DOI:
10.1039/C4QO00347K
(Research Article)
Org. Chem. Front., 2015,
2, 398-402
Pd(II)-catalyzed asymmetric addition of arylboronic acids to cyclic N-sulfonyl ketimine esters and a DFT study of its mechanism†‡
Received
23rd December 2014
, Accepted 9th February 2015
First published on 11th February 2015
Abstract
A palladium-catalyzed asymmetric arylation of cyclic N-sulfonyl ketimine esters is described. The desired products could be prepared with excellent yields (up to 99%) and enantioselectivities (up to 99% ee) under mild reaction conditions. Furthermore, a possible reaction mechanism was determined using DFT calculations.
1. Introduction
The asymmetric formation of chiral tetrasubstituted carbon centers remains a challenge until now.1 Chiral α-tertiary amines exist in numerous natural products and drugs,2 thus many chemists are interested in their synthesis.3 Among the reactions involving the construction of chiral α-tertiary amines, the metal-catalyzed asymmetric addition to imines represents a powerful methodology for the synthesis of chiral α-tertiary amines.4–9 Several groups have focused their research efforts towards the metal-catalyzed addition of arylboronic acids to ketimines.5–9 The Hayashi group6 has pioneered the rhodium-catalyzed asymmetric addition of arylboron reagents to ketimines which possess various functional groups. In addition, the groups of Lam,7 Xu,8 and Lin9 have also reported the rhodium-catalyzed asymmetric addition of organoboron reagents to ketimines. Alternatively, using palladium catalysts in the same reaction is an attractive methodology because of their lower cost compared to rhodium catalysts.10–13 The Lu group11a,b has pioneered the palladium-catalyzed asymmetric addition of arylboronic acids to aldimines. Besides, several other groups have also reported related studies.11c–f However, examples concerning the palladium-catalyzed asymmetric addition of arylboronic acids to ketimines are rare. Only recently, our group12 and Lu/Hayashi13 reported two different catalytic systems utilizing palladium catalysts for the arylation of cyclic N-sulfonyl ketimines. Therefore, more studies in this area are highly desired.
α-Tertiary amino esters reside within a wide assortment of natural products and drugs14 and can be easily converted to unnatural amino acids and amino alcohols, which possess greater potential value. In addition, to the best of our knowledge, there is no precedent for mechanistic studies concerning the palladium-catalyzed arylation of imines. Herein we report the synthesis of α-tertiary amino esters via the palladium-catalyzed asymmetric addition of arylboronic acids to cyclic N-sulfonyl ester imines. Furthermore, the mechanism of this reaction has been studied using DFT calculations.
2. Results and discussion
We realized an efficient Pd-catalyzed arylation of substrate 1a using (S)-methyl-6-(4-tert-butyl-4,5-dihydrooxazol-2-yl)nicotinate (L1a) as a chiral ligand, and Pd(TFA)2 as the Pd source in TFE at 60 °C (98% ee; Table 1, entry 1). Table 1 provides information on the impact of various reaction parameters on the efficiency of this catalytic asymmetric synthesis of α-tertiary amino esters. When different chiral pyridine-oxazoline-type ligands were tested, product yields were all excellent and L1a gave the best ee (entries 1–6). The enantioselectivity decreased with smaller R groups at the chiral position of the ligand (entries 1–3 and entries 4–6). The COOMe group on the pyridine ring plays an important role in enantioselectivity (entries 1 vs. 4, 2 vs. 5, 3 vs. 6, respectively). Different palladium salts were also investigated. When Pd(OAc)2 was tested, both the enantioselectivity and yield decreased (entry 7). However, only a trace amount of the product was obtained and most of the reactant was recovered when PdCl2 was used, probably due to the strong coordinating ability of the chloride ion to palladium. In our previous work,12 alcohols had a beneficial effect on the rate of transmetalation and protonation steps of the catalytic cycle, thus MeOH and EtOH were also screened as solvents (entries 9–11). However, only trace amounts of the desired product were detected in these cases. When the reaction was carried out under an N2 atmosphere, a slightly lower yield was obtained with no change of ee (entry 12). Probably because some Pd(0) was formed by self-coupling of phenylboronic acid, which cannot be oxidized to catalytically active Pd(II) by O2 under an N2 atmosphere.
