DOI:
10.1039/C5QO00218D
(Research Article)
Org. Chem. Front., 2015,
2, 1374-1378
Palladium-catalyzed methylene C(sp3)–H arylation of the adamantyl scaffold†
Received
9th July 2015
, Accepted 13th August 2015
First published on 14th August 2015
Abstract
The adamantyl group is prevalent as a key pharmacophore element in drugs. We describe herein a palladium-catalyzed C–H functionalization logic for the methylene C(sp3)–H arylation of the adamantyl scaffold with the assistance of an amide group. The resulting arylated adamantyl amide was smoothly converted to an amine, providing facile access to the memantine analog.
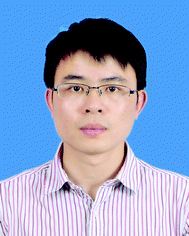 Honggen Wang | Honggen Wang was born in China in 1984, and obtained his BSc degree from Sun Yat-sen University in pharmaceutical sciences and computer science in 2006. He then moved to Guangzhou Institutes of Biomedicine and Health (GIBH), CAS, and received his PhD under the supervision of Prof. Qiang Zhu. He spent two years as a postdoctoral researcher with Prof. Frank Glorius at Westfälische Wilhelms-Universität Münster, Germany. In 2013, he started his independent research at Sun Yat-sen University. Honggen Wang was selected in the 11th batch of the “1000 Talents Program for Young Scholars” (2015). |
Introduction
Since the discovery of amantadine (1-adamantanamine) as a potent anti-influenza agent as early as 1964,1 the polycyclic adamantane motif has been recognized as the key structural element in numerous drugs, as in the anti-Alzheimer's disease agent memantine,2 anti-virus agents rimantadine3 and tromantadine,4 anti-diabetic drugs vildagliptin5 and saxagliptin,6 and the anti-acne agent adapalene7 (Fig. 1).8 It is well accepted that the incorporation of the adamantyl group increases the lipophilicity and metabolic stability of drugs, thereby improving their pharmacokinetic properties. In addition, as a bulky motif, the adamantyl group frequently occurs in ligands and catalysts in organic synthesis.9 Synthetically, the parent adamantane has become easily available since its seminal synthesis by Schleyer.10 Thereafter, the synthetic modifications of this cage hydrocarbon have yielded a number of adamantane derivatives. However, while the modifications at the adamantyl methine positions are fruitful due to the intrinsic weaker tertiary C–H bonds therein,11 the direct functionalization at the methylene positions poses a greater challenge and thus has been far less successful.12
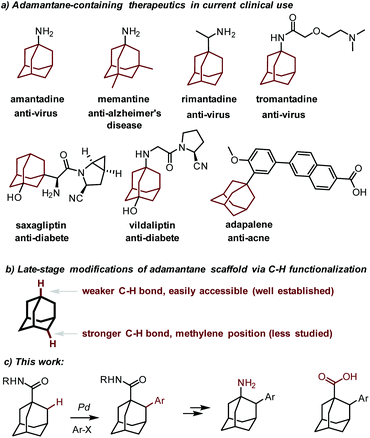 |
| Fig. 1 Adamantane-containing therapeutics and strategies for adamantane C–H functionalization. | |
The transition metal-catalyzed direct functionalization of inert C–H bonds has matured as a powerful tool for the facile assembly of a variety of C–C and C–heteroatom bonds in recent years.13 In this regard, palladium-catalyzed C(sp3)–H activation reactions are particularly useful and well suited for the functionalization of aliphatic hydrocarbons.14 For instance, it has been demonstrated that the catalytic C–H functionalization of aliphatic amines15 or carboxylic acid16 derivatives is feasible under the catalysis of palladium. Considering the lack of transformable functional groups at the methylene positions of adamantane and our preceding experience in the direct C–H functionalization of 3-pinanamine,17 we envisioned that a palladium-catalyzed C(sp3)–H activation strategy might be suitable for a late-stage modification18 of adamantane. 1-Adamantanamine and 1-adamantanecarboxylic acid are easily available and useful adamantane-containing compounds. We reasoned that the amino or the carboxylic acid functional group could serve as a directing group which would therefore facilitate the C–H activation event. Herein we report our realization of a Pd(0)-catalyzed direct arylation of the methylene C–H bond of adamantane directed by an amide group. It should be noted that the Pd(0)-catalyzed arylation reactions, especially intermolecular examples, have rarely been developed.19,20 By simple functional group manipulation, the resulting benzamides can be converted to the memantine analog (Fig. 1).
