DOI:
10.1039/D1QO00686J
(Research Article)
Org. Chem. Front., 2021,
8, 4678-4684
Aryl carbazole-based macrocycles: synthesis, their remarkably stable radical cations and host–guest complexation with fullerenes†
Received
1st May 2021
, Accepted 18th June 2021
First published on 22nd June 2021
Abstract
A series of fully conjugated macrocycles Mn (n = 4–7) consisting of N-(3,5-di-tert-butyl-4-methoxyphenyl) substituted carbazole (Cz-Ar) were successfully synthesized. The aryl carbazole and macrocycle M4 can be readily oxidized and the corresponding radical cation species were found to be highly stable. Moreover, macrocycle M5 was found to form 1
:
1 stoichiometric complexes with fullerenes C60 and C70 with association constants as high as (8.38 ± 0.33) × 104 M−1 and (7.64 ± 0.26) × 104 M−1, respectively.
Carbazole is one of the most commonly used building blocks for a wide range of organic electronic applications such as organic solar cells (OSCs), organic light-emitting diodes (OLEDs), organic field-effect transistors (OFETs) and so on.1 The inherent electron-donating ability of carbazole facilitates its hole-injection and transport, which are very conducive to realizing the excellent optoelectronic properties of carbazole-based organic functional materials, such as their good conductivity and high photoluminescence efficiency.2 Generally, the hole-injection process of the carbazole-based materials is often accompanied by the formation of a carbazole radical cation species (sometimes called a polaron), which has been intensively investigated in the fields of OSCs and OLEDs. However, the precise structural characterization of the carbazole radical cation species is relatively rare, especially the lack of the X-ray diffraction structure.3 This is largely due to the fact that most carbazole radical cation species are highly reactive and readily undergo dimerization and polymerization, and therefore usually unstable.4
Supramolecular macrocycles containing a variety of building blocks such as phenol,5 pyrrole,6 glycoluril,7para-dialkoxybenzene8 and so on9 are well known as guest receptors in supramolecular host–guest chemistry. Carbazole, one of the most common building blocks in organic synthesis,1,2 has also been widely used as a versatile building unit in supramolecular chemistry. Carbazole has several distinct merits such as its facile synthesis and easy modification, its structural rigidity with a well-defined molecular geometry, and its intriguing redox-active and luminescence properties, which make it ideal for the synthesis of a number of shape-persistent macrocycles with unique structures, novel properties, and potential applications. During the past decades, a variety of carbazole-based macrocyclic systems, including tricarbazole triazolophanes,10 carbazole-based Schiff base macrocycles,11 carbazolophanes,12 calix[n]carbazole,13 conjugated polycarbazoles,14 cyclo[4]carbazole,15 carbazole–ethynylene macrocycles,16 azacalix[2]arene[2]carbazoles,17 carbazole-based metallacycles18 and so on,19 have been successfully developed, and some of them have been shown to have potential applications in molecular recognition, catalysis, and supramolecular self-assembly (Scheme 1a). Notably, the nitrogen atom of the carbazole unit in most of the documented carbazole-based macrocycles is substituted with the alkyl side-chain, while aryl carbazole based macrocycles still remain unexplored. We infer that the substitution of the aryl group on the nitrogen atom of carbazole would have a pronounced effect on the properties of the resultant aryl carbazole based macrocycles.
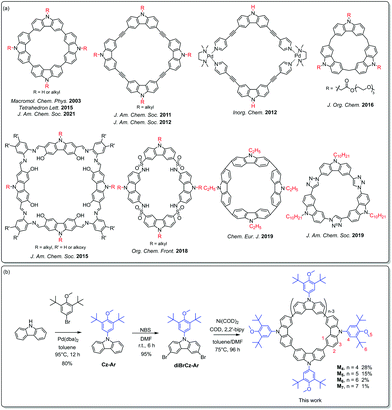 |
| Scheme 1 (a) Chemical structures of some representative carbazole-based macrocycles in the literature. (b) Synthesis of the macrocycles Mn (n = 4–7) consisting of N-(3,5-di-tert-butyl-4-methoxyphenyl) substituted carbazole (Cz-Ar). | |
In this work, we have designed and synthesized a series of fully conjugated macrocycles Mn (n = 4–7) consisting of N-(3,5-di-tert-butyl-4-methoxyphenyl) substituted carbazole (Cz-Ar) (Scheme 1b). The introduction of the 3,5-di-tert-butyl-4-methoxyphenyl group at the nitrogen atom of the carbazole reduced the ionization energy and enhanced the stability of the carbazole radical cation. Consequently, a mixed-valence radical cation of (diBrCz-Ar)2˙+ was successfully isolated in a stable single crystal form for the first time. The stability of the carbazole radical cation was further enhanced within the framework of M4 because of the efficient spin delocalization through the enlarged macrocyclic π-system. Moreover, the interesting host–guest interactions between these aryl carbazole-based macrocycles and fullerenes were also investigated.
