DOI:
10.1039/C7RA00352H
(Paper)
RSC Adv., 2017,
7, 15176-15180
TBHP-promoted direct oxidation reaction of benzylic Csp3–H bonds to ketones†
Received
9th January 2017
, Accepted 27th February 2017
First published on 7th March 2017
Abstract
A metal-free oxidation system employing tert-butyl hydroperoxide (TBHP) has been developed for selective oxidation of structurally diverse benzylic sp3 C–H bonds. This low-cost methodology allows for rapid generation of synthetically and biologically valued arylketones in good to excellent yields from readily available alkylarenes and diarylmethanes.
C–H bond activation reactions have become one of the most important classes of reactions in organic synthesis, the synthetic scope and utility of which has advanced considerably during the past decade.1 Undoubtedly, there are fundamental benefits for integrating such a strategy in organic synthesis, especially from the vantage point of environmental benignity and high atom/step economy. Furthermore, the construction of complex organic molecules nowadays can be designed in a more direct and effective fashion as these C–H functionalization transformations are an established piece in synthetic organic chemists' toolbox.1 Among this progress, the direct oxidation of C–H bonds for the synthesis of functionalized molecules has been of great interest in both academic and industrial settings.2 Many C–H oxidation methods have thus been developed to date.
Aryl ketones are widely recognized as key functional and structural motifs in a large plethora of significant molecules, including natural products, active pharmaceutical ingredients, agrochemicals and advanced materials (Fig. 1). Also, they frequently serve as useful precursors for the synthesis of complicated organic molecules.3 The classical methods to assemble such motifs involve the Friedel–Crafts acylation4 and transition metal catalyzed coupling reactions,5 which often suffer from poor selectivity and require harsh reaction conditions as well as toxic or expensive metal salts. In this way, direct benzylic Csp3–H oxidation reactions, as we proposed, could be a more ideal synthetic pathway.6 However, the literature survey suggested that most of the existing methods relied on less sustainable oxidants, such as potassium permanganate or chromium acid derivatives.7 The resulting vast metal residue not only caused operational challenges upon scale-up, but were also known as potential carcinogens, and long-term exposure to those hazardous metals might lead to central nervous system disorders.8 Consequently, less toxic, cost-effective and sustainable oxidants like peroxides or molecular oxygen, have drawn increasing research interest.
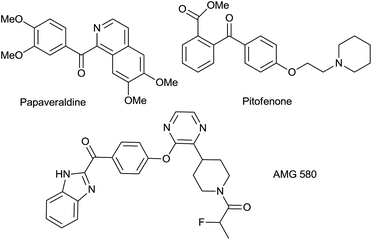 |
| Fig. 1 Selected aryl ketones. | |
In recent years, considerable progress has been made in this field.9 The group of Pandey and Lei independently reported a C–H aerobic oxidation approaches via photo-redox chemistry to synthesize aryl ketones.10 In addition, Li and Wang's group achieved the benzylic C–H oxidation by developing a recyclable TEMPO catalyst.11 Moreover, in 2016, Stahl et al. employed the electrochemical N-hydroxyphthalimide (NHPI) system to achieve benzylic oxygenation reaction to prepare (hetero)aryl ketones.12 Very recently, our group also reported a simple and efficient potassium tert-butoxide promoted (hetero)benzylic C–H oxidation reaction using oxygen as oxidant.13 Our approach provided a ready access to versatile diarylketones under mild conditions. While similar to most of other benzylic oxidation protocols, alkylbenzenes were unfortunately not amenable substrates in that report.14 Therefore, further research is highly necessary, as direct oxidative C–H functionalization of diverse substrates are still challenging and reported examples are limited in terms of substrate types. Since one of our lab's long-term goal is to develop efficient oxidation methods to construct valuable molecules, we herein would like to report our recent efforts in developing a simple and efficient tert-butyl hydroperoxide (TBHP) promoted benzylic sp3 C–H oxidation reaction to make aryl ketones.15
We began our studies by evaluating the oxygenation of ethylbenzene (1a) to acetophenone (2a), which proved to be challenging in our prior work.13 Key results of condition optimization are briefed in Table 1. The initial search for suitable oxidation conditions indicated that persulfates were ineffective oxidants (entries 1–2). We then moved to organic oxidizing agents. A wide array of peroxides and peroxy acids was thus examined (entries 3–8), among which, we were glad to find that the desired product 2a could be obtained with a superior 91% yield in the presence of TBHP (entry 8). Further investigation revealed that the amount of oxidizing agents is crucial to the reaction outcome, as the desired oxidation product was obtained with only 31% yield when 3 equivalents of TBHP was employed instead. It's also worth mentioning a decreased reaction temperature is detrimental to the rate of the oxidation step as well as the chemical yield, which is probably due to a reduced rate of radical generation step via thermal decomposition. Only moderate yield was attained when the transformation was performed at 100 °C (51% yield, entry 10).