Results and discussion
At the outset of our studies, we investigated the C–H activation of 1-adamantanamine by installing a picoloyl functionality as the directing group. We anticipated that this synthetic logic would directly give the amantadine or memantine analogs after removal of the auxiliary. To our disappointment, no desired product 2 was observed after extensive screening of the reaction conditions. We suspected that this might be attributed to the difficulty of forming a disfavored four-membered-ring cyclometalated intermediate after the C–H activation event. Realizing this, we then turned our attention to the C–H activation of 1-adamantanecarboxylic acid derivative 3, bearing an 8-aminoquinoline bidentate directing group. To our delight, the reaction of 3 with 4-iodoanisole in the presence of Pd(OAc)2 as a catalyst and AgOAc as a base indeed gave the desired arylation product 4 in 3% yield. Unfortunately, attempts to further improve the yield failed in our hands. | 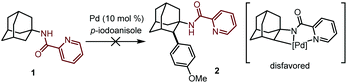 | (1) |
| 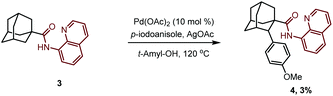 | (2) |
Encouraged by this promising result, we then investigated the reaction effected by a monodentate N-arylamide (CONHArF) auxiliary devised by Yu.19d Some representative results are summarized in Table 1. The arylation of 6a gave 7a in 14% isolated yield under the reaction conditions of Pd(OAc)2 (10 mol%), PCy3·HBF4 (10 mol%) and CsF (3.0 equiv.) in toluene at 110 °C for 20 h (Table 1, entry 1). Further optimization including the screening of different palladium sources, solvents, ligands, and bases was conducted, and it turned out that these parameters have trivial effects on the yield (entries 2–10). However, by increasing the loading of the ligand PPh3 to 50 mol% and the amount of 4-iodoanisole to 5.0 equivalents, the yield was doubled (35%, entry 11). It was found that the transformation is sensitive to the reaction concentration. When the reaction was performed at 0.5 M rather than 0.2 M, the yield was significantly improved to 53% (entry 12). As one might have predicted, a diarylation reaction (around 16% diarylation product) was also observed, which makes the separation difficult. Therefore, 6b bearing two methyl groups at the methine position was chosen as the model substrate to evaluate the substrate scope of this reaction. The methyl groups were anticipated to cause sufficient steric hindrance to the adjacent methylene position, thereby making mono-arylation exclusive. Indeed, when 6b was subjected to the standard reaction conditions, the mono-arylation product 7b was obtained in 54% yield, with no di-arylation product found (entry 13). The use of PivOH as an additive eliminated the reactivity (entry 14).
Table 1 Optimization of the reaction conditionsa
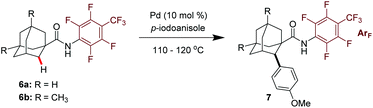
|
Entry |
R |
Ligand |
Solvent |
Additive |
Yield |
Conditions: entries 1–9: 3.0 equiv. of p-iodoanisole, 10 mol% ligand, 3.0 equiv. of base, 0.2 M; entries 11–14: 5.0 equiv. of p-iodoanisole, 50 mol% ligand.
The yield was determined by 1H NMR analysis of the crude product using dimethyl terephthalate as the internal standard.
