DOI:
10.1039/C4RA16493H
(Review Article)
RSC Adv., 2015,
5, 23810-23825
Recent progress in organic sensitizers for dye-sensitized solar cells
Received
16th December 2014
, Accepted 20th February 2015
First published on 20th February 2015
Abstract
Dye-sensitized solar cells (DSSCs) are fabricated using low-cost materials and a simple fabrication process; these advantages make them attractive candidates for research on next generation solar cells. In this type of solar cell, dye-sensitized metal oxide electrodes play an important role for achieving high performance since the porous metal oxide films provide large specific surface area for dye loading and the dye molecule possesses broad absorption covering the visible region or even part of the near-infrared (NIR). Recently, metal-free sensitizers have made great progress and become the most potential alternatives. This review mainly focuses on recent progress in metal-free sensitizers for applications in DSSCs. Besides, we also briefly report DSSCs with near-infrared (NIR) organic sensitizers, which provide the possibility to extend the absorption threshold of the sensitizers in the NIR region. Finally, special consideration has been paid to panchromatic engineering, co-sensitization, a key technique to achieve whole light absorption for improving the performance of DSSCs.
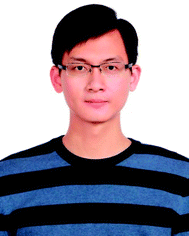 Chuan-Pei Lee | Chuan-Pei Lee received his PhD degree in Chemical Engineering at National Taiwan University in 2012. Currently, he is a post-doctoral research fellow in the Center for Condensed Matter Sciences at National Taiwan University. His research interests include solar energy and electrochemical energy materials/systems. Besides, he is also familiar with electrochemical analysis and microemulsion synthesis techniques. |
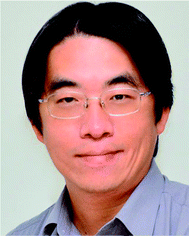 Shih-Sheng Sun | Shih-Sheng Sun completed his PhD (2001) in Supramolecular Chemistry at the State University of New York at Binghamton with Prof. Alistair J. Lees. After two-year postdoctoral studies at Northwestern University with Prof. Joseph T. Hupp and Prof. SonBinh T. Nguyen, he joined the Institute of Chemistry, Academia Sinica as an Assistant Research Fellow in 2003 and was promoted to Associated Research Fellow and Research Fellow in 2009 and 2014, respectively. His research interests focus on supramolecular materials chemistry and applications in optoelectronic materials. |
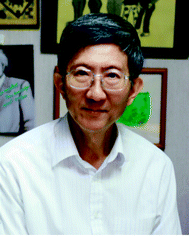 Jiann T. Lin | Jiann T. Lin received his BS degree in Chemistry from the National Tsing Hua University in 1975. He completed his PhD degree in the Chemistry Department of the University of Minnesota in 1984. After a year as a postdoctoral fellow in the Chemistry Department of the University of Michigan, he joined the Institute of Chemistry, Academia Sinica as a Research Fellow. He was a visiting scholar in Northwestern University in 1991. He is also Adjunct Professor of the National Central University. His current scientific interests involve the study of organic materials for organic light-emitting diodes, organic solar cells and two photon absorption dyes. |
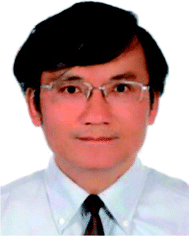 Kuo-Chuan Ho | Kuo-Chuan Ho received his BS and MS degrees in the Department of Chemical Engineering of National Cheng Kung University, Taiwan in 1978 and 1980, respectively. He received his PhD in Chemical Engineering at the University of Rochester, USA in 1986. Currently he is a Distinguished Professor jointly appointed by the Department of Chemical Engineering and the Institute of Polymer Science and Engineering at National Taiwan University. His research interests mainly surround applications of chemically modified electrodes to sensing and electro-optical devices, including dye-sensitized solar cells and electrochromic devices. |
1. Introduction
The increasing global need for energy coupled with the depletion of easily accessible, hence cheap, fossil fuel reserves, poses a serious threat to the human global economy in the near future.1 Considering in addition the harmful ecological impact of conventional energy sources, it becomes obvious that development of clean alternative energy sources is a necessity.2,3 Fortunately, the supply of energy from the sun to the earth is gigantic, i.e., 3 × 1024 joule a year or about ten thousand times more than what mankind consumes currently. This means that only 0.1% of the earth's surface covered with solar cells with an efficiency of 10% would be sufficient to satisfy our current needs.4 Therefore, solar power is considered to be one of the best sustainable energies for future generations. Current commercially available photovoltaic technologies are based on inorganic materials. Their fabrication requires high costs and large amounts of energy. In addition, they often use toxic and scarce materials. To date photovoltaics has been dominated by solid-state junction devices, usually in silicon, crystalline or amorphous, and profiting from the experience and materials availability resulting from the semiconductor industry. However, the energy-intensive high-temperature and expensive high-vacuum processes are needed for the silicon based solar cells. Therefore, the dominance of the photovoltaic field by such kind of inorganic solid-state junction devices is now being challenged by the emergence of the third generation solar cells based on interpenetrating network structures, such as dye-sensitized solar cells (DSSCs).4,5
As shown in Fig. 1, the DSSC device is composed of three adjacent thin layers, including a high band-gap nanocrystalline semiconductor-based mesoporous thin film adsorbed with a dye sensitizer on the working electrode for the absorption in the visible region, a platinized counter electrode for the collection of electrons and a redox electrolyte, sandwiched in between the two electrodes. The usual choice for the semiconductor material is titanium dioxide (TiO2), whereas ruthenium bipyridyl derivatives (N3 (No. 1), N719 (No. 2) and black dye (No. 3) etc.) are for the dye sensitizers. The electrolyte mostly contains I−/I3− redox couple, which was obtained by mixing iodine (I2) and inorganic or organic iodides in suitable non-aqueous solvents. Upon absorption of light, an electron is injected from a metal-to-ligand charge transfer excited state of the dye into the conduction band of the metal oxide. The rate of this electron injection reaction is ultrafast, typically occurring on the order of hundreds of femtoseconds to tens of picoseconds. The injected electron percolates through the TiO2 film, and is thought to move by a “hopping” mechanism driven by a chemical diffusion gradient (rather than an electric field), and is collected at a transparent conductive substrate of fluorine-doped tin oxide glass (SnO2:F), on which the TiO2 film is formed. After passing through an external circuit, the electron is reintroduced into the solar cell at a platinum counter electrode, where the triiodide is reduced to iodide. The iodide then regenerates the oxidized dye, thereby completing the circuit with no net chemical change.
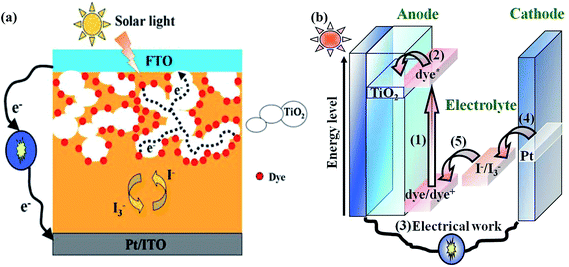 |
| Fig. 1 (a) Schematic of a typical DSSC. (b) The basic sequence of events in a DSSC. | |
Since Grätzel and coworkers introduced the nanoporous films into dye-derived wideband semiconductor research and made a breakthrough in the photoelectric conversion efficiency (η) of DSSCs, academic and commercial interests have been focused on DSSCs for their high η, potential low-cost and simple assembly technology.5 This became especially noticeable when DSSCs with the η of greater than 10% were demonstrated by using ruthenium (Ru) complexes as the sensitizers (Fig. 2).6–10 Among them, the η of DSSCs with N719, black dye, PRT-22 (No. 4), and CYC-B11 (No. 5) dyes were improved to 11.18%,8 11.10%,11 11.16%,12 and 11.50%,10 respectively.
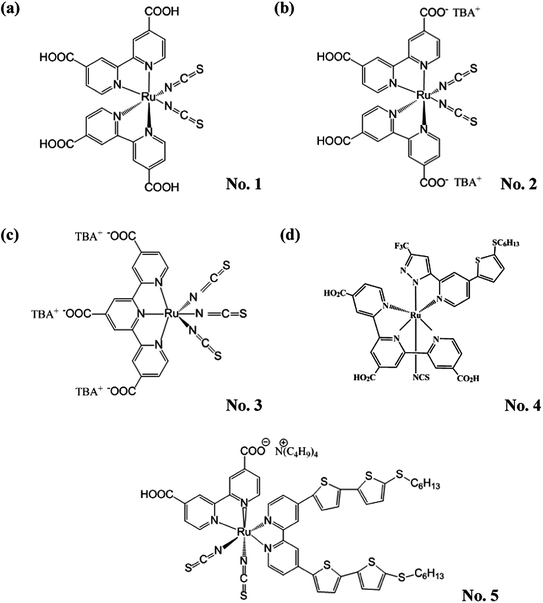 |
| Fig. 2 The structures of partial ruthenium (Ru) complexes. (a) N3 (No. 1), (b) N719 (No. 2), (c) black dye (No. 3), (d) PRT-22 (No. 4) and (e) CYC-B11 (No. 5). | |
Although the Ru-dyes have leading performance in η compared to other dyes, higher cost of Ru metal and lower extinction coefficient (usually < 2.50 × 104 M−1 cm−1 in the visible region) have limited the developing potentials of the DSSCs based on Ru-dyes. Therefore, the development of Ru-free dyes has become an attractive topic for DSSCs since they usually have much higher extinction coefficient (usually > 2.50 × 104 M−1 cm−1 in the visible region) and they can be prepared and purified in an easier and more economical way than their Ru counterparts. Compared to the Ru-dyes, the higher extinction coefficient of Ru-free dyes allows the use of much thinner TiO2 films. It is thus possible to minimize the charge transport losses and achieve a better pore filling for solid-state electrolyte of DSSCs. Recently, the DSSC with the Co(II/III) tris(bipyridyl)-based redox electrolyte has achieved a 12.30% efficiency by incorporating the novel zinc porphyrin dye (YD2-o-C8, No. 6) and co-sensitizing with another organic dye (Y123, No. 7) (Fig. 3).13 Based on the same electrolyte, the DSSC using the zinc porphyrin dye, SM315 (No. 8) reaches the record high η of 13.00% (Fig. 3).14 With these remarkable features, the Ru-free dyes have attracted considerable attention in recent years. Several review literatures are intended to explore the design strategy of these high efficient Ru-free dyes. For instance, Li et al. systematically reviewed the progress of porphyrin dyes of varied kinds, and the corresponding derivatives.15 Among the Ru-free dyes, the metal-free sensitizers (also called organic dyes) caught a lot of attention due to their possibly low cost, good structural design flexibility, and extremely high molar extinction coefficients of the charge transfer band.16 Liang et al. reported the design strategies of arylamine dyes containing various moieties; they also reported that the molecular structure contributes significantly to the performance of organic dyes.17
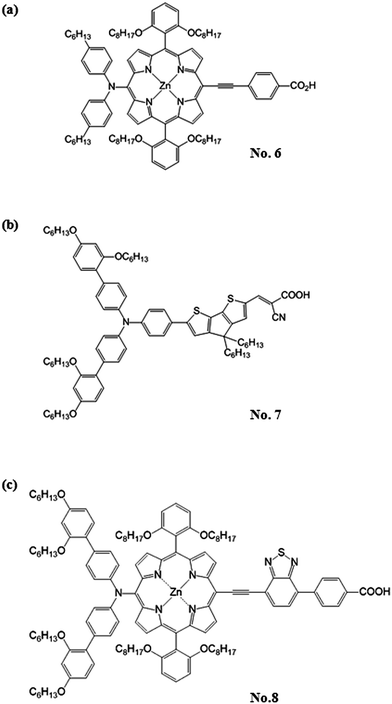 |
| Fig. 3 The structure of (a) YD2-o-C8 sensitizer (No. 6), (b) Y123 sensitizer (No. 7) and (c) SM315 sensitizer (No. 8). | |
This review mainly focuses on recent progresses in metal-free sensitizers for applications in DSSCs, especially published from 2010 to date. We also briefly report on notable near-infrared (NIR) organic sensitizers for DSSCs, which provide the possibility to extend the absorption threshold of the sensitizers in the NIR regions. Finally, special consideration has been paid to the panchromatic engineering via co-sensitization; a key technique to achieve whole light absorption for improving the performance of DSSCs.