Table 1 Reaction condition optimizationa
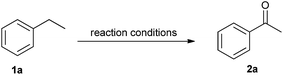
|
Entry |
Oxidant |
Yieldb (%) |
Ethylbenzene (1 mmol) and oxidant (12 mmol) were reacted at 130 °C for 10 h. Hexane is the solvent if the oxidant is solid. Determined by GC with internal standard mesitylene. TBHP (3 mmol) was used instead. Reaction temperature was 100 °C. |
1 |
(NH4)2S2O8 |
n.r. |
2 |
K2S2O8 |
n.r. |
3 |
m-CPBA |
n.r. |
4 |
DTBP |
n.r. |
5 |
CHP |
68% |
6 |
Dicumyl peroxide |
89% |
7 |
BPO |
82% |
8 |
TBHP |
91% |
9c |
TBHP |
31% |
10d |
TBHP |
51% |
With these optimized conditions in hand, we next began an exploration of the substrate scope. As revealed in Scheme 1, the benzylic C–H oxidation transformation could smoothly convert alkylarenes to the corresponding aryl ketones in excellent yields (2a–2c). 1,4-Diacetylbenzene (1d), which is a common building blocks for fluorophore type compound,16 could be obtained from corresponding starting material in 81% yield. Next, cyclic alkylarenes such as 2,3-dihydro-1H-indene (1e) and 1,2,3,4-tetrahydronaphthalene (1f) were employed to deliver the target ketone products in 81% and 87% yields respectively. Furthermore, diarylmethanes were compatible substrates, and reacted under the standard conditions to give the diarylketones (2h–2l) in good to excellent yields. In general, both the electron-donating and electron-withdrawing substitution groups had little effects on the chemical yields of oxidation products (2h–2l). In addition, fluorene (1m) proved to be suitable substrate, giving fluorenone (2m) in 81% yield. Notably, this oxidation protocol was discovered to be slightly sensitive to the steric hindrance at ortho-position of aromatic ring as 1-napthyl substituted diarylmethane substrate (1n) displayed lower reactivity to give only 75% yield to the desired ketone product 2n. Given the importance and synthetic challenges of nitrogen-containing heterocycles, we were delighted to find that pyridyl substituted substrate also reacted under this metal-free oxidation conditions to give the heteroaryl ketone 2g and 2o in good yields. Noteworthy, these pyridine-containing substrates often showed poor reactivity under metal-mediated benzylic oxidation reaction, which is probably due to the product inhibition via chelation.12 Finally, these optimized reaction conditions were examined for the oxygenation of pharmaceutically relevant imidazole substrates. 2-Benzoyl-1H-benzimidazole (2p), the core structural motif of potent phosphodiesterase inhibitor AMG 580, could be directly prepared in 70% yield.3l
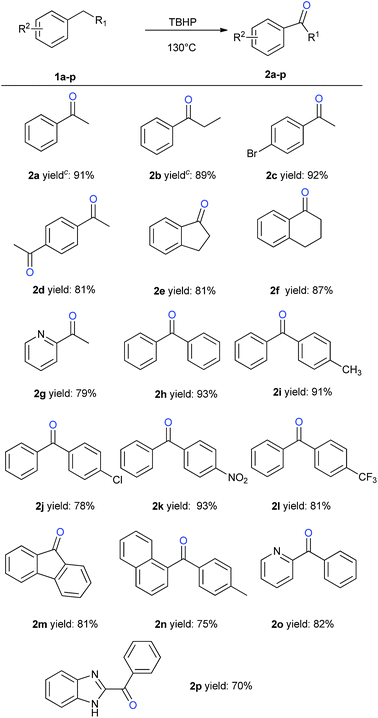 |
| Scheme 1 Substrate scope.ab a Alkylarene or diarylmethane (1 mmol), TBHP (hexane solution, 2 ml, ∼12 equiv.), 130 °C in pressure tube. b Isolated yields. c GC yields. | |
To expand the synthetic utility of this oxidative protocol, we then tested other benzylic sp3 C–H compounds as substrates. Under the identical reaction conditions, 2-phenylacetophenone (2q) underwent the oxidation reaction to give the benzoic acid (3q) instead of the desired benzyl product in 70% isolated yields (Scheme 2).10b
