DOI:
10.1039/D0RA04316H
(Review Article)
RSC Adv., 2020,
10, 39722-39730
A sodium alginate-based sustained-release IPN hydrogel and its applications
Received
14th May 2020
, Accepted 19th October 2020
First published on 30th October 2020
Abstract
Interpenetrating polymer network (IPN) hydrogels are crosslinked by two or more polymer networks, providing free volume space in the three-dimensional network structure, and providing conditions for the sustained and controlled release of drugs. The IPN hydrogels based on the natural polymer sodium alginate can form a stable porous network structure. Due to its excellent biocompatibility, the loaded drug can be sustained to the maximum extent without affecting its pharmacological effect. Sodium alginate-based IPN hydrogels have broad application prospects in the field of sustained and controlled drug release. This paper begins with an overview of the formation of alginate-based IPN hydrogels; summarizes the types of alginate-based IPN hydrogels; and discusses the pharmaceutical applications of alginate-based IPN hydrogels. We aim to give an overview of the research on IPN hydrogels based on sodium alginate in sustained and controlled drug release systems.
Introduction
Hydrogels are colloids composed of a hydrophilic polymer with a high water content and a three-dimensional (3D) crosslinked networks structure,1 widely used in tissue engineering, biomedicine and other fields.2–4 Traditional hydrogels have defects such as poor mechanical properties5 and single performance,6 and their application in biomedicine is significantly limited. In recent years, with the development of life sciences, people have submitted higher requirements for hydrogels. It is well-known that hydrogels can be widely used in the sustained and controlled release of drugs, and good biocompatibility and biodegradability are critical factors for their success.7
Interpenetrating polymer network (IPN) hydrogels are cross-linked by two or more polymer networks (such as chitosan, alginate, starch, polyacrylamide, polyacrylic acid, polyvinyl alcohol, etc.). In semi-IPN hydrogels, a network with linear polymers is embedded within the first network. IPN hydrogels contain two or more polymers, which can be cross-linked in the presence of each other to give a 3D network structure, producing a free volume for the easy encapsulation of drugs,8 and provide conditions for sustained and controlled release of drugs. IPN hydrogels also have mechanical strengthening,9 biodegradation kinetics,10 in vivo dual polymer activity,11 new nanostructures12 and other characteristics, which provide biocompatibility and convenience for molecules ranging from non-steroidal anti-inflammatory drugs (NSAIDs) to proteins and peptides and stable drug delivery systems.
Natural polymers have excellent biocompatibility and biodegradability, and are widely distributed and easily obtained.13,14 Among them, sodium alginate can be used as a matrix to prepare macroporous hydrogels.15,16 Sodium alginate is a linear block copolymer comprising 1,4-linked β-D-mannuronic and α-L-guluronic acid residues.17 It is considered to be non-toxic and biocompatible,18 and its compatibility with drugs does not affect the pharmacological effects, showing that it has more natural advantages in the sustained and controlled release of drugs. At present, scientists have conducted a lot of research on IPN hydrogels made from the natural polymer sodium alginate. This review discusses the formation and types of alginate-based IPN hydrogels, and their pharmaceutical application.
IPN hydrogels
IPNs are alloys of cross-linked polymers19 that are formed by interpenetrating entanglement of two or more cross-linked polymers. When only one polymer in the system is cross-linked, it is called a semi-IPN. IPNs are often created for the purpose of conferring key attributes of one of the components while maintaining the critical attributes of another. In some cases, entirely new, and sometimes surprising properties are exhibited by the IPN that are not observed in either of the two single networks alone.20
IPNs and semi-IPNs used in biomedicine include films,21 microspheres22 and hydrogels.20 They are used as scaffold materials for tissue engineering,23 drug delivery systems24 and in many other biomedical fields. IPN-based hydrogels have attracted the attention of researchers in the biomedical field due to their excellent properties. Hydrogels prepared from IPNs have various properties, including better mechanical strength,24 stability,25 and stimulus responsiveness.26
Sodium alginate is a polymer based on natural polysaccharides. In the presence of divalent cations, it exhibits unique gelation characteristics in aqueous solutions, but the gel is accompanied by shortcomings such as instability and fragility. It can be combined with polymers with temperature responsiveness, electric field responsiveness and other responsiveness, which can not only make up for the original defects, but also prepare various intelligent IPN hydrogels with the help of IPN technology. The fruitful precedent of combining sodium alginate with different polymers has promoted the development of IPN hydrogels, which have sustained and controlled release of drugs,27 high porosity28 and multiple responsiveness.28–30
Formation of alginate-based IPN hydrogels
The main chain of sodium alginate contains a large number of –COO− reactive groups, and the anions in aqueous solution exhibit aggregation behavior, so it has some adhesiveness and can be used as a drug carrier for the treatment of mucosal tissues.31 The pH sensitivity of sodium alginate also originates from the –COO− group. Under acidic conditions, –COO− is converted to –COOH, and the hydrogen bond interaction between the carboxyl groups of sodium alginate molecules is enhanced, which causes the molecular chain to shrink.32 When the pH value increases, the –COOH group tends to ionize and produce –COO−.33 At that time, the hydrophilicity of sodium alginate also increases,34 so that the molecular chain can be extended.