Table 1 Conditions screeninga
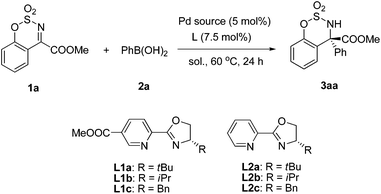
|
Entry |
Ligand |
Pd source |
Solvent |
Yieldb (%) |
eec (%) |
Reactions were carried out in air on a 0.20 mmol scale using 5 mol% PdX2, 7.5 mol% ligand in the unpurified solvent (2.0 mL) and PhB(OH)2 (0.30 mmol) at 60 °C in a test tube for 24 h which was opened to air.
Yield of the isolated product.
Determined by HPLC using a chiral Daicel column.
Palladium black was formed.
Charged with oxygen in a sealed tube. TFA = trifluoroacetic acetate, TFE = trifluoroethanol.
The reaction was carried out under N2.
|
1 |
L1a
|
Pd(TFA)2 |
TFE |
99 |
98 |
2 |
L1b
|
Pd(TFA)2 |
TFE |
99 |
73 |
3 |
L1c
|
Pd(TFA)2 |
TFE |
99 |
50 |
4 |
L2a
|
Pd(TFA)2 |
TFE |
99 |
87 |
5 |
L2b
|
Pd(TFA)2 |
TFE |
92 |
62 |
6 |
L2c
|
Pd(TFA)2 |
TFE |
96 |
50 |
7 |
L1a
|
Pd(OAc)2 |
TFE |
82 |
93 |
8 |
L1a
|
PdCl2 |
TFE |
Trace |
— |
9d |
L1a
|
Pd(TFA)2 |
MeOH |
Trace |
— |
10d |
L1a
|
Pd(TFA)2 |
EtOH |
Trace |
— |
11e |
L1a
|
Pd(TFA)2 |
EtOH |
Trace |
— |
12f |
L1a
|
Pd(TFA)2 |
TFE |
90 |
98 |
With the optimized conditions in hand, the arylboronic acid scope was examined, as shown in Table 2. The results showed that arylboronic acids bearing electron-donating substituent groups on the benzene ring gave excellent yields and enantioselectivities (3aa–3ae). Slightly electron-deficient arylboronic acids also gave good results but the temperature needed to be increased to 80 °C (3af–3ah). However, a decrease in the reaction rate was observed for arylboronic acids bearing a COOMe group (3ai). The fused-ring aryl or heteroaryl boronic acids also gave excellent ees but only moderate yields (3aj–3ak).
Table 2 Scope of arylboronic acidsa
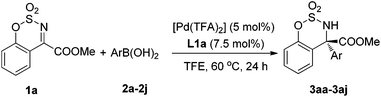
|
Entry |
2
|
Ar |
Yieldb (%) |
eec (%) |
Reactions were carried out in air on a 0.20 mmol scale using 5 mol% Pd(TFA)2, 7.5 mol% ligand and ArB(OH)2 (0.30 mmol) in the unpurified TFE (2.0 mL) at 60 °C in a test tube for 24 h which was opened to air.
Isolated yields. ees were determined by chiral HPLC.
80 °C.
Sealed tube charged with oxygen.
36 h.
|
1 |
2a
|
C6H5 |
99 |
98 |
2 |
2b
|
3-MeC6H4 |
99 |
99 |
3 |
2c
|
4-MeC6H4 |
99 |
98 |
4 |
2d
|
4-MeOC6H4 |
99 |
99 |
5 |
2e
|
4-PhC6H4 |
92 |
99 |
6c |
2f
|
4-FC6H4 |
90 |
98 |
7c |
2g
|
4-ClC6H4 |
94 |
98 |
8c |
2h
|
4-BrC6H4 |
92 |
98 |
9c,d |
2i
|
4-MeO2CC6H4 |
52 |
97 |
10c,d |
2j
|
2-Naphthyl |
74 |
99 |
11c,d,e |
2k
|
3-Thienyl |
67 |
96 |
The substrate scope was also investigated and the results are summarized in Table 3. They show that the substrates possessing both electron-donating and electron-withdrawing substituents gave the corresponding products with excellent yields and enantioselectivities (3bb–3hb). Furthermore, the substitution position on the benzene ring has little influence on the results, and excellent yields and ees could be obtained in all cases (3bb–3db). Additionally, when the aryl group was changed to naphthyl, good ee was also observed but a prolonged reaction time was required (3ib). However, the reactivity decreased sharply for substrates bearing COOEt or COOnPr ester groups. Thus, a sealed tube charged with oxygen was required to give the desired products with excellent yields and ees (3jb–3kb).