0.5 M, isolated yield.
16% diarylation product was found.
|
1 |
H |
PCy3·HBF4 |
Toluene |
CsF |
14%b |
2 |
H |
PCy3·HBF4 |
o-Xylene |
CsF |
21%b |
3 |
H |
PCy3·HBF4 |
DCE |
CsF |
14%b |
4 |
H |
PCy3·HBF4 |
CH3CN |
CsF |
9%b |
5 |
H |
PPh3 |
o-Xylene |
CsF |
21%b |
6 |
H |
PPh3 |
o-Xylene |
Cs2CO3 |
19%b |
7 |
H |
PPh3 |
o-Xylene |
K2CO3 |
9%b |
8 |
H |
PtBu3·HBF4 |
o-Xylene |
CsF |
8%b |
9 |
H |
IPr·HCl |
o-Xylene |
CsF |
17%b |
10 |
H |
— |
o-Xylene |
CsF |
16%b |
11 |
H |
PPh3 |
o-Xylene |
CsF |
35%b |
12 |
H |
PPh3 |
n-Hexane |
CsF |
53%c,d |
13
|
CH
3
|
PPh
3
|
n-Hexane
|
CsF
|
54%
|
14 |
CH3 |
PPh3 |
n-Hexane |
PivOH |
0c |
With the optimized conditions in hand (entry 13, Table 1), we next explore the scope of this reaction. As shown in Table 2, this arylation protocol permits the direct C–H arylation of adamantyl amides 6 with a wide range of aryl iodides. Functional groups regardless of their electronic nature, such as alkoxy (7a, 7b, 7p), chloro (7c, 7d, 7o), bromo (7l), fluoro (7i, 7j, 7q), aryl (7e, 7h), alkyl (7f, 7g, 7q), ester (7m, 7n) and trifluoromethyl (7k) groups, were well tolerated, affording the corresponding monoarylation products in moderate to good yields. The structure of 7d was unambiguously determined by X-ray crystallographic analysis (Fig. 2).21
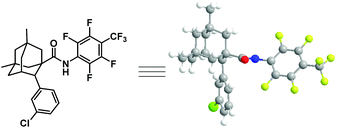 |
| Fig. 2 Absolute configuration of 7d. | |
Table 2 Substrate scope of methylene C–H functionalization
Reaction conditions: 0.1 mmol of substrate, 5.0 equiv. of Ar–X, 10 mol% Pd(TFA)2, 3.0 equiv. of CsF, 0.2 mL hexane, 120 °C, 24 h. All yields are based on the isolated products unless otherwise specified. at-AmylOH was used as a solvent. |
|
Not only aryl iodides but also aryl bromides were applicable to this transformation, albeit in lower yields. Disappointingly, the use of nitrogen or sulfur containing heterocyclic halides eliminated the reactivity completely, probably due to the deleterious coordination of the nitrogen or sulfur atoms to the palladium catalyst.
To evaluate the reaction efficacy on the preparative scale, a gram scale reaction was performed. The reaction of 6b with 1-chloro-3-iodobenzene gave 1.34 g of the arylation product 7d in 50% yield, demonstrating that the reaction is practical (Scheme 1a).
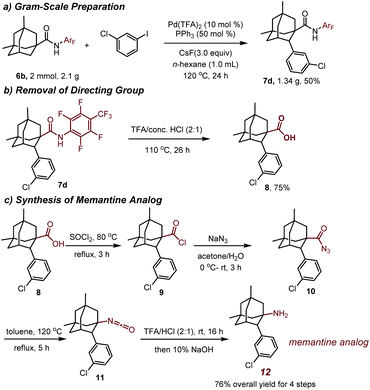 |
| Scheme 1 Gram-scale preparation, removal of the directing group and product derivatization. | |
The directing group N-arylamide (CONHArF) moiety could be conveniently removed upon the treatment with TFA/conc. HCl (2
:
1) at 110 °C, giving the corresponding carboxylic acid 8 in 75% yield (Scheme 1b). Thereafter, by a Curtius rearrangement, the carboxylic acid could be converted to an amino group, thus providing a memantine analog 12 in 76% overall yield (Scheme 1c).22 The synthesis of the arylated analogs of memantine using this protocol and their biological evaluation are underway in our laboratories and will be reported in due course.
