DOI:
10.1039/C7RP00140A
(Review Article)
Chem. Educ. Res. Pract., 2017,
18, 518-532
Reasserting the role of pre-laboratory activities in chemistry education: a proposed framework for their design
Received
24th July 2017
, Accepted 28th August 2017
First published on 28th August 2017
Abstract
In this article we summarise over 60 reports and research articles on pre-laboratory activities in higher education chemistry. In the first section of the review, we categorise these activities as follows. First are those intending to introduce chemical concepts, that typically take the form of a pre-laboratory lecture, pre-laboratory quizzes, and pre-laboratory discussion. Second are those intending to introduce laboratory techniques, that typically take the form of interactive simulations, technique videos, mental preparation, and safety information. Finally, a small number of activities intended to prepare students for affective aspects of laboratory work, in the form of enabling confidence and generating motivation are described. In the second section of the review, we consider a framework for design of pre-laboratory activities that aligns with the principles of cognitive load theory. We propose how the two tenets of such a framework – supporting learners in complex scenarios and provision of information necessary to complete tasks – can be considered for the case of preparing for laboratory learning. Of particular relevance is the nature of information provided in advance and that provided just in time, characterised as supportive and procedural information respectively. Finally, in the concluding section, we draw together the principles outlined in the framework and findings from reports of pre-laboratory work in chemistry to propose five guidelines for those wishing to incorporate pre-laboratory activities into their laboratory curriculum; an activity we argue has a significant literature basis for us to encourage.
Introduction
Laboratory education is a core component of the undergraduate chemistry curriculum. Professional bodies in chemistry detail minimum amounts of time to be spent on laboratory work; the Royal Society of Chemistry require undergraduate Bachelor degrees to include at least 300 hours of laboratory work, excluding undergraduate research projects (RSC, 2015); while the American Chemical Society require 400 hours of laboratory experience beyond introductory level (ACS, 2015).
Laboratory work emerged into the modern curriculum through the lecture demonstration, as a result of the desire for students of chemistry not only to “see” chemistry in action, but also to perform chemistry themselves. This was coupled with a desire for students to learn the technical approaches involved in determining various observations and theories of a course. Thus laboratory education became a separate and distinct component of education, with the emphasis intended to teach students about how to “do science”. Anderson formalised this distinction when he described learning about “science” and learning about “sciencing” (Anderson, 1976). Building on this, and the work Woolnough and Allsop (Woolnough and Allsop, 1985), Kirschner distinguished between substantive and syntactical structures of science (Kirschner, 1992). The former concerns the corpus of knowledge that is a result of research and development in chemistry, along with the corresponding intellectual discourses and philosophical debates. It can be considered as theory, consisting of concepts, ideas, and laws. The latter concerns the way scientists do science, encompassing habits, skills, and methods of scientific inquiry.
Thus laboratory education forms a unique and integral component of the chemistry curriculum. Much has been written about the purpose of laboratory work, and how that purpose is integrated into the manner in which the laboratory curriculum is designed and the experience of students (Boud et al., 1986; Johnstone and Al-Shuaili, 2001; Hofstein and Lunetta, 2004; Reid and Shah, 2007). In much of this work, problems with laboratory education are identified. It is not the purpose of the present article to discuss the very broad literature on laboratory learning in general, but rather to focus on issues pertinent to pre-laboratory work.
In this article, we aim to achieve the following:
(1) Summarise the literature on pre-laboratory work in higher education chemistry over the last four decades, identifying the reported nature, type, and purpose of pre-laboratory activities;
(2) Describe a framework that can be used as a basis for considering pre-laboratory activities;
(3) Propose a series of guidelines for the design and implementation of pre-laboratory activities that are consistent with the framework described.
Methods
In the first part of this article, we aim to summarise and categorise reports that describe pre-laboratory activity in chemistry at university level. In order to achieve this, the Web of Science search index was used to source articles describing laboratory preparation, pre-labs, and similar terms. Results obtained were filtered initially for chemistry, and then upon reading, filtered again to remove those that did not relate to university level chemistry. Cited and citing references of these articles were consulted to identify additional relevant material. Given the very extensive nature of literature on laboratory education, we do not consider to have captured all of the reports on pre-laboratory activities, but do consider that we have captured a suitable sample size to derive the typologies represented. It is worth stating that while interesting literature on laboratory education relevant to modern teaching extends back to at least the 1930s, our survey starts in the early 1970s, as technologies emerged that made alternatives to the pre-laboratory lecture possible. In addition, where possible, we identified the type of laboratory (general, analytical, organic, physical) and state that wherever possible when summarising a report.
