Perspectives on palladium-based nanomaterials: green synthesis, ecotoxicity, and risk assessment
Received
20th October 2020
, Accepted 16th November 2020
First published on 7th January 2021
Abstract
Palladium-based nanomaterials (Pd-NMs) have been mass produced and applied due to their remarkable properties and high earth abundance. This makes Pd-NMs come into frequent contact with the environment and enter the ecological environment. Comparative analysis of the toxicological data revealed that Pd-NMs have acute or chronic toxicity in both in vitro and in vivo biological receptors models, but only limited information has been provided on the possible environmental migration and transformation or concentration distribution in the environmental media of Pd-NMs. Therefore, a perspective is needed of the existing data to provide a more professional and comprehensive assessment of their ecotoxicity and sustainable development. This perspective describes the critical knowledge needed to assess their ecological risks. We recommend focusing on the current and future concentration and distribution of Pd-NMs in the environment, guiding the assessment of the full-cycle ecotoxicity of Pd-NMs, and strongly encourage the quantitative measurement of the concentration level of Pd-NMs in the real environment.
Environmental significance
As nanomaterials develop, it is important to consider their effects on organisms and ecosystems and so environmental protection and sustainable development need to be aimed for. Therefore, a comprehensive assessment of the ecological risk of the rapidly developing nanomaterials is needed. This perspective analyzes and discusses the full life cycle, ecotoxicity, and assessment methods and provides recommendations for the sustainable development of palladium-based nanomaterials and other similar or related nanomaterials.
|
Introduction
In recent decades, nanoscience has made major breakthroughs and has greatly improved the human life.1–3 As an important achievement of nanoscience, nanomaterials are produced and used in many technologies and consumer products owing to their inimitable properties.4–6 Among these, metal-based nanomaterials (M-NMs) have attracted widespread attention owing to their widespread application prospects.7 In particular, noble M-NMs are widely used in the fields of catalysis,8 environmental remediation,9,10 sensors,11,12 nanomedicine,13,14 and so on.15–17 However, it is challenging to ensure sustainability from the synthesis of products and to their applications and disposal.18–20
Palladium-based nanomaterials (Pd-NMs) are one of the most widely used noble M-NMs. Pd-NMs offer opportunities as efficient catalyst materials due to their high specific surface area, abundant active sites, and high catalytic activity.21–23 Trends in publishing activities highlight the continuous development of Pd-NMs and related materials. Pd-NMs have valuable catalytic and optical properties, which provide extensive opportunities for their chemical, medical, and environmental applications in human activities.23–25 However, growth in the use of Pd-NMs has been accompanied by their increasing exposure to the ecological environment. In contrast, toxicology research of Pd-NMs and the related materials is far behind their applications, but such a toxicological study would have a positive significance for their sustainable development and to ensure environmental protection from their use.
With the rapid development of Pd-NMs and related and/or similar materials, a variety of different voices have emerged in the public. On the one hand, Pd-NMs are favored by engineers in the fields of material, chemical, and energy science because of their excellent characteristics (Fig. 1).24,26,27 On the other hand, with the extensive application of Pd-NMs, the increasing interaction between Pd-NMs and the ecological environment makes individuals in the field of environmental ecology and biomedicine worry that Pd-NMs may violate the principle of sustainable development and may cause ecological harm (Fig. 1).28,29 To better protect the ecological environment, we need to control the usage amount of Pd-NMs and improve the techniques and methods used for assessing their toxicity.
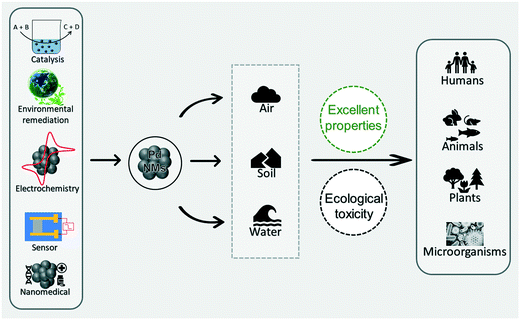 |
| Fig. 1 Diagram summary of the applications, environmental behavior, and ecological risk of Pd-NMs. | |
In 2015, Chen and Ostrom introduced in detail various synthesis methods of Pd-NMs, as well as discussing their outstanding properties and wide application range.24 Pd-NMs have unique chemical and catalytic properties, and their synthesis and application are expected to see remarkable growth over the next decade. Especially in the biomedical field, the need for real-time care and monitoring will accelerate the emergence of these materials.30–32 Pd-NMs that are released into the environment or in contact with organisms initially exist as nanoparticles (NPs), which may subsequently migrate, transform, and accumulate in the environment or in organisms, ultimately causing harm to the organisms. Existing data lack a full assessment of the biological activity of Pd-NMs, and toxicity is often neglected by folks in the design of new nanomaterials, where the outstanding properties are regarded as the only selling point of a new material system. Consequently, Egorova and Ananikov called for specific measurements of special metal catalysts, including Pd-NMs, and for their nature, toxicity, bioavailability, and possible exposure pathways to be taken into account in the development and application of these chemicals.33
Meanwhile, Leso and Iavicoli critically analyzed data from the existing literature on the toxicological and occupational risk assessment of Pd-NMs, and pointed out the negative effects of these chemicals on the ecosystem function for use in determining appropriate strategies to assess and manage the occupational risk related to these materials.34 For gaining a more comprehensive understanding of the toxicology of Pd-NMs and to provide guidance for their sustainable development, the current perspective summarizes and analyzes the risks of Pd-NMs on the environment, and discusses the in vitro and in vivo research problems related to Pd-NMs. This perspective aim to describe the ecotoxicological effects of Pd-NMs and proposes that individuals should consider the potential environmental hazards of these nanomaterials while at the same time promoting their use. The insights of this perspective apply not only to Pd-NMs but also to other similar and related M-NMs. Their excellent properties or toxicity cannot be used as the sole reason for promoting new nanomaterials or hindering their development without a proper comprehensive and dedicated evaluation.
Environmental impact of palladium-based nanomaterials
Exploration of the scalable production routes
Here, the question to ask is, “are there multiple synthesis routes and are some more sustainable that the others?” There are multiple routes to prepare Pd-NMs (such as nanofilms, nanowires, nanocrystals, nanotubes, nanospheres, nanorods, etc.), including physical synthesis methods (such as sputtering, ion or electron beam deposition, and laser ablation),35–37 hydrothermal methods (one-step synthesis),38 electrochemical deposition,39 chemical deposition,40 and other methods (such as microemulsion41 and photochemically42 assisted synthesis). The important environmental impact of these synthetic methods include the need to use toxic reaction reagents and the production of toxic by-products.
Compared to other reaction reagents, polyols have unparalleled advantages.43 First, alcohols have multifunctional properties, such as being able to act as a reducing agent, solvent, and stabilizer for metal precursors. Second, polyols have inherent hydrogen bond interaction and an adjustable number of –OH groups, which gives them an elastic structure. Meanwhile, due to the existence of hydrogen bond supramolecular structures, polyols can prevent the agglomeration of NPs.44 Third, the physicochemical properties of different polyols are different, which facilitate them to meet the different synthetic conditions for the synthesis of Pd-NMs with different properties. To illustrate, Pd-NMs synthesized by a rapid reduction of ethylene glycol (EG) at a relatively high temperature usually have a cubic or rod-like single-crystal structure.45 However, the use of other polyols and/or changing reaction conditions can affect the growth rates of different surfaces of Pd-NMs, thus affecting their shape.46 Finally, noteworthily, polyols derived from environmentally friendly plant extracts are also outstanding substrates for the synthesis of Pd-NMs.47–49
To minimize secondary contamination in the preparation of Pd-NMs, the synthesis of Pd-NMs or other related novel nanomaterials in green media (such as plant extracts)50–52 is beneficial for the sustainable development of nanomaterials. Green chemical substances in plant extract can be used as a reducing agent or stabilizer in the preparation process of Pd-NMs, which can simply and quickly reduce Pd metal ions to zero-valent Pd metal without agglomeration.53–55 Noteworthily, these phytochemicals can not only greatly reduce the adverse environmental effects of the synthesis process, but can also ensure high reaction rates and controllable yields.52,56 The actual method is based on the ability of phytochemical molecules to absorb, accumulate, transform, and recycle metal ions, which demonstrates the characteristics of economy, sustainability, and environmental protection.
In 2008, Varma et al., one of the pioneers in this field, reported the green synthesis of a large number of Pd-NMs at room temperature using coffee and tea extracts.57 The main components of these extracts were caffeine and polyphenols, which could form complexes with metal ions in solution and acted as reducing agents to reduce metal precursors to metals. Meanwhile, they could also be used as dispersants in the synthesis process to avoid the accumulation of metal NPs (MNPs). The synthesized Pd-NMs were mostly spherical in shape, but varied in size, depending on the quality and source of the extract (Fig. 2). Subsequently, Li et al. and Philip et al. extracted polyols from Cinnamomum camphora leaf58 and dried leaf powder of Anacardium occidentale,59 respectively, for the synthesis of Pd-NMs. They found the polyol component binds to the metal complex and is used to reduce the metal precursors, while the heterocyclic component stabilizes the reduced MNPs. Furthermore, they found the size of Pd NPs could be controlled by changing the concentration of Pd ions in the solution without the need for additional templates. Besides, Cacumen platycladi leaf extract,60Pulicaria glutinosa extract,61 fruit and Aloe vera juices,62 artichoke leaf extract,63Catharanthus roseus leaf extract,64 and Terminalia chebula aqueous extract65 have also been reported as reaction media for the simple and green synthesis of Pd-NMs.
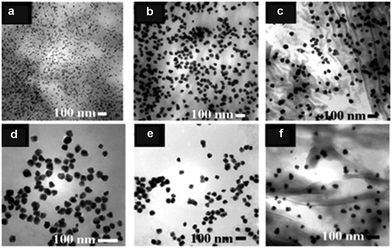 |
| Fig. 2 TEM image of Pd NPs synthesized using: (a) Sanka coffee, (b) Bigelow tea, (c) Luzianne tea, (d) Starbucks coffee, (e) Folgers coffee, and (f) Lipton tea extract at room temperature in one step without using any hazardous reducing chemicals or non-degradable capping agents. Reprinted with permission from ref. 57. Copyright 2008 the Royal Society of Chemistry. | |
Release and transformation during use
Pd-NMs are characterized by their invaluable catalytic, mechanical, and optical properties, which may offer the opportunity for their application in human activities (Fig. 3), including electrochemical reactions,66,67 nanomedicine,68,69 fine chemistry,70,71 sensors,72,73 and especially in the treatment of environmental pollutants, such as to control harmful exhaust emissions of automobiles, eliminate indoor air pollutants, and treat wastewater.74–78 The environmental concentration of Pd-NMs has prominently increased from these human activities. To illustrate, for catalytic applications, all types of Pd-based catalytic systems, even immobilized catalysts (including homogeneous, heterogeneous, metal complexes, and supported Pd-based catalysts), have been demonstrated to suffer from inevitable leaching.23,79,80 Leaching can cause metallic substances and NPs to be released directly or indirectly into the environment. When the diffused Pd-NMs come into contact with organic or inorganic ligands and water components, chemical reactions occur on the surface of Pd-NMs, resulting in morphological changes and the formation of core–shell Pd-NMs,81 which further promotes environmental migration of the Pd-NMs.
