DOI:
10.1039/D3RA08186A
(Paper)
RSC Adv., 2024,
14, 6470-6475
Ligand-free Pd-catalyzed highly selective arylation of activated and unactivated alkenes via oxidative and reductive heck coupling†
Received
30th November 2023
, Accepted 26th January 2024
First published on 21st February 2024
Abstract
In this work, an eco-friendly, green, efficient approach for oxidative and reductive Heck–Mizoroki (HM) reactions was developed, which offered acceptable yields from first-pass experiments. Mono-arylation was achieved without the use of ligands, directing groups, or prefunctionalized alkenes. Considering mild reaction conditions, good functional group compatibility, and great regioselectivity, the method can find broad applications in novel medicine and material development and discovery processes.
Introduction
Approaches employing Heck coupling have emerged as some of the most powerful tools for modern organic syntheses owing to their high efficiency and widespread applications in the synthesis of pharmaceutical drugs and functional materials.1 Although ground-breaking advances in metal-catalysed reactions have been reported in the past two decades, the pursuit of scalable, operationally simple protocols for the Heck–Mizoroki (HM) reaction has always been challenging. It is particularly true that drawbacks such as harsh reaction conditions and low yields greatly hamper its large-scale practical application, especially in the pharmaceutical industry.2 Attempts to overcome these drawbacks include attempted syntheses of efficient catalysts;3 the utilization of solvent-free reaction conditions; and the application of non-classical energy sources such as microwave irradiation, high pressure, and mechanochemical techniques.4 Despite significant advances in acyclic aliphatic alkenes and cycloenones (Scheme 1A),5a–d the synthesis of cyclic olefins involving oxidative or reductive Heck coupling continues to be particularly challenging. Additionally, chemo-selectivity is another commonly faced problem within directing-group-assisted C–H olefination.5 Hence, it is of great synthetic value to develop a practical methodology to address these long-standing issues.
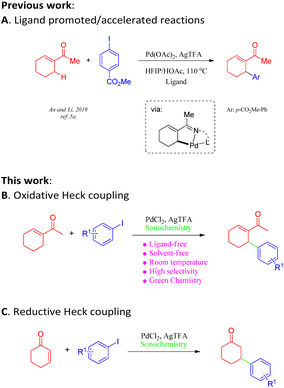 |
| Scheme 1 (A) Ligand promoted/accelerated coupling reactions with α,β-unsaturated ketone. (B) Ligand-free Pd-catalyzed oxidative heck coupling reactions. (C) Ligand-free Pd-catalyzed reductive heck coupling reactions. | |
In recent decades, the application of green methodologies that are environmentally friendly and can minimize chemical disposal and energy consumption have become the goals of organic chemists. One of the green chemistry techniques is ultrasound irradiation. Ultrasound technology has recently been applied to accelerate a large number of organic reactions under aqueous and non-aqueous conditions.6 In particular, such techniques often provide an ameliorative, sustainable system with solvent-free conditions7 for the improvement of traditional transformations8 and, most importantly, alter chemical reactivity as well as selectivity.9 The recent demand for eco-friendly chemical processes has led to the development of several clean and efficient protocols.10 Furthermore, a reliable and robust procedure for Heck reactions would be of considerable value. Herein, we report an ultrasound-assisted methodology for oxidative or reductive Heck coupling under solvent-free conditions at room temperature in open air, which can be applied to a broad range of coupling partners (Scheme 1B and C).