Results and discussion
As shown in Scheme 1b, N-(3,5-di-tert-butyl-4-methoxyphenyl) substituted carbazole (Cz-Ar) was prepared through the Buchwald–Hartwig amination of carbazole with 5-bromo-1,3-di-tert-butyl-2-methoxybenzene in 80% yield. Bromination of Cz-Ar using N-bromosuccinimide in a dichloromethane (DCM) solution gave N-aryl-3,6-dibromocarbazole (diBrCz-Ar) in 95% yield. Subsequently, the Ni(cod)2/bpy-mediated homocoupling of diBrCz-Ar afforded a series of macrocycles Mn (n = 4–7),15 which were successfully separated and purified by preparative gel permeation chromatography (GPC), using DCM as an eluent. The tetramer M4, pentamer M5, hexamer M6 and heptamer M7 were obtained in 28%, 15%, 2% and 1% yields, respectively, and they exhibited excellent solubility in common organic solvents. The modest yields of M4 and M5 were attributed to the bulky aryl substituent which significantly enhanced their solubilities. In contrast, the Yamamoto homocoupling of N-butyl-3,6-dibromocarbazole (diBrCz-C4) gave the main product of the tetramer C4–M4, while other bigger analogues including the pentamer were hardly isolated due to their extremely low yields, which are mostly caused by their low solubilities (Fig. S1†).15 The structures of Cz-Ar, diBrCz-Ar, and macrocycles Mn were thoroughly characterized by 1H and 13C NMR and HR-MS measurements (see the ESI†).
Single crystals suitable for X-ray crystallographic analysis were obtained for M4 and M5 by slow diffusion of methanol into chloroform solution (Fig. 1), which unambiguously confirmed their macrocyclic structures. Tetramer M4 adopted a slightly curved conformation in the crystalline state (Fig. 1a), in contrast to the nearly planar structure of its N-alkyl substituted analogue C4–M4.15c Macrocycle M5 further twisted and adopted a saddle-shaped conformation (Fig. 1b). The cavity diameters of M4 and M5 were determined to be approximately 6.5 and 8.5 Å, respectively. The curved conformations and defined cavities of M4 and M5 may make them ideal host candidates to recognize fullerenes (vide infra). All attempts to grow single crystals for hexamer M6 and heptamer M7 were unsuccessful. Therefore, a computational prediction of their conformations was performed at the RB3LYP/6-311G(d,p) level of theory. The calculated molecular geometries of hexamer M6 and heptamer M7 revealed that the macrocycles became further distorted with the increase of their size (Fig. S8 and S9†).
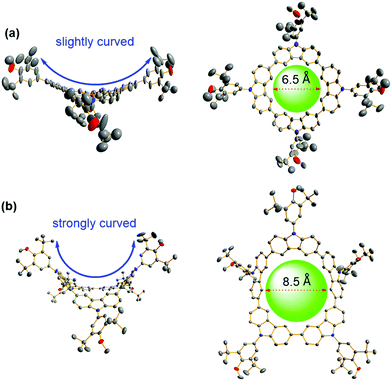 |
| Fig. 1 Crystal structures of tetramer M4 (a) and pentamer M5 (b). Ellipsoids are represented with 50% probability. Hydrogen atoms and the solvent are omitted for clarity. | |
To evaluate the effect of N-alkyl and N-aryl substituents on the ionization potential of carbazole, the redox properties of diBrCz-Ar and diBrCz-C4 were investigated by cyclic voltammetry (CV) in CH2Cl2 solution (potentials are referred vs. Fc/Fc+) (Fig. S2†). The CV of diBrCz-Ar and diBrCz-C4 exhibited one reversible oxidation wave with half-wave potentials of E1/2 = +0.94 V and +1.10 V, respectively. The ionization potential (IP) of diBrCz-Ar was estimated to be 5.26 eV, which was lower than that of diBrCz-C4 (IP = 5.35 eV), indicating that the aryl substituent group could really reduce the ionization potential of carbazole (Table S1†). Interestingly, the CV of tetramer M4 showed four quasi-reversible oxidation waves (Fig. S3†), and its ionization potential (IP = 4.66 eV) was significantly decreased compared with that of diBrCz-Ar (Table S1†). The reversible voltammogram means that the radical states of diBrCz-Ar and tetramer M4 are most likely to be very stable, which directs us to investigate their radical cation species.