Part 1: a review of pre-laboratory work
As laboratory work followed on from teaching approaches relying on lecture demonstrations, the default pre-laboratory activity was the pre-laboratory lecture. Description of a typical pre-laboratory was thus: “a ![[s with combining low line]](https://www.rsc.org/images/entities/i_char_0073_0332.gif)
![[h with combining low line]](https://www.rsc.org/images/entities/i_char_0068_0332.gif)
![[o with combining low line]](https://www.rsc.org/images/entities/i_char_006f_0332.gif)
![[r with combining low line]](https://www.rsc.org/images/entities/i_char_0072_0332.gif)
lecture presented at the start of the laboratory period… theory relevant to the experiment is reviewed – time permitting. But generally, the emphasis is placed on discussion of laboratory procedures directly related to the experiment” (underlined emphasis in original) (Fine et al., 1977). Changes in curriculum organisation because of larger class sizes meant that laboratory work began to be decoupled from lectures, and became more stand-alone in nature. In addition, the pressures of time on the pre-laboratory lecture, as indicated by the contemporary quote above, meant that there was a conflict between time spent on the pre-laboratory and time spent in the laboratory itself. Hence, new ideas about the way pre-laboratory work could be presented emerged, and along with this came new objectives of pre-laboratory work. We survey below the main themes found in reports describing pre-laboratory activities in chemistry beginning around the time of Fine's account 40 years ago. These general approaches are summarised in Fig. 1.
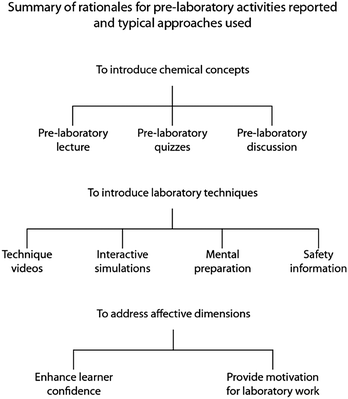 |
| Fig. 1 Summary of rationales for pre-laboratory activities reported and typical approaches used. | |
1.1 Understanding of chemical concepts
(a) Pre-laboratory lecture.
Of course changing format did not mean that the primary purpose of pre-laboratory activity changed, and much of the early literature focussed on different ways to engage students with the chemical concepts that they would meet in the laboratory session. One way was to offer a version of the pre-laboratory lecture. Indeed Fine's own work describes an innovative approach where lecture slides were prepared to explain the chemical concepts of a general chemistry laboratory; these could be used by the lecturer or by the student as “self-pacing instructional programs” at any time before the laboratory session (Fine et al., 1977). Because this approach aimed to mimic and replace the traditional pre-laboratory lecture, these materials also included details on experimental protocols (see below). Much of the work published since reflects the desire to present students with some materials relating to the chemical concepts involved in the laboratory. Indeed, one of the most recent publications in our survey described the use of pre-laboratory video lectures to introduce concepts in advance of the upper-level undergraduate laboratory sessions (Schmidt-McCormack et al., 2017). The rationale for this approach was to overcome timetabling issues that meant pre-laboratory lectures had been presented several weeks prior to the laboratory session, as well as that some students may have had to complete the laboratory before the attending the corresponding lectures. A common theme across all pre-laboratory activity is that it offers a structure upon which students will focus their efforts. Kirk and Layman describe their approach of using a pre-laboratory guide to help students connect laboratory work with both the lectures and their prior chemistry knowledge (Kirk and Layman, 1996). Students using the pre-laboratory guide felt better prepared to conduct experiments and had a clearer understanding of chemical concepts and the scientific processes.
(b) Quizzes.
As technology developed, incorporation of quizzes to ensure students had completed work were described for general (Kolodny and Bayly, 1983) and organic chemistry (Starkey and Kieper, 1983). These were designed so that students had to achieve a minimum score before being accepted into the laboratory class. In the former case, the rationale was to “force” students to read the laboratory manual, although the design itself included a valuable feedback loop. Students selecting an incorrect answer to a pre-laboratory question were automatically directed to teaching materials about that concept, with the aim of offering guidance on the correct answer. In the latter, students were required to answer 5 multiple choice questions on the theory, methodology, and safety precautions of the experiment. Students were told whether their answers were correct or not, and they needed to repeat the quiz until they got all of the answers correct.