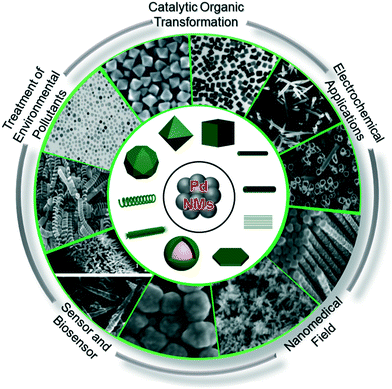 |
| Fig. 3 The source composition of Pd-NMs and the electrochemical applications, catalytic organic transformation, the treatment of environmental contaminants, and use in sensors and nanomedicine. | |
Especially from the transportation sources, with the increase of car use in densely populated areas, an increase in the environmental concentration of Pd-NMs has been well documented.82–84 Here, Pd-NMs used in automotive catalytic converters are discharged as particles in the exhaust gas and accumulate in the local soil.85–87 Subsequently, through various chemical processes (such as redox reactions and complexation reactions), their environmental mobility is enhanced, eventually leading to their interactions with a variety of organisms.88,89 Pd-NMs that enter an organism diffuse across cell membranes, and may end up in various organs or throughout the body through lymph and blood circulation.90,91
Accumulation and deposition of the nanomaterials cause damage to aquatic organisms in water environments.92,93 Changes in the surface chemistry of NPs and the chemical properties of the aquatic environment can change the degree of aggregation and deposition of the nanomaterials.94,95 Furthermore, combined effects of the environment, such as acid rain, could increase the solubility of Pd-NMs and spread them across the ecosystem through runoff or atmospheric transport.96 In summary, Pd-NMs in the environment exhibit toxicity to plants, animals, and microorganisms (Fig. 4), and the environmental behavior of Pd-NMs could aggravate their ecological risk.97
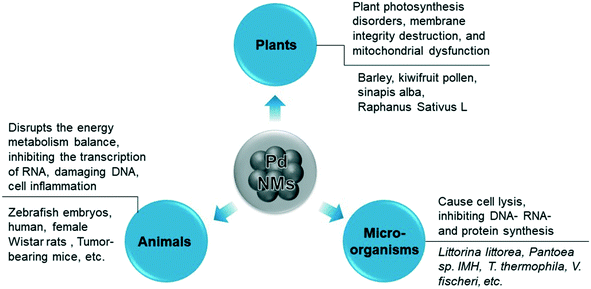 |
| Fig. 4 The toxicity effects of Pd-NMs to plants, animals, and microorganisms. | |
Bioavailability and toxicity in organisms
Acute and chronic toxicity to animals
Pd-NMs have the highest bioavailability among platinum group metals and greater fluidity than other platinum group metals.98,99 Pd-NMs have been proved to be enriched in living organisms and their retention in the organism depends on how it is administered.28,100 Pd-NMs enter animals mainly by inhalation and injection, and exert cytotoxic and pro-inflammatory effects in vitro while they affected different target organs in an animal model test. The mechanisms of the toxicity of Pd-NMs in animal models are mainly related to the toxicity of released Pd ions and the animal's oxygen stress response, which disrupts the energy metabolism balance, inhibiting the transcription of RNA, damaging DNA, and cell inflammation (Fig. 4). For example, Pd2+ ions may be slowly absorbed by animal cells and distributed in the nucleus and mitochondria and can affect and inhibit enzyme systems in animal cells.101
Pd-NMs in contact with animals in the environment could enter the organism and be transformed. Pd initially is released as metal and oxide particles, but they can then be transformed in the environment, in the digestive tract, or in the cell compartment to produce more harmful soluble substances.102 The main factors that determine the toxicity of Pd NPs in the air are related to the particle size and chemical composition, while inhalation routes are considered to pose a greater risk for health effects.98 The metal forms of Pd-NMs that are drawn into the respiratory tract from the air are usually biologically inert, but some of the metal salts can become sensitized chlorine compounds, which are strong allergens and sensitizers.103
Pd-NMs have also displayed specific cross-sensitization with nickel and can penetrate through the skin.29 As the surface-to-mass ratio of Pd-NMs increases, their biological activity increases, which in turn releases more active metal ions, ultimately leading to an increased likelihood of them penetrating the skin.29 Noteworthily, compared with intact skin, damaged skin significantly increases the absorption of Pd-NMs (Fig. 5).29 Pd content in the whole skin layer from the epidermis to dermis was found to be significantly decreased. Within the skin, these nanomaterials may have a long-term role and may be involved in sensitization or may be spread throughout the body.
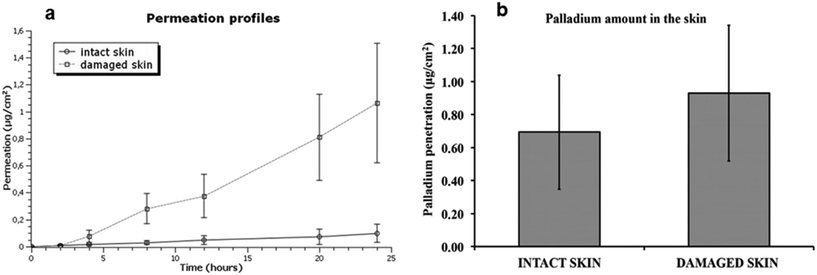 |
| Fig. 5 (a) Permeation profile of Pd after the skin application of Pd NPs solution. (b) Mean values and standard deviations of Pd amounts (mg cm−2) in intact and damaged skin. Reprinted with permission from ref. 29. Copyright 2016 Elsevier Science Ltd. | |
Significant cytotoxic effects of Pd-NMs have been observed in several human cell models, including respiratory cells,87,104 cervical,105 and liver.106 Pd-NMs released into the environment can be inhaled by humans and can accumulate in the respiratory tract, and the absorption of Pd through the digestive tract has been shown to be insignificant.107 Meanwhile, human exposure to Pd-NMs may cause strong sensitization reactions.108,109 Furthermore, Pd-NMs that enter animals may accumulate in the liver, kidneys, lungs, and bones.30,110 However, serum biochemical evaluation110 showed no significant hepatotoxicity in mice after 7 days of intravenous injection of various Pd-NMs (Fig. 6a). Moreover, no significant damage from Pd-NMs to the organs of mice within 28 days was observed in H&E stained images (Fig. 6b). Meanwhile and noteworthily, the toxicity of Pd-NMs is affected by multiple factors, among which the dose and time are the two main factors. Therefore, a systematic study of the ecotoxicity of Pd-NMs at different doses within different time periods (focusing on the long-term potential toxicity) is still required.
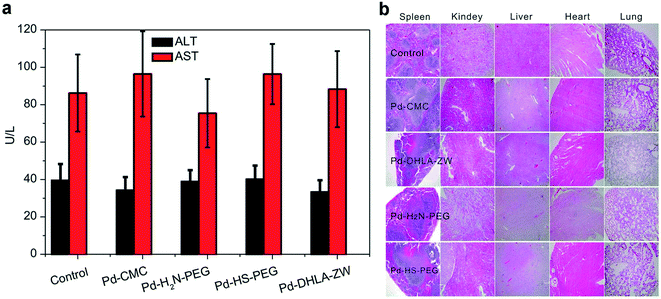 |
| Fig. 6 (a) Serum biochemistry analysis of mice treated with different coated Pd NSs at 7 days post injection. (b) Photos of H&E stained diaphragm slices from the mice treated with different coated Pd NSs at 28 days post injection. Reprinted with permission from ref. 110. Copyright 2015 American Chemical Society. | |
Soluble Pd-NMs have been found to have serious health effects on higher vertebrates in acute and chronic studies.111 Soluble Pd-NMs can enter the mouse, rat, and rabbit body and can cause mitochondrial membrane potential disorder, arrhythmia, organ dysfunction, and even death.112,113 Furthermore, the time of retention of Pd in the animals could affect the level of toxicity. Intratracheal or intravenous administration can lead to a prolonged retention time of Pd in animals more than in the oral administration of PdCl2, resulting in a higher biological toxicity. Pd-NMs accumulated in living organisms may also be expelled from living organisms, and the main way for these MNPs to be eliminated is through urine and feces.101 Several studies have shown that soluble Pd-NMs may also cause patient poisoning, such as by accumulating in organ tissues, especially in the kidneys, lungs, liver, spleen, bones, and heart.114 Meanwhile, the mitochondria are important subcellular organelles for Pd-NMs toxicity.112 Studies have shown that Pd-NMs toxicity is caused by the breakdown of the mitochondrial membrane potential and the depletion of cellular glutathione (GSH) levels,115 which proposes that Pd-NMs are more susceptible to toxicity to kidney tissue than liver tissue. Histopathological findings in the kidney indicate that they change renal tubular epithelial, which further affects the glomerular filtration function.116
Furthermore, Pd-NMs can inhibit DNA and protein synthesis, and were found to damage different types of DNA in mouse lymphoma cell lines.112 Pd-NMs can also inhibit the gene expression of multiple metal markers and induce the conformational changes and cleavage of DNA.117 Meanwhile, Pd-NMs interfere with the inflammatory process by increasing the adhesion of eosinophils on endothelial cells at low concentrations.118 More research is needed to further study the cellular and molecular mechanisms to help understand the process by which Pd-NMs induce allergies and inflammation.
To further understand the toxicity of Pd-NMs, the toxicity of Pd-NMs during embryonic development were investigated. Zebrafish have a high genetic similarity with humans. Zebrafish embryos have high optical clarity and can be screened on a large scale, and have a low operating cost, which make them powerful tools for environmental toxicity detection.119 The molecular mechanism of Pd-NMs inducing embryonic morphological changes was investigated by using zebrafish as a model. The results propose that Pd treatment resulted in zebrafish embryo experiencing pericardial edema, inhibiting embryo survival and hatchability and resulting in embryonic pericardial edema and cardiac malformation (Fig. 7), which affected the expression levels of several cardiac-related genes and antioxidant enzymes, thereby revealing the underlying molecular mechanism of how Pd-NMs induce zebrafish embryonic heart malformations.100
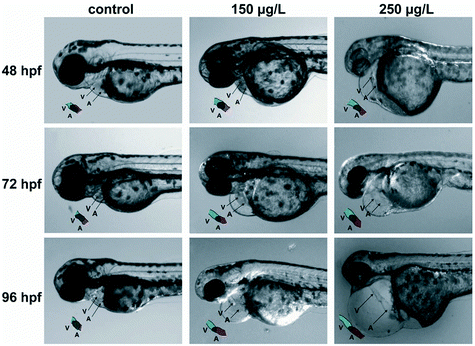 |
| Fig. 7 Pd caused pericardial edema and cardiac malformation in zebrafish embryos. Reprinted with permission from ref. 100. Copyright 2014 Elsevier Science Ltd. | |
In many cases, technical problems have prevented zebrafish model platforms from being fully established. First, zebrafish farming techniques are highly demanding, and changes in simple parameters, such as temperature, pH, and symbiotic microbes, can confuse the test results.119 Second, so far, zebrafish models have focused on a limited range of environmental chemicals that are toxic at an early life stage.120,121 Therefore, powerful new phenotypic techniques and systematic approaches to identify a wider range of chemicals are needed to investigate the toxic effects of a large number of environmental factors on zebrafish models throughout their life stages. Finally, if we want to maximize the use of zebrafish models to describe the toxicological reactions of higher-level organisms, a more detailed understanding of the similarities between zebrafish and higher-level organisms is needed, including a combination of multichannel organ-specific studies, functional genomics, and automated image analysis techniques.119 In summary, the zebrafish model as an explanatory tool in the field of toxicology is promising for the systematic exploration of nanomaterial–environment interactions. Also conceivably, with the establishment of a fully and robust zebrafish model platform, toxicity prediction and the development of new materials will eventually be synchronized.