The synthesis of the stable carbazole radical cation is highly difficult arising from the relatively high oxidation potential of carbazole and the high reactivity/instability of the carbazole radical cation.3,4 For example, unlike triarylamine derivatives that can be readily oxidized by some common oxidants such as AgSbF6, SbCl5, Cu(ClO4)2 and so on, N-substituted carbazole compounds encounter the difficulty of effective one-electron oxidation under mild reaction conditions. Besides, most of the N-substituted carbazole compounds undergo the dimerization or polymerization reaction during the oxidation process, resulting in major difficulties in the isolation and precise structural characterization of the carbazole radical cation species.4 As a consequence, 2,3-dichloro-5,6-dicyano-1,4-benzoquinone (DDQ) was employed as an oxidant because of its strong electron-withdrawing ability. The solution of diBrCz-Ar immediately turned green after the addition of DDQ (Fig. 2a), implying the successful generation of the corresponding radical cation species (diBrCz-Ar˙+).4b More specifically, the resultant green solution showed near-IR absorption beyond 1000 nm and this might suggest the formation of a singly charged primer, which was also observed in charged carbazole derivatives20 and other π-systems.21 The electron paramagnetic resonance (EPR) spectrum of diBrCz-Ar˙+ provided direct evidence for the presence of the radical species (Fig. 2b, blue solid line). In comparison, tetramer M4 could be oxidized by DDQ as well as AgSbF6 due to its lower ionization potential (Fig. 2a), which is consistent with the voltammogram results. The EPR spectrum of M4˙+ exhibited a strong single line in CH2Cl2 at room temperature (Fig. 2a, red solid line), implying efficient spin delocalization throughout the conjugated macrocycle. Notably, the radical cation species of diBrCz-Ar˙+ and M4˙+ were extremely stable, as evidenced by their almost unchanged UV–vis absorption spectra for up to one week (Fig. 2c, S4 and S5†). In contrast, the N-alkyl substituted analogue diBrCz-C4 could only be oxidized by DDQ in the presence of a strong acid (e.g., methanesulfonic acid).4b Moreover, the resultant radical cation species was very reactive, making it nearly impossible to purify and isolate diBrCz-C4˙+. The calculation results indicated that the spin density of the carbazole radical can be delocalized on the aryl group as well as the whole macrocycle, which might account for the enhanced stability of the radical cation species of diBrCz-Ar˙+ and M4˙+ (Fig. S10 and S11†).
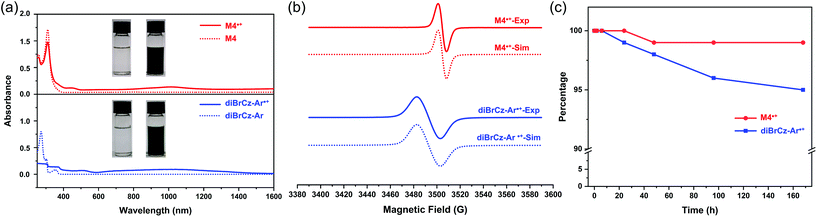 |
| Fig. 2 (a) UV-vis-NIR spectra of diBrCz-Ar (blue line, bottom) and M4 (red line, top) before (dashed line) and after (solid line) oxidation. The insets show the photographs of the solutions of neutral and oxidized diBrCz-Ar (bottom) and M4 (top). (b) EPR spectra and simulations of diBrCz-Ar˙+ (blue line, bottom) and M4˙+ (red line, top). (c) Evaluation of the stability of the radical cation species of diBrCz-Ar˙+ (blue column) and M4˙+ (red column) using UV-vis-NIR spectroscopy. | |
Fortunately, single crystals of the carbazole radical cation species suitable for X-ray crystallographic analysis were successfully obtained by slow evaporation of the dichloromethane solution (Fig. 3). Interestingly, crystal structure analysis revealed a chemical moiety with a formula of (diBrCz-Ar)2(DDQ). Two diBrCz-Ar molecules were associated into inversion-centred dyads, which further alternated with one DDQ molecule into a one-dimensional (1D) chain along the b-axis, wherein face-to-face packing was observed in the dimerized diBrCz-Ar (Fig. 3a). In contrast, the packing motif of the neutral diBrCz-Ar was different from those of (diBrCz-Ar)2(DDQ) and two diBrCz-Ar molecules stacked in an offset fashion (Fig. 3b). The large bond length alternation found in the aromatic ring of DDQ suggested a mixed-valence state in (diBrCz-Ar)2˙+(DDQ)˙− rather than a full charge-transfer in (diBrCz-Ar)22˙+(DDQ)2− (Fig. S24†).22 Thus, the carbazole radical cation species generated herein could be regarded as a mixed-valence radical cation of (diBrCz-Ar)2˙+. To the best of our knowledge, this is the first time that a quality crystal of the carbazole radical cation was presented.3 All attempts to grow single crystals for radical cation M4˙+ were unsuccessful. Thus, the structure of M4˙+ was simulated at the UB3LYP/6-311G(d,p) level of theory (Fig. S11b†), wherein the spin density was fully delocalized over the whole molecule (Fig. S11c†).