Sodium alginate hydrogels have a wide range of applications in sustained and controlled release of drugs,35–37 and its pH sensitivity can be a key element of its selective release in the stomach.38 Sodium alginate has unique gelation properties in aqueous solution in the presence of divalent cations.39 The sodium ion of gurouronic acid exchanges with the divalent cation, and the divalent cationic association proximal fraction belongs to carboxyl groups on different sodium alginate molecular chains to form a hydrogel with a cross-linked network structure.40 Such a 3D network structure provides a large number of drug-loading sites41 and is used to manufacture various drugs, proteins and even cellular controlled release systems.42,43 Because sodium alginate can have extremely mild gelation conditions, it has the stability required for preparation of pharmaceutical excipients,44 which also prevents the inactivation of pharmaceutically active substances.45
Under normal circumstances, the burst release of drug molecules from sodium alginate hydrogels which formed by Ca2+ cross-linking (Fig. 1) is a common issue in the field of controlled drug delivery systems, leading to suddenly burst release.16 In recent years, a lot of work has been conducted to improve the performance of sodium alginate hydrogels as drug carriers. Compounds of sodium alginate with other polymers such as polyvinyl alcohol (PVA),46 gelatin,47 or hydroxypropyl methyl cellulose,48 and the coating of sodium alginate beads with cationic polymers such as chitosan has been found to improve the problems of drug leaking and encapsulation rate.49 Cross-linking of sodium alginate and ovalbumin alone has also been successful.50 The formation of hydrogels may be the product of one or more cross-linking methods.
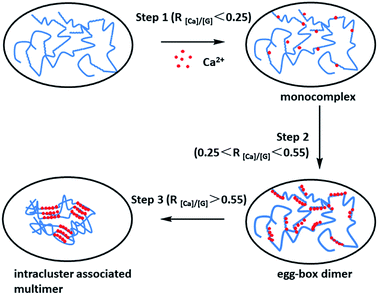 |
| Fig. 1 Schematic drawing of the multiple-step binding of Ca2+ to alginate with a considerable chain length. The zigzag lines, smooth lines, and dots stand for G-block, M-block, and Ca2+, respectively. R[Ca]/[G] is the feeding ratio of added Ca2+ to unit G residue. This figure has been adapted from ref. 50 with permission from American Chemical Society, Copyright © 2007. | |
IPN technology can use polymers with certain special properties to improve the deficiencies of sodium alginate. Taking sodium alginate/polyvinyl alcohol (PVA) hydrogels as an example, the PVA molecule is soft, and the sodium alginate gel is rigid, and the introduction of PVA can increase the elasticity of the gel.51
Jao52 et al. chose not to use chemical cross-linking agents but physical cross-linking-prepared semi-IPN hydrogel, which relied on Ca2+ cross-linking between alginate chains and hydrogen bonding interaction between PVA chains (Fig. 2). In such systems, the PVA network can provide mechanical enhancement and temperature sensitivity, while the alginate network can provide pH sensitivity. They showed that when the pH value is 4, the swelling is minimal, and the swelling ratio increases with pH value. The enthalpy of alginate/PVA hydrogel suddenly increases between 37 and 45 °C, which may be caused by hydrogen bond dissociation of alginate/PVA hydrogel. The elastic modulus and effective cross-linking density of the composite increases with increase of alginate content, which may be due to the increase of hydrophobic interaction and polymer interaction. Alginate/PVA hydrogels are sensitive to pH and temperature and can be invoked as controlled release systems in biomedical fields.
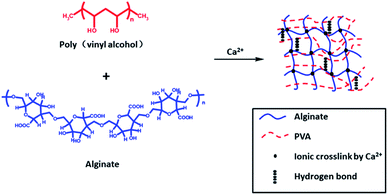 |
| Fig. 2 Physically cross-linked sodium alginate-based semi-IPN hydrogel film formation mechanism. This figure has been adapted from ref. 52 with permission from John Wiley & Sons, Ltd., Copyright © 2008. | |
Kulkarni46 and others used glutaraldehyde as a cross-linking agent to prepare sodium alginate/PVA IPN hydrogel membranes by chemical cross-linking (Fig. 3), which was used for the sustained release of the antihypertensive drug prazosin hydrochloride. Prazosin hydrochloride is a widely used drug for the treatment of hypertension and congestive heart failure that requires multiple doses to maintain blood levels of the drug. The IPN hydrogel membranes extend the drug release up to 24 h, while sodium alginate and PVA hydrogel membranes discharge the drug quickly. Cineole has shown greater enhancement in drug permeation. A study of skin irritation and skin histopathology has indicated that the prepared IPN hydrogel membranes are perfectly safe for transdermal drug delivery applications.
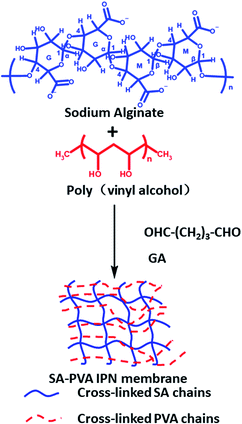 |
| Fig. 3 Formation mechanism of chemically cross-linked sodium alginate-based IPN hydrogel. This figure has been adapted from ref. 46 with permission from Elsevier B.V., copyright © 2010. | |
Boppana53 et al. used a Ca2+ + glutaraldehyde double cross-linking method to prepare IPN microspheres with ketoprofen-preloaded, polyacrylamide-grafted gum ghatti and sodium alginate (Fig. 4). Ketoprofen is an NSAID currently being utilized to treat musculoskeletal and joint diseases. It is easily absorbed from the gastrointestinal tract and has a short half-life of only 2 h. After oral administration, gastric irritation, ulcer, bleeding and other adverse reactions occurred. Therefore, in order to effectively treat musculoskeletal diseases and patient compliance, IPN hydrogel microbeads prepared in this study were used to control the release of ketoprofen and avoid its release in the stomach. As IPN microbeads contain –COO− reactive groups, they are un-ionized in the stomach pH, leading to small amounts of drug release. In contrast, the microbeads undergo ionization in the intestinal pH, leading to greater drug release. In vivo experiments in Wistar rats also confirmed this conclusion. The pH-sensitive IPN microbeads blocked the release of drugs in the stomach and reduced ulcers, bleeding and mucosal erosion. The controlled release effect of ketoprofen was also confirmed. Therefore, IPN microbeads are useful for gastro-protective controlled delivery of NSAIDs.