Results and discussion
To develop a general protocol for the arylation of olefins under mild conditions, a model coupling reaction between 1-cyclohexenyl methyl ketone (1a) and methyl 4-iodobenzoate (2a) in the presence of catalytic Pd(OAc)2 and stoichiometric amounts of AgTFA was conducted to screen optimal reaction conditions; the results are shown in Table 1. Only a trace amount of the expected product (3a) was formed when the model coupling reaction was treated with mechanical stirring at room temperature (Table 1, entry 1). However, the application of ultrasound led to a moderate improvement in the yield to 76% (Table 1, entry 2). In this case, when the substrates increased the energy of collision due to a change in the oscillations of the electrical component of the microwave field, it induced the breakage and re-establishment of intermolecular bonds. Next, the screening of silver salts was conducted, and a low yield was observed with Ag3PO4, AgOAc, Ag2O, and Ag2CO3 (entries 3–6). Further investigations indicated that the desired product was formed in the highest yield with 1.5 equivalents of AgTFA. The yields decreased with a higher or lower concentration of AgTFA (compare entries 7–10 of Table 1). The examination of different palladium catalysts revealed that all the examined reactions led to some conversion, albeit with quite different efficiencies (Table 1, entries 11–15), with PdCl2 being the most reactive. When the amount of catalyst was reduced to 0.5 mol%, the yields decreased obviously (Table 1, entry 16–18). In stark contrast, the coupling reaction proceeded less efficiently in solvents such as HFIP (hexafluoroisopropyl alcohol), DMSO, DMF, EtOH, THF, DCM, and Tol (toluene). According to the above results, the optimization study revealed that the production of 3a can be successfully accomplished in high yield (86%) by ultrasonic irradiation of a mixture of 1a and 2a in the absence of the solvent at room temperature in the open air.
Table 1 Optimization of the reaction conditionsa
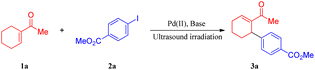
|
Entry |
Pd source (mol%) |
Base (equiv.) |
Yieldb (%) |
Reactions were performed taking reactant 1a (0.45 mmol) and 2a (0.15 mmol) under ultrasound irradiation at room temperature in the open air. Sonication was performed using an ultrasound cleaning bath with a frequency of 40 kHz and a voltage of 220 V. Isolated yield. Mechanical stirring instead of ultrasound irradiation. Solvent (1 mL): HFIP, DMSO, DMF, EtOH, THF, DCM, and Tol. |
1c |
Pd(Oac)2 (10) |
AgTFA (1.0) |
70 |
2 |
Pd(Oac)2 (10) |
AgTFA (1.0) |
76 |
3 |
Pd(Oac)2 (10) |
Ag3PO4 (1.0) |
<10 |
4 |
Pd(Oac)2 (10) |
AgOAc (1.0) |
<10 |
5 |
Pd(Oac)2 (10) |
Ag2O (1.0) |
Trace |
6 |
Pd(Oac)2 (10) |
Ag2CO3 (1.0) |
<10 |
7 |
Pd(Oac)2 (10) |
AgTFA (0.2) |
22 |
8 |
Pd(Oac)2 (10) |
AgTFA (0.6) |
61 |
9 |
Pd(Oac)2 (10) |
AgTFA (1.5) |
84 |
10 |
Pd(Oac)2 (10) |
AgTFA (2.0) |
67 |
11 |
Pd(TFA)2 (10) |
AgTFA (1.5) |
68.6 |
12 |
Pd(CH3CN)2Cl2 (10) |
AgTFA (1.5) |
85 |
13 |
Pd(PPh3)2Cl2 (10) |
AgTFA (1.5) |
<10 |
14 |
Pd(dppf)Cl2 (10) |
AgTFA (1.5) |
<10 |
15 |
PdCl2 (10) |
AgTFA (1.5) |
86 |
16 |
PdCl2 (0.5) |
AgTFA (1.5) |
Trace |
17 |
PdCl2 (1.0) |
AgTFA (1.5) |
13 |
18 |
PdCl2 (5.0) |
AgTFA (1.5) |
19 |
19d |
PdCl2 (10) |
AgTFA (1.5) |
<30 |
After determining the optimal conditions, we next investigated the substrate scope of olefins and aryl iodides (Table 2 and Scheme 2). Firstly, the substrate scope of the activated and unactivated olefins was examined for Heck coupling, using methyl 4-iodobenzoate (2a) as the model substrate. It was interesting to find that the Heck coupling of 2a with a variety of substituted cyclic olefins was achieved to afford the allylic products (3a–3e) in excellent to moderate yields, while cyclohexene and 3-methylenedihydrofuran-2(3H)-one provided the mixture of double-bond isomers (3f/3g, 3h/3i) with good yields (75% and 73%, respectively). Unexpectedly, α,β-unsaturated ketones, such as cyclohex-2-enone, cyclopent-2-enone, and cyclohept-2-enone, led to the reductive Heck products 3j, 3k, and 3l with moderate yields. Gratifyingly, a wide range of terminal olefins underwent the coupling smoothly, offering the corresponding products in satisfactory yields (up to 96%). In all the cases, only the E-isomers (3m–3s) were selectively obtained, and the mono-arylations were also regioselective.