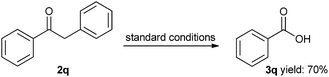 |
| Scheme 2 Oxidative reaction of 2-phenylacetophenone. | |
The scale-up synthesis of 2h also highlighted the utility and operational simplicity of this procedure. When the reaction was run at one-gram scale with diphenylmethane (1h), the product (2h) could still be obtained in a comparable 94% isolated yield (Scheme 3).
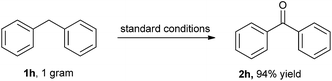 |
| Scheme 3 Scale-up synthesis. | |
To gain insight into this oxidative reaction, control experiments were employed as shown in Scheme 4. Firstly, the reaction was stopped after 5 hours, the oxidation product 2h and radical adducts 4 were both observed in 66% and 30% yields respectively. When intermediate 4 was subjected to the standard reaction conditions, the corresponding product 2h was also obtained in 91% yield. When the diphenylmethanol (5) was subjected to the standard reaction conditions, the desired ketone product 2h was also obtained with 95% yield. These results indicated that this transformation might involve benzylic peroxides and/or benzylic alcohols as the reaction intermediates.
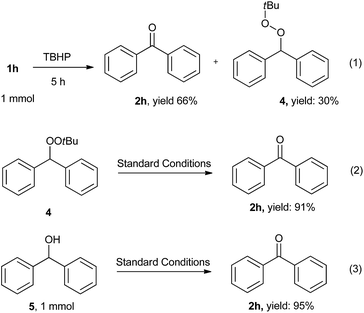 |
| Scheme 4 Control experiments. | |
On the basis of the above-mentioned experimental results and previous reports,17 a plausible reaction mechanism was proposed in Scheme 5. First, the decomposition of TBHP generated the oxygen-based radicals, which then underwent hydrogen abstraction of substrate A to generate intermediate B. The radical intermediate B can either react with ˙OH or ˙OOtBu to form the oxygenated intermediate C or D. The intermediate D either eliminated one molecule of tBuOH to directly form ketone E, or transformed into the benzyl alcohol C, which then underwent further oxidation to give final aryl ketone product E.
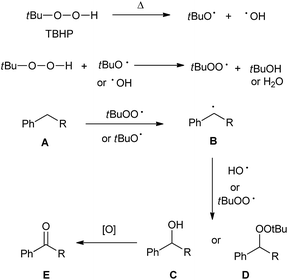 |
| Scheme 5 Proposed mechanism. | |
Conclusions
In summary, a simple metal-free TBHP system has been identified for direct oxidation of benzylic methylene groups to the corresponding aryl ketones. The radical-based reaction pathway tolerates a large variety of benzylic C–H bonds, giving the desired product in good to excellent yields. More importantly, the methodology is amenable to challenging heterocyclic substrates, such as pyridines and imidazoles. The synthetic utility of this protocol was further showcased in the gram-scale synthesis of diphenylketone. Further studies on both reaction scope and reaction mechanism are currently under investigation in our group.
Acknowledgements
We are grateful to Fundamental Research Funds for the Central Universities in Beijing University of Chemical Technology, and the Natural Science Foundation of China (21602119, U1504208), the financial support from He'nan Provincial Department of Science and Technology (152300410117).