2. Organic sensitizers for TiO2-based DSSCs
The organic sensitizer usually consists of an electron donor and an electron acceptor linked covalently through a π-conjugated spacer (Fig. 4). Acid ligands are generally used both as the electron acceptor and as the anchoring group for loading on the TiO2 surface. The π-conjugated spacer provides a bridge for electron transfer between the donor and the acceptor groups. The expansion of the structure of the π-conjugated spacer, which is the key part incorporated between the electron donor and acceptor, can induce a shift of both the highest occupied molecular orbital (HOMO) and the lowest unoccupied molecular orbital (LOMO) levels, resulting in tunable photophysical properties. Under illumination (Fig. 4), the intramolecular charge transfer occurs from the donor to the acceptor moiety through the π-conjugated spacer, which is beneficial for electron injection when dye molecule is adsorbed on the TiO2 surface. Due to the high structural tunability, the donor–π–acceptor based metal-free dyes are considered as one of the most promising sensitizers for DSSCs. Extending the absorption of the organic dyes to NIR region is possible with appropriate design of the donor–π–acceptor motif.
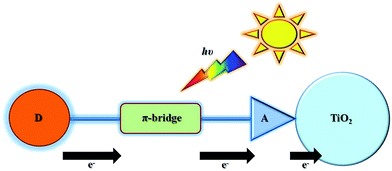 |
| Fig. 4 Schematic drawing of donor–π-bridge–acceptor organic sensitizers and their electron transfer processes under illumination. | |
In this section, we firstly review several notable examples of organic sensitizers with a π-conjugated spacer containing fluorene fragment (Fig. 5) and then put more effort on the literatures associated with the organic sensitizers with a π-conjugated spacer containing electron-deficient moieties (Fig. 6) since they usually possess more red-shifted absorption in the visible region than that of the former type. Their photovoltaic parameters are listed in Tables 1 and 2.
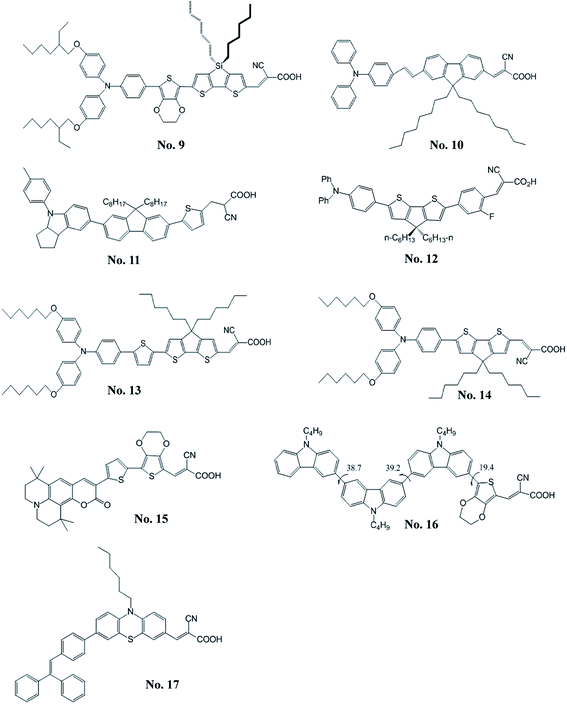 |
| Fig. 5 The structure of metal-free organic sensitizers with π-conjugated spacer containing fluorine substituent. | |
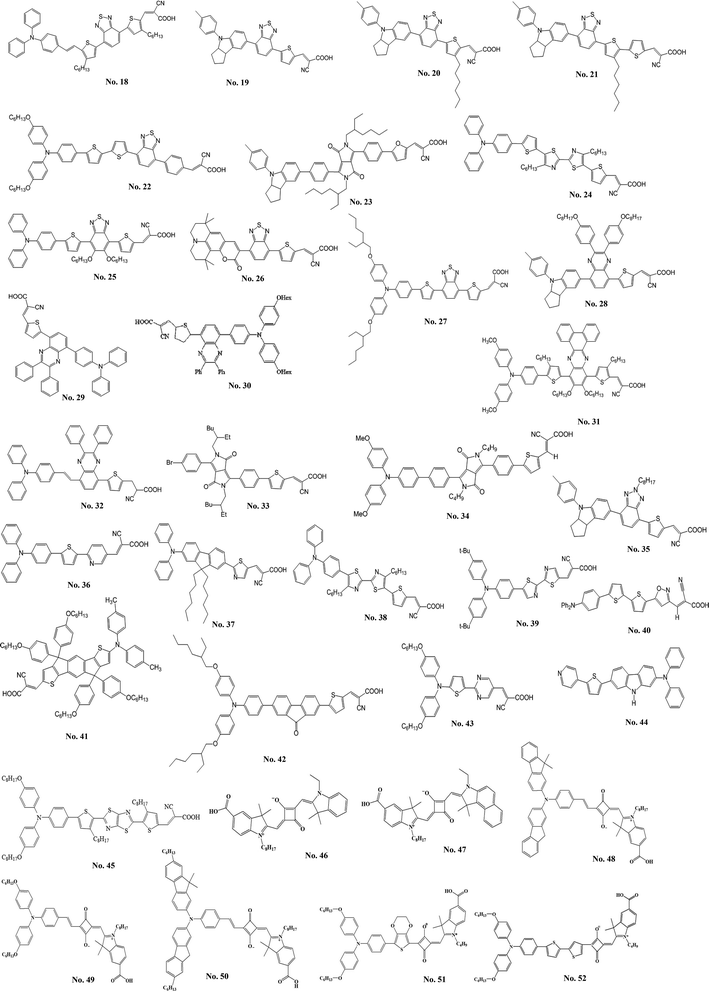 |
| Fig. 6 The structure of metal-free organic sensitizers with π-conjugated spacer containing electron-deficient moieties. | |
Table 1 The photovoltaic parameters of DSSCs with metal-free organic dyes with π-conjugated spacer containing fluorine substituent
Dye number (code; max. molar extinction coefficient (103 M−1 cm−1), wavelength (nm)) |
Electrolyte (redox couple) |
JSC (mA cm−2) |
VOC (mV) |
FF |
η (%) |
Ref. |
9 (C219; 57.5, 493) |
Liquid (I−/I3−) |
17.94 |
770 |
0.73 |
10.10 |
18 |
10 (B1; 38, 432) |
11.33 |
860 |
0.57 |
5.56 |
19 |
11 (S3; 30, 433) |
8.23 |
727 |
0.71 |
4.26 |
20 |
12 (A2-F; 53.74, 460) |
Solid (spiro-MeOTAD) |
7.52 |
910 |
0.71 |
4.86 |
21 |
13 (C229; 55, 575) |
Liquid (Co(II)/Co(III)) |
15.31 |
850 |
0.73 |
9.40 |
22 |
14 (C218; 58.9, 517) |
13.30 |
950 |
0.74 |
9.30 |
23 |
15 (HKK-CM1; 52.7, 532) |
Liquid (I−/I3−) |
14.20 |
600 |
0.70 |
6.07 |
24 |
16 (3C; 34.4, 444) |
11.67 |
796 |
0.68 |
6.33 |
25 |
17 (VP3; 20, 474) |
12.62 |
789 |
0.63 |
6.29 |
26 |
Table 2 The photovoltaic parameters of DSSCs with metal-free organic dyes with π-conjugated spacer containing electron-deficient moieties
Dye number (code; max. molar extinction coefficient (103 M−1 cm−1), wavelength (nm)) |
Electrolyte (redox couple) |
JSC (mA cm−2) |
VOC (mV) |
FF |
η (%) |
Ref. |
18 (BzTCA; 46.77, 557) |
Liquid (I−/I3−) |
16.46 |
545 |
0.67 |
6.04 |
27 |
19 (WS-2; 16.7, 533) |
17.70 |
650 |
0.76 |
8.70 |
28 |
20 (WS-6; 23.5, 547) |
15.00 |
672 |
0.77 |
7.76 |
29 |
21 (WS-9; 20.8, 536) |
18.00 |
696 |
0.72 |
9.04 |
30 |
22 (2; 29.4, 515) |
18.47 |
640 |
0.69 |
8.21 |
31 |
23 (YCD01; 46, 526) |
13.40 |
760 |
0.73 |
7.43 |
32 |
24 (BT-I; 40.7, 470) |
15.69 |
778 |
0.61 |
7.51 |
33 |
25 (R4; 25.5, 501) |
15.20 |
670 |
0.66 |
6.72 |
34 |
26 (HKK-CM4; 31, 507) |
14.30 |
580 |
0.72 |
5.97 |
35 |
27 (HKK-BTZ4; 31, 542) |
17.90 |
620 |
0.66 |
7.30 |
36 |
28 (IQ2; 21.9, 523) |
15.65 |
776 |
0.70 |
8.50 |
37 |
29 (6; 22, 460) |
15.40 |
700 |
0.68 |
7.35 |
38 |
30 (CR204; 21, 477) |
14.87 |
666 |
0.66 |
6.49 |
39 |
31 (LI-39; 46.2 & 32.1, 417 & 516) |
14.40 |
740 |
0.67 |
7.18 |
40 |
32 (RC-22; 22, 490) |
11.40 |
660 |
0.74 |
5.56 |
41 |
33 (DPP1; 21 & 14, 403 & 493) |
9.71 |
625 |
0.74 |
4.47 |
42 |
34 (DPP-I; 42.1, 524) |
9.78 |
605 |
0.69 |
4.14 |
43 |
35 (WS-5; 19.2, 496) |
13.18 |
780 |
0.78 |
8.02 |
44 |
36 (Y4; 25.1436) |
12.33 |
630 |
0.68 |
5.27 |
45 |
37 (6; 51.3, 448) |
14.55 |
710 |
0.67 |
6.88 |
46 |
38 (T1; 34.4, 457) |
11.78 |
810 |
0.60 |
5.73 |
47 |
39 (Alko1; 28.1, 450) |
13.20 |
612 |
0.69 |
5.56 |
48 |
40 (LJ6; 38.82, 416) |
8.04 |
662 |
0.62 |
3.30 |
49 |
41 (DTInDT; 27.87, 500) |
13.54 |
690 |
0.71 |
6.70 |
50 |
42 (HIQF1; 53.2 & 8.2, 396 & 484) |
12.26 |
700 |
0.73 |
6.26 |
51 |
43 (OHexDPTP; 29.3, 506) |
13.79 |
770 |
0.72 |
7.64 |
52 |
44 (NI5; 48.1, 394) |
5.80 |
540 |
0.60 |
1.89 |
53 |
45 (FNE74; 45.2, 489) |
14.48 |
568 |
0.62 |
5.10 |
54 |
2.1 Spacer containing fluorene fragments
Zeng et al.18 reported a high molar extinction coefficient (5.75 × 104 M−1 cm−1 at 493 nm) organic dye (C219, No. 9) containing a binary π-conjugated spacer of ethylenedioxythiophene and dithienosilole; the binary spacer aparts from the blocks of a lipophilic alkoxyl-substituted triphenylamine and a hydrophilic cyanoacrylic acid. The DSSC with this amphiphilic sensitizer reached a new milestone of 10.10% efficiency for the development of metal-free sensitizers. Zhou et al.19 indicated that the organic sensitizers with the appropriate π-conjugated spacer is important for improving the performance of DSSCs. Therefore, they synthesized a series of new dipolar organic dyes (B0–B2) containing the fluorenevinylene spacer. They found that the DSSC with B1 dye (No. 10) having optimal length of π-conjugated spacer shows the best η of 5.56% and the open-circuit voltage (VOC) could achieve 860 mV. Li et al.20 developed a series of novel organic sensitizers (S1–S4) containing aniline derivatives as the electron donor and a thienylfluorene as the spacer. Among these dyes, S3 (No. 11), utilizing a methyl-substituted indoline moiety as the electron donor, shows a high VOC of 727 mV and an η of 4.26%. They found that the better electron-donating indoline unit led to relatively high molar extinction of the absorption band and showed better photovoltaic performance. Moreover, the incorporation of the octyl-substituted fluorene unit inhibits the aggregation of organic sensitizer and results in relatively high VOC. Chen et al.21 reported the sensitizer, A2-F (No. 12), for fabricating the solid-state DSSC with spiro-MeOTAD. The A2-F comprises the 4,4′-dihexylcyclopenta[2,1-b:3,4-b]dithiophene and an additional phenylene group. The A2-F possesses a remarkably high absorption extinction coefficient of 5.37 × 104 M−1 cm−1 at 460 nm. The all-solid-state DSSC with A2-F exhibited good photovoltaic parameters; η, 4.86%; short-circuit current density (JSC), 7.52 mA cm−2; VOC, 910 mV; fill factor (FF), 0.71. Bai et al.22 synthesized the dye, C229 (No. 13), by incorporating the 2,6-bis(thiophen-2-yl)-4,4-dihexyl-4H-cyclopenta[2,1-b:3,4-b′]dithiophene as the π-spacer that exhibits an excellent η of 9.40% with Co(II/III) tris(bipyridyl)-based redox electrolyte. Furthermore, Xu et al.23 synthesized C218 (No. 14) sensitizer containing dihexyloxy-substituted triphenylamine. The DSSC based on light-harvesting C218 sensitizer and Co(II/III) tris(bipyridyl)-based redox electrolyte can achieve an η of 9.30%. A series of coumarin sensitizers containing an electron-deficient entity, ethylenedioxythiophene (EDOT), was reported by Seo et al.24 The HKK-CM1 (No. 15) sensitizer shows the best photovoltaic performance with JSC of 14.20 mA cm−2, VOC of 600 mV, and a FF of 0.70, corresponding to an η of 6.07%. Lai et al.25 synthesized a sensitizer with multi-carbazole units (3C, No. 16) that exhibited twisted and zigzag-shape structure. The non-planar structure of 3C which combined with multiple alkyl chains could efficiently inhibit dye aggregation and charge recombination. It showed a high VOC of 796 mV and an η of 6.33%. Chen et al.26 synthesized a series of organic sensitizers with polyphenyl-substituted ethylene end-capped groups for DSSCs. The VOC was significantly affected by the end-capped groups (diphenylethylene, triphenylethylene, and tetraphenylethylene). The VP3 (No. 17) which incorporated the triphenylethylene exhibits a high VOC of 789 mV and the highest η of 6.29%.
