DOI:
10.1039/C4QO00153B
(Research Article)
Org. Chem. Front., 2014,
1, 774-776
Copper-mediated difluoromethylation of electron-poor aryl iodides at room temperature†
Received
22nd May 2014
, Accepted 18th June 2014
First published on 20th June 2014
Abstract
A convenient copper-mediated direct difluoromethylation of electron-deficient aryl iodides, as well as heteroaryl and β-styryl iodides, using TMSCF2H has been developed. This one-step protocol proceeded at room temperature, affording various difluoromethylated products in moderate to excellent yields.
As fluorinated organic molecules are widely applied in many fields, such as pharmaceuticals, agrochemicals and materials, extensive efforts have been devoted to incorporation of fluorinated functional groups into various compounds.1 The difluoromethyl group (CF2H) is isosteric and isopolar to a hydroxy (OH)2 and thiol (SH)3 unit, and also acts as lipophilic hydrogen bond donors.4 Because of these unique properties, CF2H-containing compounds are important components of pesticides and pharmaceuticals.5 Up to now, different strategies have been developed for the synthesis of difluoromethylated compounds.1,6 However, methods for preparation of difluoromethylated arenes are still limited. A traditional method for the preparation of these compounds is fluorination of different substrates, such as aldehydes.7 Recently, transition-metal-mediated difluoroalkylation followed by further transformations has provided another efficient approach.8 In 2012, Baran reported a direct introduction of the difluoromethyl moiety into heteroarenes with a new agent (Zn(SO2CF2H)2, DFMS) via a radical process,9 but mixtures of regioisomers were observed in some cases. Compared to the above methods, transition-metal-mediated direct difluoromethylation has some advantages such as shorter reaction steps and broader substrate scope. However, this strategy was not developed until two years ago,10 probably because there were not so many stable and efficient difluoromethylation reagents.6 Only two reagents have been applied in transition-metal-mediated direct difluoromethylation of aryl halides: Me3SiCF2H10a reported by Hartwig and n-Bu3SnCF2H10b reported by Prakash. Me3SiCF2H is easily accessible and less toxic than n-Bu3SnCF2H, which makes Me3SiCF2H the first choice in the lab and industry. However, Hartwig's reaction system was only limited to electron-rich and electron-neutral iodoarenes 1 (Scheme 1a). Electron-poor substrates were transformed into the corresponding arenes 3, and the reaction of heteroaryl iodides was not reported. These drawbacks hindered the wide application of Hartwig's method. In continuation of our research on transition-metal-mediated/catalyzed difluoroalkylation reactions,11 we herein report an efficient copper-mediated difluoromethylation of electron-poor aryl iodides at room temperature (Scheme 1b). Difluoromethylated heteroarenes 4 can also be conveniently obtained in our reaction system. This work is an important complement to Hartwig's method.
 |
| Scheme 1 Copper-mediated direct difluoromethylation with TMSCF2H. | |
Although the copper-mediated/catalysed trifluoromethylation using TMSCF3 has been well established,1e,f the copper-mediated difluoromethylation with TMSCF2H is quite rare, probably because the Si–CF2H bond is more inert12 and difluoromethylcopper complexes are less stable.13 Recently, Hu reported that an appropriate Lewis base and solvent was crucial in activating the Si–CF2H bond,14 and Prakash revealed that DMF was helpful to stabilize the CuCF2H by computer calculation.10b The above two results encouraged us to explore the copper-mediated difluoromethylation of electron-deficient aryl iodides with TMSCF2H.