Evaluation of phytotoxicity
There is a need to understand w the hazards to plants are of Pd-NMs entering the environment. Rare Pd-NMs have been extensively redistributed in the biosphere as a result of human activity. Lethal toxicity and cytotoxicity of Pd-NMs in terrestrial plants are developing rapidly. There is a serious lack of public awareness of the environmental hazards caused by the widespread use of trace nano-metal pollutants. In a natural state, plants have less contact with rare metals and fewer resistance mechanisms than those found in some organisms.122 Therefore, to promote the application of these materials, it is necessary to investigate the harm they cause in plants.
For understanding the ecological toxicological effects of Pd-NMs on plants, it was found that Pd-NMs can quickly enter the kiwifruit plant in large quantities, change the shape of pollen, and cause a rapid loss of endogenous calcium in pollen grains, which would result in pollen plasma membrane damage.123,124 Moreover, Pd-NMs affect plant growth,125 and seeds germination,126 but no effect of Pd NPs on plant growth was observed within 15 days of plant culture, suggesting that NPs may not directly affect plant growth but may do indirectly.126 Further research is needed to understand whether environmental soil exposure to rare Pd NPs has a short-term or long-term impact on crop production, as well as the specific toxicity mechanisms. In this regard, pollens are highly sensitive to environmental pollutants, which would be helpful for the accurate detection of the effects of Pd-NMs on biological systems.
Taking kiwi pollen as an example, studies have found that Pd-NMs enter the kiwi pollen grains faster and more than just soluble Pd(II) enters (Fig. 8a and b).123 Compared with the effect of soluble Pd(II), under the granular action of a low concentration of Pd, the endogenous calcium of kiwifruit pollen is rapidly lost (Fig. 8c and d), thereby causing damage to the pollen plasma membrane, which seriously inhibits the growth of pollen tubes. The toxicity of Pd-NMs to plants is largely caused by the reactive oxygen species (ROS) production,122 which can result in plant photosynthesis disorders, membrane integrity destruction, and mitochondrial dysfunction by attacking the interactions of cell membranes, proteins, lipids, and DNA in cells (Fig. 4).
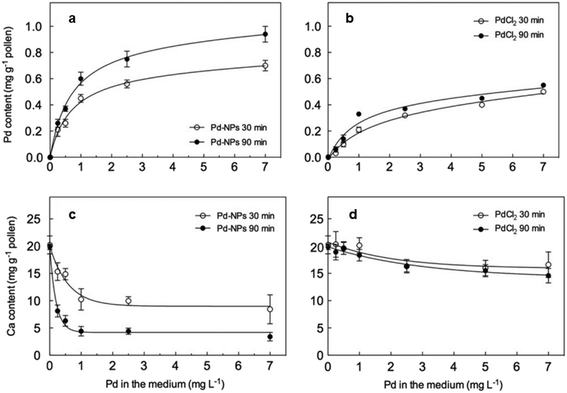 |
| Fig. 8 Pd (a and b) and Ca (c and d) content in controls and in pollen treated for 30 and 90 min with increasing Pd concentrations administered as either Pd NPs (a and c) or PdCl2 (b and d). Reprinted with permission from ref. 123. Copyright 2009 Elsevier Science Ltd. | |
Damage to microorganisms
Microorganisms complete the cycle of various trace elements and thus are often the common target of environmental toxicology research.127–129 The toxicology of Pd catalysts was explored using the marine bacteria V. fischeri as a model.130V. fischeri were cultured by designing a microenvironment that simulated the actual sampling location. During the culture process, the effects of different concentrations of Pd-NMs on respiratory metabolism were reported, and no side effects were found (Fig. 9).130 Meanwhile, the effect of Pd-NMs on the structure of a polychlorinated biphenyl (PCB)-dechlorinated microbial community, which was enriched from marine sediment, has also been reported.130 Investigations found that the Pd-NMs had no permanent impact on their community organization, and even, on the contrary, increased the biodiversity of microbial communities. All in all, the results of this work counter the hypothesis that Pd-NMs affect marine microbial communities. This was the first comprehensive study of the effects of Pd-NMs on marine microbial communities and provided significant information for the assessment of the toxicity of Pd-NMs.
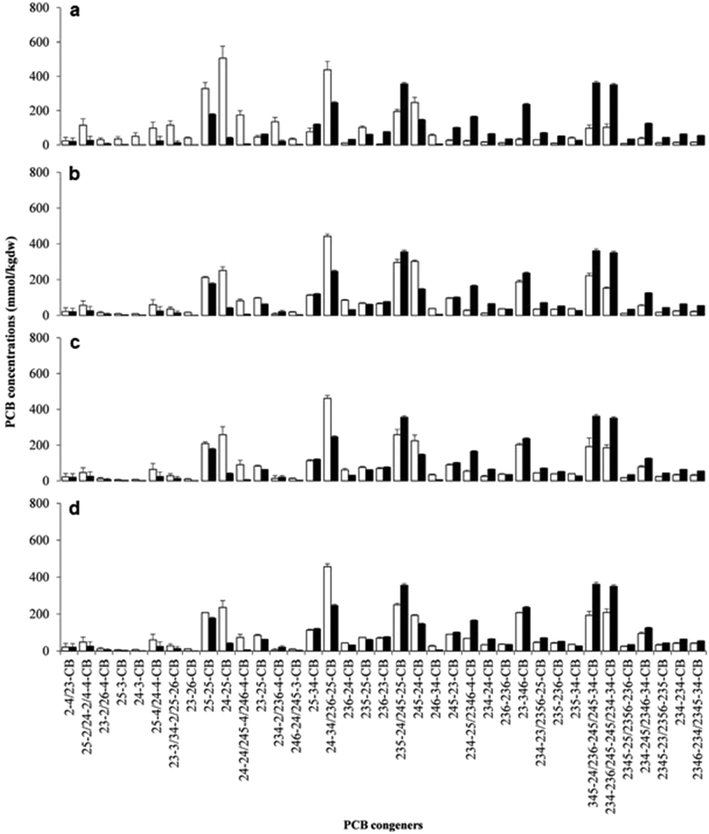 |
| Fig. 9 Concentrations of spiked PCB congeners and their dechlorination products constituting more than 1% w/w of total PCBs at the end of incubation in biologically active (white bars) and sterile (black bars) sets of spiked microcosms. (a) Unamended microcosms; (b) hydrogen-amended microcosms; (c) microcosms amended with hydrogen + bio-Pd 5 mg kg−1 dw; (d) microcosms amended with hydrogen + bio-Pd 50 mg kg−1 dw. Values are the means of triplicate microcosms with error bars representing the standard deviation. Reprinted with permission from ref. 130. Copyright 2016 Elsevier Science Ltd. | |
In the other example, the results showed that Pd-NMs cause genomic alterations in the freshwater green algae Pseudokirchneriella subcapitata,131 which in turn caused great damage to the Pseudokirchneriella subcapitata by affecting the growth process and morphology. Compared with animals and plants, the mechanism of the toxicity of Pd-NMs to microorganisms is mainly through the microbial community structure, growth, and diversity. The main mechanism of Pd-NMs to affect microorganisms is still through oxidative stress. Exposure to Pd-NMs in the environment produces ROS directly or indirectly, which then interact with membrane proteins and bacterial cell walls, and can cause cell lysis, inhibiting DNA-, RNA- and protein synthesis (Fig. 4).
Toxicity assessment methods and challenges
As described in the previous sections of this article, the toxicity of Pd-NMs to the ecosystem (cytotoxicity, genotoxicity, inflammatory response, oxidative stress response, and so on) has been demonstrated. Parallel studies and toxicity assessments of these nanomaterials are still needed before promoting their production and wide-scale application. However, the toxicity of Pd-NMs and/or other related nanomaterials and their interaction with ecosystems have not yet been systematically studied.132–134 Currently, most methods of toxicity assessment have been developed based on chemical toxicity. Due to the unique properties of Pd-NMs, the results of these assessment methods may be disturbed.106,135 Compared with traditional materials, the size, shape, specific surface area, doping degree, solubility, agglomeration state, crystallinity, and other characteristics of Pd-NMs may affect the characterization results of the biological effects.136–138 Incomplete and inaccurate characterization could lead to incorrect assessment results. Besides, the non-development of unified and standardized assessment methods is not conducive to the comparison of the toxicity assessment results among different research groups.
The detection of Pd dispersed in the environment requires a sophisticated analytical method. Many techniques for detecting Pd in environmental samples have been developed.139,140 To improve the sensitivity, it is feasible to combine the existing detection technology, such as gas chromatography-mass spectrometry (GC-MS) analysis.141 Compared to conventional solid-phase extraction methods, this newly developed strategy can achieve a higher sensitivity by increasing the concentration of the maximum allowable coexisting heavy metal ions.
From the perspective of the toxicity test, in vitro toxicity testing is the primary method to study the ecotoxicity of Pd-NMs, because it is faster, easier, and does not pose ethical problems. However, toxicity studies of cultured cell systems in vitro do not clearly explain cell–cell and cell–stromal interactions, ignoring cell diversity, and lacking a consideration of the hormonal effects in vivo. Moreover, with in vitro experiments, it can be difficult to reflect the actual effect of the Pd-NMs in vivo.142 Therefore, toxicity assessment also requires in vivo experiments, and in vivo experiments can also be used to study the long-term toxicity of Pd-NMs. Furthermore, pharmacokinetic studies help to maximize the interpretation of the correspondence between compounds in in vivo and in vitro investigations.143–145 The pharmacokinetics of the Pd-NMs are helpful for a comprehensive quantitative analysis of the target tissues or cells acted on by the materials, the residence time in vivo, the toxicity time, and the dose, but before starting the pharmacokinetic study, a simulation should be performed of the Pd-NMs effect on organisms.