Table 2 Scope of olefins
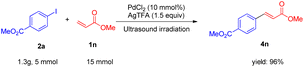 |
| Scheme 2 The scale-up Heck coupling reaction. | |
We also surveyed the scope of aryl iodides comprising various types of functional groups. Under mild reaction conditions and using simple operations, catalytic amounts of Pd(Oac)2 and stoichiometric amounts of AgTFA effected the transformations of aryl iodides into the corresponding monoarylated product (4a–4ah) in good to excellent yields. The coupling of aryl iodides bearing ortho-, meta- or para-substituent (4a–4u) with methyl acrylate furnished satisfactory yields of the monoarylated products. It is notable that the steric hindrance properties of aryl iodides did not show significant influences on the reactions (4d and 4e), and a phenolic hydroxy group (4f) and protic functional groups (4g) are well tolerated in this process. Not surprisingly, the catalytic system distinguished between iodide and other potential reactive halides (4t and 4u) (Table 3).
Table 3 Scope of aryl iodides
Moreover, this new protocol succeeded with the multi-substituted substrate (4v–4z). Furthermore, heteroaryl iodides and iodine-substituted fused-ring compounds in this process could also give very beneficial yields (4ab–4ah).
In particular, the arylations of olefins could be well scalable under the optimized conditions, which could be up to 1.3 g (Scheme 2). The proposed method offers several remarkable advantages, including shorter reaction time, milder reaction conditions, and reduction of undesired side reactions causing higher yields in comparison to the conventional methods.11 These features render our protocol particularly useful for coupling reactions of complex small molecules, which offers an excellent option for establishing a new horizon for Heck-type reactions of olefins.
On the basis of the experimental results (3a, 3j) and literature reports,12 two tentative mechanisms for the arylation of arenes are proposed, as shown in Scheme 3. We believe that an initial silver-mediated iodide abstraction from the aryl palladium iodide (A) results in the formation of the transition state ArPdTFA (B). It is presumed that the transition state is followed by an equilibrium involving ArPdTFA, ArPd+, and CF3COO−. ArPdTFA is assumed to be the reactive species instead of ArPd+. Insertion of an olefin into the C–Pd bond of ArPdTFA would result in the formation of new alkyl palladium species (C, D). There are two pathways: path a and path b.
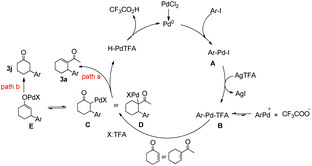 |
| Scheme 3 Plausible mechanism. | |
In path a, β-hydride elimination of intermediate D gave product 3a, the Pd(0) species was obtained in the reductive elimination of HPdTFA from the β-hydride elimination process.
In path b, enolization of intermediate C generated the palladium enolate E, finally, protonolysis of E furnished the desired β-arylated ketone with regeneration of the Pd catalyst.
Conclusions
In summary, we developed a solvent-free, mild, and efficient protocol for the Heck–Mizoroki reactions under ultrasonic irradiation in open air, which would be predictable and robust using a range of substrates. Varieties of aryl iodides were tolerated in the reaction with a wide range of olefins and provided the oxidative or reductive Heck products in satisfactory yields even at the gram scale. More importantly, the approach should find broad applications in an industrial environment and the synthesis of ubiquitous structural units in pharmaceuticals.