Table 3 Scope of substratesa
Reactions were carried out in air on a 0.20 mmol scale using 5 mol% Pd(TFA)2, 7.5 mol% ligand and ArB(OH)2 (0.30 mmol) in the unpurified TFE (2.0 mL) at 60 °C in a test tube for 24 h which was opened to air. The yields were isolated yields and ees were determined by chiral HPLC.
36 h.
48 h.
Charged with oxygen in a sealed tube.
|
|
The asymmetric arylation product 3cb could be reduced to unnatural amino alcohol 4, with the –SO2– group deprotected at the same time. The yield was high and the ee value was increased to 99.9% (Scheme 1).7a
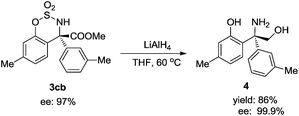 |
| Scheme 1 Reduction of arylation product 3cb. | |
The mechanism of the palladium-catalyzed conjugate addition of arylboronic acids to α,β-unsaturated compounds has been studied via DFT calculations.15 Although several groups have reported the Pd-catalyzed addition of arylboronic acids to imines, there are no reports concerning mechanistic studies for this reaction.11–13 Herein, a DFT study was carried out and the results are shown in Fig. 1. Firstly, phenylboronic acid coordinates with the Pd(II) catalyst to form intermediate 5. The benzene ring then transfers to palladium to generate a phenyl–palladium–boronic acid complex with a free energy barrier of 11.5 kcal mol−1 (5-TS and 6). Following this, trifluoroethoxyboronic acid leaves from intermediate 6 and a relatively unstable phenyl-palladium species 7 is formed. The nitrogen atom of substrate 1a coordinates with palladium to lower the positive charge so that the intermediate 8 is more stable. The phenyl group then transfers to the carbon atom which is adjacent to the nitrogen atom of the substrate with a free energy barrier of 19.8 kcal mol−1 (8 and 8-TSR). This step is the rate-determining step and also the stereoselectivity-determining step. The absolute configuration of the product was determined to be R by X-ray crystallographic analysis (Fig. 2),16 consistent with the calculation result. The enantiomeric imine insertion transition state 8-TSS requires a 7.7 kcal mol−1 higher activation free energy than 8-TSR, indicating that the formation of the S-configuration is unfavorable due to the strong steric interaction between the coordinated substrate and the But group of the catalyst. Finally, a TFE solvent molecule combines with the product-palladium species 9. A proton from the combined solvent transfers to the nitrogen atom to form the product following the release of the catalyst.
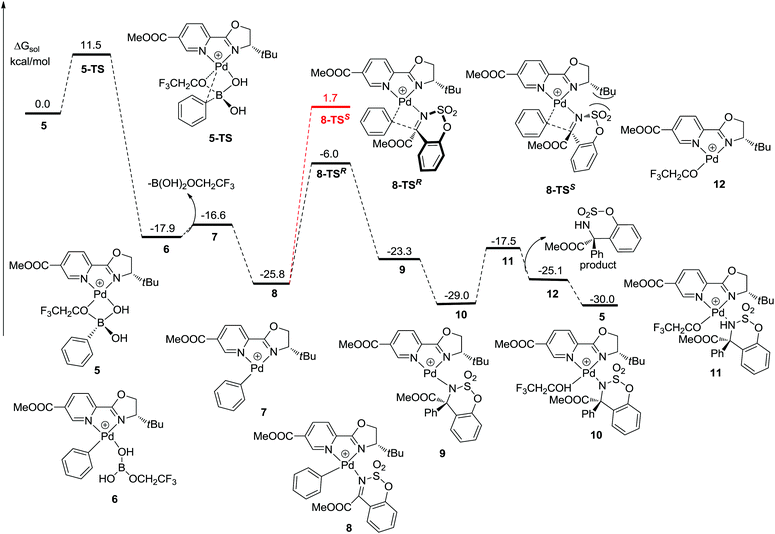 |
| Fig. 1 DFT-calculated catalytic cycle. | |
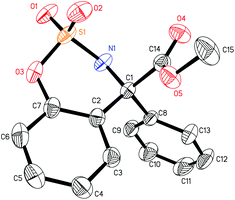 |
| Fig. 2 Single-crystal X-ray structure of 3aa and hydrogen atoms are omitted for clarity. | |
It is worth mentioning that phenylpalladium(II) complexes 7 could isomerize to 13 by the counterion CF3COO− with an energy barrier of 2.2 kcal mol−1 (Fig. 3).17 Similarly, 1a coordinates with 13 to form a stable intermediate 14. Then the phenyl group transfers to the substrate to form the product. The free energy barriers are 24.5 kcal mol−1 and 26.7 kcal mol−1 when the absolute configurations of the products are S (14-TSS) and R (14-TSR), respectively. Both of them are higher than the free energy barrier of 8-TSR but lower than 8-TSS. It means that the enantioselectivity was probably influenced by the transition state of 14-TSS.