Conclusions
In summary, we have realized a palladium-catalyzed direct arylation of methylene C(sp3)–H of the 1-adamantane carboxylic acid derivative. A variety of aromatic iodides and bromides containing different functional groups were well tolerated in this process, giving the arylated products in moderate to good yields. A gram scale reaction was conducted to showcase the efficacy of this reaction. The utility of this method was further demonstrated by the successful synthesis of a memantine analog. Given the importance of the adamantane scaffold in biologically active compounds and in organic synthesis, we anticipate that the methodology developed herein will find applications.
Acknowledgements
We are grateful for the support of this work by the “1000-Youth Talents Plan”, a start-up grant from Sun Yat-sen University and the National Natural Science Foundation of China (81402794 and 21472250).
Notes and references
-
(a) W. Davies, R. Grunert, R. Haff, J. McGahen, E. Neumayer, M. Paulshock, J. Watts, T. Wood, E. Hermann and C. Hoffmann, Science, 1964, 144, 862 CAS;
(b) T. H. Maugh 2nd, Science, 1979, 206, 1058 CAS.
- B. Reisberg, R. Doody, A. Stöffler, F. Schmitt, S. Ferris and H. J. Möbius, N. Engl. J. Med., 2003, 348, 1333 CrossRef CAS PubMed.
- E. A. Govorkova, H.-B. Fang, M. Tan and R. G. Webster, Antimicrob. Agents Chemother., 2004, 48, 4855 CrossRef CAS PubMed.
- K. Rosenthal, M. Sokol, R. Ingram, R. Subramanian and R. Fort, Antimicrob. Agents Chemother., 1982, 22, 1031 CrossRef CAS.
- B. Ahren, M. Landin-Olsson, P. A. Jansson, M. Svensson, D. Holmes and A. Schweizer, J. Clin. Endocrinol. Metab., 2004, 89, 2078 CrossRef CAS PubMed.
- D. J. Augeri, J. A. Robl, D. A. Betebenner, D. R. Magnin, A. Khanna, J. G. Robertson, A. Wang, L. M. Simpkins, P. Taunk and Q. Huang, J. Med. Chem., 2005, 48, 5025 CrossRef CAS PubMed.
- S. L. Rolewski, Dermatol. Nurs., 2003, 15, 447–450 Search PubMed.
- L. Wanka, K. Iqbal and P. R. Schreiner, Chem. Rev., 2013, 113, 3516 CrossRef CAS PubMed.
-
(a) W. Chaładaj, P. Kwiatkowski, J. Majer and J. Jurczak, Tetrahedron Lett., 2007, 48, 2405 CrossRef PubMed;
(b) B. Punji, T. J. Emge and A. S. Goldman, Organometallics, 2010, 29, 2702 CrossRef CAS;
(c) I. Ibrahem, M. Yu, R. R. Schrock and A. H. Hoveyda, J. Am. Chem. Soc., 2009, 131, 3844 CrossRef CAS PubMed;
(d) K. D. Hesp and M. Stradiotto, J. Am. Chem. Soc., 2010, 132, 18026 CrossRef CAS PubMed;
(e) A. Zapf, A. Ehrentraut and M. Beller, Angew. Chem., Int. Ed., 2000, 39, 4153 CrossRef CAS;
(f) K. D. Hesp, R. J. Lundgren and M. Stradiotto, J. Am. Chem. Soc., 2011, 133, 5194 CrossRef CAS PubMed;
(g) N. Hadei, E. A. B. Kantchev, C. J. O'Brie and M. G. Organ, Org. Lett., 2005, 7, 3805 CrossRef CAS PubMed.