Conflicts of interest
There are no conflicts to declare.
Acknowledgements
This research was financially supported by the Yunnan Fundamental Research Projects (No. 202001AU070096), PhD research startup foundation of Yunnan University of Chinese Medicine (No. 11370102815), Yunnan Provincial Science, and Technology Department-Applied Basic Research Joint Special Funds of Chinese Medicine (No. 202301AZ070001-021).
Notes and references
- For selected reviews and examples, see:
(a) J. L. Bras and J. Muzart, Intermolecular dehydrogenative Heck reactions, Chem. Rev., 2011, 111, 1170–1214 CrossRef PubMed;
(b) J. Yamaguchi, A. D. Yamaguchi and K. Itami, C-H bond functionalization: emerging synthetic tools for natural products and pharmaceuticals, Angew. Chem., Int. Ed., 2012, 51, 8960–9009 CrossRef CAS PubMed;
(c) H. P. L. Gemoets, V. Hessel and T. Noël, Aerobic C-H olefination of indoles via a cross-dehydrogenative coupling in continuous flow, Org. Lett., 2014, 16, 5800–5803 CrossRef CAS PubMed;
(d) C. J. C. Lamb, B. G. Nderitu, G. McMurdo, J. M. Tobin, F. Vilela and A. L. Lee, Auto-Tandem Catalysis: PdII -Catalysed Dehydrogenation/Oxidative Heck Reaction of Cyclopentane-1,3-diones, Chem.–Eur. J., 2017, 23, 18282–18288 CrossRef CAS PubMed;
(e) W. Li, Y. Zhao, S. Mai and Q. Song, Thiocarbamate-Directed Tandem Olefination-Intramolecular Sulfuration of Two Ortho C-H Bonds: Application to Synthesis of a COX-2 Inhibitor, Org. Lett., 2018, 20, 1162–1166 CrossRef CAS PubMed;
(f) A. Crusco, H. Whiteland, R. Baptista, J. E. F. Thomas, M. Beckmann, L. A. J. Mur, R. J. Nash, A. D. Westwell and K. F. Hoffmann, Antischistosomal Properties of Sclareol and Its Heck-Coupled Derivatives: Design, Synthesis, Biological Evaluation, and Untargeted Metabolomics, ACS Infect. Dis., 2019, 5, 1188–1199 CrossRef CAS PubMed;
(g) A. Kumar, A. Gour, S. Dhiman, N. Hudait, P. Kumar, D. Vashishth, S. Kaur, S. T. Abdullah, U. Nandi and D. Mukherjee, Stereoselective and regioselective Heck arylation at C-17 exocyclic double bond of andrographolide to generate labdane-based lea, J. Mol. Struct., 2023, 1286, 135568 CrossRef CAS;
(h) T. J. M. Byrne, M. E. Mylrea and J. D. Cuthbertson, A Redox-Relay Heck Approach to Substituted Tetrahydrofurans, Org. Lett., 2023, 25, 2361–2365 CrossRef CAS PubMed;
(i) S. Yu, L. Zhou, S. Ye and X. Tong, Domino Sequences Involving Stereoselective Hydrazone-Type Heck Reaction and Denitrogenative [1,5]-Sigmatropic Rearrangement, J. Am. Chem. Soc., 2023, 145, 7621–7627 CrossRef CAS PubMed;
(j) P. J. Anju, M. Neetha and G. Anilkumar, Recent Advances on N-Heterocyclic Carbene-Palladium-catalyzed Heck Reaction, ChemistrySelect, 2022, 7, e202103564 CrossRef CAS;
(k) A. N. Reznikov, M. A. Ashatkina and Y. N. Klimochkin, Recent developments in asymmetric Heck type cyclization reactions for constructions of complex molecules, Org. Biomol. Chem., 2021, 19, 5673–5701 RSC;
(l) D. Paul, S. Das, S. Saha, H. Sharma and R. K. Goswami, Intramolecular Heck Reaction in Total Synthesis of Natural Products: An Update, Eur. J. Org Chem., 2021, 2021, 2057–2076 CrossRef CAS;
(m) S. Gao, L. Shi, L. Chang, B. Wang and J. Fu, Recent developments in Heck-type reaction of unactivated alkenes and alkyl electrophiles, Synthesis, 2021, 53, 861–878 CrossRef CAS;