2.2 Spacer containing electron-deficient moieties
Tang et al.27 developed a novel benzothiadiazole containing organic sensitizer (BzTCA, No. 18). The photophysical property of BzTCA indicates that its absorption exhibits a wide coverage of the visible region. Its absorption features commence at about 800 nm on the TiO2 film and it possesses a maximum absorption at 587 nm. The DSSC with BzTCA achieved an η of 6.04%. Zhu et al.28 incorporated the electron-withdrawing entity, benzothiadiazole, in the organic sensitizer (WS-2, No. 19). The DSSC with WS-2 exhibited broad action spectrum, which keeps a high plateau at visible region until 720 nm and extends the onset to the NIR region at about 850 nm, exhibited a high η of 8.70% (JSC = 17.70 mA cm−2, VOC = 650 mV, and FF = 0.76) with the co-adsorption of DCA. The same group also developed the WS-6 (No. 20) based on the WS-2 by incorporating an n-hexyl chain onto the thiophene unit.29 The η of 7.76% was achieved by using the WS-6 sensitizer along without co-adsorbed DCA. Furthermore, the WS-9 (No. 21) has an extra n-hexylthiophene unit in the π-conjugated spacer compared to WS-2.30 Co-sensitized DSSCs based on WS-9 and DCA had an η of 9.04% (JSC of 18.00 mA cm−2 and VOC of 696 mV). The sensitizer (2, No. 22) using benzothiadiazole (BTDA)/cyanoacrylic acid as the acceptor developed by Haid et al.31 The insertion of an additional phenyl ring significant improves the η (8.21%) by over 6.5 times compared to the sensitizer without phenyl unit (1.24%). The YCD01 (No. 23) including the 2-ethyl-hexyl chain in the diketopyrrolopyrroles moiety was reported by Qu et al.32 It showed a high η of 7.43% with a JSC of 13.40 mA cm−2, VOC of 760 mV, a FF of 0.73 and an excellent stability. The sensitizers comprising bithiazole unit were developed by He's group.33 The BT-I dye (No. 24), with bithiazole motifs flanked by a thiophene and triphenylamine, exhibited an η of 7.51% (JSC = 15.69 mA cm−2, VOC = 778 mV, FF = 0.61). The dipolar sensitizer (R4, No. 25) containing a 5,6-bis-hexyloxy-benzo[2,1,3]thiadiazole entity in the π-conjugated spacer was synthesized by Chou et al.34 The R4 showed a high JSC (15.20 mA cm−2). Seo et al.35 developed a coumarin-based sensitizer containing an electron-deficient benzothiadiazole. The HKK-CM4 (No. 26) sensitizer showed an η of 5.97% with the co-adsorption of DCA. Lee et al.36 synthesized the HKK-BTZ4 dye (No. 27) containing benzothiadiazole moiety as the electron acceptor. An η of 7.30% was achieved with the DSSC based on HKK-BTZ4. Pei et al.37 employed the quinoxaline moiety as the π-conjugated spacer for an electron-withdrawing organic sensitizer (IQ2, No. 28). The IQ2 exhibited a high η of 8.50% with JSC = 15.65 mA cm−2, VOC = 776 mV, a FF = 0.70. Li et al.38 reported an easily prepared organic dye (6, No. 29), comprised an electron-deficient diphenylquinoxaline moiety integrated in the π-conjugated spacer between the electron donor and acceptor moieties. The sensitizer 6 produced a good η of 7.35%. The electron-deficient diphenylquinoxaline moiety effectively reduces the energy gap of the dyes and broadly extends the spectral coverage. Therefore, they reported another organic sensitizer (CR204, No. 30) containing an additional phenyl group between the diarylamine donor and the diphenylquinoxaline π-spacer to achieve an η of 6.49%.39 The LI-39 (No. 31) exploited 11,12-bishexyloxy dibenzo[a,c]phenazine unit as an auxiliary acceptor in the π-conjugated spacer was reported by Shi et al.40 The LI-39 shows an η of 7.18% without any co-adsorbent. Chang et al.41 also explored the quinoxaline-based sensitizer (RC-22, No. 32) that led to an η of 5.56%. The organic sensitizers with highly stable diketopyrrolopyrrole skeleton were investigated by Warnan et al.42 The electronic communication of the synthesized dyes with TiO2 can be tuned by varying the anchoring groups from a cyanoacrylic acid to a rhodanine acid. Among them, chenodeoxycholic acid proved to be useful in limiting the aggregation of DPP1 (No. 33), resulting in a better performance of 4.47% as compared to rhodanine acid-based sensitizer (0.44%) under the same experimental conditions. Qu et al.43 studied the diketopyrrolopyrrole-based sensitizer (DPP-I, No. 34). The DSSC based on DPP-I showed a JSC of 9.78 mA cm−2, a VOC of 605 mV, and a FF of 0.69 to achieve an η of 4.14%. Cui et al.44 synthesized the indoline-based sensitizer (WS-5, No. 35) by incorporating benzotriazole unit with octyl group into its π-conjugated spacer. The octyl group on the benzotriazole unit shows the good ability to suppress charge recombination rate resulting in a good performance of 8.02% and a good VOC of 780 mV for the DSSC. Chou et al.45 synthesized the dipolar sensitizer, Y4 (No. 36), incorporating the 2,5-pyridyl entity. The corresponding DSSC with Y4 exhibited an η of 5.27%. Chen et al.46 used the heteroaromatic ring as the conjugating bridge of the sensitizer (6, No. 37), which exhibited very high molar extinction coefficient (5.13 × 104 M−1 cm−1 at 448 nm) in the absorption spectra. The corresponding DSSC achieved an η of 6.88% (JSC = 14.55 mA cm−2, VOC = 710 mV, and FF = 0.67). He et al.47 designed the T1 (No. 38), which comprises two hexyl chains substituted bithiazole. It exhibited an η of 5.73% with JSC = 11.78 mA cm−2, VOC = 810 mV, and FF = 0.60. Chen et al.48 synthesized the 3-(5′-(4-(bis(4-tert-butylphenyl)amino)phenyl)-2,2′-bithiazol-5-yl)-2-cyanoacrylic acid (Alko1, No. 39) for the DSSC. The DSSC with Alko1 achieved an η of 5.56% (JSC = 13.20 mA cm−2, VOC = 612 mV, and FF = 0.69). Li et al.49 synthesized a new organic sensitizer, 2-cyano-3-(5-(5′-(4-(diphenylamino)phenyl)-2,2′-bithiophen-5-yl) isoxazol-3-yl) acetic acid (LJ6, No. 40), for the application in DSSC, and the DSSC with LJ6 reached an η of 3.30%. Chen et al.50 reported an indacenodithiophene based sensitizer, DTInDT (No. 41), which was functionalized with 4-(n-hexoxy)phenyl groups on the sp3-carbon bridge. The DTInDT showed an η of 6.70% (JSC = 13.54 mA cm−2, VOC = 690 mV, and FF = 0.71). Qin et al.51 explored the novel fluorenone-based sensitizer, HIQF1 (No. 42), in which fluorenone was used as an additional acceptor. They studied the photovoltaic performance of the DSSC with HIQF1 and achieved a good η of 6.26% (JSC = 12.26 mA cm−2, VOC = 700 mV, and FF = 0.73). Lin et al.52 reported the OHexDPTP dye (No. 43), in which diphenylthienylamine donor with two hexyloxy chains and cyanoacrylic acid acceptor are bridged by an electron-deficient pyrimidine ring. The DSSC employing sensitizer
OHexDPTP exhibited high η of 7.64% (JSC = 13.79 mA cm−2, VOC = 770 mV, and FF = 0.72). Ooyama et al.53 proposed to use a pyridine ring as an electron-withdrawing and anchoring group in place of a conventional carboxylate group. Thus, they synthesized [9-butyl-7-(5-pyridin-4-yl-thiophen-2-yl)-9H-carbazol-2-yl]-diphenyl-amine (NI5, No. 44) for the application in the DSSC. It exhibited an η of 1.89% (JSC = 5.80 mA cm−2, VOC = 540 mV, and FF = 0.60). Zhang et al.54 developed the FNE74 dye (No. 45) incorporating a thiazolo[5,4-d]thiazole unit in the π-spacer, and the quasi-solid-state DSSC based on FNE74 exhibited a good η of 5.10%.