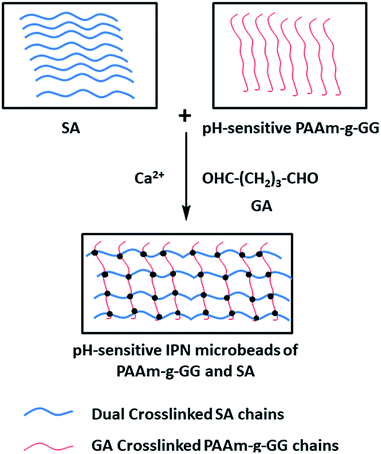 |
| Fig. 4 Formation mechanism of sodium alginate-based IPN gel microspheres with double cross-linking of Ca2+ + GA. This figure has been adapted from ref. 53 with permission from Elsevier B.V., copyright © 2015. | |
Wen54 et al. prepared IPN hydrogels composed of gelatin and sodium alginate by the double cross-linking method of enzymes and ions (Fig. 5). The gel can support cell adhesion and diffusion in vitro. Surprisingly, IPN hydrogels can even withstand traditional steam sterilization, which may seriously damage the morphology and mechanical properties of traditional hydrogels. The enzymatic and calcium cross-linking methods are straightforward, efficient and free of harmful and toxic chemicals. The results show that the sodium alginate IPN hydrogel has excellent biocompatibility and robust mechanical properties, and provides an opportunity to be used as a tissue engineering scaffold.
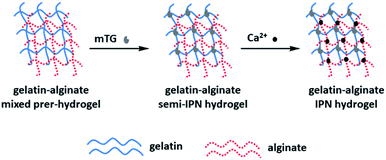 |
| Fig. 5 Formation mechanism of sodium alginate-based IPN hydrogel with double cross-linked of Enzyme and ion. This figure has been adapted from ref. 54 with permission from WILEY-VCH Verlag GmbH&Co. KGaA, Weinheim, copyright © 2014. | |
Types of IPN hydrogels based on sodium alginate
In recent years, researchers have controlled the release of drugs by changing the structure of hydrogels, and can sense changes in environmental information such as temperature,55 pH value,56 or chemical substances57 at the lesion site to achieve the effect of sustained and controlled release, increasing the safety of drug-loaded hydrogels, and other advantages. Although a single component sodium alginate hydrogel is pH sensitive, no matter what kind of cross-linking method is used, the sustained and controlled release of drugs is not ideal. Therefore, sodium alginate is generally used in combination with other polymer compounds, and researchers have prepared a series of smart IPN hydrogels with the help of IPN technology.
The pH-sensitive hydrogels
Khalid58 et al. prepared PVA-grafted poly(acrylic acid)/sodium alginate semi-IPN hydrogel by chemical cross-linking, and the hydrogels supported loxoprofen sodium. The prepared hydrogels had a high pH sensitivity, and showed maximal swelling and drug loading and release at pH 7.4, and lower at pH 1.2. The biodegradability and biocompatibility of sodium alginate and poly(methacrylic acid) semi-IPN hydrogel has been used to control the release of theophylline.59 Upadhay and others60 used the natural polymers locust bean gum and sodium alginate to develop an IPN sodium alginate hydrogel for capecitabine. In vitro drug release studies have shown sustained release for 12 h. Basu61 et al. developed a nano-silver composite semi-IPN hydrogel of sodium alginate and acrylamide, and explored its drug-release characteristics. The encapsulated nano-silver particles and ciprofloxacin molecules showed sustained release.
Due to the addition of the second component, the pH-responsive sodium alginate-based IPN hydrogels show better sustained and controlled release of drugs, as well as excellent mechanical strength.
Temperature/pH double-sensitive hydrogels
It can be seen from the above that sodium alginate hydrogels have pH sensitivity. However, single responsive hydrogels can no longer respond to the needs of contemporary medicine. IPN hydrogels prepared by various cross-linking methods from two or more non-interfering, single sensitive polymer monomers have double/multiple responsiveness. In addition to temperature changes,62 the focal site of patients with cancer or inflammation often has localized acidification. These two characteristics usually could be used for specific drug delivery.63 Recent in-depth study of multiple responsive hydrogels has broadened the potential applications of smart hydrogels. Research hotspots are concentrated on the preparation of temperature/pH dual-response hydrogels and their applications in the field of biomedicine. This is because temperature and pH are important physiological parameters in human systems, and they are also easy to sense and control stimulus signals.64–67
The IPN hydrogel formed by doxorubicin (DOX)-loaded sodium alginate and poly(N-isopropylacrylamide) copolymer showed sustained-release of the drug at physiological pH and temperature (Fig. 6). DOX released from the hydrogels is encapsulated in the micelles formed by the co-polymers, i.e., the hydrogels release DOX-encapsulated micelles in a sustained manner that has been found to be effective in cancer treatment.29 Eswaramma and others19 prepared IPN hydrogel microbeads based on sodium alginate and functionally modified guar gum. They showed that the microbeads may be sensitive to both pH and temperature. At 37 °C and pH 7.4, the release time of zidovudine (an anti-HIV drug) was extended to 34 h. Jalababu and others68 used modified sodium alginate, N-isopropylacrylamide and acrylamide as raw materials, and adopted a single-step free radical polymerization reaction to cross-link with N,N′-methylenebisacrylamide to synthesize a new polymer. The pH/temperature dual-response type was used to control the IPN hydrogel released by the anticancer drug was extended to 48 h. Vasanta Madhavi and others69 used locust bean gum and sodium alginate to prepare IPN hydrogels beads through glutaraldehyde cross-linking to study the controlled release of the anti-inflammatory drug nimesulide. In vitro dissolution experiments showed that nimesulide had prolonged release of 48 h.