The use of quizzes with feedback to reinforce chemical concepts relating to the laboratory have continued into the last decade. Quizzes with questions designed to improve links between theory and practical work by means of providing immediate feedback to students were described for students in general chemistry courses (Chittleborough et al., 2007). Correct responses were reinforced, while incorrect answers prompted some guiding feedback, with students being allowed a second attempt. The overall exercise was worth 2% of the laboratory mark, but evaluation indicated that students appreciated the feedback cycle and felt it helped their learning. In addition, reflecting earlier work, students reported that it “forced” them to prepare in advance. Pre-laboratory quizzes that presented different questions to different students were also reported (Gammon and Hutchinson, 2001), although in this case they were hand-graded with feedback after the event.
(c) Discussion.
Another approach for introducing chemical concepts in advance of the laboratory was to facilitate discussion in advance of the practical session. Domin described, as part of a problem-based learning practical course, the introduction of problems in advance of the laboratory class that students would use to source information in advance, and use as a basis to work coöperatively in the laboratory to develop a procedure to solve a given problem (Domin, 2007). Interviews with students highlighted that they were more cognitively engaged with laboratory work than they were during more traditional expository laboratories. “Pre-laboratory Preparation Periods” were used by Isom and Rowsey (Isom and Rowsey, 1986). Students in a large general chemistry class were divided into groups of about 12, which met for 45 minutes one or two days in advance of the laboratory session. The purpose of the session was to brief students and allow for discussion on the forthcoming session. Students who were assigned to the small group sessions performed significantly better in laboratory reports overall, although the difference varied depending on the nature of the laboratory; a larger difference in performance was noted for those practicals that were based on more abstract concepts. A similar approach worked well for high school chemistry (Smith, 1987).
A model for increasing and formalising the amount of pre-laboratory discussion was reported for a traditional Hess' Law experiment, with the authors reporting that students had a much better grasp of core concepts as a result of the formalised discussion (Davidowitz et al., 2003). A related model where students had to do some pre-laboratory planning before coming to the inorganic chemistry lab, and subsequently use this planning as a basis for discussion with a demonstrator before beginning practical work was reported (Johnstone et al., 1994). Students reported that the pre-laboratory preparation helped them understand what was occurring in the lab, as well being useful for their post-laboratory analysis. The latter observation regarding linking pre-laboratory and post-laboratory work was noted in several reports (Kolodny and Bayly, 1983; Nichols, 1999; McKelvy, 2000; Limniou et al., 2009).
1.2. Laboratory skills and the laboratory process
A second set of objectives around a theme of preparation for the technical aspects of laboratory work was found in the literature on pre-laboratory activities. This was often in parallel with the introduction of chemical concepts underpinning a laboratory, described above, that is unsurprising given that this is based on what would be considered to be the overall purpose of a traditional pre-laboratory lecture. De Meo has provided a useful review of approaches taken to teach chemical technique, including preparatory aspects, since Michael's Faraday's book Chemical Manipulation (DeMeo, 2001). We supplement this below by considering general themes proposed by various educators to prepare students for laboratory tasks that they would be completing. It should be noted that there are many more reports describing this set of objectives than there are for any other aspect of pre-laboratory preparation.
(a) Interactive simulations.
New computer capabilities led to the introduction of electronic quizzes, described above, that were also used for testing students’ knowledge of technical aspects of laboratory work (Starkey and Kieper, 1983). The new possibilities computers offered were also quickly harnessed by using interactive simulations, that aimed to allow students mimic some processes in the laboratory on the computer in advance. An impressive early example of this involved a graphical interface allowing students mix reagents, set up apparatus and use instrumentation (Moore et al., 1980). The authors reported that the simulations facilitated students' performance, particularly when the experiment was less structured, and decision-making steps were incorporated. Simulations have also been used with reported positive outcomes for introducing experimental design aspects (Limniou et al., 2007, 2009; Abdulwahed and Nagy, 2009, 2011). Various aspects in relation to the design of simulations are discussed in work describing a simulation of an extraction technique in organic chemistry (Supasorn et al., 2008). Nichols described a suite of interactive activities students needed to complete before attending the inorganic chemistry laboratory. These included aspects of performing the technique (e.g. weighing by difference) and subsequent calculations. Students needed to achieve 70% in the associated test before being allowed into the laboratory. Nichols reported that in general students needed more than one attempt to achieve the minimum mark, but often continued to repeat the pre-laboratory work until their score was closer to 100% (Nichols, 1999). Winberg and Berg used a simulation on buffers to prepare students for a pH laboratory that allowed students to vary experimental parameters and found that as a result of the simulation, students asked more theoretical questions compared to those in a control group (Winberg and Berg, 2007). Simulations are also becoming more common in school education (Gryczka et al., 2016) that may result in students arriving at university considering them to be part of the overall laboratory teaching approach.