3. Near-infrared organic sensitizers
In contrast to Si-based solar cells, conventional sensitizers for DSSCs usually do not have a light absorption up to 900 nm. To generate a large photocurrent response and improve the η, it is necessary to extend the absorption threshold of the sensitizers in the visible and red/near infrared light (NIR) regions, which is 45% of the total solar energy. The theoretical calculation has proved that the η of DSSCs will be greatly improved with a broadened absorption spectrum.55 In this section, we briefly report on near-infrared (NIR) organic sensitizers (Fig. 7) for DSSCs, which provide the possibility to extend the absorption threshold of the sensitizers in the NIR regions. Their photovoltaic parameters are listed in Table 3. The development of organic molecules that enables efficient conversion of light in the red/NIR region is a research field of great activity. Much attention has been devoted to the synthesis of squarylium,56,57 cyanine,58 perylene,59 porphyrin,60 and phthalocyanine sensitizers.61
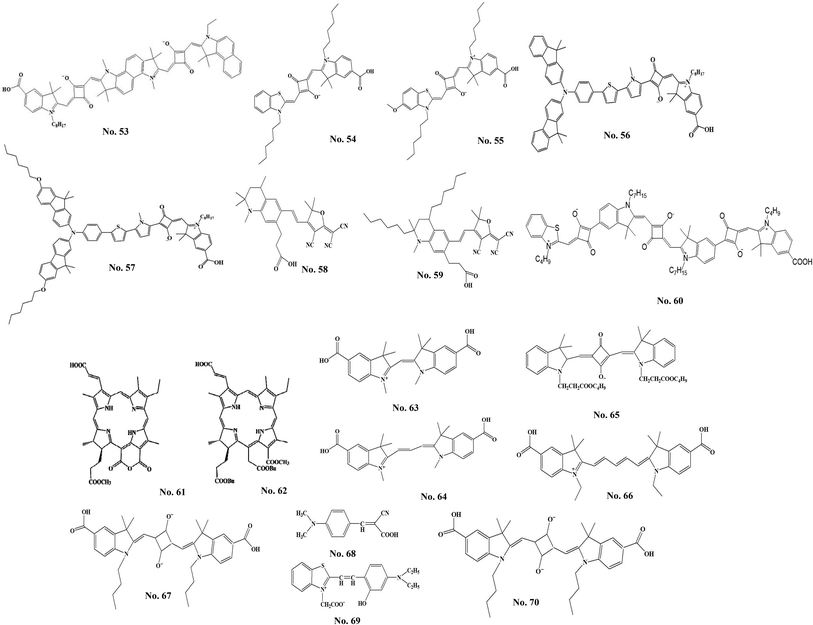 |
| Fig. 7 The structure of metal-free NIR organic sensitizers. | |
Table 3 The photovoltaic parameters of DSSCs with metal-free NIR organic sensitizers
Dye number (code; max. molar extinction coefficient (103 M−1 cm−1), wavelength (nm)) |
Electrolyte (redox couple) |
JSC (mA cm−2) |
VOC (mV) |
FF |
η (%) |
Ref. |
46 (SQ01; 292, 647) |
Solid (spiro-MeOTAD) |
10.50 |
600 |
0.71 |
4.50 |
62 |
47 (SQ02; 319, 662) |
11.30 |
670 |
0.72 |
5.40 |
62 |
48 (JK-64; 6.62 & 43, 470 & 656) |
Liquid (I−/I3−) |
13.60 |
493 |
0.70 |
4.70 |
63 |
49 (JK-65; 2.8 & 54, 460 & 667) |
7.50 |
500 |
0.72 |
2.70 |
63 |
50 (JK-64Hx; 7.86 & 55, 472 & 662) |
12.17 |
509 |
0.79 |
4.91 |
63 |
51 (JYL-SQ5; 109, 691) |
11.12 |
420 |
0.56 |
2.61 |
64 |
52 (JYL-SQ6; 75, 650) |
9.40 |
430 |
0.58 |
2.34 |
64 |
53 (BSQ01; 389, 730) |
N.A. |
3.10 |
550 |
0.76 |
1.30 |
66 |
54 (SK-11; 118.43, 640) |
Solid (spiro-MeOTAD) |
2.00 |
630 |
0.67 |
0.85 |
67 |
55 (SK-12; 144.37, 648) |
1.30 |
660 |
0.66 |
0.55 |
67 |
56 (JK-216; 93.4, 669) |
Liquid (I−/I3−) |
13.90 |
610 |
0.74 |
6.29 |
68 |
57 (JK-217; 77.9, 672) |
13.80 |
610 |
0.73 |
5.99 |
68 |
58 (HY103; 66.11, 610) |
11.80 |
460 |
0.67 |
3.70 |
69 |
59 (HY113; 88.87, 615) |
13.40 |
520 |
0.73 |
5.10 |
70 |
60 (TSQb; 347, 848) |
8.89 |
450 |
0.61 |
2.43 |
71 |
61 (H2P; 130 & 24.5 & 40, 410 & 545 & 700) |
0.48 |
330 |
0.65 |
0.10 |
72 |
62 (Chlorin e6; N.A.) |
15.60 |
650 |
0.66 |
6.70 |
73 |
Squaraines are well-known for their intense absorption in the red/NIR regions. Geiger et al.62 developed unsymmetrical squaraine sensitizer, 5-carboxy-2-[[3-[(1,3-dihydro-3,3-dimethyl-1-ethyl-2H-indol-2-ylidene)methyl]-2-hydroxy-4-oxo-2-cyclobuten-1-ylidene]methyl]-3,3-trimethyl-1-octyl-3H-indolium (SQ01, No. 46), which possesses intense absorption in the far red domain accompanied with high monochromatic external quantum efficiencies in DSSCs. 5-Carboxy-2-[[3-[(2,3-dihydro-1,1-dimethyl-3-ethyl-1H-benzo[e]indol-2-ylidene)methyl]-2-hydroxy-4-oxo-2-cyclobuten-1-ylidene]methyl]-3,3-dimethyl-1-octyl-3H-indolium (SQ02, No. 47) designed by slight modification of molecular structure of SQ01 reveals an η increment by approximately 20%. Both sensitizers show intense narrow absorption bands. However, the absorption maximum of SQ02 (662 nm) is slightly red shifted compared with that of SQ01 (647 nm) due to a more extended π-conjugated spacer in the former. Choi et al.63 designed and synthesized three unsymmetrical squaraine dyes, JK-64 (No. 48), JK-65 (No. 49), and JK-64Hx (No. 50), containing a bulky spirobifluorene or hexyloxyphenyl unit. The optimized cell of JK-64Hx gave a JSC of 12.82 mA cm−2, a VOC of 540 V and a FF of 0.75, corresponding to an η of 5.20%. The sensitized TiO2 films show an extra new absorption band in the high energy region resulting in a panchromatic response as well as enhanced unidirectional flow of electrons. Li et al.64 applied two unsymmetrical squaraines, JYL-SQ5 (No. 51) and JYL-SQ6 (No. 52), where the electron-rich 3,4-ethylenedioxythiophene or bithiophene conjugated fragment was used to link unconventionally the squaraine core and the hexyloxyphenyl amino group. The corresponding photovoltaic devices exhibit an attractive panchromatic response and a portion of the near-infrared photons is indeed converted to electricity.
There are several strategies to shift the absorption of sensitizers into the NIR region. One such possibility was reported by Kiprianov65 who described a bathochromic shift in polymethine dyes containing two chromophores connected via a conjugated moiety. The shift is explained by the extension of the linear conjugated system. Kuster et al.66 describes the implementation of the approach made by Kiprianov65 to the formally named squaraine sensitizers to achieve a fully conjugated unsymmetrical dimeric squaraine sensitizer, BSQ01 (No. 53). The dimer exhibits outstanding optical properties such as absorption maximum in the NIR region of 745 nm with a huge molar absorption coefficient. The pertinent cell showed a JSC of 3.11 mA cm−2, a VOC of 550 V and a FF of 0.76, leading to an η of 1.3%. Kim et al.67 designed functionalized unsymmetrical benzothiazole squaraine organic sensitizers of 5-carboxy-2-({3-[(3-hexylbenzothiazol-2(3H)-ylidene)methyl]-2-hydroxy-4-oxo-2-cyclobuten-1-ylidene}methyl)-1-hexyl-3,3-dimethyl-3H-indolium (SK-11, No. 54) and 5-carboxy-2-({3-[(3-hexyl-5-methoxybenzothiazol-2(3H)-ylidene)methyl]-2-hydroxy-4-oxo-2-cyclobuten-1-ylidene}ethyl)-1-hexyl-3,3-dimethyl-3H-indolium (SK-12, No. 55), and observed an intense and wider absorption band in the red/NIR wavelength region. The absorption of SK-11 at 640 nm is due to the charge transfer transition of the conjugated molecule. The charge transfer transition of SK-12 sensitizer appears at slightly longer wavelength (648 nm). A solid-state DSSC sensitized with SK-11 dye has the following performance parameters: JSC of 2.00 mA cm−2, VOC of 630 V, FF of 0.67, and η of 0.85%. In comparison, the SK-12 sensitizer has slightly inferior cell performance: JSC of 1.26 mA cm−2, VOC of 660 V, FF of 0.66, and η of 0.55%.
Major factors for the low η of NIR dyes are the formation of dye aggregate and the lack of charge directionality in the excited state. Another disadvantage of near-IR dyes is the low stability under thermal stress and light-soaking test. Therefore, molecular engineering of NIR dyes with a high photovoltaic performance and an enhanced stability is strongly desired. By incorporating the nonplanar dimethylfluoreneaniline and unsymmetrical squaraine unit into the organic framework, not only dye aggregation was suppressed, but also long-term stability of the device was improved. Paek et al.68 designed new series of stable, unsymmetrical squaraine NIR sensitizers, JK-216 (No. 56) and JK-217 (No. 57), with both thiophenyl pyrrolyl and indolium groups. Dyes JK-216 and JK-217 have an absorption bands at 669 nm and 672 nm and their cells have η of 6.29% and 5.99%, respectively. Hao et al.69 reported a new strategy in which the anchoring group (lateral anchoring group) is separated from the acceptor groups of the sensitizers. Among these dyes, the HY103 (No. 58) sensitizer showed a maximum IPCE of 86% at 660 nm and an η of 3.7%. To further improve this system, the authors70 synthesized HY113 (No. 59), which has a similar structure with HY103 except that the methyl groups of the donor in the latter were replaced with flexible and long carbon chains. The aim of the long carbon chain was to prevent the formation of molecular aggregates on the semiconductor nanoparticles, thereby blocking charge recombination to retain relatively high VOC and JSC. The DSSC with HY113 dye showed a maximum IPCE of 93% at 660 nm and an η of 5.1%. A novel squarylium dye (TSQb, No. 60), which exhibited intense absorption peak at 850 nm and an absorption onset at 950 nm, was reported by Maeda et al.,71 and the DSSC with TSQb exhibited an η of 2.4%.