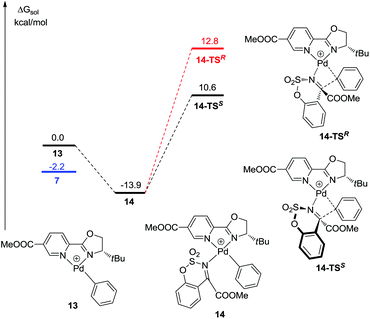 |
| Fig. 3 Alternative pathways of arylation. | |
3. Conclusions
To summarize, we have synthesized a series of α-tertiary amino esters via a palladium-catalyzed asymmetric addition of arylboronic acids to cyclic N-sulfonyl imine esters. All the substrates gave excellent yields (up to 99%) and enantioselectivities (up to 99% ee value) under mild reaction conditions. The DFT study revealed the mechanism of enantioselection. Computations indicated that the imine insertion step (8–8TSR) is the rate and stereoselectivity-determining step.
Acknowledgements
This work was partly supported by the National Nature Science Foundation of China (no. 21302124, 21172144), the China Postdoctoral Science Foundation (no. 2012M520882), Nippon Chemical Industrial Co. Ltd, Shanghai Jiao Tong University (SJTU), and Campus Asia Program of Tohoku University. We thank Prof. Tsuneo Imamoto and Dr Masashi Sugiya for help in discussions and the Instrumental Analysis Center of SJTU for determination of HR-MS.
Notes and references
-
(a) J. T. Mohr and B. M. Stoltz, Chem. – Asian J., 2007, 2, 1476 CrossRef CAS PubMed;
(b) M. Shibasaki and M. Kanai, Chem. Rev., 2008, 108, 2853 CrossRef CAS PubMed.
-
(a) K. Awang, T. Sevenet, M. Païs and A. H. A. Hadi, J. Nat. Prod., 1993, 56, 1134 CrossRef CAS;
(b) T. Kam, G. Subramaniam, K. Lim and Y. Choo, Tetrahedron Lett., 2004, 45, 5995 CrossRef CAS PubMed;
(c) W. Yap, C. Gan, Y. Low, Y. Choo, T. Etoh, M. Hayashi, K. Komiyama and T. Kam, J. Nat. Prod., 2011, 74, 1309 CrossRef CAS PubMed;
(d) R. A. Ramli, W. Lie and S. G. Pyne, J. Nat. Prod., 2014, 77, 894 CrossRef CAS PubMed;
(e) Y. Ying, W. Shan and Z. Zhan, J. Nat. Prod., 2014, 77, 2054 CrossRef CAS PubMed;
(f) K. Ko, S. Lee, S. Kim, E. Kim, K. Oh, J. Shin and D. Oh, J. Nat. Prod., 2014, 77, 2099 CrossRef CAS PubMed;
(g) B. Moreau, J. A. O'Meara, J. Bordeleau, M. Garneau, C. Godbout, V. Gorys, M. Leblanc, E. Villemure, P. W. White and M. Llinàs-Brunet, J. Med. Chem., 2014, 57, 1770 CrossRef CAS PubMed.
- For reviews on synthesis of chiral α-tertiary amines, see:
(a) O. Riant and J. Hannedouche, Org. Biomol. Chem., 2007, 5, 873 RSC;
(b) P. G. Cozzi, R. Hilgraf and N. Zimmermann, Eur. J. Org. Chem., 2007, 5969 CrossRef CAS For selected papers, see:
(c) S. Mouri, Z. Chen, H. Mitsunuma, M. Furutachi, S. Matsunaga and M. Shibasaki, J. Am. Chem. Soc., 2010, 132, 1255 CrossRef CAS PubMed;
(d) Z. Zhang, F. Xie, J. Jia and W. Zhang, J. Am. Chem. Soc., 2010, 132, 15939 CrossRef CAS PubMed;
(e) S. A. Moteki, S. Xu, S. Arimitsu and K. Maruoka, J. Am. Chem. Soc., 2010, 132, 17074 CrossRef CAS PubMed;
(f) B. M. Trost and L. C. Czabaniuk, J. Am. Chem. Soc., 2012, 134, 5778 CrossRef CAS PubMed;
(g) G. Yang, C. Shen and W. Zhang, Angew. Chem., Int. Ed., 2012, 51, 9141 CrossRef CAS PubMed;
(h) L. Yin, Y. Otsuka, H. Takada, S. Mouri, R. Yazaki, N. Kumagai and M. Shibasaki, Org. Lett., 2013, 15, 698 CrossRef CAS PubMed;
(i) M. Zhao, H. Bi, R. Jiang, X. Xu and M. Shi, Org. Lett., 2014, 16, 4566 CrossRef CAS PubMed;
(j) M. Holmquist, G. Blay and J. R. Pedro, Chem. Commun., 2014, 50, 9309 RSC.