In terms of microbiological inhibition, the toxicological evaluation results of Pd-NMs are different under different experimental conditions and in different microbial communities. For example, Pd-NMs showed a strong growth inhibition effect on bacteria in the culture of a single microorganism.146,147 However, in the simulated native microbial environment, as in the soil microbial system and the marine microbial system, the toxicity inhibition of Pd-NMs on the microbial community can be ignored.126,130 This indicates that toxicity evaluation is multi-directional and environmental media can protect a microbial community and prevent the toxicity of Pd-NMs to the microbial community. Therefore, in addition to a single toxicity test, microbial metabolism and microbial community composition should also be assessed in the toxicological investigation of Pd-NMs on the actual microbial community, so as to understand the toxic effects of Pd-NMs on the microbial community from the perspective of molecular mechanisms.
Conclusions and perspectives
The widespread applications of Pd-NMs lead to their close contact with the ecological environment and their diffusion into the atmosphere, soil, water, and sediments. Subsequently, they migrate and transform in the environment and/or in the organism, and eventually cause harm to the organism. Much progress has been made in the investigation of Pd-NMs release, absorption, transport, and risk assessment in animal models, contaminated soil–plant systems, and microbial communities (Table 1).21,28,29,87,100,103,110,116,123,125,130,148–158 However, research progress in the toxicology of Pd-NMs lags far behind their production rate, and also the lack of uniform criteria and guidelines for evaluating research projects and outcomes in this field. Also, the unique nature of Pd-NMs as new materials has led to many problems in evaluating the toxicology of these Pd-NMs. All these problems could lead to conflicts and hinder the development of emerging nanomaterials.
Table 1 The toxicity of Pd-NMs to organisms
Pd-NMs |
Investigated model |
Findings |
Ref. |
NPs: nanoparticles; HNT: halloysite nanotube; SP-ICP-MS: single particle inductively coupled plasma mass spectrometry; HNMs: hybrid NMs; NPs: nanoparticles; NSs: nanosheets; IL-8: interleukin-8; PGE2: prostaglandin E2.; ISO 11348-3: International Organization for Standardization; ROS: reactive oxygen species; DCFH2-DA: 2′,7′ dichlorodihydro-fluoresceindiacetate.
|
Toxicity to animals |
Pd(OH)2 |
Simulated lung fluids |
Formed in the respiratory tract, and were toxic and allergic to humans and other organisms |
103
|
PdCl2 solution |
Zebrafish embryos |
Inhibited the survival rate and hatchability; leading to pericardial edema and cardiac malformation; inhibited the heartbeat rate; induced the maladjustment of stress-related genes |
100
|
Pd/magnetite HNMs |
Human skin (HaCaT) cell lines; human colon (CaCo-2) cell lines; rainbow trout gills (RTgill-W1) cell line |
Did not trigger the production of ROS; did not affect the viability of selected mammalian and fish cell lines |
28
|
ZnO/Pd HNMs |
Human skin cells |
ZnO/Pd NPs were more photocytotoxic than ZnO NPs on the viability of human skin fibroblasts |
148
|
PdO/Co3O4 HNMs |
BEAS-2B cells; RAW 264.7 cells; mouse lung |
Superoxide production, glutathione depletion, cytokine production, and hierarchical cellular responses involving cytotoxicity in epithelial and macrophage lines; acute pro-inflammatory effects for mouse lung |
149
|
Pd NPs |
Human primary bronchial epithelial cells (PBEC); human alveolar carcinoma cell line (A549) |
Absorbed by cells in PBEC; reduce the response of PBEC to the pro-inflammatory cytokine TNF-R |
87
|
Pd NPs |
Female Wistar rats |
Caused significant tubular dysfunction of female Wistar rats; significantly altered the epithelial cells of proximal and distal renal tubules with varying degrees of severity |
116
|
Pd NPs |
Intact and damaged human skin in Franz cell |
Permeated the skin in an in vitro system; a potential long-term effect inside the skin |
29
|
Pd NPs |
Human ovarian cancer cells (SKOV3) |
Caused a decrease in cell activity and proliferation ability; caused the increase of cytotoxicity when the concentration increased; induced SKOV3 cell apoptosis by inducing mitochondrial dysfunction |
150
|
Pd NSs |
Tumor-bearing mice |
No obvious hepatic toxicity by blood biochemistry assay; no detectable organ (spleen, kidney, liver, heart, and lung) damage by hematoxylin and eosin-stained imaging |
110
|
Pd NSs |
Female Balb/c mice |
Slight lipid accumulation in the liver; led to spleen inflammation |
158
|
Pd NSs |
ICR mice |
Accumulated in the liver, spleen, tumor, and kidney; cleared faster in the oral administration group |
151
|
Phytotoxicity |
Pd element |
Barley |
Caused stress to the leaves at a low nutrient concentration |
125
|
Pd NPs |
Kiwifruit pollen |
Changed the morphology of kiwifruit pollen; led to the rapid loss of endogenous calcium in pollen, resulting in the damage of the pollen plasma membrane |
123
|
Pd NPs |
Sinapis alba
|
The largest amount was found in leaves, followed by stems and roots |
21
|
HNT-Pd |
Raphanus sativus L. |
Increased the number of aberrations in low-vigor seeds |
152
|
Damage to microorganisms |
Pd solution |
Littorina littorea
|
Diet is the most important carrier of Pd accumulation for Littorina littorea |
153
|
Pd(II) |
Pantoea sp. IMH |
Induced the expression of anti-stress protein; induced detoxification of glutathione |
154
|
Pd NPs |
T. thermophila
|
Accumulated extensively in the food vacuoles of T. thermophila |
155
|
Pd NPs |
V. fischeri
|
Increased the biodiversity of V. fischeri; no adverse effect on the overall structure of V. fischeri |
130
|
Pd NPs |
Candida albicans ATCC10231; Aspergillus niger |
Caused cell wall damage and oxidative stress |
156
|
Pd element |
Caenorhabditis elegans
|
Affected the growth ability of nematodes and had an effect on their reproductive ability |
157
|
These Pd-NMs may affect the ecosystem function, exert cytotoxic and pro-inflammatory effects in vitro, and induce early changes in different target organs in in vivo model tests. Further studies are needed to more comprehensively and deeply characterize the physicochemical properties of Pd-NMs to explain in detail the complex interaction between their intrinsic characteristics and their toxic effects. Also, the nano-size of Pd-NMs makes it difficult to track them in the environment, so it is necessary to exploit a more accurate method to detect trace amounts of Pd-NMs in the environment. These problems should be analyzed by a systematic approach that involves the use of new high-precision equipment or new technologies and the characterization of Pd-NMs by multi-technique joint analysis.
Most in vitro studies have reported that Pd-NMs induce severe cytotoxic effects and dysfunction in different animal and human cells (Table 1). In vitro studies are helpful to understand the toxic molecular mechanism of Pd-NMs. However, current data on the toxicity of Pd-NMs in vitro are insufficient to reach a unified conclusion on the mechanisms, such as oxidative stress response, apoptotic pathways, cell cycle disorders, and DNA damage. In addition, the physicochemical properties, organic ligands, and surfactant interference with the toxicological properties of the Pd-NMs should also be considered. Therefore, it is necessary to further investigate the properties of Pd-NMs and the effects of their complex interactions between cell growth mediators on different toxic patterns. As an example, differences in particle intake and culture conditions in cell models simulating upper and lower respiratory tract environments can interfere with the toxicological characteristics of Pd-NMs.87 Faced with this situation, it is necessary to establish more complex in vitro models to obtain more comprehensive data and to help infer actual in vivo pathological characteristics.
In vivo experiments have shown that exposure to palladium-based nanomaterials affects multiple organ systems, including the endocrine system and kidney system (Table 1). However, the toxicokinetic behavior, and changes in short-term biological indicators (cytokines, hormone serum concentrations, and urine protein content, etc.) reported in these reports, remains to be explained as well as whether they are stable at low doses and under long-term exposure conditions. From this perspective, Pd-NMs have a long-term and complex interaction with biological systems in the in vivo environment. Meanwhile, the toxicological characteristics of Pd-NMs may be related to the exposure patterns, differences in individual immune systems, and concentrations in biological media. Future investigations should focus on the toxicological effects of Pd-NMs on animals under low-dose, long-term exposure conditions (in practice, some workers may be exposed to low-dose Pd-NMs for a long period of time).
Many studies have focused on the biological hazards of nanoscale risk assessment, but few have studied the current and future concentrations and distribution of Pd-NMs in the environment. This requires a multidisciplinary team effort involving experts in materials, molecular biology, environmental toxicology, and physical chemistry. Also, innovative experimental methods and protocols are needed to guide the assessment of the full-cycle ecotoxicity of Pd-NMs. Furthermore, quantitative measurement of the concentration level of Pd-NMs in the real environment is strongly encouraged. Considering the lack of standardized testing procedures, the diversity of exposure pathways, and the differences in toxicity tolerance among individuals, there are still many difficulties in the environmental monitoring of Pd-NMs in the actual environment. Therefore, biological monitoring can be carried out at the same time, focusing on the investigation of biomarkers that mark physiological toxicity exposed in the real environment.
In summary, effectively defining the risk from the release of Pd-NMs, and the use of multidisciplinary methods to provide guidance are beneficial. Both would help to protect the health and safety of the ecological environment, and would be conducive to clarify the toxicity of Pd-NMs, thus contributing to paving the way for the development and application of sustainable nanomaterials.
Conflicts of interest
The authors declare no conflicts of interest.
Acknowledgements
The National Natural Science Foundation of China (51521006, 51679085, 51039001, 51378190, 51979103, 51909085, 51108166), the Fundamental Research Funds for the Central Universities of China (531107050930), the Key Research and Development Project of Hunan Province of China (2017SK2240).
References
- R. Jin, C. Zeng, M. Zhou and Y. Chen, Atomically precise colloidal metal nanoclusters and nanoparticles: Fundamentals and opportunities, Chem. Rev., 2016, 116, 10346–10413 CrossRef CAS.
- J.-M. Nam, J.-W. Oh, H. Lee and Y. D. Suh, Plasmonic nanogap-enhanced raman scattering with nanoparticles, Acc. Chem. Res., 2016, 49, 2746–2755 CrossRef CAS.
- D. Wang and D. Astruc, The recent development of efficient Earth-abundant transition-metal nanocatalysts, Chem. Soc. Rev., 2017, 46, 816–854 RSC.
- R. S. Selinsky, Q. Ding, M. S. Faber, J. C. Wright and S. Jin, Quantum dot nanoscale heterostructures for solar energy conversion, Chem. Soc. Rev., 2013, 42, 2963–2985 RSC.
- A. Schneemann, J. L. White, S. Kang, S. Jeong, L. F. Wan, E. S. Cho, T. W. Heo, D. Prendergast, J. J. Urban, B. C. Wood, M. D. Allendorf and V. Stavila, Nanostructured metal hydrides for hydrogen storage, Chem. Rev., 2018, 118, 10775–10839 CrossRef CAS.
- C. Forster and K. Heinze, Photophysics and photochemistry with Earth-abundant metals - fundamentals and concepts, Chem. Soc. Rev., 2020, 49, 1057–1070 RSC.
- X. Liu, D. Huang, C. Lai, G. Zeng, L. Qin, H. Wang, H. Yi, B. Li, S. Liu, M. Zhang, R. Deng, Y. Fu, L. Li, W. Xue and S. Chen, Recent advances in covalent organic frameworks (COFs) as a smart sensing material, Chem. Soc. Rev., 2019, 48, 5266–5302 RSC.