On the other hand, chlorophyll derivative-based organic sensitizers have shown good light-harvesting ability in NIR region. A purpurin sensitizer (trans-32-carboxy-purpurin-18 methyl ester), H2P (No. 61), which exhibited intense absorption peaks at 410, 545, and 700 nm, has been synthesized and used in DSSCs by Wang et al.72 However, this sensitizer has been found in aggregated morphology on a semiconductor surface to give a low JSC value resulting in an η of 0.1%. They subsequently improved the photovoltaic performance of a DSSC with a well-known chlorophyll derivative, chlorin e6 (No. 62), by introducing alkyl ester substituents at the C15 and C17 positions of the chlorin macrocycle of chlorin e6.73 The IPCE of the DSSC with chlorin e6 dye exhibited a substantially broad wavelength range from 350 to 775 nm. The pertinent DSSC exhibited a good η of 6.70%.
The development of NIR sensitizers are useful for tandem DSSCs, hybrid DSSCs and co-sensitized DSSCs.74–76 In viewing that the NIR dyes currently used for DSSCs exhibit narrow absorption bands with cutoff usually below 800 nm, it is necessary to develop NIR dyes with strong and broad absorptions between 800 and 1200 nm for high performance DSSCs.
4. Co-sensitization (panchromatic engineering)
Since the η of DSSCs depends mainly on the sensitizing ability of dyes, extensive efforts have been made to extend the optical threshold wavelength for panchromatic sensitization. Synthesis of sensitizers having both a broad absorption spectrum and matched energy levels simultaneously for DSSCs is very challenging, therefore, co-sensitization method using multiple dyes with compensatory absorption spectra is considered as a convenient approach. In this section, we reported the progresses on the panchromatic engineering in the DSSCs field. The associated sensitizers are shown in Fig. 8 and the previous figures. The photovoltaic parameters of the co-sensitized DSSCs are listed in Table 4.
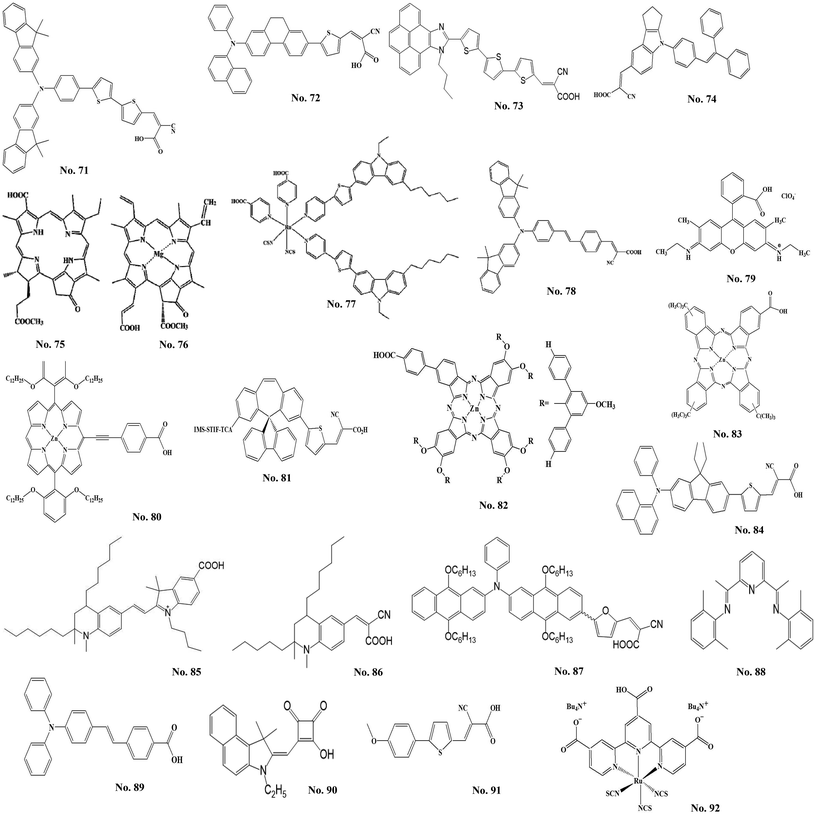 |
| Fig. 8 The structure of sensitizers for panchromatic engineering in DSSCs. | |
Table 4 The photovoltaic parameters for co-sensitized DSSCs
Sensitizer code x/y/z (dye number) |
η (%) for x/y/z |
η (%) for co-sensitized DSSCs; electrolyte (redox couple) |
JSC (mA cm−2) |
VOC (mV) |
FF |
Ref. |
YD2-o-C8/Y123 (6/7) |
11.90/8.40 |
12.30; Liquid (Co(II)/Co(III)) |
18.30 |
935 |
0.74 |
13 |
Cy0/Cy1/SQ (63/64/65) |
0.80/2.30/2.40 |
3.10; Liquid (I−/I3−) |
11.50 |
470 |
0.57 |
77 |
A/B (66/67) |
1.30/2.90 |
3.40; Liquid (I−/I3−) |
5.80 |
520 |
0.47 |
78 |
Y/R/B (68/69/70) |
2.80/4.60/3.90 |
6.50; Liquid (I−/I3−) |
15.80 |
525 |
0.63 |
79 |
JK2/SQ01 (71/46) |
7.00/4.23 |
7.36; Liquid (I−/I3−) |
16.20 |
649 |
0.70 |
80 |
BP-2/SQ02 (72/47) |
5.95/3.78 |
8.14; Liquid (I−/I3−) |
21.33 |
660 |
0.58 |
82 |
SQ02/5c (47/73) |
3.98/5.33 |
6.24; Liquid (I−/I3−) |
17.86 |
610 |
0.57 |
83 |
Black dye/D131 (3/74) |
10.00/4.73 |
11.08; Liquid (I−/I3−) |
23.23 |
676 |
0.71 |
84 |
Phe a/Chl c2 (75/76) |
3.40/3.80 |
5.40; Liquid (I−/I3−) |
14.00 |
600 |
0.64 |
90 |
JK-142/JK-62 (77/78) |
7.28/5.36 |
10.20; Liquid (I−/I3−) |
18.61 |
740 |
0.74 |
91 |
RhCL/N3 (79/1) |
0.63/2.37 |
4.74; Liquid (I−/I3−) |
11.00 |
695 |
0.62 |
92 |
LD12/CD5 (80/81) |
7.50/5.70 |
9.00; Liquid (I−/I3−) |
16.47 |
740 |
0.73 |
93 |
PcS15/D131 (82/74) |
5.30/— |
6.20; Liquid (I−/I3−) |
15.30 |
630 |
0.64 |
96 |
JK2/TT1 (71/83) |
7.08/3.52 |
7.74; Liquid (I−/I3−) |
16.20 |
666 |
0.72 |
97 |
N719/FL (2/84) |
4.89/2.67 |
5.10; Liquid (I−/I3−) |
11.42 |
715 |
0.63 |
98 |
CMR103/CM203/HY113 (86/85/59) |
3.10/5.00/4.40 |
8.20; Liquid (I−/Sx2−/S) |
20.10 |
597 |
0.68 |
99 |
Ant3/SQ02 (87/47) |
7.52/3.81 |
8.08; Liquid (I−/I3−) |
18.01 |
690 |
0.65 |
100 |
DM/N719 (88/2) |
0.69/5.43 |
7.00; Liquid (I−/I3−) |
16.57 |
720 |
0.59 |
101 |
N719/SQ01 (2/46) |
6.00/3.70 |
7.90; Liquid (I−/I3−) |
15.00 |
820 |
0.63 |
102 |
6/N719 (89/2) |
1.20/5.60 |
7.50; Liquid (I−/I3−) |
15.45 |
740 |
0.65 |
103 |
7b/SQ02 (90/47) |
5.00/3.40 |
6.10; Liquid (I−/I3−) |
14.61 |
660 |
0.63 |
104 |
N719/black dye (2/3) |
—/— |
10.50; Gel (I−/I3−) |
21.10 |
545 |
— |
105 |
P5/N719/N749 (91/2/92) |
1.00/2.60/3.60 |
4.80; Liquid (I−/I3−) |
10.60 |
612 |
0.73 |
106 |
JK2/SQ01 (71/46) |
7.48/4.02 |
8.01; Liquid (I−/I3−) |
16.90 |
662 |
0.71 |
108 |
Two possible configurations are proposed when several sensitizers are deposited on a semiconductor film. In the first configuration, the sensitizers are homogeneously deposited on the semiconductor film from a ‘cocktail’ solution.77–79 In this configuration, various sensitizers are in close contact. The highest η record for the cell co-sensitized by this configuration was obtained by Yella et al.13 They reported the co-sensitization of a zinc porphyrin dye (YD2-o-C8, No. 6) with an organic dye (Y123, No. 7) by immersing TiO2 films in a mixture of the two. An η of 12.30% was achieved for the pertinent DSSC using a Co(II/III)tris(bipyridyl)-based redox electrolyte. Earlier, other groups also constructed co-sensitized DSSCs similarly. Sayama et al.77 investigated various kinds of cyanine (Cy0, No. 63; Cy1, No. 64) and merocyanine (SQ, No. 65) organic sensitizers having short anchoring groups. Compared with the DSSCs using single sensitizer, the pertinent DSSC showed improved JSC and η of 11.5 mA cm−2 and 3.10%, respectively. Guo et al.78 used pentamethylcyanine derivative (A, No. 66), trimethylcyanine derivative (B, No. 67) for co-sensitized DSSCs, and found that the mixture with the volume ratio of 1
:
3 (A
:
B) generated an η of 3.40%. Chen et al.79 reported a co-sensitized DSSC composed of three organic sensitizers (Y, No. 68; R, No. 69; B, No. 70), and the pertinent DSSC achieved a high η of 6.5%. Yum et al.80 prepared organic sensitizers, JK2 (No. 71)81 and SQ01 (No. 46),62 for co-sensitization; the former dye absorbs the light in the blue part of the visible spectrum while latter dye absorbs the light in the red/near IR region. The co-sensitized DSSC showed a higher η of 7.38%, as compared to those sensitized by either JK2 (7.00%) or SQ01 (4.23%). The results are attributed to the complementary spectra for these two sensitizers. Lin et al.82 synthesized a metal-free sensitizer containing a 9,10-dihydrophenanthrene unit in the spacer (BP-2, No. 72), and fabricated co-sensitized DSSCs from BP-2 and SQ02 (No. 47) sensitizers. A notable η of 8.14% was reached with this all metal-free dyes system. Chang et al.83 investigated the DSSCs performance co-sensitized by a mixing solution containing pyrenoimidazole-based organic dye (5c, No. 73 and SQ02 (No. 47) dye in different volumetric ratios. The absorption spectrum of the co-sensitized TiO2 thin film becomes more intense and broader, and the cell efficiency reaches 6.24% at the molar ratio of SQ02/5c = 4/6. Aggregation suppression of one dye by the other dye at the TiO2 surface should be advantageous for the co-sensitization system since dye aggregation at the TiO2 surface causes a decrease of cell performance in the DSSCs.84–89 Ozawa et al.84 fabricated a co-sensitized DSSC by immersing TiO2 films into the mixed solution containing the terpyridine complex (black dye, No. 3) and an organic sensitizer (D131, No. 74). There was potential suppression of black dye aggregation at the TiO2 surface when D131 was co-absorbed, and the better JSC (23.23 mA cm−2) and η (11.08%) were achieved for the pertinent DSSC. Wang et al.90 succeeded in demonstrating the chlorophyll co-sensitized solar cells. An enhanced η of up to 5.40% has been obtained for the DSSC based on co-sensitization with Phe a (No. 75) and Chl c2 (No. 76).