Notes and references
- For selected recent reviews, see:
(a) L. McMurray, F. O'Hara and M. J. Gaunt, Chem. Soc. Rev., 2011, 40, 1885 RSC;
(b) C.-L. Sun, B.-J. Li and Z. J. Shi, Chem. Rev., 2011, 111, 1293 CrossRef CAS PubMed;
(c) T. Brueckl, R. D. Baxter, Y. Ishihara and P. S. Baran, Acc. Chem. Res., 2012, 45, 826 CrossRef PubMed;
(d) K. M. Engle, T.-S. Mei, M. Wasa and J.-Q. Yu, Acc. Chem. Res., 2012, 45, 788 CrossRef CAS PubMed;
(e) J. Yamaguchi, A. D. Yamaguchi and K. Itami, Angew. Chem., Int. Ed., 2012, 51, 8960 CrossRef CAS PubMed;
(f) S. R. Neufeldt and M. S. Sanford, Acc. Chem. Res., 2012, 45, 936 CrossRef CAS PubMed , and reference therein.
- For selected recent reviews, see:
(a) W. R. Gutekunst and P. S. Baran, Chem. Soc. Rev., 2011, 40, 1976 RSC;
(b) T. Newhouse and P. S. Baran, Angew. Chem., Int. Ed., 2011, 50, 3362 CrossRef CAS PubMed;
(c) C. Liu, H. Zhang, W. Shi and A. Lei, Chem. Rev., 2011, 111, 1780 CrossRef CAS PubMed;
(d) A. N. Campbell and S. S. Stahl, Acc. Chem. Res., 2012, 45, 851 CrossRef CAS PubMed , and reference therein.
-
(a) H. G. Franck and J. W. Stadelhofer, Industrial Aromatic Chemistry, Springer-Verlag, Berlin, 1988 Search PubMed;
(b) N. J. Lawrence, J. Chem. Soc., Perkin Trans. 1, 1998, 1739 RSC;
(c) R. K. Dieter, Tetrahedron, 1999, 55, 4177 CrossRef CAS;
(d) H. Surburg and J. Panten, Common Fragrance and Flavor Materials, Wiley-VCH, Weinheim, Germany, 5th edn, 2006 Search PubMed;
(e) M. Mizuno, A. Inagaki, M. Yamashita, N. Soma, Y. Maeda and H. Nakatani, Tetrahedron, 2006, 62, 4065 CrossRef CAS;
(f) A. J. Whitehead, R. A. Ward and M. F. Jones, Tetrahedron Lett., 2007, 48, 911 CrossRef CAS;
(g) H. B. Yang, K. Ghosh, Y. Zhao, B. H. Northrop, M. M. Lyndon, D. C. Muddiman, H. S. White and P. J. Stang, J. Am. Chem. Soc., 2008, 130, 839 CrossRef CAS PubMed;
(h) S. K. Vooturi, C. M. Cheung, M. J. Rybak and S. M. Firestine, J. Med. Chem., 2009, 52, 5020 CrossRef CAS PubMed;
(i) J. R. Luque-Ortega, P. Reuther, L. Rivas and C. Dardonville, J. Med. Chem., 2010, 53, 1788 CrossRef CAS PubMed;
(j) A. Shimada, I. Takahashi, T. Kawano and Y. Kimura, Z. Naturforsch., B: J. Chem. Sci., 2011, 56, 797 Search PubMed;
(k) Q.-F. Hu, B. Zhou, J.-M. Huang, X.-M. Gao, L.-D. Shu, G.-Y. Yang and C.-T. Che, J. Nat. Prod., 2013, 76, 292 CrossRef CAS PubMed;
(l) H. Chen, D. Lester-Zeiner, J. Shi, S. Miller, C. Glaus, E. Hu, N. Chen, J. Able, C. Biorn, J. Wong, J. Ma, K. Michelsen, G. Hill Della Puppa, T. Kazules, H. Dou, S. Talreja, X. Zhao, A. Chen, S. Rumfelt, R. K. Kunz, H. Ye, O. R. Thiel, T. Williamson, C. Davis, A. Porter, D. Immke, J. R. Allen and J. Treanor, J. Pharmacol. Exp. Ther., 2015, 352, 327 CrossRef PubMed.