- P. R. von Schleyer, J. Am. Chem. Soc., 1957, 79, 3292 CrossRef.
-
(a) V. Bagchi, P. Paraskevopoulou, P. Das, L. Chi, Q. Wang, A. Choudhury, J. S. Mathieson, L. Cronin, D. B. Pardue and T. R. Cundari, J. Am. Chem. Soc., 2014, 136, 11362 CrossRef CAS PubMed;
(b) M. Wang, C. Wang, X.-Q. Hao, X. Li, T. J. Vaughn, Y.-Y. Zhang, Y. Yu, Z.-Y. Li, M.-P. Song and H.-B. Yang, J. Am. Chem. Soc., 2014, 136, 10499 CrossRef CAS PubMed;
(c) V. A. Schmidt, R. K. Quinn, A. T. Brusoe and E. J. Alexanian, J. Am. Chem. Soc., 2014, 136, 14389 CrossRef CAS PubMed;
(d) H. Koch and W. Haaf, Angew. Chem., 1960, 72, 628 CrossRef CAS PubMed;
(e) R. Khusnutdinov, N. Shchadneva, A. Bayguzina, T. Oshnyakova, Y. Y. Mayakova and U. Dzhemilev, Russ. J. Org. Chem., 2013, 49, 1557 CrossRef CAS;
(f) G. A. Olah, J. G. Shih, B. P. Singh and B. Gupta, J. Org. Chem., 1983, 48, 3356 CrossRef CAS;
(g) S. Hara and M. Aoyama, Synthesis, 2008, 2510 CrossRef CAS;
(h) S. Bloom, C. R. Pitts, D. C. Miller, N. Haselton, M. G. Holl, E. Urheim and T. Lectka, Angew. Chem., Int. Ed., 2012, 51, 10580 CrossRef CAS PubMed;
(i) T. Higuchi, K. Shimada, N. Maruyama and M. Hirobe, J. Am. Chem. Soc., 1993, 115, 7551 CrossRef CAS;
(j) Z. Cohen, H. Varkony, E. Keinan and Y. Mazur, Org. Synth., 1979, 59, 176 CrossRef;
(k) C. J. Pierce and M. K. Hilinski, Org. Lett., 2014, 16, 6504 CrossRef CAS PubMed;
(l) O. Onomura, Y. Yamamoto, N. Moriyama, F. Iwasaki and Y. Matsumura, Synlett, 2006, 2415 CAS;
(m) B. H. Brodsky and J. Du Bois, J. Am. Chem. Soc., 2005, 127, 15391 CrossRef CAS PubMed;
(n) Y. Ishii, K. Matsunaka and S. Sakaguchi, J. Am. Chem. Soc., 2000, 122, 7390 CrossRef CAS.
-
(a) H. Geluk and V. Keizer, Org. Synth., 1973, 8 CAS;
(b) N. Basaric, M. Horvat, K. Mlinaric-Majerski, E. Zimmermann, J. r. Ceudörfl and A. G. Griesbeck, Org. Lett., 2008, 10, 3965 CrossRef CAS PubMed;
(c) I. Moiseev, V. Konovalova and S. Novikov, Zh. Org. Khim., 1978, 14, 1868 CAS.