-
(a) R. Wada, T. Shibuguchi, S. Makino, K. Oisaki, M. Kanai and M. Shibasaki, J. Am. Chem. Soc., 2006, 128, 7687 CrossRef CAS PubMed;
(b) C. Lauzon and A. Charette, Org. Lett., 2006, 8, 2743 CrossRef CAS PubMed;
(c) P. Fu, M. L. Snapper and A. H. Hoveyda, J. Am. Chem. Soc., 2008, 130, 5530 CrossRef CAS PubMed.
-
(a) D. N. Tran and N. Cramer, Angew. Chem., Int. Ed., 2011, 50, 11098 CrossRef CAS PubMed;
(b) H. H. Jung, A. W. Buesking and J. A. Ellman, Org. Lett., 2011, 13, 3912 CrossRef CAS PubMed.
-
(a) R. Shintani, M. Takeda, T. Tsuji and T. Hayashi, J. Am. Chem. Soc., 2010, 132, 13168 CrossRef CAS PubMed;
(b) R. Shintani, M. Takeda, Y. Soh, T. Ito and T. Hayashi, Org. Lett., 2011, 13, 2977 CrossRef CAS PubMed;
(c) T. Nishimura, A. Noishiki, G. C. Tsui and T. Hayashi, J. Am. Chem. Soc., 2012, 134, 5056 CrossRef CAS PubMed;
(d) T. Nishimura, Y. Ebe, H. Fujimoto and T. Hayashi, Chem. Commun., 2013, 49, 5504 RSC.
-
(a) Y. Luo, A. J. Carnell and H. W. Lam, Angew. Chem., Int. Ed., 2012, 51, 6762 CrossRef CAS PubMed;
(b) Y. Luo, H. B. Hepburn, N. Chotsaeng and H. W. Lam, Angew. Chem., Int. Ed., 2012, 51, 8309 CrossRef CAS PubMed.
-
(a) H. Wang, T. Jiang and M. Xu, J. Am. Chem. Soc., 2013, 135, 971 CrossRef CAS PubMed;
(b) H. Wang and M. Xu, Synthesis, 2013, 2125 CAS;
(c) H. Wang, Y. Li and M. Xu, Org. Lett., 2014, 16, 3962 CrossRef CAS PubMed.
-
(a) Y. Chen, Y. Chen, C. Feng and G. Lin, Org. Lett., 2014, 16, 3400 CrossRef CAS PubMed.
- For reviews on palladium-catalyzed conjugate addition reactions involving arylboron reagents, see:
(a) Y. Yamamoto, T. Nishikata and N. Miyaura, Pure Appl. Chem., 2008, 80, 807 CrossRef CAS;
(b) N. Miyaura, Synlett, 2009, 2039 CAS;
(c)
G. Berton and T. Hayashi, in Catalytic Asymmetric Conjugate Reactions, ed. A. Córdova, Wiley-VCH, Weinheim, 2010 Search PubMed;
(d) Y. Sun, P. Zhu, Q. Xu and M. Shi, RSC Adv., 2013, 3, 3153 RSC For selected examples, see:
(e) T. Nishikata, Y. Yamamoto and N. Miyaura, Angew. Chem., Int. Ed., 2003, 42, 2768 CrossRef CAS PubMed;
(f) F. Gini, B. Hessen and A. J. Minnaard, Org. Lett., 2005, 7, 5309 CrossRef CAS PubMed;
(g) X. Lu and S. Lin, J. Org. Chem., 2005, 70, 9651 CrossRef CAS PubMed;
(h) Z. Tao and M. Shi, Chem. – Eur. J., 2008, 14, 3759 CrossRef PubMed;
(i) S. Lin and X. Lu, Org. Lett., 2010, 12, 2536 CrossRef CAS PubMed;
(j) K. Kikushima, J. C. Holder, M. Gatti and B. M. Stoltz, J. Am. Chem. Soc., 2011, 133, 6902 CrossRef CAS PubMed;
(k) F. Wang, S. Li, M. Qu, M. Zhao, L. Liu and M. Shi, Chem. Commun., 2011, 47, 12813 RSC;
(l) J. C. Holder, A. N. Marziale, M. Gatti, B. Mao and B. M. Stoltz, Chem. – Eur. J., 2013, 19, 74 CrossRef CAS PubMed;
(m) I. Ibrahem, G. Ma, S. Afewerki and A. Córdova, Angew. Chem., Int. Ed., 2013, 52, 878 CrossRef CAS PubMed;
(n) F. Wang, F. Chen, M. Qu, T. Li, Y. Liu and M. Shi, Chem. Commun., 2013, 49, 3360 RSC.