-
(a) A. Furstner, D. Voigtlander, W. Schrader, D. Giebel and M. T. Reetz, Org. Lett., 2001, 3, 417 CrossRef CAS PubMed;
(b) S. Gmouh, H. L. Yang and M. Vaultier, Org. Lett., 2003, 5, 2219 CrossRef CAS PubMed;
(c) G. Sartori and R. Maggi, Advances in Friedel–Crafts Acylation Reactions, CRC Press, Boca Raton, FL, 2010 Search PubMed;
(d) P. H. Tran, P. E. Hansen, H. T. Nguyen and T. N. Le, Tetrahedron Lett., 2015, 56, 612 CrossRef CAS.
- For a most recent review, see:
(a) X.-F. Wu, Chem.–Eur. J., 2015, 21, 12252 CrossRef CAS PubMed; For selected transition-metal-catalyzed reactions, see:
(b) S. Ma and Z. Gu, Angew. Chem., Int. Ed., 2005, 44, 7512 CrossRef CAS PubMed;
(c) X. Jia, S. Zhang, W. Wang, F. Luo and J. Cheng, Org. Lett., 2009, 11, 3120 CrossRef CAS PubMed;
(d) C. W. Chan, Z. Zhou, A. S. C. Chan and W. Y. Yu, Org. Lett., 2010, 12, 3926 CrossRef CAS PubMed;
(e) Y. Lu, D. Leow, X. Wang, K. M. Engle and J.-Q. Yu, Chem. Sci., 2011, 2, 967 RSC;
(f) M. Z. Li, C. Wang and H. B. Ge, Org. Lett., 2011, 13, 2062 CrossRef CAS PubMed;
(g) Z. Yin and P. Sun, J. Org. Chem., 2012, 77, 11339 CrossRef CAS PubMed;
(h) Q. Zhang, F. Yang and Y. Wu, Chem. Commun., 2013, 49, 6837 RSC;
(i) P. Mamone, G. Danoun and L. J. Gooßen, Angew. Chem., Int. Ed., 2013, 52, 6704 CrossRef CAS PubMed;
(j) C. Pi, X. Cui, X. Liu, M. Guo, H. Zhang and Y. Wu, Org. Lett., 2014, 16, 5164 CrossRef CAS PubMed;
(k) S. D. Ramgren and N. K. Garg, Org. Lett., 2014, 16, 824 CrossRef CAS PubMed , and reference therein.
- For selected recent reports, see:
(a) X. Fan, Y. He, X. Zhang, S. Guo and Y. Wang, Tetrahedron, 2011, 67, 6369 CrossRef CAS;
(b) C. Zhang, Z. Xu, L. Zhang and N. Jiao, Angew. Chem., Int. Ed., 2011, 50, 11088 CrossRef CAS PubMed;
(c) X. Fan, Y. He, Y. Wang, Z. Xue, X. Zhang and J. Wang, Tetrahedron Lett., 2011, 52, 899 CrossRef CAS;
(d) J. De Houwer, K. Abbaspour Tehrani and B. U. W. Maes, Angew. Chem., Int. Ed., 2012, 51, 2745 CrossRef CAS PubMed;
(e) C. Zhang, Z. Xu, L. Zhang and N. Jiao, Tetrahedron, 2012, 68, 5258 CrossRef CAS;
(f) Y.-F. Wang, F.-L. Zhang and S. Chiba, Synthesis, 2012, 44, 1526 CrossRef CAS;
(g) C. Menendez, S. Gau, S. Ladeira, C. Lherbet and M. Baltas, Eur. J. Org. Chem., 2012, 409 CrossRef CAS;
(h) Z. Xu, C. Zhang and N. Jiao, Angew. Chem., Int. Ed., 2012, 51, 11367 CrossRef CAS PubMed;
(i) C. Zhang, L. Zhang and N. Jiao, Adv. Synth. Catal., 2012, 354, 1293 CrossRef CAS;
(j) S. Cacchi, G. Fabrizi, A. Goggiamani, A. Iazzetti and R. Verdiglione, Synthesis, 2013, 45, 1701 CrossRef CAS;
(k) B. Pieber and C. O. Kappe, Green Chem., 2013, 15, 320 RSC;
(l) A. Dos Santos, L. El Kaïm and L. Grimaud, Org. Biomol. Chem., 2013, 11, 3282 RSC;
(m) Q. Li, Y. Huang, T. Chen, Y. Zhou, Q. Xu, S.-F. Yin and L.-B. Han, Org. Lett., 2014, 16, 3672 CrossRef CAS PubMed;
(n) J.-W. Yu, S. Mao and Y.-Q. Wang, Tetrahedron Lett., 2015, 56, 1575 CrossRef CAS;