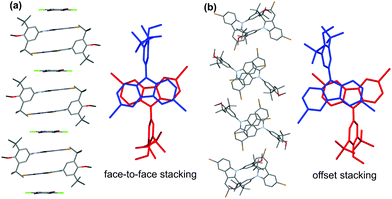 |
| Fig. 3 The crystal structures and packing motifs of (diBrCz-Ar)2(DDQ) (a) and diBrCz-Ar (b). Hydrogen atoms and the solvent are omitted for clarity. | |
Since tetramer M4 and pentamer M5 were relatively electron rich and possessed the distinct curved conformations and defined cavities, we speculated that they may serve as macrocyclic receptors towards fullerene recognition. The host–guest interactions between the macrocycles and fullerenes C60 and C70 were first examined by NMR spectroscopy. The 1H NMR spectrum of M4 remained unchanged when mixed with fullerene C60 or C70, implying negligible host–guest interactions between M4 and the fullerenes (Fig. 4a). Indeed, the cavity diameter (∼6.5 Å) of M4 did not match well with the size of the fullerenes (∼7.1 Å), and thus, it was too small to associate with them. In contrast, the addition of C60 or C70 to a solution of pentamer M5 in toluene-d8 resulted in an obvious downfield shift of proton H1 and slight upfield shifts of protons H2, H3, and H4 of M5 (Fig. 4b), illustrating their considerable host–guest interactions. The observed set of NMR peaks suggested that the host–guest complexation between M5 and the fullerenes was a fast-exchange process within the NMR timescale at 298 K.9l Moreover, the significant downfield shift of the inner proton H1 was likely to indicate that the fullerenes might be encapsulated within the curved surface of M5. In addition, the fluorescence intensity of M5 was quenched constantly with increasing concentration of C60 and C70 (Fig. 4c and d), further indicating their distinct host–guest interactions. To determine the binding stoichiometry of M5 and the fullerenes, the titration data were firstly obtained using the nonlinear regression models. The data were fitted to the association equilibria corresponding to the formation of inclusion complexes of 1
:
1 and 2
:
1 stoichiometries and a mixture of the two, respectively. Only the 1
:
1 model met the data, which indicated the presence of a 1
:
1 complex. Subsequently, Job plot analysis and MALDI-TOF MS also revealed a 1
:
1 stoichiometry of the complexation of M5 and the fullerenes (Fig. S18 and S19†). Fluorescence titration experiments in toluene solution also provided the association constants of (8.38 ± 0.33) × 104 M−1 and (7.64 ± 0.26) × 104 M−1 for M5 ⊃ C60 and M5 ⊃ C70, respectively, by fitting the concentration-dependent change of the emission intensity of M5. The moderate association constants unambiguously demonstrated the existence of the host–guest interactions between M5 and the fullerenes. Furthermore, the additional peak observed in the UV–vis spectrum of the mixture of M5 and C60 revealed the intriguing charge transfer interactions between the electron-rich M5 and the electron-deficient fullerene (Fig. S17†). To gain insight into the structural information of the host–guest complex and their host–guest interaction, molecular mechanics simulations at the RB3LYP-D3/6-311+G(d,p) level of theory were also performed. The simulated structures of the host–guest complexes M5 ⊃ C60 and M5 ⊃ C70 revealed that the fullerenes fitted perfectly inside the concave cavity of M5 through multiple π–π interactions (Fig. 4e and f). Therefore, the above results indicate that both the electron-rich nature of M5 and its distinct curved conformation play a key role in fullerene recognition.
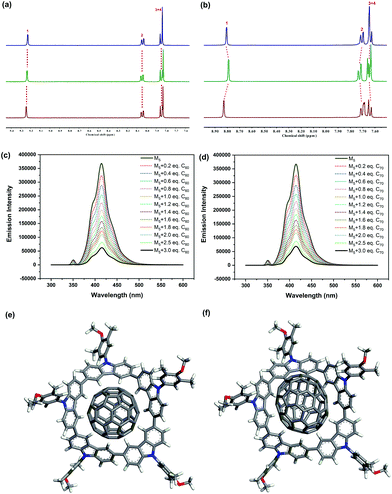 |
| Fig. 4 (a) Partial 1H NMR spectra (400 MHz, toluene-d8, 298 K) of solutions of 2.0 mM M4 + 2.0 mM C70 (top), 2.0 mM M4 (middle), and 2.0 mM M4 + 2.0 mM C60 (bottom). (b) Partial 1H NMR spectra (400 MHz, toluene-d8, 298 K) of solutions of 2.0 mM M5 + 2.0 mM C70 (top), 2.0 mM M5 (middle), and 2.0 mM M5 + 2.0 mM C60 (bottom). (c) Complexation process of M5 and C60. (d) Complexation process of M5 and C70. (e) Top view of the optimized geometry of M5 ⊃ C60 at the RB3LYP-D3/6-311+G(d,p) level of theory. (f) Top view of the optimized geometry of M5 ⊃ C70 at the RB3LYP-D3/6-311+G(d,p) level of theory. | |
Conclusions
In summary, we have successfully synthesized a series of fully conjugated aryl carbazole-based macrocycles Mn (n = 4–7) bearing a 3,5-di-tert-butyl-4-methoxyphenyl substituent. The bulky aryl group not only improved the solubility of the macrocycles but also reduced the ionization potential of the carbazole building block as well as the macrocycles. As a consequence, the aryl carbazole and M4 were readily oxidized into radical cation species in the presence of DDQ. The resultant radical cation species were found to be very stable because the spin density can be delocalized on the aryl group as well as the whole macrocycle. Notably, a mixed-valence radical cation of (diBrCz-Ar)2˙+ was successfully isolated and confirmed by X-ray crystallography. Moreover, pentamer M5 possessed a curved conformation and defined cavity, making it an ideal macrocyclic host candidate to recognize fullerenes. The excellent fullerene recognition ability of M5 was also attributed to the electron-rich nature of aryl carbazole, leading to the obvious charge transfer interactions between M5 and C60. The aryl-functionalization strategy in this study will shed some light on the design of novel macrocyclic arenes, which may exhibit some distinct properties compared with their alkyl substituted analogues.