(b) Mental preparation.
Pre-laboratory work in preparation for discussions about experimental technique were also reported, where students were asked to write out the procedure of the practical and submit it with their pre-laboratory questions (Rollnick et al., 2001). De Meo groups approaches such as discussion and advanced written work under a general theme of mental preparation (DeMeo, 2001). Most of the reported work in chemistry laboratory education on mental practice is reported by Beasley (Beasley, 1979, 1985; Beasley and Heikkinen, 1983). This involved prompting students to think out in their mind the steps they will complete in an experimental technique (a titration), and to relate these steps to an illustration provided. He found that there was no difference in performance between students who completed mental practice alone, physical practice, and mental and physical practice, but that there was a difference between these treatment groups and the control group. That is to say, some form of practice had an effect. Pickering described his efforts on encouraging procedural preparation with very high-achieving students (Pickering, 1987). The students were required to prepare their procedure in advance of the laboratory by writing out notes that they could bring into the laboratory. Students were not allowed bring in laboratory books. Students who prepared a detailed procedure which they followed in the laboratory did not perform as well as those who prepared a rough procedure that they updated during their practical work (Pickering, 1987). The later study also found that students who prepared by just writing out the procedure step by step in advance did not complete the laboratory as quickly (or as accurately) as those who used “telegraphic summaries”, although it didn’t matter whether these summaries were in the form of a flow chart or a table of instructions.
A similar strategy was implemented as part of ongoing changes to a general chemistry laboratory course described by Smith (Smith, 1987). In this case, pre-laboratory quizzes were replaced by pre-laboratory assignments that required students to outline what data would be collected, what equipment was needed, and a workflow of the laboratory procedure. This was coupled with the incorporation of “pre-discussion” exercises, to prepare students for small group work involving pooling experimental data or adding in additional data to their analyses. While the focus of the preparative work was on procedural details, students reported in feedback that they had a better understanding of concepts. Technology is continuing to enable new possibilities in facilitating on-going discussion and preparation: the use of a cloud-sharing platform was described by Weibel to allow annotations and discussion with students on their laboratory preparation work (Weibel, 2016).
(c) Technique videos.
A third category of approaches to preparing students for laboratory work is the use of technique videos. Very early work on video demonstrations was completed at Surrey, UK, with reports on videotape materials being used to support laboratory teaching. An observation reported was that staff and teaching assistants had to intervene less that was reported prior to their use (Simpson, 1973; Watson, 1977). A more formal study exploring the effect of providing students with video demonstrations of organic chemistry procedures prior to their entry into the laboratory resulted in an improvement in laboratory activity (Kempa and Palmer, 1974). Students were either shown videos and given written instructions, shown a video only, or given written instructions only, and both groups who were shown the video demonstrated superior experimental skills in the laboratory when compared to the students who had only received written instructions A subsequent study explored the effect of a 24 hour time-delay between watching video and completing laboratory work on this performance and found little difference from the original experiment, except that the performance of those students receiving both written and video instructions was superior to video alone (Neerinck and Palmer, 1977). The use of video became increasing popular; a report in 1993 stated that nine of the seventeen UK universities responding to a survey reported that they used videos in their laboratory courses for teaching materials (Meester and Maskill, 1993). An approach to designing these videos was published at this time. Researchers videoed students completing a procedure, and completed an analysis of the videos to identify errors. These were found to fall into the categories: preparation of equipment; level of care taken and concern for accuracy; and students performing procedures “without thinking of the likely consequences of their actions” (McNaught et al., 1993). These were used to design simulations which included video clips on technique. The approach led to a reported improvement in performance of technique.