(o) Y. Huang, T. Chen, Q. Li, Y. Zhou and S.-F. Yin, Org. Biomol. Chem., 2015, 13, 7289 RSC;
(p) H. Xie, Y. Liao, S. Chen, Y. Chen and G.-J. Deng, Org. Biomol. Chem., 2015, 13, 6944 RSC;
(q) L. Ren, L. Wang, Y. Lv, G. Li and S. Gao, Org. Lett., 2015, 17, 2078 CrossRef CAS PubMed;
(r) Y. Jeong, Y. Moon and S. Hong, Org. Lett., 2015, 17, 3252 CrossRef CAS PubMed;
(s) H. Sterckx, J. DeHouwer, C. Mensch, I. Caretti, K. A. Tehrani, W. A. Herrebout, S. Van Doorslaer and B. U. W. Maes, Chem. Sci., 2016, 7, 346 RSC , and reference therein.
- P. Anastas and N. Eghbali, Chem. Soc. Rev., 2010, 39, 301 RSC.
-
(a) A. Wells, M. Teria and T. Eve, Biochem. Soc. Trans., 2006, 34, 304 CrossRef CAS PubMed;
(b) D. Avila, R. Puntel and M. Aschner, Interrelations Between Essential Metal Ions and Human Diseases, ed. A. Sigel, H. Sigel and R. K. O. Sigel, Springer, Netherlands, 2013, vol. 13, pp. 199–227 Search PubMed.
-
(a) A. M. Khenkin and R. Neumann, J. Am. Chem. Soc., 2002, 124, 4198 CrossRef CAS PubMed;
(b) L. Gonsalvi, I. W. C. E. Arends, P. Moilanen and R. A. Sheldon, Adv. Synth. Catal., 2003, 345, 1321 CrossRef CAS;
(c) A. J. Catino, J. M. Nichols, H. Choi, S. Gottipamula and M. P. Doyle, Org. Lett., 2005, 7, 5167 CrossRef CAS PubMed;
(d) C. Pavan, J. Legros and C. Bolm, Adv. Synth. Catal., 2005, 347, 703 CrossRef CAS;
(e) Y. Bonvin, E. Callens, L. Larrosa, D. A. Henderson, J. Oldham, A. J. Burton and A. G. M. Barrett, Org. Lett., 2005, 7, 4549 CrossRef CAS PubMed;
(f) M. Nakanishi and C. Bolm, Adv. Synth. Catal., 2007, 349, 861 CrossRef CAS;
(g) C. S. Yi, K. H. Kwon and D. W. Lee, Org. Lett., 2009, 11, 1567 CrossRef CAS PubMed;
(h) J. Zhang, Z. Wang, Y. Wang, C. Wan, X. Zheng and Z. Wang, Green Chem., 2009, 11, 1973 RSC;
(i) H. Li, Z. Li and Z. Shi, Tetrahedron, 2009, 65, 1856 CrossRef CAS;
(j) R. A. Kumar, C. U. Maheswari, S. Ghantasala, C. Jyothi and K. R. Reddy, Adv. Synth. Catal., 2011, 353, 401 CrossRef CAS;
(k) K. Moriyama, M. Takemura and H. Togo, Org. Lett., 2012, 14, 2414 CrossRef CAS PubMed;
(l) Y. J. Xie, Y. Z. Yang, L. H. Huang, X. B. Zhang and Y. H. Zhang, Org. Lett., 2012, 14, 1238 CrossRef CAS PubMed;
(m) A. V. Biradar and T. Asefa, Appl. Catal., A, 2012, 19 CrossRef CAS;
(n) H. Peng, A. Lin, Y. Zhang, H. Jiang, J. Zhou, Y. Cheng, C. Zhu and H. Hu, ACS Catal., 2012, 2, 163 CrossRef CAS;
(o) X. F. Wu, Tetrahedron Lett., 2012, 53, 6123 CrossRef CAS;
(p) L. Meng, J. Su, Z. Zha, L. Zhang, Z. Zhang and Z. Wang, Chem.–Eur. J., 2013, 19, 5542 CrossRef CAS PubMed;
(q) C. He, X. H. Zhang, R. F. Huang, J. Pan, J. Q. Li, X. G. Ling, Y. Xiong and X. M. Zhu, Tetrahedron Lett., 2014, 55, 4458 CrossRef CAS;
(r) D. Shen, C. Miao, S. Wang, C. Xia and W. Sun, Org. Lett., 2014, 16, 1108 CrossRef CAS PubMed;
(s) W. J. Ang and Y. L. Lam, Org. Biomol. Chem., 2015, 13, 1048 RSC , and reference therein.