We initiated our investigation by reacting ethyl 4-iodobenzoate 1a with TMSCF2H (2.0 equiv.) in the presence of KF (2.0 equiv.) and CuI (1.0 equiv.) in DMF (1.0 mL) at room temperature under an Ar atmosphere. However, most of 1a was not converted, and the desired product 2a was not observed (Table 1, entry 1). Switching to other F-based initiators such as CsF and TBAT had no effects on the reaction (entries 2 and 3). 25% yield of the desired product 2a was obtained when the t-BuOK was used as the initiator (entry 4). Further screening of t-BuONa and t-BuOLi gave no better results (entries 5 and 6). To improve the yield of 2a, we evaluated a series of copper salts such as CuBr, CuCl, CuOAc and Cu(OAc)2 (entries 7–10). CuCl was the optimal base giving 2a in 35% yield (entry 7). Since the ligands play a key role in transition-mediated fluoroalkyl cross-coupling reactions, we next investigated the influence of the ligands. 1,10-Phenanthroline (phen) was found to be more effective than other ligands and dramatically increased the product yield to 70% (entries 11–14). A higher yield of 2a was obtained when the reaction was conducted under the conditions of TMSCF2H (2.4 equiv.), CuCl (1.2 equiv.), phen (1.2 equiv.) and t-BuOK (2.4 equiv.) (entry 15). Further increasing the amount of TMSCF2H, CuCl, phen and t-BuOK resulted in a slight lower yield (entry 16).
Table 1 Optimization of reaction conditionsa

|
Entry |
CuX |
Base |
Ligand |
Yieldb (%) |
Reaction conditions: 1a (0.2 mmol), TMSCF2H (2.0 equiv.), copper salt (1.0 equiv.), ligand (1.0 equiv.), base (2.0 equiv.), DMF (1.0 mL), rt.
Yield was determined by 19F NMR using benzotrifluoride as an internal standard.
TMSCF2H (2.4 equiv.), CuCl (1.2 equiv.), phen (1.2 equiv.), base (2.4 equiv.).
TMSCF2H (3.0 equiv.), CuCl (1.5 equiv.), phen (1.5 equiv.), base (3.0 equiv.).
|
1 |
CuI |
KF |
— |
NR |
2 |
CuI |
CsF |
— |
Trace |
3 |
CuI |
TBAT |
— |
NR |
4 |
CuI |
t-BuOK |
— |
25 |
5 |
CuI |
t-BuONa |
— |
8 |
6 |
CuI |
t-BuOLi |
— |
NR |
7 |
CuCl |
t-BuOK |
— |
35 |
8 |
CuBr |
t-BuOK |
— |
31 |
9 |
CuOAc |
t-BuOK |
— |
Trace |
10 |
Cu(OAc)2 |
t-BuOK |
— |
NR |
11 |
CuCl |
t-BuOK |
Phen |
70 |
12 |
CuCl |
t-BuOK |
Bipy |
45 |
13 |
CuCl |
t-BuOK |
TMEDA |
43 |
14 |
CuCl |
t-BuOK |
Et2NCH2CH2NEt2 |
30 |
15c |
CuCl |
t-BuOK |
Phen |
85 |
16d |
CuCl |
t-BuOK |
Phen |
84 |
With the optimal conditions in hand, we next examined the substrate scope of the Cu-mediated difluoromethylation of aryl and heteroaryl iodides (Table 2). In contrast to the reaction reported by Hartwig's group that is limited to electron-rich and electron-neutral iodoarenes described,10a electron-deficient aryl iodides reacted in good to excellent yields under the optimal conditions. A variety of electron-withdrawing functional groups such as cyano, ester, and nitro were well-tolerated in the reaction (2a–2f). Sterically hindered aryl iodides with a substituent in the ortho position also served as a suitable coupling partner and afforded good yields (2b, 2d). However, the substrates bearing electron-donating groups gave relatively lower yields. The iodo-substituted heteroaromatic compounds were also effective in this reaction, producing the desired products in good to excellent yields (4a–4c).
Table 2 Copper-mediated difluoromethylation of aryl and heteroaryl iodidesa,b
Reaction conditions: 1 (0.2 mmol), TMSCF2H (2.4 equiv.), CuCl (1.2 equiv.), phen (1.2 equiv.), t-BuOK (2.4 equiv.) under argon in DMF (1.0 mL) at room temperature.
Isolated yield.
|
|
This difluoromethylation protocol was also applied in the direct difluoromethylation of β-styryl iodides (Scheme 2). The corresponding allylic difluorinated alkenes 6a and 6b were obtained in moderate to good yields, with retention of configuration.