-
(a) D. A. Colby, R. G. Bergman and J. A. Ellman, Chem. Rev., 2010, 110, 624 CrossRef CAS PubMed;
(b) T. W. Lyons and M. S. Sanford, Chem. Rev., 2010, 110, 1147 CrossRef CAS PubMed;
(c) L. Ackermann, Chem. Rev., 2011, 111, 1315 CrossRef CAS PubMed;
(d) C. S. Yeung and V. M. Dong, Chem. Rev., 2011, 111, 1215 CrossRef CAS PubMed;
(e) S. H. Cho, J. Y. Kim, J. Kwak and S. Chang, Chem. Soc. Rev., 2011, 40, 5068 RSC;
(f) J. Wencel-Delord, T. Dröge, F. Liu and F. Glorius, Chem. Soc. Rev., 2011, 40, 4740 RSC;
(g) N. Kuhl, M. N. Hopkinson, J. Wencel-Delord and F. Glorius, Angew. Chem., Int. Ed., 2012, 51, 10236 CrossRef CAS PubMed;
(h) K. M. Engle, T.-S. Mei, M. Wasa and J.-Q. Yu, Acc. Chem. Res., 2012, 45, 788 CrossRef CAS PubMed;
(i) C.-L. Sun, B.-J. Li and Z.-J. Shi, Chem. Rev., 2011, 111, 1293 CrossRef CAS PubMed;
(j) C. Zhu, R. Wang and J. R. Falck, Chem. – Asian J., 2012, 7, 1502 CrossRef CAS PubMed;
(k) P. B. Arockiam, C. Bruneau and P. H. Dixneuf, Chem. Rev., 2012, 112, 5879 CrossRef CAS PubMed;
(l) M. C. White, Science, 2012, 335, 807 CrossRef CAS PubMed.
-
(a) R. Jazzar, J. Hitce, A. Renaudat, J. Sofack-Kreutzer and O. Baudoin, Chem. – Eur. J., 2010, 16, 2654 CrossRef CAS PubMed;
(b) T. Newhouse and P. S. Baran, Angew. Chem., Int. Ed., 2011, 50, 3362 CrossRef CAS PubMed;
(c) J. L. Roizen, M. E. Harvey and J. Du Bois, Acc. Chem. Res., 2012, 45, 911 CrossRef CAS PubMed;
(d) H. Li, B.-J. Li and Z.-J. Shi, Catal. Sci. Technol., 2011, 1, 191 RSC.
- Selected examples:
(a) K. S. Chan, M. Wasa, L. Chu, B. N. Laforteza, M. Miura and J.-Q. Yu, Nat. Chem., 2014, 6, 146 CrossRef CAS PubMed;
(b) S.-Y. Zhang, G. He, Y. Zhao, K. Wright, W. A. Nack and G. Chen, J. Am. Chem. Soc., 2012, 134, 7313 CrossRef CAS PubMed;
(c) G. He and G. Chen, Angew. Chem., Int. Ed., 2011, 50, 5192 CrossRef CAS PubMed;
(d) A. McNally, B. Haffemayer, B. S. Collins and M. J. Gaunt, Nature, 2014, 510, 129 CrossRef CAS PubMed;
(e) K. S. L. Chan, H.-Y. Fu and J.-Q. Yu, J. Am. Chem. Soc., 2015, 137, 2042 CrossRef CAS PubMed;
(f) L.-S. Zhang, G. Chen, X. Wang, Q.-Y. Guo, X.-S. Zhang, F. Pan, K. Chen and Z.-J. Shi, Angew. Chem., Int. Ed., 2014, 53, 3899 CrossRef CAS PubMed.