Conflicts of interest
There are no conflicts to declare.
Acknowledgements
X. S. acknowledges the financial support sponsored by the NSFC, China (No. 22071061 and 52003081), the Shanghai Sailing Program (19YF1412900) and the Microscale Magnetic Resonance Platform of ECNU.
References
-
(a) J. V. Grazulevicius, P. Strohriegl, J. Pielichowski and K. Pielichowskic, Carbazole-containing polymers: synthesis, properties and applications, Prog. Polym. Sci., 2003, 28, 1297–1353 CrossRef CAS
;
(b) K. Ivaniuk, V. Cherpak, P. Stakhira, Z. Hotra, B. Minaev, G. Baryshnikov, E. Stromylo, D. Volyniuk, J. V. Grazulevicius, A. Lazauskas, S. Tamulevicius, B. Witulski, M. E. Light, P. Gawrys, R. J. Whitby, G. Wiosna-Salyga and B. Luszczynska, Highly luminous sky-blue organic light-emitting diodes based on the bis[(1,2)(5,6)]indoloanthracene emissive layer, J. Phys. Chem. C, 2016, 120, 6206–6217 CrossRef CAS
;
(c) Z. Zeng, X. Shi, C. Chi, J. T. L. Navarrete, J. Casado and J. Wu, Pro-aromatic and anti-aromatic π-conjugated molecules: an irresistible wish to be diradicals, Chem. Soc. Rev., 2015, 44, 6578–6596 RSC
.
-
(a) F. Dumur, Carbazole-based polymers as hosts for solution-processed organic light-emitting diodes: Simplicity, efficacy, Org. Electron., 2015, 25, 345–361 CrossRef CAS
;
(b) B. Wex and B. R. Kaafarani, Perspective on carbazole-based organic compounds as emitters and hosts in TADF applications, J. Mater. Chem. C, 2017, 5, 8622–8653 RSC
.
-
(a) Y. Tsujii, A. Tsuchida, Y. Onogi and M. Yamamoto, Stabilization of carbazole radical cation formed in poly (N-vinylcarbazole) by charge delocalization, Macromolecules, 1990, 23, 4019–4023 CrossRef CAS
;
(b) L. Fajarí, R. Papoular, M. Reig, E. Brillas, J. Jorda, O. Vallcorba, J. Rius, D. Velasco and L. Juliá, Charge transfer states in stable neutral and oxidized radical adducts from carbazole derivatives, J. Org. Chem., 2014, 79, 1771–1777 CrossRef PubMed
;
(c) S. Kasemthaveechok, L. Abella, M. Jean, M. Cordier, T. Roisnel, N. Vanthuyne, T. Guizouarn, O. Cador, J. Autschbach, J. Crassous and L. Favereau, Axially and helically chiral cationic radical bicarbazoles: SOMO–HOMO level inversion and chirality impact on the stability of mono- and diradical cations, J. Am. Chem. Soc., 2020, 142, 20409–20418 CrossRef CAS PubMed
.
-
(a) K. Karon and M. Lapkowski, Carbazole electrochemistry: a short review, J. Solid State Electrochem., 2015, 19, 2601–2610 CrossRef CAS
;
(b) S. Mallick, S. Maddala, K. Kollimalayan and P. Venkatakrishnan, Oxidative coupling of carbazoles: a substituent-governed regioselectivity profile, J. Org. Chem., 2019, 84, 73–93 CrossRef CAS PubMed
.
-
(a) N. Morohashi, F. Narumi, N. Iki, T. Hattori and S. Miyano, Thiacalixarenes, Chem. Rev., 2006, 106, 5291–5316 CrossRef CAS PubMed
;
(b) M.-X. Wang, Nitrogen and oxygen bridged calixaromatics: synthesis, structure, functionalization, and molecular recognition, Acc. Chem. Res., 2012, 45, 182–195 CrossRef CAS PubMed
;
(c) Y. Zhou, H. Li and Yi.-W. Yang, Controlled drug delivery systems based on calixarenes, Chin. Chem. Lett., 2015, 26, 825–828 CrossRef CAS
;
(d) J.-M. Yang, Y. Yu and J. Rebek Jr., Selective macrocycle formation in cavitands, J. Am. Chem. Soc., 2021, 143, 2190–2193 CrossRef CAS PubMed
.