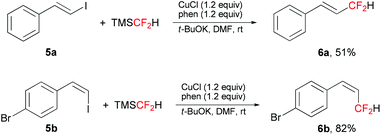 |
| Scheme 2 Copper-mediated difluoromethylation of β-styryl iodides. | |
The differences between Hartwig's and our reaction systems are shown in Table 3. First, an excess amount of TMSCF2H (5.0 equiv.) was needed in their system, probably for the generation of more stable intermediate Cu(CF2H)2−.10a In our system, only 2.4 equiv. of TMSCF2H was added, and the ligand phen was necessary to achieve high yields. Second, the weak base CsF was used in their system, while a strong base t-BuOK was needed in our system. Last but not least, the temperature was totally different (120 °C in their system vs. rt in our system). All these different reaction conditions, combined together, gave totally different results, as mentioned in Scheme 1.
Table 3 Comparing Hartwig's with our reaction systems
System |
TMSCF2H |
Ligand |
Base |
Temperature |
Hartwig's |
5.0 equiv. |
— |
CsF |
120 °C |
Our |
2.4 equiv. |
Phen |
t-BuOK |
rt |
Conclusions
In summary, we have developed a convenient method for one-step introduction of the difluoromethyl group into different substrates by employing copper-mediated direct difluoromethylation using TMSCF2H at room temperature. The mild reaction conditions make this method attractive for the synthesis of a series of difluoromethylated compounds. Ongoing studies will focus on the mechanism and extension of the scope of this transformation.
Acknowledgements
We thank the National Natural Science Foundation of China (21072028, 20832008) and the National Basic Research Program of China (2012CB21600) for funding this work.
Notes and references
- For recent reviews, see:
(a) K. Müller, C. Faeh and F. Diederich, Science, 2007, 317, 1881 CrossRef PubMed;
(b) J.-A. Ma and D. Cahard, Chem. Rev., 2008, 108, PR1 CrossRef CAS;
(c) S. Purser, P. R. Moore, S. Swallow and V. Gouverneur, Chem. Soc. Rev., 2008, 37, 320 RSC;
(d) K. L. Kirk, Org. Process Res. Dev., 2008, 12, 305 CrossRef CAS;
(e) O. A. Tomashenko and V. V. Grushin, Chem. Rev., 2011, 111, 4475 CrossRef CAS PubMed;
(f) T. Liang, C. N. Neumann and T. Ritter, Angew. Chem., Int. Ed., 2013, 52, 8214 CrossRef CAS PubMed.
- G. K. S. Prakash, M. Mandal, S. Schweizer, N. A. Petasis and G. A. Olah, J. Org. Chem., 2002, 67, 3718 CrossRef CAS PubMed.
- F. Narjes, K. F. Koehler, U. Koch, B. Gerlach, S. Colarusso, C. Steinkühler, M. Brunetti, S. Altamura, R. De Francesco and V. G. Matassa, Bioorg. Med. Chem. Lett., 2002, 12, 701 CrossRef CAS.
- J. A. Erickson and J. I. McLoughlin, J. Org. Chem., 1995, 60, 1626 CrossRef CAS.
-
(a) N. A. Meanwell, J. Med. Chem., 2011, 54, 2529 CrossRef CAS PubMed;
(b) J. Wang, M. Sánchez-Roselló, J. L. Aceña, C. del Pozo, A. E. Sorochinsky, S. Fustero, V. A. Soloshonok and H. Liu, Chem. Rev., 2014, 114, 2432 CrossRef CAS PubMed.
- J. Hu, W. Zhang and F. Wang, Chem. Commun., 2009, 7465 RSC.