- Selected examples:
(a) B. S. Reddy, L. R. Reddy and E. Corey, Org. Lett., 2006, 8, 3391 CrossRef CAS PubMed;
(b) V. G. Zaitsev, D. Shabashov and O. Daugulis, J. Am. Chem. Soc., 2005, 127, 13154 CrossRef CAS PubMed;
(c) R.-Y. Zhu, J. He, X.-C. Wang and J.-Q. Yu, J. Am. Chem. Soc., 2014, 136, 13194 CrossRef CAS PubMed;
(d) Y. Deng, W. Gong, J. He and J.-Q. Yu, Angew. Chem., Int. Ed., 2014, 53, 6692 CrossRef CAS PubMed;
(e) T. M. Figg, M. Wasa, J.-Q. Yu and D. G. Musaev, J. Am. Chem. Soc., 2013, 135, 14206 CrossRef CAS PubMed;
(f) J. He, S. Li, Y. Deng, H. Fu, B. N. Laforteza, J. E. Spangler, A. Homs and J.-Q. Yu, Science, 2014, 343, 1216 CrossRef CAS PubMed;
(g) K. Chen, S.-Q. Zhang, H.-Z. Jiang, J.-W. Xu and B.-F. Shi, Chem. – Eur. J., 2015, 21, 3264 CrossRef CAS PubMed;
(h) F. Pan, P.-X. Shen, L.-S. Zhang, X. Wang and Z.-J. Shi, Org. Lett., 2013, 15, 4758 CrossRef CAS PubMed;
(i) M. Al-Amin, M. Arisawa, S. Shuto, Y. Ano, M. Tobisu and N. Chatani, Adv. Synth. Catal., 2014, 356, 1631 CrossRef CAS PubMed;
(j) A. Renaudat, L. Jean-Gérard, R. Jazzar, C. E. Kefalidis, E. Clot and O. Baudoin, Angew. Chem., Int. Ed., 2010, 49, 7261 CrossRef CAS PubMed;
(k) S.-Y. Zhang, Q. Li, G. He, W. A. Nack and G. Chen, J. Am. Chem. Soc., 2013, 135, 12135 CrossRef CAS PubMed;
(l) L. D. Tran and O. Daugulis, Angew. Chem., Int. Ed., 2012, 51, 5188 CrossRef CAS PubMed;
(m) N. Hoshiya, T. Kobayashi, M. Arisawa and S. Shuto, Org. Lett., 2013, 15, 6202 CrossRef CAS PubMed.
- W. Cui, S. Chen, J.-Q. Wu, X. Zhao, W. Hu and H. Wang, Org. Lett., 2014, 16, 4288 CrossRef CAS PubMed.
-
(a) J. Wencel-Delord and F. Glorius, Nat. Chem., 2013, 5, 369 CrossRef CAS PubMed;
(b) L. McMurray, F. O'Hara and M.
J. Gaunt, Chem. Soc. Rev., 2011, 40, 1885 RSC;
(c) J. Yamaguchi, A. D. Yamaguchi and K. Itami, Angew. Chem., Int. Ed., 2012, 51, 8960 CrossRef CAS PubMed.
-
(a) G. Dyker, Angew. Chem., Int. Ed. Engl., 1994, 33, 103 CrossRef PubMed;
(b) D. Garcia-Cuadrado, A. A. C. Braga, F. Maseras and A. M. Echavarren, J. Am. Chem. Soc., 2006, 128, 1066 CrossRef CAS PubMed;
(c) M. Lafrance and K. Fagnou, J. Am. Chem. Soc., 2006, 128, 16496 CrossRef CAS PubMed;
(d) M. Wasa, K. M. Engle and J.-Q. Yu, J. Am. Chem. Soc., 2009, 131, 9886 CrossRef CAS PubMed.
- For related C(sp3)–H activation via the Pd(0)/Pd(II) mechanism, see:
(a) J. He, M. Wasa, K. S. L. Chan and J.-Q. Yu, J. Am. Chem. Soc., 2013, 135, 3387 CrossRef CAS PubMed;
(b) J. He, T. Shigenari and J.-Q. Yu, Angew. Chem., Int. Ed., 2015, 26, 6545 CrossRef PubMed.
- CCDC number: 1047296.
- T. Sasaki, S. Eguchi and T. Okano, Synthesis, 1980, 472 CrossRef CAS.
Footnote |
† Electronic supplementary information (ESI) available. CCDC 1047296. For ESI and crystallographic data in CIF or other electronic format see DOI: 10.1039/c5qo00218d |
|
This journal is © the Partner Organisations 2015 |