However, mixing two dyes sometimes resulted in a decrease of η, probably due to the decreased injection efficiency caused by intermolecular interactions between the two dyes. Thus, prevention of electronic interactions between the dyes on the TiO2 surface is important for the development of efficient cocktail-type DSSCs. The second configuration is the semi-tandem type, in which sensitizers are deposited alternately on the film to generate a layer-by-layer configuration. An excellent η for the cell co-sensitized by this configuration was obtained by Fan et al.91 They synthesized a ruthenium complex (JK-142, No. 77) with an ancillary bipyridyl ligand substituted by a 3-carbazole-2-thiophenyl moiety, and explored the performance of the co-sensitized solar cells in combination with an organic sensitizer (JK-62, No. 78) by dipping TiO2 films in JK-142 and JK-62 sensitizers layer-by-layer. The η of 10.20% was achieved for the pertinent DSSC. Other groups also utilized the same configuration to assemble co-sensitized DSSCs. Saxena et al.92 has refluxed TiO2 film in RhCL dye (No. 79) solution for 2 h and then dipped the sensitized film in N3 dye (No. 1) solution for different time intervals. An improved η of 4.74% was achieved for the co-sensitized DSSC, as compared to those of the cells with RhCL dye (0.63%) and N3 dye (2.37%). Lan et al.93 applied co-sensitization of a zinc porphyrin bearing two ortho-substituted long alkoxyl chains (LD12, No. 80)94 with a spirally configured donor–acceptor organic dye (CD5, No. 81)95 by immersing the TiO2 film first in a LD12 solution and then in a CD5 solution. The co-sensitized solar cell showed significantly enhanced VOC, JSC and η (9.00%) relative to the individual single-dye sensitized devices. Kimura et al.96 reported the co-sensitization of the TiO2 film by PcS15 (No. 82) and an orange organic sensitizer (D131, No. 74), which showed little interaction between the dyes and, thus, resulted in a dramatic enhancement of the photocurrent response throughout the entire visible-light region. Cid et al.97 fabricated DSSCs with the TiO2 films immersed into JK2 (No. 71) solution and TT1 (No. 83) solution, sequentially. The η of the co-sensitized solar cell reached 7.74%, which is higher than those of the cells sensitized with JK2 dye (7.08%) and TT1 dye (3.52%). Lee et al.98 accomplished stepwise co-sensitization by dipping the TiO2 films for different time intervals in N719 (No. 2) and FL (No. 84) dyes, separately, and the pertinent DSSC exhibited an η of 5.10% with conducting plastics as the substrates. Cheng et al.99 synthesized a cyanine organic dye (CM203, No. 85) for a DSSC, which shows a performance of 5.00% with the incorporation of a mixing electrolyte containing iodide and polysulfide redox couples. To improve the cell performance, a co-sensitized DCCS was fabricated from CMR103 (No. 86), CM203 (No. 85), and HY113 (No. 59) dyes, and the pertinent η can achieve 8.20%. Lin et al.100 developed a series of novel organic dyes containing a 2,6-conjugated anthracene unit as the π-spacer. The most efficient dye (Ant3, No. 87) reached a good η of 7.52% for its DSSC. The co-sensitized DSSC exhibited an improved efficiency of 8.08% by stepwise dipping the TiO2 films in the Ant3 and SQ02 (No. 47) dye solutions for different durations. Wei et al.101 synthesized an organic dye containing 2,6-bis(iminoalkyl)pyridine (DM, No. 88) for a DM/N719 (No. 2) co-sensitized DSSC via the layer-by-layer dye loading technique. The aggregation of N719 was alleviated and its absorption response was enhanced in the region from 400 to 750 nm, thus the DM/N719 co-sensitized DSSC performance of 7.00% can be obtained. On the other hand, Holliman and coworkers102 presented a rapid (5 min) and controlled co-sensitization method for injecting or pumping N719 (No. 2) and SQ01 (No. 46) dyes through the cavity of the photoanode and the counter electrode in TiO2 films for a period of minutes. Their pertinent DSSC showed an η up to 7.9%. The authors also synthesized a new triarylamine dye (6, No. 89)103 with a donor–spacer–acceptor (triarylamine–vinyl–benzyl linker) and co-sensitized a TiO2 photoanode with N719 (No. 2) dye using an ultra-fast dye sensitization method within 5 min of dye soaking. The pertinent co-sensitized DSSC achieved an η of 7.50%. In addition, they further developed the new half-squaraine dye (7b, No. 90)104 based on a common chromophoric unit consisting of linked indoline and squaric acid moieties. By applying the same rapid pump dyeing method mentioned above, the co-sensitization of 7b with another commercial squaraine dye (SQ02, No. 47) resulted in a broader spectral response and an improved η of 6.10%.
Although the co-sensitization of multiple sensitizers has been successfully fulfilled by immersing TiO2 films in a mixed solution of two dyes or in solutions with individual dyes step by step, a decrease in photocurrent may sometimes occur owing to the negative interactions between different sensitizers. To avoid the negative effect caused by different dyes, other approaches for co-sensitization were applied. Dürr et al.105 assembled a tandem cell with improved photocurrent by adsorbing N719 (No. 2) dye and black dye (No. 3) in two different compartments of a former cell and a latter cell, and this kind of device reached an η of 10.50%. To further lower the cell cost, the concept that the single photoanode sensitized with two or more different dyes (P5, No. 91; N719, No. 2; N749, No. 92) were co-adsorbed separately in different layers of the TiO2 film, was proposed by using a selective position method. With this method one adsorbed dye in a controlled thickness of TiO2 film was desorbed followed by the adsorbing of another dye, and the DSSC with this kind of photoanode achieved an η of 4.80%.106 On the other hand, Clifford et al.107 provided a novel co-sensitization strategy based on the deposition of a secondary metal oxide layer between the first and second sensitizers. This approach was developed further by Choi et al.108 by first sensitizing TiO2 films with JK2 dye (No. 71), depositing Al2O3 layer on the reluctant film, and then sensitizing the TiO2 film with SQ01 dye (No. 46). The charge transfer processes in the multilayer co-sensitized TiO2 film is shown in Fig. 9. The JK2/Al2O3/SQ01 cell resulted in the JSC and η of 17.6 mA cm−2 and 8.65%, respectively. In comparison, the JK2/SQ01 sensitized cell (without Al2O3 layer) showed the JSC and η of only 16.9 mA cm−2 and 8.01%, respectively.
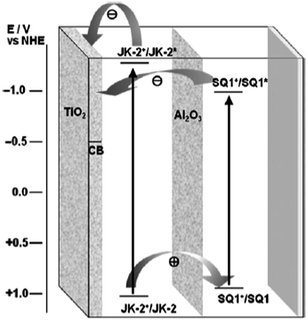 |
| Fig. 9 Charge-transfer processes in multilayer co-sensitized TiO2 films with a Al2O3 layer (CB = conduction band, NHE = normal hydrogen electrode). Reproduced by permission of The Royal Society of Chemistry. | |
5. Conclusion
In the past two decades, most efficiency records of DSSCs are made by metal-based dyes because of their broad absorption spectra and favourable photovoltaic properties. However, high cost and complicated synthesis of metal-based dyes have limited their developing potentials in DSSCs. Recently, metal-free organic dyes have been considered as a promising alternative to metal-based dyes due to their relatively high efficiency, possibly low cost, structurally good design flexibility, and extremely high molar extinction coefficients. On the other hand, during the developing stage of solid-state DSSCs, the perovskite CH3NH3PbI3 sensitizer has attracted great attention due to its superb light-harvesting characteristics.109 Though the perovskite solar cells achieved a remarkable η record of 19.30%, they possess several disadvantages, namely reliance on an environmentally hazardous heavy metal and the low toleration to moist air and water vapour.110 Therefore, on the aspects of environmental friendly materials and durability issues, the metal-free organic dyes are still rather competitive.
This review has summarized the recent progresses on metal-free organic sensitizers and their application in DSSCs. The η of the DSSC with an organic sensitizer (C219) utilizing a binary π-conjugated spacer of ethylenedioxythiophene and dithienosilole (spacer containing fluorene fragments) has achieved 10.10% record. On the other hand, a DSSC with an organic sensitizer (WS-9) incorporating the benzothiadiazole moiety adjacent to indoline donor and an n-hexylthiophene unit into the π-conjugated spacer containing electron-deficient moieties also shows a remarkable η of 9.04%. For the DSSC with NIR sensitizer, an η of 6.29% can be obtained by using an unsymmetrical squaraine sensitizer, JK-216, with thiophenyl pyrrolyl and indolium groups, which exhibit a maximum absorption peak at 669 nm. All of these results have demonstrated that the DSSC with an organic sensitizer can achieve an η as good as Ru-sensitizer-based cell.
The co-sensitization approach is a convenient method to improve the performance of DSSCs by using multiple sensitizers for panchromatic absorption. The DSSC with the Co(II/III)tris(bipyridyl)-based redox electrolyte has achieved an 12.30% efficiency record by incorporating the novel zinc porphyrin dye (YD2-o-C8) and co-sensitizing with another organic dye (Y123). Moreover, a co-sensitized DSSC with metal-free organic sensitizers and Al2O3 layer, JK2/Al2O3/SQ01, has reached an appealing η of 8.65%.
In fact, it is well known that there are several factors determining the η of DSSCs. In the future, we need to optimize every material within the DSSC for further achieving a breakthrough on the η of DSSC. Undoubtedly, the development of a conceptually new design for constructing metal-free organic sensitizers is one of the most important keys to the full success of this field.
Acknowledgements
We acknowledge financial supports from the Ministry of Science and Technology (MOST) of Taiwan and the Academia Sinica, Taipei, Taiwan.
Notes and references
- N. Armaroli and V. Balzani, Angew. Chem., Int. Ed., 2007, 46, 52–66 CrossRef CAS PubMed.
- D. Gust, T. A. Moore and A. L. Moore, Acc. Chem. Res., 2009, 42, 1890–1898 CrossRef CAS PubMed.
- D. G. Nocera, Inorg. Chem., 2009, 48, 10001–10017 CrossRef CAS PubMed.
- M. Grätzel, Nature, 2001, 414, 338–344 CrossRef PubMed.
- B. O'Regan and M. Grätzel, Nature, 1991, 353, 737–740 CrossRef.
- C. J. Barbé, F. Arendse, P. Comte, M. Jirousek, F. Lenzmann, V. Shklover and M. Grätzel, J. Am. Ceram. Soc., 1997, 80, 3157–3171 CrossRef PubMed.
- A. Hagfeldt and M. Grätzel, Acc. Chem. Res., 2000, 33, 269–277 CrossRef CAS PubMed.
- M. K. Nazeeruddin, F. De Angelis, S. Fantacci, A. Selloni, G. Viscardi, P. Liska, S. Ito, B. Takeru and M. Grätzel, J. Am. Chem. Soc., 2005, 127, 16835–16847 CrossRef CAS PubMed.