-
(a) G. Pandey, S. Pal and R. Laha, Angew. Chem., Int. Ed., 2013, 52, 5146 CrossRef CAS PubMed;
(b) H. Yi, C. Bian, X. Hu, L. Niu and A. Lei, Chem. Commun., 2015, 51, 14046 RSC.
- Z. Zhang, Y. Gao, Y. Liu, J. Li, H. Xie, H. Li and W. Wang, Org. Lett., 2015, 17, 5492 CrossRef CAS PubMed.
-
(a) D. P. Hruszkewycz, K. C. Miles, O. R. Thiel and S. S. Stahl, Chem. Sci., 2017, 8, 1282 RSC. For selected reviews on oxidation reaction mediated by NHPI, see:
(b) F. Recupero and C. Punta, Chem. Rev., 2007, 107, 3800 CrossRef CAS PubMed;
(c) L. Melone and C. Punta, Beilstein J. Org. Chem., 2013, 9, 1296 CrossRef CAS PubMed.
- H.-Q. Wang, Z. Wang, H.-C. Huang, J.-J. Tan and K. Xu, Org. Lett., 2016, 18, 5680 CrossRef CAS PubMed.
- For selected examples on the oxidation of alkylarenes to Ketones by TBHP, see:
(a) H. He, B.-J. Pei and A. W. M. Lee, Green Chem., 2009, 11, 1857 RSC;
(b) J. T. Zhang, Z. T. Wang, Y. Wang, C. F. Wan, X. Q. Zheng and Z. Y. Wang, Green Chem., 2009, 11, 1973 RSC , and reference therein.
- For examples on oxidation reactions of benzylic Csp3–H bond to ketones under metal-free conditions, see: K. X. Chen, P. F. Zhang, Y. Wang and H. R. Li, Green Chem., 2014, 16, 2344 RSC , and references cited therein.
- J. Y. Koo, C. H. Heo, Y.-H. Shin, D. Kim, C. S. Lim, B. R. Cho, H. M. Kim and S. B. Park, Chem.–Eur. J., 2016, 22, 14166 CrossRef CAS PubMed.
-
(a) J. Muzart, Tetrahedron Lett., 1986, 27, 3139 CrossRef CAS;
(b) J. Muzart, Synth. Commun., 1989, 19, 2061 CrossRef CAS;
(c) A. A. Tarlani, A. Riahi, M. Abedini, M. Mohammadpour Amini and J. Muzart, Appl. Catal., A, 2006, 315, 150 CrossRef CAS;
(d) J. A. S. Coelho, A. F. Trindade, R. Wanke, B. G. M. Rocha, L. F. Veiros, P. M. P. Gois, A. J. L. Pombeiro and C. A. M. Afonso, Eur. J. Org. Chem., 2013, 1471 CrossRef CAS.
Footnote |
† Electronic supplementary information (ESI) available. See DOI: 10.1039/c7ra00352h |
|
This journal is © The Royal Society of Chemistry 2017 |
Click here to see how this site uses Cookies. View our privacy policy here.