- Q. Liang, X. Liu, G. Zeng, Z. Liu, L. Tang, B. Shao, Z. Zeng, W. Zhang, Y. Liu, M. Cheng, W. Tang and S. Gong, Surfactant-assisted synthesis of photocatalysts: Mechanism, synthesis, recent advances and environmental application, Chem. Eng. J., 2019, 372, 429–451 CrossRef CAS.
- Z. Zeng, S. Ye, H. Wu, R. Xiao, G. Zeng, J. Liang, C. Zhang, J. Yu, Y. Fang and B. Song, Research on the sustainable efficacy of g-MoS2 decorated biochar nanocomposites for removing tetracycline hydrochloride from antibiotic-polluted aqueous solution, Sci. Total Environ., 2019, 648, 206–217 CrossRef CAS.
- Y. Liu, M. Cheng, Z. Liu, G. Zeng, H. Zhong, M. Chen, C. Zhou, W. Xiong, B. Shao and B. Song, Heterogeneous Fenton-like catalyst for treatment of rhamnolipid-solubilized hexadecane wastewater, Chemosphere, 2019, 236, 124387 CrossRef CAS.
- C. Zhang, C. Lai, G. Zeng, D. Huang, L. Tang, C. Yang, Y. Zhou, L. Qin and M. Cheng, Nanoporous Au-based chronocoulometric aptasensor for amplified detection of Pb2+ using DNAzyme modified with Au nanoparticles, Biosens. Bioelectron., 2016, 81, 61–67 CrossRef CAS.
- G. Zeng, C. Zhang, D. Huang, C. Lai, L. Tang, Y. Zhou, P. Xu, H. Wang, L. Qin and M. Cheng, Practical and regenerable electrochemical aptasensor based on nanoporous gold and thymine-Hg2+-thymine base pairs for Hg2+ detection, Biosens. Bioelectron., 2017, 90, 542–548 CrossRef CAS.
- M. Chen, X. Qin and G. Zeng, Biodiversity change behind wide applications of nanomaterials?, Nano Today, 2017, 17, 11–13 CrossRef CAS.
- H. Kim, S. Beack, S. Han, M. Shin, T. Lee, Y. Park, K. S. Kim, A. K. Yetisen, S. H. Yun, W. Kwon and S. K. Hahn, Multifunctional photonic nanomaterials for diagnostic, therapeutic, and theranostic applications, Adv. Mater., 2018, 30, 1701460 CrossRef.
- P. Xu, G. M. Zeng, D. L. Huang, C. L. Feng, S. Hu, M. H. Zhao, C. Lai, Z. Wei, C. Huang, G. X. Xie and Z. F. Liu, Use of iron oxide nanomaterials in wastewater treatment: A review, Sci. Total Environ., 2012, 424, 1–10 CrossRef CAS.
- W.-W. Tang, G.-M. Zeng, J.-L. Gong, J. Liang, P. Xu, C. Zhang and B.-B. Huang, Impact of humic/fulvic acid on the removal of heavy metals from aqueous solutions using nanomaterials: A review, Sci. Total Environ., 2014, 468, 1014–1027 CrossRef.
- D. Huang, Z. Li, G. Zeng, C. Zhou, W. Xue, X. Gong, X. Yan, S. Chen, W. Wang and M. Cheng, Megamerger in photocatalytic field: 2D g-C3N4 nanosheets serve as support of 0D nanomaterials for improving photocatalytic performance, Appl. Catal., B, 2019, 240, 153–173 CrossRef CAS.
- E. Burello, Computational design of safer nanomaterials, Environ. Sci.: Nano, 2015, 2, 454–462 RSC.
- Q. Zhang, W. Zhou, Y. Cui, C. Lyu, T. Liu, R. Zhang, R. Zhang, J. Zheng, Z. Shi, C. Lu and L. Guo, Iron triad nanomaterials and their sustainable application in the environment, Environ. Sci.: Nano, 2018, 5, 246–256 RSC.
- H. A. Maitlo, K.-H. Kim, V. Kumar, S. Kim and J.-W. Park, Nanomaterials-based treatment options for chromium in aqueous environments, Environ. Int., 2019, 130, 104748 CrossRef CAS.
- K. Kińska, J. Jiménez-Lamana, J. Kowalska, B. Krasnodębska-Ostręga and J. Szpunar, Study of the uptake and bioaccumulation of palladium nanoparticles by Sinapis alba using single particle ICP-MS, Sci. Total Environ., 2018, 615, 1078–1085 CrossRef.
- A. M. Trzeciak and A. W. Augustyniak, The role of palladium nanoparticles in catalytic C–C cross-coupling reactions, Coord. Chem. Rev., 2019, 384, 1–20 CrossRef CAS.
- S. Luo, Z. Zeng, G. Zeng, Z. Liu, R. Xiao, M. Chen, L. Tang, W. Tang, C. Lai, M. Cheng, B. Shao, Q. Liang, H. Wang and D. Jiang, Metal organic frameworks as robust host of palladium nanoparticles in heterogeneous catalysis: Synthesis, application, and prospect, ACS Appl. Mater. Interfaces, 2019, 11, 32579–32598 CrossRef CAS.
- A. Chen and C. Ostrom, Palladium-based nanomaterials: Synthesis and electrochemical applications, Chem. Rev., 2015, 115, 11999–12044 CrossRef CAS.
- Y. Liu, Z. Liu, D. Huang, M. Cheng, G. Zeng, C. Lai, C. Zhang, C. Zhou, W. Wang, D. Jiang, H. Wang and B. Shao, Metal or metal-containing nanoparticle@MOF nanocomposites as a promising type of photocatalyst, Coord. Chem. Rev., 2019, 388, 63–78 CrossRef CAS.
- R. Long, H. Huang, Y. Li, L. Song and Y. Xiong, Palladium-based nanomaterials: A platform to produce reactive oxygen species for catalyzing oxidation reactions, Adv. Mater., 2015, 27, 7025–7042 CrossRef CAS.
- S. K. Konda and A. Chen, Palladium based nanomaterials for enhanced hydrogen spillover and storage, Mater. Today, 2016, 19, 100–108 CrossRef CAS.
- H. Hildebrand, D. Kuehnel, A. Potthoff, K. Mackenzie, A. Springer and K. Schirmer, Evaluating the cytotoxicity of palladium/magnetite nano-catalysts intended for wastewater treatment, Environ. Pollut., 2010, 158, 65–73 CrossRef CAS.
- F. Larese Filon, M. Crosera, M. Mauro, E. Baracchini, M. Bovenzi, T. Montini, P. Fornasiero and G. Adami, Palladium nanoparticles exposure: Evaluation of permeation through damaged and intact human skin, Environ. Pollut., 2016, 214, 497–503 CrossRef CAS.
- M. Chen, S. Tang, Z. Guo, X. Wang, S. Mo, X. Huang, G. Liu and N. Zheng, Core-shell Pd@Au nanoplates as theranostic agents for in-vivo photoacoustic imaging, ct imaging, and photothermal therapy, Adv. Mater., 2014, 26, 8210–8216 CrossRef CAS.
- Z. Zhao, S. Shi, Y. Huang, S. Tang and X. Chen, Simultaneous photodynamic and photothermal therapy using photosensitizer-functionalized Pd nanosheets by single continuous wave laser, ACS Appl. Mater. Interfaces, 2014, 6, 8878–8885 CrossRef CAS.
- X. Huang, S. Tang, X. Mu, Y. Dai, G. Chen, Z. Zhou, F. Ruan, Z. Yang and N. Zheng, Freestanding palladium nanosheets with plasmonic and catalytic properties, Nat. Nanotechnol., 2011, 6, 28–32 CrossRef CAS.
- K. S. Egorova and V. P. Ananikov, Which metals are green for catalysis? Comparison of the toxicities of Ni, Cu, Fe, Pd, Pt, Rh, and Au salts, Angew. Chem., Int. Ed., 2016, 55, 12150–12162 CrossRef CAS.
- V. Leso and I. Iavicoli, Palladium nanoparticles: Toxicological effects and potential implications for occupational risk assessment, Int. J. Mol. Sci., 2018, 19, 503 CrossRef.
- J. A. Spencer, S. G. Rosenberg, M. Barclay, Y.-C. Wu, L. McElwee-White and D. Howard Fairbrother, Understanding the electron-stimulated surface reactions of organometallic complexes to enable design of precursors for electron beam-induced deposition, Appl. Phys. A: Mater. Sci. Process., 2014, 117, 1631–1644 CrossRef CAS.
- M. Favaro, S. Agnoli, L. Perini, C. Durante, A. Gennaro and G. Granozzi, Palladium nanoparticles supported on nitrogen-doped HOPG: a surface science and electrochemical study, Phys. Chem. Chem. Phys., 2013, 15, 2923–2931 RSC.
- Z. J. Li, B. C. Yang, S. R. Zhang and C. M. Zhao, Graphene oxide with improved electrical conductivity for supercapacitor electrodes, Appl. Surf. Sci., 2012, 258, 3726–3731 CrossRef CAS.
- M. Yuan, A. Liu, M. Zhao, W. Dong, T. Zhao, J. Wang and W. Tang, Bimetallic PdCu nanoparticle decorated three-dimensional graphene hydrogel for non-enzymatic amperometric glucose sensor, Sens. Actuators, B, 2014, 190, 707–714 CrossRef CAS.
- A. Renjith and V. Lakshminarayanan, One step preparation of ‘ready to use’ Au@Pd nanoparticle modified surface using deep eutectic solvents and a study of its electrocatalytic properties in methanol oxidation reaction, J. Mater. Chem. A, 2015, 3, 3019–3028 RSC.
- Y. Wang, S.-I. Choi, X. Zhao, S. Xie, H.-C. Peng, M. Chi, C. Z. Huang and Y. Xia, Polyol synthesis of ultrathin pd nanowires via attachment-based growth and their enhanced activity towards formic acid oxidation, Adv. Funct. Mater., 2014, 24, 131–139 CrossRef CAS.
- P. F. Siril, L. Ramos, P. Beaunier, P. Archirel, A. Etcheberry and H. Remita, Synthesis of ultrathin hexagonal palladium nanosheets, Chem. Mater., 2009, 21, 5170–5175 CrossRef CAS.
- A. A. Ismail, D. W. Bahnemann and S. A. Al-Sayari, Synthesis and photocatalytic properties of nanocrystalline Au, Pd and Pt photodeposited onto mesoporous RuO2-TiO2 nanocomposites, Appl. Catal., A, 2012, 431–432, 62–68 CrossRef CAS.
- I. Favier, D. Pla and M. Gómez, Palladium nanoparticles in polyols: Synthesis, catalytic couplings, and hydrogenations, Chem. Rev., 2020, 120, 1146–1183 CrossRef CAS.
- Y.-J. Zhu and F. Chen, Microwave-assisted preparation of inorganic nanostructures in liquid phase, Chem. Rev., 2014, 114, 6462–6555 CrossRef CAS.