In a study mirroring those reported by Palmer, above, the times required for students to complete a kinetics experiment were measured for three different scenarios, with each one having a different format preparatory information. In the first, students were provided with written instructions. In the second, students were given video instruction where the text was presented as audio to augment pictures of what is being described. And in the third, students were provided with an interactive computer programme which included videos (Burewicz and Miranowicz, 2006). The researchers found that the time spent on preparation was shortest for written instruction, but that students assigned to the video and interactive program groups were much more efficient in their practical task, especially with regards to setting up apparatus, using software and taking measurements. Students who were given only written preparatory material made almost 6 times as many mistakes as those who received video and interactive preparation, and were over 4 times more likely to complete tasks incorrectly or with uncertainty. Overall the authors report that manual activities were assisted equally well by video and interactive activities, while training in computer programme use was best assisted by preparation using interactive activities. No difference between formats was observed with regards to theoretical preparation.
While video has a long history, its use in the last two decades has expanded dramatically and some recent examples representing current approaches are summarised here. Echoing Kempa's early work, use of videos in organic chemistry were found to increase the post-test laboratory quiz scores of students who had watched videos compared to those who didn't, and these students also completed the practical work more quickly (Nadelson et al., 2015). These authors observed a pre-/post-test quiz score increase of over 10% for students who had watched videos, compared to 4% for those who hadn't. Nadelson's work was grounded in transfer of knowledge – the transfer of task-specific knowledge by means of experts modeling or demonstrating a process for novice learners. This expert-modelling approach was used in a study on the value of preparative videos for teaching laboratory skills (Seery et al., 2017). Students were required to use these exemplar videos as a basis for preparing videos of their own techniques in the laboratory class. The intention was grounded in the literature on formative assessment, advocating the provision of exemplary approaches so that learners could consider their own work in comparison to the exemplar, and make any changes prior to presenting their work for assessment (Sadler, 1989; Hendry, 2013). Analysis found that students' ability to answer technique-related questions improved as a result of the process. Similar findings were reported by Powell and Mason, who reported that students in general chemistry who had access to video (described as podcasts) needed fewer scaffolding interactions in the laboratory compared to those who didn’t, and these students were able to acquire their results more efficiently (Powell and Mason, 2013).
Students using video preparation were reported to need less support in an organic chemistry laboratory compared to students who received in-laboratory instruction from teaching assistants (Jordan et al., 2015). A parallel study also explored the different types of video that students found most useful (Box et al., 2017). Videos relating to technique (microscale distillation), use of instrumentation (GC), and calculation based on instrumental (GC) output were prepared; each of the three being relevant to an experiment students were to complete. Students' responses to questionnaire were better in the experimental group, and in particular a large effect size was noted for the questions associated with use of instrumentation (GC), although students themselves ranked the techniques video most useful. It was observed in both studies that students who watched videos spent less time on the tasks in the lab.
Tan and co-workers described the implementation of videos via a framework of flipped teaching, offering students video in advance of laboratories in introductory inorganic chemistry and an organic chemistry lab, both involving the provision of videos about synthetic procedures (Teo et al., 2014). The ‘flipped’ framework, increasingly common in lecture courses (Seery, 2015), was used here to explicitly ensure links between pre-laboratory work and in laboratory work were tangible. Interviews with students suggested that the videos helped “unpack” written laboratory procedures that students found difficult to interpret by means of showing the videos in practice. In addition, while the focus was on improving technique, students reported that they also felt more comfortable with the underpinning theory as a result of watching videos in advance, mirroring the findings of Winberg and Berg (2007). Fung also describes the use of pre-laboratory videos as “flipped”, and outlines a novel procedure for creation of first-person perspective videos (Fung, 2015).
Cole and co-workers described the use of videos to complement advanced practicals in physical and analytical chemistry, which because of the rotation nature of experiments, mean that pre-laboratory lectures at the start of a semester can result in a long lag time between pre-laboratory information and the completion of the laboratory work. As such, videos summarising theory, experimental detail, and data analysis were provided to students (Schmidt-McCormack et al., 2017). The authors stated that the approach alleviated some of the challenges of rotation style experiments, with students becoming more autonomous and confident in their laboratory work, and it was also noted that queries regarding instrumentation tended to be more complex, in place of queries about routine approaches that were covered in pre-laboratory videos. This article also presented some design aspects of pre-laboratory videos, grounded in cognitive theory of multimedia learning.
(d) Safety Information.