-
(a) M. Ciardi, F. Tancini, G. Gil-Ramírez, E. C. E. Adán, C. Massera, E. Dalcanale and P. Ballester, Switching from separated to contact ion-pair binding modes with diastereomeric calix[4]pyrrole bis-phosphonate receptors, J. Am. Chem. Soc., 2012, 134, 13121–13132 CrossRef CAS PubMed
;
(b) S. K. Kim and J. L. Sessler, Calix[4]pyrrole-based ion pair receptors, Acc. Chem. Res., 2014, 47, 2525–2536 CrossRef CAS PubMed
.
-
(a) J. W. Lee, S. Samal, N. Selvapalam, H.-J. Kim and K. Kim, Cucurbituril homologues and derivatives: new opportunities in supramolecular chemistry, Acc. Chem. Res., 2003, 36, 621–630 CrossRef CAS PubMed
;
(b) S. J. Barrow, S. Kasera, M. J. Rowland, J. D. Barrio and O. A. Scherman, Cucurbituril-based molecular recognition, Chem. Rev., 2015, 115, 12320–12406 CrossRef CAS PubMed
;
(c) Y. Dong and L. Cao, Functionalization of Cucurbit uril, Prog. Chem., 2016, 28, 1039–1053 CAS
.
-
(a) T. Ogoshi, S. Kanai, S. Fujinami, T.-A. Yamagishi and Y. Nakamoto, Para-bridged symmetrical pillar[5]arenes: their Lewis acid catalyzed synthesis and host-guest property, J. Am. Chem. Soc., 2008, 130, 5022–5023 CrossRef CAS PubMed
;
(b) D. Cao, Y. Kou, J. Liang, Z. Chen, L. Wang and H. Meier, A facile and efficient preparation of pillararenes and a pillarquinone, Angew. Chem., Int. Ed., 2009, 48, 9721–9723 CrossRef CAS PubMed
;
(c) M. Xue, Y. Yang, X. Chi, Z. Zhang and F. Huang, Pillararenes, a new class of macrocycles for supramolecular chemistry, Acc. Chem. Res., 2012, 45, 1294–1308 CrossRef CAS PubMed
;
(d) T. Ogoshi, T.-A. Yamagishi and Y. Nakamoto, Pillararenes, a new class of macrocycles for supramolecular chemistry, Chem. Rev., 2016, 116, 7937–8002 CrossRef CAS PubMed
.
-
(a) R. Jasti, J. Bhattacharjee, J. B. Neaton and C. R. Bertozzi, Synthesis, characterization, and theory of [9]-,[12]-, and [18] cycloparaphenylene: carbon nanohoop structures, J. Am. Chem. Soc., 2008, 130, 17646–17647 CrossRef CAS PubMed
;
(b) A. Ito, Y. Yokoyama, R. Aihara, K. Fukui, S. Eguchi, K. Shizu, T. Sato and K. Tanaka, Preparation and Characterization of N-Anisyl-Substituted Hexaaza[16]paracyclophane, Angew. Chem., Int. Ed., 2010, 49, 8205–8208 CrossRef CAS PubMed
;
(c) W. Si, Z.-T. Li and J.-L. Hou, Voltage-driven reversible insertion into and leaving from a lipid bilayer: Tuning transmembrane transport of artificial channels, Angew. Chem., Int. Ed., 2014, 53, 4578–4581 CrossRef CAS PubMed
;
(d) H. Chen, J. Fan, X. Hu, J. Ma, S. Wang, J. Li, Y. Yu, X. Jia and C. Li, Biphen[n]arenes, Chem. Sci., 2015, 6, 197–202 RSC
;
(e) Y. Zhou, H.-Y. Zhang, Z.-Y. Zhang and Y. Liu, Tunable luminescent lanthanide supramolecular assembly based on photoreaction of anthracene, J. Am. Chem. Soc., 2017, 139, 7168–7171 CrossRef CAS PubMed
;
(f) Y. Yang, P. He, Y. Wang, H. Bai, S. Wang, J.-F. Xu and X. Zhang, Supramolecular radical anions triggered by bacteria in situ for selective photothermal therapy, Angew. Chem., Int. Ed., 2017, 56, 16239–16242 CrossRef CAS PubMed
;
(g) L. Mao, W. Pan, Y. Fu, L. Chen, M. Xu, Y. Ren, W. Feng and L. Yuan, Reversibly tunable lower critical solution temperature behavior induced by H-bonded aromatic amide macrocycle and imidazolium host–guest complexation, Org. Lett., 2017, 19, 18–21 CrossRef CAS PubMed
;
(h) S. Guo, Y. Song, Y. He, X.-Y. Hu and L. Wang, Highly efficient artificial light-harvesting systems constructed in aqueous solution based on supramolecular self-assembly, Angew. Chem., Int. Ed., 2018, 57, 3163–3167 CrossRef CAS PubMed
;
(i) W. Wang, C. Chen, C. Shu, S. Rajca, X. Wang and A. Rajca, S = 1 Tetraazacyclophane diradical dication with robust stability: a case of low-temperature one-dimensional antiferromagnetic chain, J. Am. Chem. Soc., 2018, 140, 7820–7826 CrossRef CAS PubMed
;
(j) H. Ke, L.-P. Yang, M. Xie, Z. Chen, H. Yao and W. Jiang, Shear-induced assembly of a transient yet highly stretchable hydrogel based on pseudopolyrotaxanes, Nat. Chem., 2019, 11, 470–477 CrossRef CAS PubMed
;
(k) L. Mao, Y. Hu, Q. Tu, W.-L. Jiang, X.-L. Zhao, W. Wang, D. Yuan, J. Wen and X. Shi, Highly efficient synthesis of non-planar macrocycles possessing intriguing self-assembling behaviors and ethene/ethyne capture properties, Nat. Commun., 2020, 11, 5806 CrossRef CAS PubMed
;
(l) J.-Q. Wang, Y. Han and C.-F. Chen, 3,6-Fluoren[5]arenes: synthesis, structure and complexation with fullerenes C60 and C70, Chem. Commun., 2021, 57, 3987–3990 RSC
;
(m) Y. Wang, H. Wu and J. F. Stoddart, Molecular triangles: a new class of macrocycles, Acc. Chem. Res., 2021, 54, 2027–2039 CrossRef CAS PubMed
;
(n) X. Lu, T. Y. Gopalakrishna, Y. Han, Y. Ni, Y. Zou and J. Wu, Bowl-shaped carbon nanobelts showing size-dependent properties and selective encapsulation of C70, J. Am. Chem. Soc., 2019, 141, 5934–5941 CrossRef CAS PubMed
.