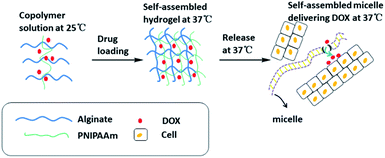 |
| Fig. 6 Mechanism of temperature/pH double-sensitive IPN hydrogels based on sodium alginate release at physiological temperature. This figure has been adapted from ref. 29 with permission from American Chemical Society, copyright © 2017. | |
The sustained release capability of these newly developed drug-loaded temperature/pH dual-response IPN hydrogels may be advantageous because it can achieve the purpose of reducing the dosing interval and is beneficial to patient compliance. The temperature/pH dual-response IPN hydrogels can be a promising candidate preparation for targeted and sustained release of anti-cancer and anti-inflammatory drugs.
Electric field/pH double-sensitive hydrogels
Electric-field-sensitive hydrogels control the release of drugs by monitoring the on/off of electrical signals. Sodium alginate is a polymer polyelectrolyte, and its hydrogels are responsive to electrical stimulation.
Shi30 et al. prepared a semi-IPN hydrogel based on bacterial cellulose nanofibers and sodium alginate. This semi-IPN is a double-stimulated drug release system. The system has the characteristics of swelling and drug release in response to pH and electric field stimuli. The swelling rate of sodium alginate bacterial cellulose increased from <8 times (pH 1.5) to >13 times when the pH value was 11.8. In addition, the swelling rate is impacted by the electric field. When the electric field changes from 0 to 0.5 V, the expansion rate of the semi-IPN system increases from 8 to 14 times in the dry state. This kind of IPN hydrogel uses the change in its swelling rate in the electric field to realize the electric field control of the drugs release. Researchers have used this semi-IP system to study the loading and release of the NSAID ibuprofen. The drug release can be controlled by deprotonation or protonation of sodium alginate in hydrogels under different pH conditions. The system demonstrates that the drug release is faster under alkaline conditions and slower under acidic conditions.
Salt/pH double-sensitive hydrogels
The characteristic of salt-sensitive hydrogels is that the swelling properties of the gel can undergo abrupt changes under the action of added salt. Salt is one of the most important substances for human survival, and can regulate the osmotic balance between cells and blood as well as normal water and salt metabolism.
Yin28 et al. synthesized an IPN super-porous hydrogel containing sodium alginate. The results show that these hydrogels have a large number of interconnected pores and IPNs at the same time. Their swelling rate is lower than that of super-porous hydrogels, with an increased sodium alginate/monomer ratio and lower. The in situ lactate dehydrogenase test was conducted on AD293 cells by the thiazole blue method, and morphological observation of the rat intestinal tissue was performed. The results showed that the polymer caused no obvious cell and mucosal damage to the rat intestinal tissue. The good news is that the rapid swelling of this hydrogel, superior mechanical properties, pH responsiveness, salt sensitivity and biocompatibility indicate that it is a potential candidate in the field of drug delivery systems.
Application of sodium alginate-based IPN sustained and controlled release hydrogels in pharmacy
Sodium alginate has the characteristics of degradability, good biocompatibility, non-toxicity, easy availability, low cost, and no immunogenicity, so that it has an excellent performance in the field of biomedicine. IPN sustained and controlled release hydrogels based on sodium alginate have been extensively studied for targeted drug and protein administration.
Delivery of small chemical drugs
Inherent pH sensitivity of sodium alginate makes it an ideal agent for colon-specific drug delivery. It has been used extensively as a drug carrier because of its ability to encapsulate and release multiple drugs in a gentle and biocompatible manner. After oral, subcutaneous, vascular, mucosal, pulmonary and transdermal administration of sodium alginate,70–76 biocompatibility in vivo has been verified. Sodium alginate-based IPN hydrogels can be used for continuous and local administration of traditional small chemical drugs. Traditional methods of administration (mostly oral administration) often result in the burst release of the drug, and poor targeting, leading to adverse effects and ineffectiveness. Oral preparations are now the most common and most preferred method in pharmaceutical applications. The design of oral dosage forms generally follows one of the following two principles: (1) the entire drug dose is in the same physical unit; or (2) the dose is encapsulated in an assembly of small subunits.77 In the latter case, the subunits are further compressed into tablets, thereby creating a barrier that can provide controlled release properties. The sodium alginate hydrocolloid immediately hydrates, forming a high viscosity layer around it, acting as a diffusion barrier, thereby reducing the migration of small chemical drugs and maintaining drug delivery. By adjusting the drug-sodium alginate interaction, the release kinetics of low molecular weight drugs in the sodium alginate gel can be monitored. When there is no chemical reaction between the drug and the polymer, the release mainly depends on the charge polarization of the molecule; that is, the hydrophilic molecule diffuses quickly, while the hydrophobic drug diffuses slowly through the pores of the gel.78 Table 1 summarizes the latest progress of sodium alginate-based IPN slow-release controlled hydrogels and their delivery in small chemical drugs.