- H. Dong, Y. C. Chen and C. Feldmann, Polyol synthesis of nanoparticles: status and options regarding metals, oxides, chalcogenides, and non-metal elements, Green Chem., 2015, 17, 4107–4132 RSC.
- Y. Xia, Y. Xiong, B. Lim and S. E. Skrabalak, Shape-controlled synthesis of metal nanocrystals: Simple chemistry meets complex physics?, Angew. Chem., Int. Ed., 2009, 48, 60–103 CrossRef CAS.
- F. Qazi, Z. Hussain and M. N. Tahir, Advances in biogenic synthesis of palladium nanoparticles, RSC Adv., 2016, 6, 60277–60286 RSC.
- P. Vishnukumar, S. Vivekanandhan and S. Muthuramkumar, Plant-mediated biogenic synthesis of palladium nanoparticles: Recent trends and emerging opportunities, ChemBioEng Rev., 2017, 4, 18–36 CrossRef.
- M. Nasrollahzadeh, M. Sajjadi, J. Dadashi and H. Ghafuri, Pd-based nanoparticles: Plant-assisted biosynthesis, characterization, mechanism, stability, catalytic and antimicrobial activities, Adv. Colloid Interface Sci., 2020, 276, 102103 CrossRef CAS.
- A. Santoshi Kumari, M. Venkatesham, D. Ayodhya and G. Veerabhadram, Green synthesis, characterization and catalytic activity of palladium nanoparticles by xanthan gum, Appl. Nanosci., 2015, 5, 315–320 CrossRef CAS.
- A. Bankar, B. Joshi, A. R. Kumar and S. Zinjarde, Banana peel extract mediated novel route for the synthesis of palladium nanoparticles, Mater. Lett., 2010, 64, 1951–1953 CrossRef CAS.
- V. Manikandan, P. Velmurugan, J.-H. Park, N. Lovanh, S.-K. Seo, P. Jayanthi, Y.-J. Park, M. Cho and B.-T. Oh, Synthesis and antimicrobial activity of palladium nanoparticles from Prunus × yedoensis leaf extract, Mater. Lett., 2016, 185, 335–338 CrossRef CAS.
- S. Momeni and I. Nabipour, A simple green synthesis of palladium nanoparticles with Sargassum Alga and their electrocatalytic activities towards hydrogen peroxide, Appl. Biochem. Biotechnol., 2015, 176, 1937–1949 CrossRef CAS.
- J. Xu, A. R. Wilson, A. R. Rathmell, J. Howe, M. Chi and B. J. Wiley, Synthesis and catalytic properties of Au–Pd nanoflowers, ACS Nano, 2011, 5, 6119–6127 CrossRef CAS.
- K. Anand, C. Tiloke, A. Phulukdaree, B. Ranjan, A. Chuturgoon, S. Singh and R. M. Gengan, Biosynthesis of palladium nanoparticles by using Moringa oleifera flower extract and their catalytic and biological properties, J. Photochem. Photobiol., B, 2016, 165, 87–95 CrossRef CAS.
- K. S. Siddiqi and A. Husen, Green synthesis, characterization and uses of palladium/platinum nanoparticles, Nanoscale Res. Lett., 2016, 11, 482 CrossRef.
- M. N. Nadagouda and R. S. Varma, Green synthesis of silver and palladium nanoparticles at room temperature using coffee and tea extract, Green Chem., 2008, 10, 859–862 RSC.
- X. Yang, Q. Li, H. Wang, J. Huang, L. Lin, W. Wang, D. Sun, Y. Su, J. B. Opiyo, L. Hong, Y. Wang, N. He and L. Jia, Green synthesis of palladium nanoparticles using broth of Cinnamomum camphora leaf, J. Nanopart. Res., 2010, 12, 1589–1598 CrossRef CAS.
- D. S. Sheny, D. Philip and J. Mathew, Rapid green synthesis of palladium nanoparticles using the dried leaf of Anacardium occidentale, Spectrochim. Acta, Part A, 2012, 91, 35–38 CrossRef CAS.
- G. Zhan, J. Huang, M. Du, I. Abdul-Rauf, Y. Ma and Q. Li, Green synthesis of Au–Pd bimetallic nanoparticles: Single-step bioreduction method with plant extract, Mater. Lett., 2011, 65, 2989–2991 CrossRef CAS.
- M. Khan, M. Khan, M. Kuniyil, S. F. Adil, A. Al-Warthan, H. Z. Alkhathlan, W. Tremel, M. N. Tahir and M. R. H. Siddiqui, Biogenic synthesis of palladium nanoparticles using Pulicaria glutinosa extract and their catalytic activity towards the Suzuki coupling reaction, Dalton Trans., 2014, 43, 9026–9031 RSC.
- M. Rakibuddin and H. Kim, Facile green syntheses of palladium nanoparticles using fruit and aloe vera juices, and their surface–enhanced Raman scattering (SERS) activities, Mater. Res. Express, 2018, 5, 085001 CrossRef.
- Z. Katircioğlu, H. Şakalak, M. Ulaşan, A. C. Gören and M. S. Yavuz, Facile synthesis of “green” gold nanocrystals using cynarin in an aqueous solution, Appl. Surf. Sci., 2014, 318, 191–198 CrossRef.
- A. Kalaiselvi, S. M. Roopan, G. Madhumitha, C. Ramalingam and G. Elango, Synthesis and characterization of palladium nanoparticles using Catharanthus roseus leaf extract and its application in the photo-catalytic degradation, Spectrochim. Acta, Part A, 2015, 135, 116–119 CrossRef CAS.
- K. Mohan Kumar, B. K. Mandal, K. Siva Kumar, P. Sreedhara Reddy and B. Sreedhar, Biobased green method to synthesise palladium and iron nanoparticles using Terminalia chebula aqueous extract, Spectrochim. Acta, Part A, 2013, 102, 128–133 CrossRef CAS.
- L. Zhang, Q. Chang, H. Chen and M. Shao, Recent advances in palladium-based electrocatalysts for fuel cell reactions and hydrogen evolution reaction, Nano Energy, 2016, 29, 198–219 CrossRef CAS.
- Z. Cao, J. S. Derrick, J. Xu, R. Gao, M. Gong, E. M. Nichols, P. T. Smith, X. Liu, X. Wen, C. Coperet and C. J. Chang, Chelating N-heterocyclic carbene ligands enable tuning of electrocatalytic CO2 reduction to formate and carbon monoxide: Surface organometallic chemistry, Angew. Chem., Int. Ed., 2018, 57, 4981–4985 CrossRef CAS.
- A. Dumas and P. Couvreur, Palladium: a future key player in the nanomedical field?, Chem. Sci., 2015, 6, 2153–2157 RSC.
- M. A. Miller, B. Askevold, H. Mikula, R. H. Kohler, D. Pirovich and R. Weissleder, Nano-palladium is a cellular catalyst for in vivo chemistry, Nat. Commun., 2017, 8, 15906 CrossRef CAS.
- A. Biffis, P. Centomo, A. Del Zotto and M. Zecca, Pd metal catalysts for cross-couplings and related reactions in the 21st century: A critical review, Chem. Rev., 2018, 118, 2249–2295 CrossRef CAS.
- N. Armenise, N. Tahiri, N. N. H. M. Eisink, M. Denis, M. Jager, J. G. De Vries, M. D. Witte and A. J. Minnaard, Deuteration enhances catalyst lifetime in palladium-catalysed alcohol oxidation, Chem. Commun., 2016, 52, 2189–2191 RSC.
- M. Matuschek, D. P. Singh, H.-H. Jeong, M. Nesterov, T. Weiss, P. Fischer, F. Neubrech and N. Liu, Chiral plasmonic hydrogen sensors, Small, 2018, 14, 1702990 CrossRef.
- M. Raj, J.-M. Moon, R. N. Goyal, D.-S. Park and Y.-B. Shim, Simultaneous detection of ATP metabolites in human plasma and urine based on palladium nanoparticle and poly(bromocresol green) composite sensor, Biosens. Bioelectron., 2019, 126, 758–766 CrossRef CAS.
- T. Hennebel, S. De Corte, W. Verstraete and N. Boon, Microbial production and environmental applications of Pd nanoparticles for treatment of halogenated compounds, Curr. Opin. Biotechnol., 2012, 23, 555–561 CrossRef CAS.
- Q. Zheng, R. Farrauto and M. Deeba, Part ii: Oxidative thermal aging of Pd/Al2O3 and Pd/CexOy-ZrO2 in automotive three way catalysts: the effects of fuel shutoff and attempted fuel rich regeneration, Catalysts, 2015, 5, 1797–1814 CrossRef CAS.
- H. Tan, J. Wang, S. Yu and K. Zhou, Support morphology-dependent catalytic activity of Pd/CeO2 for formaldehyde oxidation, Environ. Sci. Technol., 2015, 49, 8675–8682 CrossRef CAS.
- H. Hildebrand, K. Mackenzie and F.-D. Kopinke, Pd/Fe3O4 nano-catalysts for selective dehalogenation in wastewater treatment processes-Influence of water constituents, Appl. Catal., B, 2009, 91, 389–396 CrossRef CAS.
- J. Choi, S. Chan, H. Joo, H. Yang and F. K. Ko, Three-dimensional (3D) palladium-zinc oxide nanowire nanofiber as photo-catalyst for water treatment, Water Res., 2016, 101, 362–369 CrossRef CAS.
- R. H. Crabtree, Resolving heterogeneity problems and impurity artifacts in operationally homogeneous transition metal catalysts, Chem. Rev., 2012, 112, 1536–1554 CrossRef CAS.
- C. Deraedt and D. Astruc, “Homeopathic” palladium nanoparticle catalysis of cross carbon–carbon coupling reactions, Acc. Chem. Res., 2014, 47, 494–503 CrossRef CAS.
- N. D. Burrows, C. R. H. Hale and R. L. Penn, Effect of ionic strength on the kinetics of crystal growth by oriented aggregation, Cryst. Growth Des., 2012, 12, 4787–4797 CrossRef CAS.
- F. Zereini, H. Alsenz, C. L. S. Wiseman, W. Püttmann, E. Reimer, R. Schleyer, E. Bieber and M. Wallasch, Platinum group elements (Pt, Pd, Rh) in airborne particulate matter in rural vs. urban areas of Germany: Concentrations and spatial patterns of distribution, Sci. Total Environ., 2012, 416, 261–268 CrossRef CAS.
- S. Rauch, B. Peucker-Ehrenbrink, L. T. Molina, M. J. Molina, R. Ramos and H. F. Hemond, Platinum group elements in airborne particles in mexico city, Environ. Sci. Technol., 2006, 40, 7554–7560 CrossRef CAS.
- K. Leopold, M. Maier, S. Weber and M. Schuster, Long-term study of palladium in road tunnel dust and sewage sludge ash, Environ. Pollut., 2008, 156, 341–347 CrossRef CAS.
- A. Turner and S. Price, Bioaccessibility of platinum group elements in automotive catalytic converter particulates, Environ. Sci. Technol., 2008, 42, 9443–9448 CrossRef CAS.