(n) J. Xie, R. Liang and Y. Jia, Recent Advances of Catalytic Enantioselective Heck Reactions and Reductive-Heck Reactions, Chin. J. Chem., 2024, 39, 710–728 Search PubMed;
(o) U. S. Kanchana, E. J. Diana, T. V. Mathew and G. Anilkumar, Recent developments in the metal catalysed cross-coupling reactions for the synthesis of the enone system of chalcones, Appl. Organomet. Chem., 2020, 34, e5987 CrossRef;
(p) B. Ju and W. Kong, Recent progress in the consecutive double Heck reaction, Asian J. Org. Chem., 2020, 9, 1154–1161 CrossRef CAS;
(q) L. J. Oxtoby, J. A. Gurak, S. R. Wisniewski, M. D. Eastgate and K. M. Engle, Palladium-Catalyzed Reductive Heck Coupling of Alkenes, Trends Chem., 2019, 1, 572–587 CrossRef CAS PubMed.
- J. Magano and J. R. Dunetz, Large-scale applications of transition metal-catalyzed couplings for the synthesis of pharmaceuticals, Chem. Rev., 2011, 111, 2177–2250 CrossRef CAS PubMed.
- For selected reviews and examples, see:
(a) L. Mohammadi and M. R. Vaezi, Palladium Nanoparticle-Decorated Porous Metal-Organic-Framework (Zr)@Guanidine: Novel Efficient Catalyst in Cross-Coupling (Suzuki, Heck, and Sonogashira) Reactions and Carbonylative Sonogashira under Mild Conditions, ACS Omega, 2023, 8, 16395–16410 CrossRef CAS PubMed;
(b) V. W. Bhoyare, E. Daiann Sosa Carrizo, C. C. Chintawar, V. Gandon and N. T. Patil, Gold-Catalyzed Heck Reaction, J. Am. Chem. Soc., 2023, 145, 8810–8816 CrossRef CAS PubMed;
(c) M. Çalışkan, H. D. Güzel and T. Baran, Pd nanoparticles decorated on Schiff base-modified chitosan/CeO2 as a heterogeneous and retriable nanocatalyst for Heck reactions and remediation of environmental pollutants, Int. J. Biol. Macromol., 2023, 240, 124453 CrossRef PubMed;
(d) T. Nishikata, Y. Noda, R. Fujimoto and T. Sakashita, An efficient generation of a functionalized tertiary-alkyl radical for copper-catalyzed tertiary-alkylative Mizoroki-Heck type reaction, J. Am. Chem. Soc., 2013, 135, 16372–16375 CrossRef CAS PubMed;
(e) A. M. Ghadiri, M. Farhang, P. Hassani, A. Salek, A. T. Ramezani and A. R. Akbarzadeh, Recent advancements review Suzuki and Heck reactions catalyzed by metalloporphyrins, Inorg. Chem. Commun., 2023, 149, 110359 CrossRef CAS;
(f) D. E. Jose, U. S. Kanchana and T. V. Mathew, Recent developments of supported Palladium nanocatalyst and magnetically separable supported Palladium nanocatalysts for Heck cross-coupling reactions, J. Nanopart. Res., 2022, 24, 89 CrossRef CAS;
(g) M. Dohendou, K. Pakzad, Z. Nezafat, M. Nasrollahzadeh and M. G. Dekamin, Progresses in chitin, chitosan, starch, cellulose, pectin, alginate, gelatin and gum based (nano)catalysts for the Heck coupling reactions: A review, Int. J. Biol. Macromol., 2021, 192, 771–819 CrossRef CAS PubMed;
(h) J. Soni, A. Sethiya, N. Sahiba and S. Agarwal, Recent advancements in organic synthesis catalyzed by graphene oxide metal composites as heterogeneous nanocatalysts, Appl. Organomet. Chem., 2021, 35, e6162 CrossRef CAS;
(i) S. Bhakta and T. Ghosh, Emerging nickel catalysis in Heck reactions: Recent developments, Adv. Synth. Catal., 2020, 362, 5257–5274 CrossRef CAS;
(j) J. Josep Soldevila-Barreda and N. Metzler-Nolte, Intracellular Catalysis with Selected Metal Complexes and Metallic Nanoparticles: Advances toward the Development of Catalytic Metallodrugs, Chem. Rev., 2019, 119, 829–869 CrossRef PubMed.