Table 1 Sodium alginate-based IPN sustained and controlled release hydrogels and its latest progress in small chemical drug delivery
Route of administration |
Components of IPN |
Drugs |
Entrapment efficiency |
Oral |
Poly(vinyl alcohol), xanthan gum |
Norfloxacin |
83% (ref. 79) |
Locust bean gum |
Nimesulide |
74% (ref. 69) |
Locust bean gum, methacrylic acid |
Simvastatin |
70% (ref. 80) |
Polyacrylamide,Gum ghatti |
Ketoprofen |
90% (ref. 53) |
Locust bean gum |
Capecitabine |
81% (ref. 60) |
Carboxymethyl cellulose |
Melbine |
73% (ref. 81) |
N-Acryloyl-L-phenylalanine, ethylene glycol vinyl ether, hydroxyethyl acrylate |
Curcumin |
73% (ref. 82) |
Locust bean gum |
Capecitabine |
84% (ref. 83) |
Carboxymethyl locust bean gum |
Glipizide |
93% (ref. 84) |
O-Carboxymethyl tamarind gum |
Acyclovir |
70% (ref. 85) |
Functional modified guar gum |
Zidovudine |
68% (ref. 19) |
Modified karaya gum |
D-Penicillamine |
71% (ref. 86) |
Starch, poly(acrylic acid) |
Diclofenac sodium |
72% (ref. 87) |
Acrylic acid-divinyl benzene |
Ciprofloxacin |
92% (ref. 88) |
Karaya gum |
Fluorine formamide |
74% (ref. 89) |
Subcutaneously |
Poly(N-isopropyl acrylamide) |
Adriamycin |
—29 |
Poly(ethylene glycol) Methacrylate, N-isopropyl acrylamide |
Diclofenac sodium |
—90 |
Transdermal |
Poly(vinyl alcohol) |
Prazosin hydrochloride |
—46 |
It can be seen from the above table that oral dosing of the drug dosing way great proportion, because oral drug administration has the characteristics of convenience, economy and safety; however, short residence time of drugs in the gut limits the use of the oral route. The inherent pH responsiveness of sodium alginate makes them into an excellent agent for colon-specific drug delivery. The IPN between various functional polymers and sodium alginate gives hydrogels multiple responses, among which the temperature response is the most commonly used. They can be used as targeted control drug delivery systems. A large number of sodium alginate-based IPN hydrogels have been developed for controlled release of targeted drugs. The potential of sodium alginate IPN hydrogels as sustained drug delivery formulations has been demonstrated. Reports in the literature of alginate-based IPN hydrogels indicate that the drug release time can be extended from several hours to several days.
Protein delivery
Route of administration. The inside of sodium alginate is almost an inert aqueous environment. Its organic solvent-free encapsulation process and high gel porosity at room temperature can achieve a high diffusion rate of macromolecules. Not only that, the porosity can be easily controlled through a simple procedure, and the system can be biodegraded under normal physiological conditions. These unique characteristics make it a matrix for the encapsulation and delivery of multiple protein drugs.91 In order to realize the sustained release of protein drugs, the protein diffuses out via the porosity. There have been some reports on the controlled release of proteins in sodium alginate-based IPN hydrogels. Table 2 summarizes them.
Table 2 Sodium alginate-based IPN sustained and controlled release hydrogels and its latest progress in protein delivery
Route of administration |
Components of IPN |
Protein |
Entrapment efficiency |
Oral |
Sodium acrylate, N-vinylpyrrolidone |
BSA |
—92 |
L-Arginine |
BSA |
—93 |
Carboxymethyl chitosan |
BSA |
—16 |
Subcutaneously |
Poly(ethylene glycol) methacrylate, N-isopropyl acrylamide |
BSA |
—90 |
Dextran methacrylate derivative |
Myoglobin |
—94 |
Pulmonary |
Modified N-phthalyl chitosan |
BSA |
90% (ref. 95) |
As with IPN hydrogels for delivery of small chemotherapeutic agents, oral delivery is still the main route for protein drug delivery, but in the oral delivery route, the drug must overcome several obstacles before getting into the systemic circulation. In the gastrointestinal tract, protein drugs can be affected by digestive enzymes. Sodium alginate has strong biological adhesion, which can help the contact time between drugs and the conjunctival mucosa. Many unique characteristics make it a substrate for the encapsulation and delivery of various protein drugs. Bovine serum albumin has served as a model drug to evaluate the drug delivery effect of hydrogels. Surprisingly, the drug's release lasted for about 15 days. These hydrogel controlled-release proteins have been recorded to validate their effectiveness as a continuous protein delivery system for improving patient compliance.
Pharmaceutical excipients. Momoh and others96 conducted a study in which they delivered an IPN hydrogel dressing made of sodium alginate and glycerin containing therapeutic proteins to the wound, and found that the protein could be released for more than 72 h. This dressing also shows ideal moisture content, which is required for protein conformation, and shows a good balance between flexibility and toughness.
Tissue engineering. Pescosolido97 et al. have developed an IPN hydrogels based on a physical network of alginate, interpenetrated with a chemical one based on hydroxyethyl-methacrylate-derivatized dextran. In about 15 days, bovine serum albumin was gradually released from the hydrogel. They showed that these IPN hydrogels had the potential to serve as a scaffold for the proliferation and differentiation of encapsulated cells.
Conclusions
Alginate-based IPN hydrogels have been extensively used for the delivery of drugs and proteins or as basic materials for tissue repair and regeneration. They have potential research applications in the field of sustained and controlled drug release.
However, at present, most of the sodium alginate hydrogels are still limited to the theoretical research stage in the laboratory. Their actual application still needs to be improved considerably. Not only that, sodium alginate is highly hydrophilic, which results in poor sustained and controlled release of water-soluble drugs. Single-response and dual-response sodium alginate-based IPN hydrogels have been reported in a large number of studies, and most of them are only for one drug. Today, the treatment of diseases often involves the synergy of multiple drugs or some diseases are subject to a considerable variety of complications. The development of multiple-response intelligent sodium alginate hydrogels that can release multiple drugs accurately and in an orderly manner, and how to improve the targeting of drug-loaded hydrogels will be important directions in future research.
Conflicts of interest
The authors declare no conflict of interest.
Acknowledgements
We gratefully acknowledge the financial support of the Project of Enhancing School with Innovation of Guangdong provincial (530003002057), the Project of Zhanjiang city non-funded (2019B01049), the Project of Enhancing School with Innovation of Guangdong Ocean University (CXXL2019295), and the Project of Enhancing School with Innovation of Guangdong Ocean University (CXXL2019303).