- J. R. Dobscha, H. D. Castillo, Y. Li, R. E. Fadler, R. D. Taylor, A. A. Brown, C. Q. Trainor, S. L. Tait and A. H. Flood, Sequence-defined macrocycles for understanding and controlling the build-up of hierarchical order in self-assembled 2D arrays, J. Am. Chem. Soc., 2019, 141, 17588–17600 CrossRef CAS PubMed
.
- S.-i. Kawano, Y. Ishida and K. Tanaka, Columnar liquid-crystalline metallomacrocycles, J. Am. Chem. Soc., 2015, 137, 2295–2302 CrossRef CAS PubMed
.
-
(a) K. Tani, Y. Tohda, H. Takemura, H. Ohkita, S. Itoc and M. Yamamoto, Synthesis and photophysical properties of [3.3](3, 9) carbazolophanes, Chem. Commun., 2001, 1914–1915 RSC
;
(b) N. Sharma, E. Spuling, C. M. Mattern, W. Li, O. Fuhr, Y. Tsuchiya, C. Adachi, S. Bräse, I. D. W. Samuel and E. Zysman-Colman, Turn on of sky-blue thermally activated delayed fluorescence and circularly polarized luminescence (CPL) via increased torsion by a bulky carbazolophane donor, Chem. Sci., 2019, 10, 6689–6696 RSC
.
- P. Yang, Y. Jian, X. Zhou, G. Li, T. Deng, H. Shen, Z. Yang and Z. Tian, Calix[3]carbazole: one-step synthesis and host-guest binding, J. Org. Chem., 2016, 81, 2974–2980 CrossRef CAS PubMed
.
-
(a) D. Myśliwiec, M. Kondratowicz, T. Lis, P. J. Chmielewski and M. Stępień, Highly strained nonclassical nanotube end-caps. A single-step solution synthesis from strain-free, non-macrocyclic precursors, J. Am. Chem. Soc., 2015, 137, 1643–1649 CrossRef PubMed
;
(b) F. Lucas, L. Sicard, O. Jeannin, J. Rault-Berthelot, E. Jacques, C. Quinton and C. Poriel, [4]Cyclo-N-ethyl-2,7-carbazole synthesis, structural, electronic and charge transport properties, Chem. – Eur. J., 2019, 25, 7740–7748 CrossRef CAS PubMed
;
(c) Y. Kuroda, Y. Sakamoto, T. Suzuki, E. Kayahara and S. Yamago, Tetracyclo(2,7-carbazole)s: diatropicity and paratropicity of inner regions of nanohoops, J. Org. Chem., 2016, 81, 3356–3363 CrossRef CAS PubMed
;
(d) K. Senthilkumar, M. Kondratowicz, T. Lis, P. J. Chmielewski, J. Cybińska, J. Zafra, J. Casado, T. Vives, J. Crassous, L. Favereau and M. Stępien, Lemniscular [16]cycloparaphenylene: a radially conjugated figure-eight aromatic molecule, J. Am. Chem. Soc., 2019, 141, 7421–7427 CrossRef CAS PubMed
;
(e) M. Hermann, D. Wassy and B. Esser, Conjugated nanohoops incorporating donor, acceptor, hetero- or polycyclic aromatics, Angew. Chem., Int. Ed., 2021 DOI:10.1002/anie.202007024
.