- F. Gao, Y. Wang, D. Shi, J. Zhang, M. Wang, X. Jing, R. Humphry-Baker, P. Wang, S. M. Zakeeruddin and M. Grätzel, J. Am. Chem. Soc., 2008, 130, 10720–10728 CrossRef CAS PubMed.
- C. Y. Chen, M. Wang, J. Y. Li, N. Pootrakulchote, L. Alibabaei, C. H. Ngoc-Le, J. D. Decoppet, J. H. Tsai, C. Grätzel, C. G. Wu, S. M. Zakeeruddin and M. Grätzel, ACS Nano, 2009, 3, 3103–3109 CrossRef CAS PubMed.
- Y. Chiba, A. Islam, Y. Watanabe, R. Komiya, N. Koide and L. Han, Jpn. J. Appl. Phys., Part 1, 2006, 45, L638–L640 CrossRef CAS.
- S. W. Wang, C. C. Chou, F. C. Hu, K. L. Wu, Y. Chi, J. N. Clifford, E. Palomares, S. H. Liu, P. T. Chou, T. C. Wei and T. Y. Hsiao, J. Mater. Chem. A, 2014, 2, 17618–17627 CAS.
- A. Yella, H. W. Lee, H. N. Tsao, C. Yi, A. K. Chandiran, M. K. Nazeeruddin, E. W. G. Diau, C. Y. Yeh, S. M. Zakeeruddin and M. Grätzel, Science, 2011, 334, 629–634 CrossRef CAS PubMed.
- S. Mathew, A. Yella, P. Gao, R. Humphry-Baker, B. F. E. Curchod, N. Ashari-Astani, I. Tavernelli, U. Rothlisberger, M. K. Nazeeruddin and M. Grätzel, Nat. Chem., 2014, 6, 242–247 CrossRef CAS PubMed.
- L. L. Li and E. W. G. Diau, Chem. Soc. Rev., 2013, 42, 291–304 RSC.
- Y. S. Yen, H. H. Chou, Y. C. Chen, C. Y. Hsu and J. T. Lin, J. Mater. Chem., 2012, 22, 8734–8747 RSC.
- M. Liang and J. Chen, Chem. Soc. Rev., 2013, 42, 3453–3488 RSC.
- W. Zeng, Y. Cao, Y. Bai, Y. Wang, Y. Shi, M. Zhang, F. Wang, C. Pan and P. Wang, Chem. Mater., 2010, 22, 1915–1925 CrossRef CAS.
- H. Zhou, P. Xue, Y. Zhang, X. Zhao, J. Jia, X. Zhang, X. Liu and R. Lu, Tetrahedron, 2011, 67, 8477–8483 CrossRef CAS PubMed.
- W. Li, Y. Wu, X. Li, Y. Xie and W. Zhu, Energy Environ. Sci., 2011, 4, 1830–1837 CAS.
- D. Y. Chen, Y. Y. Hsu, H. C. Hsu, B. S. Chen, Y. T. Lee, H. Fu, M. W. Chung, S. H. Liu, H. C. Chen, Y. Chi and P. T. Chou, Chem. Commun., 2010, 46, 5256–5258 RSC.
- Y. Bai, J. Zhang, D. Zhou, Y. Wang, M. Zhang and P. Wang, J. Am. Chem. Soc., 2011, 133, 11442–11445 CrossRef CAS PubMed.
- M. Xu, D. Zhou, N. Cai, J. Liu, R. Li and P. Wang, Energy Environ. Sci., 2011, 4, 4735–4742 CAS.
- K. D. Seo, H. M. Song, M. J. Lee, M. Pastore, C. Anselmi, F. De Angelis, M. K. Nazeeruddin, M. Gräetzel and H. K. Kim, Dyes Pigm., 2011, 90, 304–310 CrossRef CAS PubMed.
- H. Lai, J. Hong, P. Liu, C. Yuan, Y. Li and Q. Fang, RSC Adv., 2012, 2, 2427–2432 RSC.
- C. Chen, J. Y. Liao, Z. Chi, B. Xu, X. Zhang, D. B. Kuang, Y. Zhang, S. Liu and J. Xu, RSC Adv., 2012, 2, 7788–7797 RSC.
- Z. M. Tang, T. Lei, K. J. Jiang, Y. L. Song and J. Pei, Chem.–Asian J., 2010, 5, 1911–1917 CrossRef CAS PubMed.
- W. Zhu, Y. Wu, S. Wang, W. Li, X. Li, J. Chen, Z. Wang and H. Tian, Adv. Funct. Mater., 2011, 21, 756–763 CrossRef CAS.
- Y. Wu, X. Zhang, W. Li, Z. S. Wang, H. Tian and W. Zhu, Adv. Energy Mater., 2012, 2, 149–156 CrossRef CAS.
- Y. Wu, M. Marszalek, S. M. Zakeeruddin, Q. Zhang, H. Tian, M. Gratzel and W. Zhu, Energy Environ. Sci., 2012, 5, 8261–8272 CAS.
- S. Haid, M. Marszalek, A. Mishra, M. Wielopolski, J. Teuscher, J. E. Moser, R. Humphry-Baker, S. M. Zakeeruddin, M. Grätzel and P. Bäuerle, Adv. Funct. Mater., 2012, 22, 1291–1302 CrossRef CAS.
- S. Qu, C. Qin, A. Islam, Y. Wu, W. Zhu, J. Hua, H. Tian and L. Han, Chem. Commun., 2012, 48, 6972–6974 RSC.
- J. He, F. Guo, X. Li, W. Wu, J. Yang and J. Hua, Chem.–Eur. J., 2012, 18, 7903–7915 CrossRef CAS PubMed.
- H. H. Chou, Y. C. Chen, H. J. Huang, T. H. Lee, J. T. Lin, C. Tsai and K. Chen, J. Mater. Chem., 2012, 22, 10929–10938 RSC.
- K. D. Seo, I. T. Choi, Y. G. Park, S. Kang, J. Y. Lee and H. K. Kim, Dyes Pigm., 2012, 94, 469–474 CrossRef CAS PubMed.
- D. H. Lee, M. J. Lee, H. M. Song, B. J. Song, K. D. Seo, M. Pastore, C. Anselmi, S. Fantacci, F. De Angelis, M. K. Nazeeruddin, M. Gräetzel and H. K. Kim, Dyes Pigm., 2011, 91, 192–198 CrossRef CAS PubMed.
- K. Pei, Y. Wu, W. Wu, Q. Zhang, B. Chen, H. Tian and W. Zhu, Chem.–Eur. J., 2012, 18, 8190–8200 CrossRef CAS PubMed.
- S. R. Li, C. P. Lee, H. T. Kuo, K. C. Ho and S. S. Sun, Chem.–Eur. J., 2012, 18, 12085–12095 CrossRef CAS PubMed.
- S. R. Li, C. P. Lee, C. W. Liao, W. L. Su, C. T. Li, K. C. Ho and S. S. Sun, Tetrahedron, 2014, 70, 6276–6284 CrossRef CAS PubMed.
- J. Shi, J. Chen, Z. Chai, H. Wang, R. Tang, K. Fan, M. Wu, H. Han, J. Qin, T. Peng, Q. Li and Z. Li, J. Mater. Chem., 2012, 22, 18830–18838 RSC.
- D. W. Chang, H. J. Lee, J. H. Kim, S. Y. Park, S. M. Park, L. Dai and J. B. Baek, Org. Lett., 2011, 13, 3880–3883 CrossRef CAS PubMed.
- J. Warnan, L. Favereau, Y. Pellegrin, E. Blart, D. Jacquemin and F. Odobel, J. Photochem. Photobiol., A, 2011, 226, 9–15 CrossRef CAS PubMed.
- S. Qu, W. Wu, J. Hua, C. Kong, Y. Long and H. Tian, J. Phys. Chem. C, 2009, 114, 1343–1349 Search PubMed.
- Y. Cui, Y. Wu, X. Lu, X. Zhang, G. Zhou, F. B. Miapeh, W. Zhu and Z. S. Wang, Chem. Mater., 2011, 23, 4394–4401 CrossRef CAS.
- H. H. Chou, C. Y. Hsu, Y. C. Hsu, Y. S. Lin, J. T. Lin and C. Tsai, Tetrahedron, 2012, 68, 767–773 CrossRef CAS PubMed.
- C. H. Chen, Y. C. Hsu, H. H. Chou, K. R. J. Thomas, J. T. Lin and C. P. Hsu, Chem.–Eur. J., 2010, 16, 3184–3193 CrossRef CAS PubMed.
- J. He, W. Wu, J. Hua, Y. Jiang, S. Qu, J. Li, Y. Long and H. Tian, J. Mater. Chem., 2011, 21, 6054–6062 RSC.
- B. S. Chen, Y. J. Chen and P. T. Chou, J. Mater. Chem., 2011, 21, 4090–4094 RSC.
- Y. T. Li, C. L. Chen, Y. Y. Hsu, H. C. Hsu, Y. Chi, B. S. Chen, W. H. Liu, C. H. Lai, T. Y. Lin and P. T. Chou, Tetrahedron, 2010, 66, 4223–4229 CrossRef CAS PubMed.
- J. H. Chen, C. H. Tsai, S. A. Wang, Y. Y. Lin, T. W. Huang, S. F. Chiu, C. C. Wu and K. T. Wong, J. Org. Chem., 2011, 76, 8977–8985 CrossRef CAS PubMed.
- C. Qin, A. Islam and L. Han, J. Mater. Chem., 2012, 22, 19236–19243 RSC.
- L. Y. Lin, C. H. Tsai, K. T. Wong, T. W. Huang, C. C. Wu, S. H. Chou, F. Lin, S. H. Chen and A. I. Tsai, J. Mater. Chem., 2011, 21, 5950–5958 RSC.
- Y. Ooyama, S. Inoue, T. Nagano, K. Kushimoto, J. Ohshita, I. Imae, K. Komaguchi and Y. Harima, Angew. Chem., Int. Ed., 2011, 50, 7429–7433 CrossRef CAS PubMed.
- W. Zhang, Q. Feng, Z. S. Wang and G. Zhou, Chem.–Asian J., 2013, 8, 939–946 CrossRef CAS PubMed.
- Q. Miao, J. Gao, Z. Wang, H. Yu, Y. Luo and T. Ma, Inorg. Chim. Acta, 2011, 376, 619–627 CrossRef CAS PubMed.
- J. H. Yum, P. Walter, S. Huber, D. Rentsch, T. Geiger, F. Nüesch, F. De Angelis, M. Grätzel and M. K. Nazeeruddin, J. Am. Chem. Soc., 2007, 129, 10320–10321 CrossRef CAS PubMed.
- A. Burke, L. Schmidt Mende, S. Ito and M. Grätzel, Chem. Commun., 2007, 234–236 RSC.
- X. Ma, J. Hua, W. Wu, Y. Jin, F. Meng, W. Zhan and H. Tian, Tetrahedron, 2008, 64, 345–350 CrossRef CAS PubMed.
- C. Li, J. H. Yum, S. J. Moon, A. Herrmann, F. Eickemeyer, N. G. Pschirer, P. Erk, J. Schöneboom, K. Müllen, M. Grätzel and M. K. Nazeeruddin, ChemSusChem, 2008, 1, 615–618 CrossRef CAS PubMed.