-
(a) H. Dai, M. Yang and X. Lu, Adv. Synth. Catal., 2008, 350, 249 CrossRef CAS;
(b) H. Dai and X. Lu, Tetrahedron Lett., 2009, 50, 3478 CrossRef CAS PubMed;
(c) G. Ma, T. Zhang and M. Shi, Org. Lett., 2009, 11, 875 CrossRef CAS PubMed;
(d) C. S. Marques and A. J. Burke, Eur. J. Org. Chem., 2010, 1639 CrossRef CAS;
(e) Z. Liu and M. Shi, Tetrahedron, 2010, 66, 2619 CrossRef CAS PubMed;
(f) J. Chen, X. Lu, W. Lou, Y. Ye, H. Jiang and W. Zeng, J. Org. Chem., 2012, 77, 8541 CrossRef CAS PubMed.
- G. Yang and W. Zhang, Angew. Chem., Int. Ed., 2013, 52, 7540 CrossRef CAS PubMed.
- C. Jiang, Y. Lu and T. Hayashi, Angew. Chem., Int. Ed., 2014, 53, 9936 CrossRef CAS PubMed.
-
(a) K. Suwanborirux, K. Charupant, S. Amnuoypol, S. Pummangura, A. Kubo and N. Saito, J. Nat. Prod., 2002, 65, 935 CrossRef CAS PubMed;
(b) N. Ünver and G. İ. Kaya, Turk. J. Chem., 2005, 29, 547 Search PubMed;
(c) P. Saktrakulkla, S. Toriumi, M. Tsujimoto, C. Patarapanich, K. Suwanborirux and N. Saito, Bioorg. Med. Chem., 2011, 19, 4421 CrossRef CAS PubMed;
(d) M. Tsujimoto, W. Lowtangkitcharoen, N. Mori, W. Pangkruang, P. Putongking, K. Suwanborirux and N. Saito, Chem. Pharm. Bull., 2013, 61, 1052 CrossRef CAS PubMed.
-
(a) T. Nishikata, Y. Yamamoto, I. D. Gridnev and N. Miyaura, Organometallics, 2005, 24, 5025 CrossRef CAS;
(b) Y. Lan and K. N. Houk, J. Org. Chem., 2011, 76, 4905 CrossRef CAS PubMed;
(c) Q. Peng, H. Yan, X. Zhang and Y.-D. Wu, J. Org. Chem., 2012, 77, 7487 CrossRef CAS PubMed;
(d) J. C. Holder, L. Zou, A. N. Marziale, P. Liu, Y. Lan, M. Gatti, K. Kikushima, K. N. Houk and B. M. Stoltz, J. Am. Chem. Soc., 2013, 135, 14996 CrossRef CAS PubMed.
- CCDC 1047798 (3aa) contain the supplementary crystallographic data for this paper.
- Y. Dang, S. Qu, Z. Wang and X. Wang, J. Am. Chem. Soc., 2014, 136, 986 CrossRef CAS PubMed.
Footnotes |
† Dedicated to Professor Ei-ichi Negishi on the occasion of his 80th birthday. |
‡ Electronic supplementary information (ESI) available. CCDC 1047798. For ESI and crystallographic data in CIF or other electronic format see DOI: 10.1039/c4qo00347k |
§ These authors contributed equally to this work. |
|
This journal is © the Partner Organisations 2015 |
Click here to see how this site uses Cookies. View our privacy policy here.