Notes and references
- S. Li, S. Dong, W. Xu, S. Tu, L. Yan, C. Zhao, J. Ding and X. Chen, Adv. Sci., 2018, 5, 1700527 CrossRef.
- Y. Zhang, J. Yu, K. Ren, J. Zuo, J. Ding and X. Chen, Biomacromolecules, 2019, 20, 1478–1492 CrossRef CAS.
- C. Wang, N. Feng, F. Chang, J. Wang, B. Yuan, Y. Cheng, H. Liu, J. Yu, J. Zou and J. Ding, Adv. Healthcare Mater., 2019, 8, 1900312 CrossRef.
- D. S. Lee, J. I. Kang, B. H. Hwang and K. M. Park, Macromol. Res., 2019, 27, 205–211 CrossRef CAS.
- R. Na, Y. Liu, N. Lu, S. Zhang, F. Liu and G. Wang, Chem. Eng. J., 2019, 374, 738–747 CrossRef CAS.
- G. Wu, K. Jin, L. Liu and H. Zhang, Soft Matter, 2020, 16, 3319–3324 RSC.
- Y. Wang, Z. Jiang, W. Xu, Y. Yang, X. Zhuang, J. Ding and X. Chen, ACS Appl. Mater. Interfaces, 2019, 11, 8725–8730 CrossRef CAS.
- H. Qiu, H. Guo, D. Li, Y. Hou, T. Kuang and J. Ding, Trends Biotechnol., 2020, 38, 579–583 CrossRef CAS.
- S. Park, S. Edwards, S. Hou, R. Boudreau, R. Yee and K. J. Jeong, Biomater. Sci., 2019, 7, 1276–1280 RSC.
- J. Liao and H. Huang, Cellulose, 2020, 27, 825–838 CrossRef CAS.
- G. G. de Lima, J. K. Elter, B. S. Chee, W. L. E. Magalhães, D. M. Devine, M. J. Nugent and M. J. de Sá, Biomed. Mater., 2019, 14, 054101 CrossRef.
- T. Tominaga, V. R. Tirumala, E. K. Lin, J. P. Gong, H. Furukawa, Y. Osada and W.-l. Wu, Polymer, 2007, 48, 7449–7454 CrossRef CAS.
- Z. Shi, X. Gao, M. W. Ullah, S. Li, Q. Wang and G. Yang, Biomaterials, 2016, 111, 40–54 CrossRef CAS.
- Y. Jiang, Y. Wang, Q. Li, C. Yu and W. Chu, Curr. Med. Chem., 2019, 27, 2631–2657 CrossRef.
- C. Chang, B. Duan and L. Zhang, Polymer, 2009, 50, 5467–5473 CrossRef CAS.
- Y. Hu, J. Peng, L. Ke, D. Zhao, H. Zhao and X. Xiao, J. Polym. Res., 2016, 23, 129 CrossRef.
- A. Stanisci, A. Tøndervik, M. Gaardløs, A. Lervik, G. Skjåk-Bræk, H. Sletta and F. L. Aachmann, ACS Omega, 2020, 5, 4352–4361 CrossRef CAS.
- A. H. King, Food Hydrocolloids, 1983, 2, 115–188 CAS.
- S. Eswaramma and K. K. Rao, Carbohydr. Polym., 2017, 156, 125–134 CrossRef CAS.
- D. Myung, D. Waters, M. Wiseman, P. E. Duhamel, J. Noolandi, C. N. Ta and C. W. Frank, Polym. Adv. Technol., 2008, 19, 647–657 CrossRef CAS.
- C. Silan, A. Akcali, M. T. Otkun, N. Ozbey, S. Butun, O. Ozay and N. Sahiner, Colloids Surf., B, 2012, 89, 248–253 CrossRef CAS.
- K. Mallikarjuna Reddy, V. Ramesh Babu, K. Krishna Rao, M. Subha, K. Chowdoji Rao, M. Sairam and T. Aminabhavi, J. Appl. Polym. Sci., 2008, 107, 2820–2829 CrossRef.
- J. George, J. Onodera and T. Miyata, J. Biomed. Mater. Res., Part A, 2008, 87, 1103–1111 CrossRef.
- L. Alka, S. Garima, B. S. Sankar and V. Anurag, J. Drug Delivery, 2014, 2014, 1–11 Search PubMed.
- S. Ekici and D. Saraydin, Drug Delivery, 2004, 11, 381–388 CrossRef CAS.
- W. Wu and D.-s. Wang, React. Funct. Polym., 2010, 70, 684–691 CrossRef CAS.
- S. Mandal, S. K. Basu and B. Sa, Carbohydr. Polym., 2010, 82, 867–873 CrossRef CAS.
- L. Yin, L. Fei, C. Tang and C. Yin, Polymer International, 2007, 56, 1563–1571 CrossRef CAS.
- M. Liu, X. Song, Y. Wen, J.-L. Zhu and J. Li, ACS Appl. Mater. Interfaces, 2017, 9, 35673–35682 CrossRef CAS.
- X. Shi, Y. Zheng, G. Wang, Q. Lin and J. Fan, RSC Adv., 2014, 4, 47056–47065 RSC.
- K. Moebus, J. Siepmann and R. Bodmeier, Eur. J. Pharm. Sci., 2012, 45, 358–366 CrossRef CAS.
- K.-L. Deng, Y.-B. Gou, L.-R. Dong, Q. Li, L.-B. Bai, T. Gao, C.-Y. Huang and S.-L. Wang, Frontiers of Materials Science in China, 2010, 4, 353–358 CrossRef.
- S. Hua, H. Ma, X. Li, H. Yang and A. Wang, Int. J. Biol. Macromol., 2010, 46, 517–523 CrossRef CAS.