- W. Susanto, C. Y. Chu, W. J. Ang, T. C. Chou, L. C. Lo and Y. Lam, Fluorous oxime palladacycle: a precatalyst for carbon-carbon coupling reactions in aqueous and organic medium, J. Org. Chem., 2012, 77, 2729–2742 CrossRef CAS PubMed; M. A. Andrade and L. Martins, New Trends in C–C Cross-Coupling Reactions: The Use of Unconventional Conditions, Molecules, 2020, 25, 5506–5538 CrossRef PubMed.
-
(a) Y. Cheng, J. Zheng, C. Tian, Y. He, C. Zhang, Q. Tan, G. An and G. Li, Palladium-Catalyzed C-H Arylation of Aliphatic and Aromatic Ketones using Dipeptide Transient Directing Groups, Asian J. Org. Chem., 2019, 8, 526–531 CrossRef CAS;
(b) M. Bera, S. K. Sahoo and D. Maiti, Room-Temperature meta-Functionalization: Pd(II)-Catalyzed Synthesis of 1,3,5-Trialkenyl Arene and meta-Hydroxylated Olefin, ACS Catal., 2016, 6, 3575–3579 CrossRef CAS;
(c) S. Bag, T. Patra, A. Modak, A. Deb, S. Maity, U. Dutta, A. Dey, R. Kancherla, A. Maji, A. Hazra, M. Bera and D. Maiti, Remote para-C-H Functionalization of Arenes by a D-Shaped Biphenyl Template-Based Assembly, J. Am. Chem. Soc., 2015, 137, 11888–11891 CrossRef CAS PubMed;
(d) T. Patra, S. Bag, R. Kancherla, A. Mondal, A. Dey, S. Pimparkar, S. Agasti, A. Modak and D. Maiti, Palladium-Catalyzed Directed para C-H Functionalization of Phenols, Angew. Chem., Int. Ed., 2016, 55, 7751–7755 CrossRef CAS PubMed;
(e) N. Gigant, F. Quintin and J.-E. Bäckvall, Preparation of tetrasubstituted olefins using mono or double aerobic direct C-H functionalization strategies: importance of steric effects, J. Org. Chem., 2015, 80, 2796–2803 CrossRef CAS PubMed;
(f) P. Annamalai, K.-C. Hsu, S. Raju, H.-C. Hsiao, C.-W. Chou, G.-Y. Lin, C.-M. Hsieh, P.-L. Chen, Y.-H. Liu and S.-C. Chuang, Palladium(II)-Catalyzed Mono- and Bis-alkenylation of N-Acetyl-2-aminobiaryls through Regioselective C-H Bond Activation, J. Org. Chem., 2018, 83, 3840–3856 CrossRef CAS PubMed.