- For selected examples, see:
(a) T. Umemoto, R. P. Singh, Y. Xu and N. Saito, J. Am. Chem. Soc., 2010, 132, 18199 CrossRef CAS PubMed;
(b) N. Turkman, L. An and M. Pomerantz, Org. Lett., 2010, 12, 4428 CrossRef CAS PubMed;
(c) T. Müller, L. Johann, B. Jannack, M. Brückner, D. A. Lanfranchi, H. Bauer, C. Sanchez, V. Yardley, C. Deregnaucourt, J. Schrével, M. Lanzer, R. H. Schirmer and E. Davioud-Charvet, J. Am. Chem. Soc., 2011, 133, 11557 CrossRef PubMed;
(d) Y. Hagooly and S. Rozen, Org. Lett., 2012, 14, 1114 CrossRef CAS PubMed;
(e) J.-B. Xia, C. Zhu and C. Chen, J. Am. Chem. Soc., 2013, 135, 17494 CrossRef CAS PubMed;
(f) S. Mizuta, I. S. R. Stenhagen, M. O'Duill, J. Wolstenhulme, A. K. Kirjavainen, S. J. Forsback, M. Tredwell, G. Sandford, P. R. Moore, M. Huiban, S. K. Luthra, J. Passchier, O. Solin and V. Gouverneur, Org. Lett., 2013, 15, 2648 CrossRef CAS PubMed;
(g) P. Xu, S. Guo, L. Wang and P. Tang, Angew. Chem., Int. Ed., 2014, 53, 5955 CrossRef CAS PubMed.
-
(a) K. Fujikawa, Y. Fujioka, A. Kobayashi and H. Amii, Org. Lett., 2011, 13, 5560 CrossRef CAS PubMed;
(b) K. Fujikawa, A. Kobayashi and H. Amii, Synthesis, 2012, 3015 CAS;
(c) S. Ge, W. Chaładaj and J. F. Hartwig, J. Am. Chem. Soc., 2014, 136, 4194 Search PubMed.
-
(a) Y. Fujiwara, J. A. Dixon, R. A. Rodriguez, R. D. Baxter, D. D. Dixon, M. R. Collins, D. G. Blackmond and P. S. Baran, J. Am. Chem. Soc., 2012, 134, 1494 CrossRef CAS PubMed;
(b) Y. Fujiwara, J. A. Dixon, F. O'Hara, E. D. Funder, D. D. Dixon, R. A. Rodriguez, R. D. Baxter, B. Herlé, N. Sach, M. R. Collins, Y. Ishihara and P. S. Baran, Nature, 2012, 492, 95 CrossRef CAS PubMed.
-
(a) P. S. Fier and J. F. Hartwig, J. Am. Chem. Soc., 2012, 134, 5524 CrossRef CAS PubMed;
(b) G. K. S. Prakash, S. K. Ganesh, J.-P. Jones, A. Kulkarni, K. Masood, J. K. Swabeck and G. A. Olah, Angew. Chem., Int. Ed., 2012, 51, 12090 CrossRef CAS PubMed.
-
(a) C. Guo, R. Wang and F.-L. Qing, J. Fluorine Chem., 2012, 143, 135 CrossRef CAS PubMed;
(b) X. Jiang, L. Chu and F.-L. Qing, Org. Lett., 2012, 14, 2870 CrossRef CAS PubMed;
(c) Q. Lin, L. Chu and F.-L. Qing, Chin. J. Chem., 2013, 31, 885 CrossRef CAS;
(d) X. Jiang, L. Chu and F.-L. Qing, New J. Chem., 2013, 37, 1736 RSC.
- T. Hagiwara and T. Fuchikami, Synlett, 1995, 717 CrossRef CAS PubMed.
-
(a) R. Eujen, B. Hoge and D. J. Brauer, J. Organomet. Chem., 1996, 519, 7 CrossRef CAS;
(b) D. J. Burton and G. A. Hartgraves, J. Fluorine Chem., 2007, 128, 1198 CrossRef CAS PubMed.
- Y. Zhao, W. Huang, J. Zheng and J. Hu, Org. Lett., 2011, 13, 5342 CrossRef CAS PubMed.
Footnote |
† Electronic supplementary information (ESI) available. See DOI: 10.1039/c4qo00153b |
|
This journal is © the Partner Organisations 2014 |
Click here to see how this site uses Cookies. View our privacy policy here.