-
(a) J. Ostrauskaite and P. Strohriegl, Formation of macrocycles in the synthesis of poly(N-(2-ethylhexyl)carbazol-3,6-diyl), Macromol. Chem. Phys., 2003, 204, 1713–1718 CrossRef CAS
;
(b) L. S. Coumont and J. G. C. Veinot, Ni(COD)2 coupling of 3, 6-dibromocarbazoles as a route to all-carbazole shape persistent macrocycles, Tetrahedron Lett., 2015, 56, 5595–5598 CrossRef CAS
;
(c) H. Zhu, B. Shi, K. Chen, P. Wei, D. Xia, J. H. Mondal and F. Huang, Cyclo[4]carbazole, an iodide anion macrocyclic receptor, Org. Lett., 2016, 18, 5054–5057 CrossRef CAS PubMed
;
(d) H. Zhu, I. Badía-Domínguez, B. Shi, Q. Li, P. Wei, H. Xing, M. C. R. Delgado and F. Huang, Cyclization-promoted ultralong low-temperature phosphorescence via boosting intersystem crossing, J. Am. Chem. Soc., 2021, 143, 2164–2169 CrossRef CAS PubMed
.
- K. Balakrishnan, A. Datar, W. Zhang, X. Yang, T. Naddo, J. Huang, J. Zuo, M. Yen, J. S. Moore and L. Zang, Nanofibril self-assembly of an arylene ethynylene macrocycle, J. Am. Chem. Soc., 2006, 128, 6576–6577 CrossRef CAS PubMed
.
- H. Xu, F.-J. Qian, Q.-X. Wu, M. Xue, Y. Yang and Y.-X. Chen, Azacalix[2]arene[2]carbazoles: synthesis, structure and properties, RSC Adv., 2016, 6, 27988–27992 RSC
.
- S. Shanmugaraju, V. Vajpayee, S. Lee, K.-W. Chi, P. J. Stang and P. S. Mukherjee, Coordination-driven self-assembly of 2D-metallamacrocycles using a new carbazole-based dipyridyl donor: synthesis, characterization, and C60 binding study, Inorg. Chem., 2012, 51, 4817–4823 CrossRef CAS PubMed
.
-
(a) A. Rüütel, V. Yrjänä, S. A. Kadam, I. Saar, M. Ilisson, A. Darnell, K. Haav, T. Haljasorg, L. Toom, J. Bobacka and I. Leito, Design, synthesis and application of carbazole macrocycles in anion sensors, Beilstein J. Org. Chem., 2020, 16, 1901–1914 CrossRef PubMed
;
(b) D. Myśliwiec, T. Lis, J. Gregoliński and M. Stępień, Stereoselective Wittig Olefination as a macrocyclization tool. synthesis of large carbazolophanes, J. Org. Chem., 2015, 80, 6300–6312 CrossRef PubMed
.
-
(a) T. Suzuki, W. Nojo, Y. Sakano, R. Katoono, Y. Ishigaki, H. Ohno and K. Fujiwara, Redox-induced conformational changes in 1,3-propylene- and m-xylylenebis[5-(10-butyl-5,10-dihydrobenzo[a]indolo[2,3-c]carbazole)]: twin-BIC donors that form sandwich-like dimeric cations exhibiting NIR absorption, Chem. Lett., 2016, 45, 720–722 CrossRef CAS
;
(b) W. Nojo, Y. Ishigaki, T. Takeda, T. Akutagawa and T. Suzuki, Selective formation of a mixed-valence state from linearly bridged oligo(aromatic diamines): drastic structural change into a folded columnar stack for half-filled polycations, Chem. – Eur. J., 2019, 25, 7759–7765 CrossRef CAS PubMed
.
-
(a) L. Ballester, A. Gutieérrez, M. F. Perpnñán and S. Rico, Magnetic behavior and crystal structure of [Fe(cyclam)(NCS)2](TCNQ)2: an unusual one-dimensional (TCNQ)2− radical-ion system, Inorg. Chem., 1999, 38, 4430–4434 CrossRef CAS PubMed
;
(b) V. Ganesan, S. V. Rosokha and J. K. Kochi, Isolation of the latent precursor complex in electron-transfer dynamics. Intermolecular association and self-exchange with acceptor anion radicals, J. Am. Chem. Soc., 2003, 125, 2559–2571 CrossRef CAS PubMed
;
(c) J.-M. Lü, S. V. Rosokha and J. K. Kochi, Stable (long-bonded) dimers via the quantitative self-association of different cationic, anionic, and uncharged π-radicals: structures, energetics, and optical transitions, J. Am. Chem. Soc., 2003, 125, 12161–12171 CrossRef PubMed
.
- J. S. Miller and D. A. Dixon, Dianion stabilization by (M(C5(CH3)5)2)+: theoretical evidence for a localized ring in (DDQ)2−, Science, 1987, 235, 871–873 CrossRef CAS PubMed
.
Footnote |
† Electronic supplementary information (ESI) available. CCDC 2071725 (diBrCz-Ar)2(DDQ), 2071727 (M4), 2071729 (M5) and 2073892 (diBrCz-Ar). For ESI and crystallographic data in CIF or other electronic format see DOI: 10.1039/d1qo00686j |
|
This journal is © the Partner Organisations 2021 |
Click here to see how this site uses Cookies. View our privacy policy here.