- H. Guo, X. Zheng, X. Luo and B. Mai, Leaching of brominated flame retardants (BFRs) from BFRs-incorporated plastics in digestive fluids and the influence of bird diets, J. Hazard. Mater., 2020, 393, 122397 CrossRef CAS.
- K. E. Wilkinson, L. Palmberg, E. Witasp, M. Kupczyk, N. Feliu, P. Gerde, G. A. Seisenbaeva, B. Fadeel, S.-E. Dahlén and V. G. Kessler, Solution-engineered palladium nanoparticles: Model for health effect studies of automotive particulate pollution, ACS Nano, 2011, 5, 5312–5324 CrossRef CAS.
- F. Zereini, C. L. S. Wiseman and W. Püttmann, In vitro investigations of platinum, palladium, and rhodium mobility in urban airborne particulate matter (PM10, PM2.5, and PM1) using simulated lung fluids, Environ. Sci. Technol., 2012, 46, 10326–10333 CAS.
- M. Geiser and W. G. Kreyling, Deposition and biokinetics of inhaled nanoparticles, Part. Fibre Toxicol., 2010, 7, 2 CrossRef.
- D. M. Aruguete, A. Wallace, T. Blakney, R. Kerr, G. Gerber and J. Ferko, Palladium release from catalytic converter materials induced by road de-icer components chloride and ferrocyanide, Chemosphere, 2020, 245, 125578 CrossRef CAS.
- G. Oberdörster, E. Oberdörster and J. Oberdörster, Nanotoxicology: An Emerging Discipline Evolving from Studies of Ultrafine Particles, Environ. Health Perspect., 2005, 113, 823–839 CrossRef.
- B. Song, P. Xu, G. Zeng, J. Gong, X. Wang, J. Yan, S. Wang, P. Zhang, W. Cao and S. Ye, Modeling the transport of sodium dodecyl benzene sulfonate in riverine sediment in the presence of multi-walled carbon nanotubes, Water Res., 2018, 129, 20–28 CrossRef CAS.
- M. Chen, S. Zhou, Y. Zhu, Y. Sun, G. Zeng, C. Yang, P. Xu, M. Yan, Z. Liu and W. Zhang, Toxicity of carbon nanomaterials to plants, animals and microbes: Recent progress from 2015-present, Chemosphere, 2018, 206, 255–264 CrossRef CAS.
- T. Y. Sun, F. Gottschalk, K. Hungerbühler and B. Nowack, Comprehensive probabilistic modelling of environmental emissions of engineered nanomaterials, Environ. Pollut., 2014, 185, 69–76 CrossRef CAS.
- D. Zhou, A. I. Abdel-Fattah and A. A. Keller, Clay particles destabilize engineered nanoparticles in aqueous environments, Environ. Sci. Technol., 2012, 46, 7520–7526 CrossRef CAS.
- F. Zereini, C. L. S. Wiseman, M. Vang, P. Albers, W. Schneider, R. Schindl and K. Leopold, Geochemical behaviour of palladium in soils and Pd/PdO model substances in the presence of the organic complexing agents l-methionine and citric acid, Environ. Sci.: Processes Impacts, 2016, 18, 22–31 RSC.
- X. Wang, C. H. Chang, J. Jiang, Q. Liu, Y.-P. Liao, J. Lu, L. Li, X. Liu, J. Kim, A. Ahmed, A. E. Nel and T. Xia, The crystallinity and aspect ratio of cellulose nanomaterials determine their pro-inflammatory and immune adjuvant effects in vitro and in vivo, Small, 2019, 15, 1901642 CrossRef CAS.
- C. L. S. Wiseman and F. Zereini, Airborne particulate matter, platinum group elements and human health: A review of recent evidence, Sci. Total Environ., 2009, 407, 2493–2500 CrossRef CAS.
- C. L. S. Wiseman, J. Niu, C. Levesque, M. Chenier and P. E. Rasmussen, An assessment of the inhalation bioaccessibility of platinum group elements in road dust using a simulated lung fluid, Environ. Pollut., 2018, 241, 1009–1017 CrossRef CAS.
- M. Chen, S. Chen, M. Du, S. Tang, M. Chen, W. Wang, H. Yang, Q. Chen and J. Chen, Toxic effect of palladium on embryonic development of zebrafish, Aquat. Toxicol., 2015, 159, 208–216 CrossRef CAS.
- J. C. Wataha and C. T. Hanks, Biological effects of palladium and risk of using palladium in dental casting alloys, J. Oral Rehabil., 1996, 23, 309–320 CrossRef CAS.
- S. Wagner, A. Gondikas, E. Neubauer, T. Hofmann and F. von der Kammer, Spot the difference: Engineered and natural nanoparticles in the environment—release, behavior, and fate, Angew. Chem., Int. Ed., 2014, 53, 12398–12419 CAS.
- C. Colombo, A. J. Monhemius and J. A. Plant, Platinum, palladium and rhodium release from vehicle exhaust catalysts and road dust exposed to simulated lung fluids, Ecotoxicol. Environ. Saf., 2008, 71, 722–730 CrossRef CAS.
- I. Iavicoli, M. Farina, L. Fontana, D. Lucchetti, V. Leso, C. Fanali, V. Cufino, A. Boninsegna, K. Leopold, R. Schindl, D. Brucker and A. Sgambato, In vitro evaluation of the potential toxic effects of palladium nanoparticles on fibroblasts and lung epithelial cells, Toxicol. In Vitro, 2017, 42, 191–199 CrossRef CAS.
- E. Dahal, J. Curtiss, D. Subedi, G. Chen, J. P. Houston and S. Smirnov, Evaluation of the catalytic activity and cytotoxicity of palladium nanocubes: The role of oxygen, ACS Appl. Mater. Interfaces, 2015, 7, 9364–9371 CrossRef CAS.
- Y.-T. Pan, C. E. Smith, K. S. Kwok, J. Chen, H. Kong and H. Yang, Functionalized ultrathin palladium nanosheets as patches for HepG2 cancer cells, Chem. Commun., 2015, 51, 14171–14174 RSC.
- F. Kastury, E. Smith and A. L. Juhasz, A critical review of approaches and limitations of inhalation bioavailability and bioaccessibility of metal(loid)s from ambient particulate matter or dust, Sci. Total Environ., 2017, 574, 1054–1074 CrossRef CAS.
- A. Faurschou, T. Menné, J. D. Johansen and J. P. Thyssen, Metal allergen of the 21st century—a review on exposure, epidemiology and clinical manifestations of palladium allergy, Contact Dermatitis, 2011, 64, 185–195 CrossRef.
- J. Muris, A. Goossens, M. Gonçalo, A. J. Bircher, A. Giménez-Arnau, C. Foti, T. Rustemeyer, A. J. Feilzer and C. J. Kleverlaan, Sensitization to palladium and nickel in Europe and the relationship with oral disease and dental alloys, Contact Dermatitis, 2015, 72, 286–296 CrossRef CAS.
- S. Shi, Y. Huang, X. Chen, J. Weng and N. Zheng, Optimization of surface coating on small Pd nanosheets for in vivo near-infrared photothermal therapy of tumor, ACS Appl. Mater. Interfaces, 2015, 7, 14369–14375 CrossRef CAS.
- F. Zereini, C. L. S. Wiseman, J. Poprizki, P. Albers, W. Schneider and K. Leopold, Assessing the potential of inorganic anions (Cl−, NO3−, SO42− and PO43−) to increase the bioaccessibility of emitted palladium in the environment: Experimental studies with soils and a Pd model substance, Environ. Pollut., 2017, 220, 1050–1058 CrossRef CAS.
- T. Peric, V. Jakovljevic, V. Zivkovic, J. Krkeljic, Z. D. Petrovic, D. Simijonovic, S. Novokmet, D. M. Djuric and S. M. Jankovic, Toxic effects of palladium compounds on the isolated rat heart, Med. Chem., 2012, 8, 9–13 CrossRef CAS.
- S. Luderwald, F. Seitz, G. A. Seisenbaeva, V. G. Kessler, R. Schulz and M. Bundschuh, Palladium Nanoparticles: Is There a Risk for Aquatic Ecosystems?, Bull. Environ. Contam. Toxicol., 2016, 97, 153–158 CrossRef.
- M. Crosera, M. Mauro, M. Bovenzi, G. Adami, E. Baracchini, G. Maina and F. Larese Filon, In vitro permeation of palladium powders through intact and damaged human skin, Toxicol. Lett., 2018, 287, 108–112 CrossRef CAS.
- M. J. Hosseini, I. Jafarian, S. Farahani, R. Khodadadi, S. H. Tagavi, P. Naserzadeh, A. Mohammadi-Bardbori and N. Arghavanifard, New mechanistic approach of inorganic palladium toxicity: impairment in mitochondrial electron transfer, Metallomics, 2016, 8, 252–259 RSC.
- L. Fontana, V. Leso, A. Marinaccio, G. Cenacchi, V. Papa, K. Leopold, R. Schindl, B. Bocca, A. Alimonti and I. Iavicoli, The effects of palladium nanoparticles on the renal function of female Wistar rats, Nanotoxicology, 2014, 18, 1–9 Search PubMed.
- E. Amoozadeh, M. Malek, R. Rashidinejad, S. Nabavi, M. Karbassi, R. Ghayoumi, G. Ghorbanzadeh-Zafarani, H. Salehi and B. Sures, Marine organisms as heavy metal bioindicators in the Persian Gulf and the Gulf of Oman, Environ. Sci. Pollut. Res., 2014, 21, 2386–2395 CrossRef CAS.
- P. Chhay, M. Murphy-Marion, Y. Samson and D. Girard, Activation of human eosinophils with palladium nanoparticles (Pd NPs): importance of the actin cytoskeleton in Pd NPs-induced cellular adhesion, Environ. Toxicol. Pharmacol., 2018, 57, 95–103 CrossRef CAS.
- R. T. Peterson and C. A. Macrae, Systematic approaches to toxicology in the zebrafish, Annu. Rev. Pharmacol. Toxicol., 2012, 52, 433–453 CrossRef CAS.
- D. Kokel, J. Bryan, C. Laggner, R. White, C. Y. J. Cheung, R. Mateus, D. Healey, S. Kim, A. A. Werdich, S. J. Haggarty, C. A. MacRae, B. Shoichet and R. T. Peterson, Rapid behavior-based identification of neuroactive small molecules in the zebrafish, Nat. Chem. Biol., 2010, 6, 231–237 CrossRef CAS.
- S. Scholz, S. Fischer, U. Gündel, E. Küster, T. Luckenbach and D. Voelker, The zebrafish embryo model in environmental risk assessment—applications beyond acute toxicity testing, Environ. Sci. Pollut. Res., 2008, 15, 394–404 CrossRef CAS.
- K. S. Egorova, A. A. Sinjushin, A. V. Posvyatenko, D. B. Eremin, A. S. Kashin, A. S. Galushko and V. P. Ananikov, Evaluation of phytotoxicity and cytotoxicity of industrial catalyst components (Fe, Cu, Ni, Rh and Pd): A case of lethal toxicity of a rhodium salt in terrestrial plants, Chemosphere, 2019, 223, 738–747 CrossRef CAS.