- A. C. Hodsdon, J. R. Mitchell, M. C. Davies and C. D. Melia, J. Controlled Release, 1995, 33, 143–152 CrossRef CAS.
- C. K. Kuo and P. X. Ma, Biomaterials, 2001, 22, 511–521 CrossRef CAS.
- R. Censi, P. Di Martino, T. Vermonden and W. E. Hennink, J. Controlled Release, 2012, 161, 680–692 CrossRef CAS.
- S. J. Bidarra, C. C. Barrias and P. L. Granja, Acta Biomater., 2014, 10, 1646–1662 CrossRef CAS.
- R. Schellekens, F. Stellaard, D. Mitrovic, F. Stuurman, J. Kosterink and H. Frijlink, J. Controlled Release, 2008, 132, 91–98 CrossRef CAS.
- A. Blandino, M. Macías and D. Cantero, Enzyme Microb. Technol., 2000, 27, 319–324 CrossRef CAS.
- T. W. Chung, J. Yang, T. Akaike, K. Y. Cho, J. W. Nah, S. I. Kim and C. S. Cho, Biomaterials, 2002, 23, 2827–2834 CrossRef CAS.
- M. Rajaonarivony, C. Vauthier, G. Couarraze, F. Puisieux and P. Couvreur, J. Pharm. Sci., 1993, 82, 912–917 CrossRef CAS.
- Y. S. Kim, H. W. Kim, S. H. Lee, K. S. Shin, H. W. Hur and Y. H. Rhee, Int. J. Biol. Macromol., 2007, 41, 36–41 CrossRef CAS.
- P. Sriamornsak, J. Nunthanid, M. Luangtana-anan and S. Puttipipatkhachorn, Eur. J. Pharm. Biopharm., 2007, 67, 227–235 CrossRef CAS.
- H. H. Tønnesen and J. Karlsen, Drug Dev. Ind. Pharm., 2002, 28, 621–630 CrossRef.
- M. Grassi, I. Colombo and R. Lapasin, J. Controlled Release, 2001, 76, 93–105 CrossRef CAS.
- R. V. Kulkarni, V. Sreedhar, S. Mutalik, C. M. Setty and B. Sa, Int. J. Biol. Macromol., 2010, 47, 520–527 CrossRef CAS.
- U. Bhutani, A. Laha, K. Mitra and S. Majumdar, Mater. Lett., 2016, 164, 76–79 CrossRef CAS.
- Y. Hu, S. Zhang, D. Han, Z. Ding, S. Zeng and X. Xiao, J. Polym. Res., 2018, 25, 148 CrossRef.
- K. Ramesh, M. Krishnapriya, A. Paul and C. N. Sreeja, Asian J. Pharm. Clin. Res., 2017, 10, 271–276 CrossRef CAS.
- Y. Fang, S. Al-Assaf, G. O. Phillips, K. Nishinari, T. Funami, P. A. Williams and L. Li, J. Phys. Chem. B, 2007, 111, 2456–2462 CrossRef CAS.
- M. Yonese, K. Baba and H. Kishimoto, Polym. J., 1992, 24, 395–404 CrossRef CAS.
- W. C. Jao, H. C. Chen, C. H. Lin and M. C. Yang, Polym. Adv. Technol., 2009, 20, 680–688 CrossRef CAS.
- R. Boppana, G. K. Mohan, U. Nayak, S. Mutalik, B. Sa and R. V. Kulkarni, Int. J. Biol. Macromol., 2015, 75, 133–143 CrossRef CAS.
- C. Wen, L. Lu and X. Li, Macromol. Mater. Eng., 2014, 299, 504–513 CrossRef CAS.
- H. Huang, X. Qi and Y. Chen, Saudi Pharm. J., 2019, 27, 990–999 CrossRef CAS.
- C. Spedalieri, C. Sicard, M. Perullini, R. Brayner, T. Coradin, J. Livage, S. A. Bilmes and M. Jobbágy, J. Mater. Chem. B, 2015, 3, 3189–3194 RSC.
- T. Ogoshi, Y. Takashima, H. Yamaguchi and A. Harada, J. Am. Chem. Soc., 2007, 129, 4878–4879 CrossRef CAS.
- I. Khalid, M. Ahmad, M. U. Minhas and K. Barkat, Polym. Bull., 2018, 75, 1075–1099 CrossRef CAS.
- S. Ganguly, P. P. Maity, S. Mondal, P. Das, P. Bhawal, S. Dhara and N. C. Das, Materials Science and Engineering: C, 2018, 92, 34–51 CrossRef CAS.
- M. Upadhyay, S. K. R. Adena, H. Vardhan, S. Pandey and B. Mishra, Drug Dev. Ind. Pharm., 2018, 44, 511–521 CrossRef CAS.
- S. Basu, H. S. Samanta and J. Ganguly, Soft Mater., 2018, 16, 7–19 CrossRef CAS.
- Y. Lu, A. A. Aimetti, R. Langer and Z. Gu, Nat. Rev. Mater., 2016, 2, 1–17 Search PubMed.
- C.-Y. Chen, T. H. Kim, W.-C. Wu, C.-M. Huang, H. Wei, C. W. Mount, Y. Tian, S.-H. Jang, S. H. Pun and A. K.-Y. Jen, Biomaterials, 2013, 34, 4501–4509 CrossRef CAS.
- K. N. Plunkett, K. L. Berkowski and J. S. Moore, Biomacromolecules, 2005, 6, 632–637 CrossRef CAS.
- N. Bhattarai, H. R. Ramay, J. Gunn, F. A. Matsen and M. Zhang, J. Controlled Release, 2005, 103, 609–624 CrossRef CAS.
