 1992, 45(45), 87–110 Search PubMed.
-
S. J. Brown, The Effects of Abandoned Metal Mines at Rhandirmwyn on the Quality of the Upper River Tywi SW/86/20, 1986 Search PubMed.
-
Welsh Water, Final Water Resources Management Plan, Water Resources Management, 2019;(August), available from: https://www.south-staffs-water.co.uk/community_environment/wrmp.asp Search PubMed.
-
G. W. Hall, Nantymwyn Mine, 2011, available from: https://www.aditnow.co.uk/Mines/Nantymwyn-Lead-Mine_4295/ Search PubMed.
-
A. M. L. Todd, I. Robertson, R. P. D. Walsh, P. Byrne, P. Edwards and T. Williams, Source Apportionment of Trace Metals at the Abandoned Nantymwyn Lead-Zinc Mine, Wales, IMWA 2021 - “Mine Water Management for Future Generations.”, 2021;(December), pp. 563–568 Search PubMed.
-
Natural Resources Wales, UK Water Quality Sampling Harmonised Monitoring Scheme Detailed Data, 2021, electronic dataset, available from: https://libcat.naturalresources.wales/folio/?oid=116338 Search PubMed.
-
Water Framework Directive, The Water Framework Directive (Standards and Classification) Directions (England and Wales), Water Framework Directive, 2015, vol. 40, Chapter 2, p. 66, https://www.legislation.gov.uk/uksi/2015/1623/pdfs/uksiod_20151623_en_auto.pdf Search PubMed.
- Y. Liang, X. Cao, L. Zhao, X. Xu and W. Harris, Phosphorus Release from Dairy Manure, the Manure-Derived
Biochar, and Their Amended Soil: Effects of Phosphorus Nature and Soil Property, J. Environ. Qual., 2014, 43(4), 1504–1509 CrossRef PubMed.
- S. Cairns, I. Robertson, P. Holliman and A. Street-Perrott, Treatments of wood ash amended biochar to reduce nutrient leaching and immobilise lead, copper, zinc and cadmium in aqueous solution: column experiments, Environ. Sci.: Water Res. Technol., 2022 10.1039/D1EW00962A.
-
Environment Agency, Taking Field Measurements: Using a Multi-Parameter Meter - Operational Instruction 528_06, Bristol, 2014 Search PubMed.
-
OECD, Test No. 106: Adsorption – Desorption Using a Batch Equilibrium Method, OECD Guidelines for the Testing of Chemicals, 2000, Section 1 Search PubMed.
-
D. L. Parkhurst and C. A. J. Appelo, User's Guide to PHREEQC (Version 2): A Computer Program for Speciation, Batch-Reaction, One-Dimensional Transport, and Inverse Geochemical Calculations. US GEological Survey Professional Paper 312, 1999, vol. 312, available from: https://www.xs4all.nl/%7Eappt/index.html Search PubMed.
- Y. Yang, Z. Wei, X. Zhang, X. Chen, D. Yue and Q. Yin,
et al., Biochar from Alternanthera philoxeroides could remove Pb(II) efficiently, Bioresour. Technol., 2014, 171(1), 227–232, DOI:10.1016/j.biortech.2014.08.015.
- B. Palumbo-Roe, B. Klinck, V. Banks and S. Quigley, Prediction of the long-term performance of abandoned lead zinc mine tailings in a Welsh catchment, J. Geochem. Explor., 2009, 100(2–3), 169–181 CrossRef CAS.
- D. Chen, R. Li, R. Bian, L. Li, S. Joseph and D. Crowley,
et al., Contribution of Soluble Minerals in Biochar to Pb2+ Adsorption in Aqueous Solutions, BioResources, 2016, 12(1), 1662–1679 Search PubMed.
- T. Zhang, X. Zhu, L. Shi, J. Li, S. Li and J. Lü,
et al., Efficient removal of lead from solution by celery-derived biochars rich in alkaline minerals, Bioresour. Technol., 2017, 235, 185–192, DOI:10.1016/j.biortech.2017.03.109.
-
C. M. Dean, J. J. Sansalone, F. K. Cartledge and J. H. Pardue, Influence of Hydrology on Rainfall-Runoff Metal Element Speciation, 2005;(April):pp. 632–643 Search PubMed.
- M. Uchimiya, I. M. Lima, T. Klasson, S. Chang, L. H. Wrtelle and J. E. Rodgers, Immobilization of Heavy Metal Ions ( Cu II, Cd II, Ni II, and Pb II ) by Broiler Litter-Derived Biochars in Water and Soil, J. Agric. Food Chem., 2010, 5538–5544 CrossRef CAS PubMed.
- J. Song, S. Zhang, G. Li, Q. Du and F. Yang, Preparation of montmorillonite modified biochar with various temperatures and their mechanism for Zn ion removal, J. Hazard. Mater., 2020, 391, 121692 CrossRef CAS PubMed.
- M. Iqbal, A. Saeed and I. Kalim, Characterization of adsorptive capacity and investigation of mechanism of Cu2+, Ni2+ and Zn2+ adsorption on mango peel waste from constituted metal solution and genuine electroplating effluent, Sep. Sci. Technol., 2009, 44(15), 3770–3791 CrossRef CAS.
|
This journal is © The Royal Society of Chemistry 2022 |
Click here to see how this site uses Cookies. View our privacy policy here.