- A. Speranza, K. Leopold, M. Maier, A. R. Taddei and V. Scoccianti, Pd-nanoparticles cause increased toxicity to kiwifruit pollen compared to soluble Pd(II), Environ. Pollut., 2010, 158, 873–882 CrossRef CAS.
- K.-J. Dietz and S. Herth, Plant nanotoxicology, Trends Plant Sci., 2011, 16, 582–589 CrossRef CAS.
- F. Battke, K. Leopold, M. Maier, U. Schmidhalter and M. Schuster, Palladium exposure of barley: uptake and effects, Plant Biol., 2008, 10, 272–276 CrossRef CAS.
- V. Shah and I. Belozerova, Influence of metal nanoparticles on the soil microbial community and germination of lettuce seeds, Water, Air, Soil Pollut., 2009, 197, 143–148 CrossRef CAS.
- Y. Liu, G. Zeng, H. Zhong, Z. Wang, Z. Liu, M. Cheng, G. Liu, X. Yang and S. Liu, Effect of rhamnolipid solubilization on hexadecane bioavailability: enhancement or reduction?, J. Hazard. Mater., 2017, 322, 394–401 CrossRef CAS.
- Z. Huang, K. He, Z. Song, G. Zeng, A. Chen, L. Yuan, H. Li, L. Hu, Z. Guo and G. Chen, Antioxidative response of Phanerochaete chrysosporium against silver nanoparticle-induced toxicity and its potential mechanism, Chemosphere, 2018, 211, 573–583 CrossRef CAS.
- L. Zhang, J. Zhang, G. Zeng, H. Dong, Y. Chen, C. Huang, Y. Zhu, R. Xu, Y. Cheng, K. Hou, W. Cao and W. Fang, Multivariate relationships between microbial communities and environmental variables during co-composting of sewage sludge and agricultural waste in the presence of PVP-AgNPs, Bioresour. Technol., 2018, 261, 10–18 CrossRef CAS.
- A. Nuzzo, B. Hosseinkhani, N. Boon, G. Zanaroli and F. Fava, Impact of bio-palladium nanoparticles (bio-Pd NPs) on the activity and structure of a marine microbial community, Environ. Pollut., 2017, 220, 1068–1078 CrossRef CAS.
- C. Vannini, G. Domingo, M. Marsoni, A. Fumagalli, R. Terzaghi, M. Labra, F. De Mattia, E. Onelli and M. Bracale, Physiological and molecular effects associated with palladium treatment in Pseudokirchneriella subcapitata, Aquat. Toxicol., 2011, 102, 104–113 CrossRef CAS.
- B. Hosseinkhani, T. Hennebel, S. Van Nevel, S. Verschuere, M. M. Yakimov, S. Cappello, M. Blaghen and N. Boon, Biogenic nanopalladium based remediation of chlorinated hydrocarbons in marine environments, Environ. Sci. Technol., 2014, 48, 550–557 CrossRef CAS.
- S. Zimmermann and B. Sures, Lessons learned from studies with the freshwater mussel Dreissena polymorpha exposed to platinum, palladium and rhodium, Sci. Total Environ., 2018, 615, 1396–1405 CrossRef CAS.
- X. Hou, X. Chen, S. Bi, K. Li, C. Zhang, J. Wang and W. Zhang, Catalytic degradation of TCE by a PVDF membrane with Pd-coated nanoscale zero-valent iron reductant, Sci. Total Environ., 2020, 702, 135030 CrossRef CAS.
- C. Balu, G. Sougata, N. Rahul, D. Ankush, G. B. Tomar, C. Rohan, M. Piyush, K. Rohini, K. Sangeeta and B. Jayesh, Novel platinum–palladium bimetallic nanoparticles synthesized by Dioscorea bulbifera: anticancer and antioxidant activities, Int. J. Nanomed., 2015, 10, 7477–7490 Search PubMed.
- Y.-L. Wu, N. Putcha, K. W. Ng, D. T. Leong, C. T. Lim, S. C. J. Loo and X. Chen, Biophysical responses upon the interaction of nanomaterials with cellular interfaces, Acc. Chem. Res., 2013, 46, 782–791 CrossRef CAS.
- J. Ging, R. Tejerina-Anton, G. Ramakrishnan, M. Nielsen, K. Murphy, J. M. Gorham, N. Tinh and A. Orlov, Development of a conceptual framework for evaluation of nanomaterials release from nanocomposites: Environmental and toxicological implications, Sci. Total Environ., 2014, 473, 9–19 CrossRef.
- J. A. J. Meesters, W. J. G. M. Peijnenburg, A. J. Hendriks, D. Van de Meent and J. T. K. Quik, A model sensitivity analysis to determine the most important physicochemical properties driving environmental fate and exposure of engineered nanoparticles, Environ. Sci.: Nano, 2019, 6, 2049–2060 RSC.
- E. Abdulbur-Alfakhoury, S. Van Zutphen and M. Leermakers, Development of the diffusive gradients in thin films technique (DGT) for platinum (Pt), palladium (Pd), and rhodium (Rh) in natural waters, Talanta, 2019, 203, 34–48 CrossRef CAS.
- J. E. Jaine and M. R. Mucalo, Rapid determination of rhodium, palladium, and platinum in supported metal catalysts using multivariate analysis of laser induced breakdown spectroscopy data, Spectrochim. Acta, Part B, 2018, 145, 58–63 CrossRef CAS.
- J. Jia, C. Bi, X. Guo, X. Wang, X. Zhou and Z. Chen, Characteristics, identification, and potential risk of polycyclic aromatic hydrocarbons in road dusts and agricultural soils from industrial sites in Shanghai, China, Environ. Sci. Pollut. Res., 2017, 24, 605–615 CrossRef CAS.
- D. B. Warheit, C. M. Sayes and K. L. Reed, Nanoscale and fine zinc oxide particles: Can in vitro assays accurately forecast lung hazards following inhalation exposures?, Environ. Sci. Technol., 2009, 43, 7939–7945 CrossRef CAS.
- A. Tan, A. Martin, N. Tri-Hung, B. J. Boyd and C. A. Prestidge, Hybrid nanomaterials that mimic the food effect: Controlling enzymatic digestion for enhanced oral drug absorption, Angew. Chem., Int. Ed., 2012, 51, 5475–5479 CrossRef CAS.
- M. Elsabahy and K. L. Wooley, Data mining as a guide for the construction of cross-linked nanoparticles with low immunotoxicity via control of polymer chemistry and supramolecular assembly, Acc. Chem. Res., 2015, 48, 1620–1630 CrossRef CAS.
- X. Liu, M. Wu, Q. Hu, H. Bai, S. Zhang, Y. Shen, G. Tang and Y. Ping, Redox-activated light-up nanomicelle for precise imaging-guided cancer therapy and real-time pharmacokinetic monitoring, ACS Nano, 2016, 10, 11385–11396 CrossRef CAS.
- C. P. Adams, K. A. Walker, S. O. Obare and K. M. Dochert, Size-dependent antimicrobial effects of novel palladium nanoparticles, PLoS One, 2014, 9, e85981 CrossRef.
- H. Munmi, B. Debajit, B. Popymita, A. R. Silva and D. Pankaj, Biogenic synthesis of palladium nanoparticles and their applications as catalyst and antimicrobial agent, PLoS One, 2017, 12, e0184936 CrossRef.
- W. He, H. Wu, W. G. Wamer, H.-K. Kim, J. Zheng, H. Jia, Z. Zheng and J.-J. Yin, Unraveling the enhanced photocatalytic activity and phototoxicity of ZnO/metal hybrid nanostructures from generation of reactive oxygen species and charge carriers, ACS Appl. Mater. Interfaces, 2014, 6, 15527–15535 CrossRef CAS.
- H. Zhang, S. Pokhrel, Z. Ji, H. Meng, X. Wang, S. Lin, C. H. Chang, L. Li, R. Li, B. Sun, M. Wang, Y.-P. Liao, R. Liu, T. Xia, L. Maedler and A. E. Nel, PdO doping tunes band-gap energy levels as well as oxidative stress responses to a Co3O4 p-type semiconductor in cells and the lung, J. Am. Chem. Soc., 2014, 136, 6406–6420 CrossRef CAS.
- S. Gurunathan, M. Qasim, C. H. Park, M. A. Iqbal, H. Yoo, J. H. Hwang, S. J. Uhm, H. Song, C. Park, Y. Choi, J.-H. Kim and K. Hong, Cytotoxicity and Transcriptomic Analyses of Biogenic Palladium Nanoparticles in Human Ovarian Cancer Cells (SKOV3), Nanomaterials, 2019, 9, 787 CrossRef CAS.
- X. Chen, J. Li, Y. Huang, J. Wei, D. Sun and N. Zheng, The biodistribution, excretion and potential toxicity of different-sized Pd nanosheets in mice following oral and intraperitoneal administration, Biomater. Sci., 2017, 5, 2448–2455 RSC.
- L. Bellani, L. Giorgetti, S. Riela, G. Lazzara, A. Scialabba and M. Massaro, Ecotoxicity of halloysite nanotube-supported palladium nanoparticles in Raphanus sativus L, Environ. Toxicol. Chem., 2016, 35, 2503–2510 CrossRef CAS.
- R. Mulholland and A. Turner, Accumulation of platinum group elements by the marine gastropod Littorina littorea, Environ. Pollut., 2011, 159, 977–982 CrossRef CAS.
- W. Liu, Y. Wang and C. Jing, Transcriptome analysis of silver, palladium, and selenium stresses in Pantoea sp IMH, Chemosphere, 2018, 208, 50–58 CrossRef CAS.
- V. Aruoja, S. Pokhrel, M. Sihtmaae, M. Mortimer, L. Maedler and A. Kahru, Toxicity of 12 metal-based nanoparticles to algae, bacteria and protozoa, Environ. Sci.: Nano, 2015, 2, 630–644 RSC.
- M. Samira Athie-Garcia, H. Amelia Pinon-Castillo, L. Nayzzel Munoz-Castellanos, A. Laura Ulloa-Ogaz, P. Ivonne Martinez-Varela, A. Quintero-Ramos, R. Duran, J. Guadalupe Murillo-Ramirez and E. Orrantia-Borunda, Cell wall damage and oxidative stress in Candida albicans ATCC10231 and Aspergillus niger caused by palladium nanoparticles, Toxicol. In Vitro, 2018, 48, 111–120 CrossRef.
- G. Schertzinger, S. Zimmermann, D. Grabner and B. Sures, Assessment of sublethal endpoints after chronic exposure of the nematode Caenorhabditis elegans to palladium, platinum and rhodium, Environ. Pollut., 2017, 230, 31–39 CrossRef CAS.
- M. Chen, S. Chen, C. He, S. Mo, X. Wang, G. Liu and N. Zheng, Safety profile of two-dimensional Pd nanosheets for photothermal therapy and photoacoustic imaging, Nano Res., 2017, 10, 1234–1248 CrossRef CAS.
|
This journal is © The Royal Society of Chemistry 2021 |
Click here to see how this site uses Cookies. View our privacy policy here.