- Q. Wang, W. M. Campbell, E. E. Bonfantani, K. W. Jolley, D. L. Officer, P. J. Walsh, K. Gordon, R. Humphry Baker, M. K. Nazeeruddin and M. Grätzel, J. Phys. Chem. B, 2005, 109, 15397–15409 CrossRef CAS PubMed.
- J. J. Cid, J. H. Yum, S. R. Jang, M. K. Nazeeruddin, E. Martínez Ferrero, E. Palomares, J. Ko, M. Grätzel and T. Torres, Angew. Chem., Int. Ed., 2007, 46, 8358–8362 CrossRef CAS PubMed.
- T. Geiger, S. Kuster, J. H. Yum, S. J. Moon, M. K. Nazeeruddin, M. Grätzel and F. Nüesch, Adv. Funct. Mater., 2009, 19, 2720–2727 CrossRef CAS.
- H. Choi, J. J. Kim, K. Song, J. Ko, M. K. Nazeeruddin and M. Gräetzel, J. Mater. Chem., 2010, 20, 3280–3286 RSC.
- J. Y. Li, C. Y. Chen, C. P. Lee, S. C. Chen, T. H. Lin, H. H. Tsai, K. C. Ho and C. G. Wu, Org. Lett., 2010, 12, 5454–5457 CrossRef CAS PubMed.
- A. I. Kiprianov, Russ. Chem. Rev., 1971, 40, 594–607 CrossRef PubMed.
- S. Kuster, F. Sauvage, M. K. Nazeeruddin, M. Grätzel, F. A. Nüesch and T. Geiger, Dyes Pigm., 2010, 87, 30–38 CrossRef CAS PubMed.
- S. Kim, G. K. Mor, M. Paulose, O. K. Varghese, C. Baik and C. A. Grimes, Langmuir, 2010, 26, 13486–13492 CrossRef CAS PubMed.
- S. Paek, H. Choi, C. Kim, N. Cho, S. So, K. Song, M. K. Nazeeruddin and J. Ko, Chem. Commun., 2011, 47, 2874–2876 RSC.
- Y. Hao, X. Yang, J. Cong, H. Tian, A. Hagfeldt and L. Sun, Chem. Commun., 2009, 4031–4033 RSC.
- Y. Hao, X. Yang, M. Zhou, J. Cong, X. Wang, A. Hagfeldt and L. Sun, ChemSusChem, 2011, 4, 1601–1605 CrossRef CAS PubMed.
- T. Maeda, S. Arikawa, H. Nakao, S. Yagi and H. Nakazumi, New J. Chem., 2013, 37, 701–708 RSC.
- X. F. Wang, L. Wang, N. Tamai, O. Kitao, H. Tamiaki and S. I. Sasaki, J. Phys. Chem. C, 2011, 115, 24394–24402 CAS.
- X. F. Wang, H. Tamiaki, O. Kitao, T. Ikeuchi and S. I. Sasaki, J. Power Sources, 2013, 242, 860–864 CrossRef CAS PubMed.
- T. Yamaguchi, Y. Uchida, S. Agatsuma and H. Arakawa, Sol. Energy Mater. Sol. Cells, 2009, 93, 733–736 CrossRef CAS PubMed.
- D. Kuang, P. Walter, F. Nüesch, S. Kim, J. Ko, P. Comte, S. M. Zakeeruddin, M. K. Nazeeruddin and M. Grätzel, Langmuir, 2007, 23, 10906–10909 CrossRef CAS PubMed.
- F. Inakazu, Y. Noma, Y. Ogomi and S. Hayase, Appl. Phys. Lett., 2008, 93, 093304 CrossRef PubMed.
- K. Sayama, S. Tsukagoshi, T. Mori, K. Hara, Y. Ohga, A. Shinpou, Y. Abe, S. Suga and H. Arakawa, Sol. Energy Mater. Sol. Cells, 2003, 80, 47–71 CrossRef CAS.
- M. Guo, P. Diao, Y. J. Ren, F. Meng, H. Tian and S. M. Cai, Sol. Energy Mater. Sol. Cells, 2005, 88, 23–35 CrossRef CAS PubMed.
- Y. Chen, Z. Zeng, C. Li, W. Wang, X. Wang and B. Zhang, New J. Chem., 2005, 29, 773–776 RSC.
- J. H. Yum, S. R. Jang, P. Walter, T. Geiger, F. Nüesch, S. Kim, J. Ko, M. Grätzel and M. K. Nazeeruddin, Chem. Commun., 2007, 4680–4682 RSC.
- S. Kim, J. K. Lee, S. O. Kang, J. Ko, J. H. Yum, S. Fantacci, F. De Angelis, D. Di Censo, M. K. Nazeeruddin and M. Grätzel, J. Am. Chem. Soc., 2006, 128, 16701–16707 CrossRef CAS PubMed.
- R. Y. Y. Lin, Y. S. Yen, Y. T. Cheng, C. P. Lee, Y. C. Hsu, H. H. Chou, C. Y. Hsu, Y. C. Chen, J. T. Lin, K. C. Ho and C. Tsai, Org. Lett., 2012, 14, 3612–3615 CrossRef CAS PubMed.
- J. Chang, C. P. Lee, D. Kumar, P. W. Chen, L. Y. Lin, K. R. J. Thomas and K. C. Ho, J. Power Sources, 2013, 240, 779–785 CrossRef CAS PubMed.
- H. Ozawa, R. Shimizu and H. Arakawa, RSC Adv., 2012, 2, 3198–3200 RSC.
- M. K. Nazeeruddin, R. Humphry Baker, M. Grätzel and B. A. Murrer, Chem. Commun., 1998, 719–720 RSC.
- A. C. Khazraji, S. Hotchandani, S. Das and P. V. Kamat, J. Phys. Chem. B, 1999, 103, 4693–4700 CrossRef CAS.
- J. He, G. Benkö, F. Korodi, T. Polívka, R. Lomoth, B. Åkermark, L. Sun, A. Hagfeldt and V. Sundström, J. Am. Chem. Soc., 2002, 124, 4922–4932 CrossRef CAS PubMed.
- K. Hara, Y. Dan oh, C. Kasada, Y. Ohga, A. Shinpo, S. Suga, K. Sayama and H. Arakawa, Langmuir, 2004, 20, 4205–4210 CrossRef CAS.
- X. Ren, Q. Feng, G. Zhou, C. H. Huang and Z. S. Wang, J. Phys. Chem. C, 2010, 114, 7190–7195 CAS.
- X. F. Wang, Y. Koyama, O. Kitao, Y. Wada, S. I. Sasaki, H. Tamiaki and H. Zhou, Biosens. Bioelectron., 2010, 25, 1970–1976 CrossRef CAS PubMed.
- S. Q. Fan, C. Kim, B. Fang, K. X. Liao, G. J. Yang, C. J. Li, J. J. Kim and J. Ko, J. Phys. Chem. C, 2011, 115, 7747–7754 CAS.
- V. Saxena, P. Veerender, A. K. Chauhan, P. Jha, D. K. Aswal and S. K. Gupta, Appl. Phys. Lett., 2012, 100, 133303 CrossRef PubMed.
- C. M. Lan, H. P. Wu, T. Y. Pan, C. W. Chang, W. S. Chao, C. T. Chen, C. L. Wang, C. Y. Lin and E. W. G. Diau, Energy Environ. Sci., 2012, 5, 6460–6464 CAS.
- Y. C. Chang, C. L. Wang, T. Y. Pan, S. H. Hong, C. M. Lan, H. H. Kuo, C. F. Lo, H. Y. Hsu, C. Y. Lin and E. W. G. Diau, Chem. Commun., 2011, 47, 8910–8912 RSC.
- W. S. Chao, K. H. Liao, C. T. Chen, W. K. Huang, C. M. Lan and E. W. G. Diau, Chem. Commun., 2012, 48, 4884–4886 RSC.
- M. Kimura, H. Nomoto, N. Masaki and S. Mori, Angew. Chem., Int. Ed., 2012, 51, 4371–4374 CrossRef CAS PubMed.
- J. J. Cid, J. H. Yum, S. R. Jang, M. K. Nazeeruddin, E. M. Ferrero, E. Palomares, J. Ko, M. Grätzel and T. Torres, Angew. Chem., Int. Ed., 2007, 119, 8510–8514 CrossRef.
- K. M. Lee, Y. C. Hsu, M. Ikegami, T. Miyasaka, K. R. Justin Thomas, J. T. Lin and K. C. Ho, J. Power Sources, 2011, 196, 2416–2421 CrossRef CAS PubMed.
- M. Cheng, X. Yang, J. Li, F. Zhang and L. Sun, ChemSusChem, 2013, 6, 70–77 CrossRef CAS PubMed.
- R. Y. Y. Lin, H. W. Lin, Y. S. Yen, C. H. Chang, H. H. Chou, P. W. Chen, C. Y. Hsu, Y. C. Chen, J. T. Lin and K. C. Ho, Energy Environ. Sci., 2013, 6, 2477–2486 Search PubMed.
- L. Wei, Y. Yang, R. Fan, P. Wang, L. Li, J. Yu, B. Yang and W. Cao, RSC Adv., 2013, 3, 25908–25916 RSC.
- P. J. Holliman, M. L. Davies, A. Connell, B. V. Velasco and T. M. Watson, Chem. Commun., 2010, 46, 7256–7258 RSC.
- P. J. Holliman, M. Mohsen, A. Connell, M. L. Davies, K. Al-Salihi, M. B. Pitak, G. J. Tizzard, S. J. Coles, R. W. Harrington, W. Clegg, C. Serpa, O. H. Fontes, C. Charbonneau and M. J. Carnie, J. Mater. Chem., 2012, 22, 13318–13327 RSC.
- A. Connell, P. J. Holliman, M. L. Davies, C. D. Gwenin, S. Weiss, M. B. Pitak, P. N. Horton, S. J. Coles and G. Cooke, J. Mater. Chem. A, 2014, 2, 4055–4066 CAS.
- M. Dürr, A. Bamedi, A. Yasuda and G. Nelles, Appl. Phys. Lett., 2004, 84, 3397–3399 CrossRef PubMed.
- K. Lee, S. W. Park, M. J. Ko, K. Kim and N. G. Park, Nat. Mater., 2009, 8, 665–671 CrossRef CAS PubMed.
- J. N. Clifford, E. Palomares, M. K. Nazeeruddin, R. Thampi, M. Grätzel and J. R. Durrant, J. Am. Chem. Soc., 2004, 126, 5670–5671 CrossRef CAS PubMed.
- H. Choi, S. Kim, S. O. Kang, J. Ko, M. S. Kang, J. N. Clifford, A. Forneli, E. Palomares, M. K. Nazeeruddin and M. Grätzel, Angew. Chem., Int. Ed., 2008, 47, 8259–8263 CrossRef CAS PubMed.
-
(a) A. Kojima, K. Teshima, Y. Shirai and T. Miyasaka, J. Am. Chem. Soc., 2009, 131, 6050–6051 CrossRef CAS PubMed;
(b) M. M. Lee, J. Teuscher, T. Miyasaka, T. N. Murakami and H. J. Snaith, Science, 2012, 338, 643–647 CrossRef CAS PubMed;
(c) N. G. Park, J. Phys. Chem. Lett., 2013, 4, 2423–2429 CrossRef CAS.
- A. G. Martin, H. B. Anita and J. S. Henry, Nat. Photonics, 2014, 8, 506–514 CrossRef.
|
This journal is © The Royal Society of Chemistry 2015 |
Click here to see how this site uses Cookies. View our privacy policy here.