- C. De las Heras Alarcón, S. Pennadam and C. Alexander, Chem. Soc. Rev., 2005, 34, 276–285 RSC.
- Y. Zhao, J. Kang and T. Tan, Polymer, 2006, 47, 7702–7710 CrossRef CAS.
- R. Jalababu, S. S. Veni and K. S. Reddy, J. Drug Delivery Sci. Technol., 2018, 44, 190–204 CrossRef CAS.
- C. Madhavi, P. Kumara Babu, Y. Maruthi, A. Parandhama, O. Sreekanth Reddy and K. Chowdoji Rao, Int. J. Pharmacol. Pharm. Sci., 2017, 9, 245–252 CrossRef CAS.
- M. Afshar, G. Dini, S. Vaezifar, M. Mehdikhani and B. Movahedi, J. Drug Delivery Sci. Technol., 2020, 56, 101530 CrossRef CAS.
- K. Campbell, D. Hadley, D. Kukis and E. Silva, PLoS One, 2017, 12, e0181484 CrossRef.
- J. Amirian, T. T. T. Van, S.-H. Bae, H.-I. Jung, H.-J. Choi, H.-D. Cho and B.-T. Lee, Int. J. Biol. Macromol., 2017, 105, 143–153 CrossRef CAS.
- H.-J. Kao, Y.-L. Lo, W.-J. Vong, Y.-J. Lin and H.-R. Lin, J. Biomater. Sci., Polym. Ed., 2006, 17, 1191–1205 CrossRef CAS.
- M. J. Martín, A. C. Calpena, F. Fernández, M. Mallandrich, P. Gálvez and B. Clares, Carbohydr. Polym., 2015, 117, 140–149 CrossRef.
- J. Du, PhD thesis, The University of Texas at Austin, 2015.
- P. Yuan, X. Qiu, R. Jin, Y. Bai, S. Liu and X. Chen, Biomater. Sci., 2018, 6, 820–826 RSC.
- K. Nahar, M. K. Hossain and T. A. Khan, J. Pharm. Sci. Res., 2017, 9, 606 CAS.
- A. D. Augst, H. J. Kong and D. J. Mooney, Macromol. Biosci., 2006, 6, 623–633 CrossRef CAS.
- K. Ghosal, S. Adak, C. Agatemor, G. Praveen, N. Kalarikkal and S. Thomas, J. Polym. Res., 2020, 27, 1–11 CrossRef.
- M. Chintha, S. R. Obireddy, P. Areti, S. Marata Chinna Subbarao, C. R. Kashayi and J. K. Rapoli, J. Dispersion Sci. Technol., 2019, 1–11 CrossRef.
- B. Y. Swamy and Y.-S. Yun, Int. J. Biol. Macromol., 2015, 77, 114–119 CrossRef CAS.
- V. P. Rudhrabatla, R. Jalababu, K. K. Rao and K. S. Reddy, J. Drug Delivery Sci. Technol., 2019, 51, 438–453 CrossRef.
- M. Upadhyay, S. K. R. Adena, H. Vardhan, S. K. Yadav and B. Mishra, Int. J. Biol. Macromol., 2018, 115, 907–919 CrossRef CAS.
- P. Dey, B. Sa and S. Maiti, Int. J. Biol. Macromol., 2015, 76, 176–180 CrossRef CAS.
- S. Jana, R. Sharma, S. Maiti and K. K. Sen, Int. J. Biol. Macromol., 2016, 92, 1034–1039 CrossRef CAS.
- O. S. Reddy, M. Subha, T. Jithendra, C. Madhavi and K. C. Rao, Polym. Polym. Compos., 2020, 0967391120904477 Search PubMed.
- A. Chang, Iran. Polym. J., 2015, 24, 161–169 CrossRef CAS.
- A. M. Rajesh, S. A. Bhatt, H. Brahmbhatt, P. S. Anand and K. M. Popat, Asian J. Pharm. Sci., 2015, 10, 331–340 CrossRef.
- G. Nagarjuna, P. K. Babu, Y. Maruthi, A. Parandhama, C. Madhavi, M. Subha and K. Chowdojirao, J. Appl. Pharm. Sci., 2016, 6, 011–019 CrossRef CAS.
- J. Zhao, X. Zhao, B. Guo and P. X. Ma, Biomacromolecules, 2014, 15, 3246–3252 CrossRef CAS.
- W. R. Gombotz and S. Wee, Adv. Drug Delivery Rev., 1998, 31, 267–285 CrossRef CAS.
- D. S. Lima, E. T. Tenório-Neto, M. K. Lima-Tenório, M. R. Guilherme, D. B. Scariot, C. V. Nakamura, E. C. Muniz and A. F. Rubira, J. Mol. Liq., 2018, 262, 29–36 CrossRef CAS.
- M. S. M. Eldin, E. A. Kamoun, M. A. Sofan and S. M. Elbayomi, Arabian J. Chem., 2015, 8, 355–365 CrossRef.
- P. Matricardi, M. Pontoriero, T. Coviello, M. A. Casadei and F. Alhaique, Biomacromolecules, 2008, 9, 2014–2020 CrossRef CAS.
- I. M. El-Sherbiny and H. D. Smyth, Int. J. Pharm., 2010, 395, 132–141 CrossRef CAS.
- F. U. Momoh, J. S. Boateng, S. C. Richardson, B. Z. Chowdhry and J. C. Mitchell, Int. J. Biol. Macromol., 2015, 81, 137–150 CrossRef CAS.
- L. Pescosolido, T. Vermonden, J. Malda, R. Censi, W. J. Dhert, F. Alhaique, W. E. Hennink and P. Matricardi, Acta Biomater., 2011, 7, 1627–1633 CrossRef CAS.
|
This journal is © The Royal Society of Chemistry 2020 |
Click here to see how this site uses Cookies. View our privacy policy here.