- For selected reviews and examples, see:
(a) M. A. P. Martins, C. M. P. Pereira, W. Moura, S. Cunico, F. A. Rosa, R. L. Peres, P. Machado, N. Zanatta and H. G. Bonacorso, Ultrasound promoted synthesis of 5-hydroxy-5-trihalomethyl-4,5-dihydroisoxazoles and beta-enamino trihalomethyl ketones in water, Ultrason. Sonochem., 2006, 13, 364–370 CrossRef CAS PubMed;
(b) M. Rouhani, A. Ramazani and S. W. Joo, Novel, fast and efficient one-pot sonochemical synthesis of 2-aryl-1,3,4-oxadiazoles, Ultrason. Sonochem., 2014, 21, 262–267 CrossRef CAS PubMed;
(c) B. Banerjee, Recent developments on ultrasound assisted catalyst-free organic synthesis, Ultrason. Sonochem., 2017, 35, 1–14 CrossRef CAS PubMed;
(d) B. Goutam, N. Nayana, M. Mullicka, B. Anindita and K. Indrajit, Ultrasound-promoted Organic Synthesis-A Recent Update, Curr. Org. Chem., 2021, 25, 1539–1565 CrossRef;
(e) L. Rubab, A. Anum, S. A. Al-Hussain, A. Irfan, S. Ahmad, S. Ullah, A. A. Al-Mutairi and M. E. A. Zaki, Green Chemistry in Organic Synthesis: Recent Update on Green Catalytic Approaches in Synthesis of 1,2,4-Thiadiazole, Catalysts, 2022, 12, 1329–1353 CrossRef CAS;
(f) B. Keval, P. Dhara, R. Mrudangsinh, P. Ashish and S. Drashti, An Update on the Recent Green Synthetic Approaches for Imidazo[1, 2-a] Pyridine: A Privileged Scaffold, Curr. Org. Chem., 2022, 26, 2016–2054 Search PubMed;
(g) S. Bhavani, M. Ashraf Ashfaq, D. Rambabu, M. V. Basaveswara Rao and M. Pal, Ultrasound assisted Mizoroki–Heck reaction catalyzed by Pd/C: Synthesis of 3-vinyl indoles as potential cytotoxic agents, Arabian J. Chem., 2019, 12, 3836–3846 CrossRef CAS;
(h) K. Saïd, Y. Moussaoui, M. Kammoun and R. Ben Salem, Ultrasonic activation of Heck type reactions in the presence of Aliquat-336, Ultrason. Sonochem., 2011, 18, 23–27 CrossRef PubMed;
(i) Z. Zhang, Z. Zha, C. Gan, C. Pan, Y. Zhou, Z. Wang and M. Zhou, Catalysis and Regioselectivity of the Aqueous Heck Reaction by Pd(0) Nanoparticles under Ultrasonic Irradiation, J. Org. Chem., 2006, 71, 4339–4342 CrossRef CAS PubMed;
(j) R. R. Deshmukh, R. Rajagopal and K. V. Srinivasan, Ultrasound promoted C–C bond formation: Heck reaction at ambient conditions in room temperature ionic liquids, Chem. Commun., 2001, 17, 1544–1545 RSC;
(k) G. An, X. Ji, J. Han and Y. Pan, Ultrasound-Promoted Ligand-Free Heck Reaction in Water, Synth. Commun., 2011, 41, 1464–1471 CrossRef CAS.
-
(a) G. N. Hermann, P. Becker and C. Bolm, Mechanochemical Iridium(III)-Catalyzed C-H Bond Amidation of Benzamides with Sulfonyl Azides under Solvent-Free Conditions in a Ball Mill, Angew. Chem., Int. Ed., 2016, 55, 3781–3784 CrossRef CAS PubMed;
(b) T. Raj, H. Sharma, Mayank, A. Singh, T. Aree, N. Kaur, N. Singh and D. O. Jang, “Solvent-Less” Mechanochemical Approach to the Synthesis of Pyrimidine Derivatives, ACS Sustainable Chem. Eng., 2017, 5, 1468–1475 CrossRef CAS;
(c) A. Y. Li, A. Segalla, C.-J. Li and A. Moores, Mechanochemical Metal-Free Transfer Hydrogenation of Carbonyls Using Polymethylhydrosiloxane as the Hydrogen Source, ACS Sustainable Chem. Eng., 2017, 5, 11752–11760 CrossRef CAS;
(d) Z.-Z. Gu, F.-C. Guo, P. Zhang, Y.-J. Qin and Z.-X. Guo, Solvent-free mechanochemical synthesis of diacylfuroxans, Tetrahedron Lett., 2019, 60, 1687–1690 CrossRef CAS.
-
(a) G.-W. Wang, Chem. Soc. Rev., 2013, 42, 7668–7700 RSC;
(b) Y. Pang, T. Ishiyama, K. Kubota and H. Ito, Iridium(I)-Catalyzed C-H Borylation in Air by Using Mechanochemistry, Ito, Chem.–Eur. J., 2019, 25, 4654–4659 CrossRef CAS PubMed.
- R. B. N. Baig and R. S. Varma, Alternative energy input: mechanochemical, microwave and ultrasound-assisted organic synthesis, Chem. Soc. Rev., 2012, 41, 1559–1584 RSC.
-
(a) J. S. Yadav, B. V. S. Reddy, P. Sreedhar, G. Kondaji and K. Nagaiah, Mild and efficient conversion of aldehydes to gem-diacetates using 2nd generation ionic liquids, Catal. Commun., 2008, 9, 590–593 CrossRef CAS;
(b) B. H. Lipshutz, S. Ghorai and M. Cortes-Clerget, The Hydrophobic Effect Applied to Organic Synthesis: Recent Synthetic Chemistry “in Water”, Chem.–Eur. J., 2018, 24, 6672–6695 CrossRef CAS PubMed;
(c) T. N. Ansari, F. Gallou and S. Handa, Palladium-catalyzed micellar cross-couplings: An outlook, Coord. Chem. Rev., 2023, 488, 215158–215174 CrossRef CAS.
-
(a) D. Xu, C. Lu and W. Chen, Palladium-Catalyzed Double Arylations of Terminal Olefins in Acetic Acid, Tetrahedron, 2012, 68, 1466–1474 CrossRef CAS;
(b) G. Zha, Q. Zheng, J. Leng, P. Wu, H. Qin and K. B. Sharpless, Palladium-Catalyzed Fluorosulfonylvinylation of Organic Iodides, Angew. Chem., Int. Ed., 2017, 56, 4849–4852 CrossRef CAS PubMed;
(c) P. M. Murray, J. F. Bower, D. K. Cox, E. K. Galbraith, J. S. Parker and J. B. Sweeney, A robust first-pass protocol for the Heck–Mizoroki reaction, Org. Process Res. Dev., 2013, 17, 397–405 CrossRef CAS;
(d) C. Shen, H. Shen, M. Yang, C. i. Xia and P. Zhang, A novel d-glucosamine-derived pyridyl-triazole@palladium catalyst for solvent-free Mizoroki–Heck reactions and its application in the synthesis of Axitinib, Green Chem., 2015, 17, 225–230 RSC.
- For selected reviews and examples, see:
(a) J. Xie, R. Liang and Y. Jia, Recent Advances of Catalytic Enantioselective Heck Reactions and Reductive-Heck Reactions, Chin. J. Chem., 2021, 39, 710–728 CrossRef CAS;
(b) R. Liang and Y. Jia, Aromatic π-Components for Enantioselective Heck Reactions and Heck/Anion-Capture Domino Sequences, Acc. Chem. Res., 2022, 55, 734–745 CrossRef CAS PubMed;
(c) J. Yu, H. Shou, W. Yu, H. Chen and W. Su, Mechanochemical Oxidative Heck Coupling of Activated and Unactivated Alkenes: A Chemo-, Regio- and Stereo-Controlled Synthesis of Alkenylbenzenes, Adv. Synth. Catal., 2019, 361, 5133–5139 CrossRef CAS;
(d) D. Xu, C. Lu and W. Chen, Palladium-catalyzed double arylations of terminal olefins in acetic acid, Tetrahedron, 2012, 68, 1466–1474 CrossRef CAS.
|
This journal is © The Royal Society of Chemistry 2024 |