A final category identified in the preparation of students for laboratory activities was that relating to safety information. While it is likely that many of the technique videos discussed in the literature did describe safety aspects (e.g.Nichols, 1999; Chaytor et al., 2017), only a few reports mentioned this as an explicit consideration. Meester and Maskill describe in their survey of English and Welsh universities that students may be asked to assess safety aspects or submit a safety data sheet in advance of the practical session (Meester and Maskill, 1995). Polles explored students' opinions of their laboratory experiences and found that they thought that they were not adequately prepared for safety issues, and this observation only came to light after experience of dealing with irritating fumes (Polles, 2006). In their discussion on hygiene and safety in the laboratory, Miller et al. advocate the incorporation of a safety component into pre-laboratory work (Miller et al., 2000).
This could be achieved by requiring students to research the Material Safety Data Sheet (MSDS) as part of the pre-laboratory assignment (Walters et al., 2017). An interesting example of incorporating safety into pre-laboratory work is reported by Alaimo et al., who describe the integration of activities to develop awareness of safety into their laboratory curriculum (Alaimo et al., 2010). Students in organic chemistry laboratories were given safety lecture in advance of the laboratory course which included demonstrating and trialling safety equipment such as showers. For each week of the course, different groups of students were assigned to be the “Safety Team” for that week, responsible for a range of activities, including a pre-laboratory safety presentation delivered to the rest of their class. This took the form of a 5 minute discussion and was supplemented with a handout prepared by students on the hazards and risks associated with an experiment – these having been checked by the instructor prior to the session. The authors reported several benefits to this pre-laboratory aspect of their initiative, including students taking an increased level of responsibility in the laboratory session, as evidenced by, for example, students pointing out ways that their laboratory work could be conducted more safely (Alaimo et al., 2010).
1.3. Affective experiences
(a) Confidence.
There is little literature on the use of preparation materials for laboratory work with the rationale of addressing any of the affective components of completing practical chemistry. However, many authors report that as an observation, student confidence improves (or anxiety reduces) (Kolodny and Bayly, 1983; Merritt et al., 1993; Kirk and Layman, 1996; Nicholls, 1998; Koehler and Orvis, 2003; Chittleborough et al., 2007; Keen-Rocha, 2008; Limniou et al., 2009; Box et al., 2017; Chaytor et al., 2017). In the analysis by Seery et al. on the use of pre-laboratory videos to prepare for laboratory techniques, it was found that students' confidence increased significantly from before (Seery et al., 2017), although it should be noted that this effect was also observed in the work of Towns (Towns et al., 2015; Hensiek et al., 2016) which also described in-laboratory demonstrations by students, but did not involve preparatory activity. An exploration of students' sense of preparedness for analytical chemistry laboratories reported that the availability of pre-laboratory videos and quizzes in advance of class resulted in more students feeling somewhat or very prepared when compared to their responses at the beginning of semester (Jolley et al., 2016). Similar findings regarding students' increased sense of preparedness were reported by Chaytor (Chaytor et al., 2017).
(b) Motivation.
In fact there is little work on affective experiences generally in the chemistry laboratory, but Galloway and Bretz's work point to the importance of considering the affective domain, including in preparative activities. In rich and descriptive studies, they quote one student who, upon reflecting on the laboratory work completed, stated that he felt intimidated in advance, whereas if some detail (pictures) of the procedure were available, he mightn't have felt so (Galloway and Bretz, 2016; Galloway et al., 2016). Other observations relating to preparative work highlight the sense students felt about being organised for a practical session. This resulted in the student expressing confidence a sense of motivation, along with the use of preparation to reduce anxiety. Motivation was also an observed outcome of a practical course described by Pogačnik and Cigić, who described the use of introductory discussions and a pre-laboratory test for general and biochemistry courses (Pogačnik and Cigić, 2006). They found that, even faced with increased demands because study and preparation time, students were more motivated to enter the laboratory. In a large scale study on the general laboratory experience in Australia, the authors reported that there was a very clear relationship between generating interest in an experiment and motivation, which resulted in a positive overall laboratory experience (Barrie et al., 2015). While this was indicated for the laboratory generally, there are clear lessons for the role of pre-laboratory activities in generating such interest.
1.4. Summary
In this first part of the review, we have summarised a survey of over 60 research studies and reports and categorised them by purpose according to three general intentions: to prepare students for theoretical aspects of laboratory work; to prepare them for experimental work; and to generate positive affective aspects relating to laboratory work. Our summary has demonstrated a general principle that has been repeatedly observed in a variety of educational contexts and student levels within university education and by a variety of approaches: pre-laboratory activities result in multiple benefits with regards to subsequent learning in the laboratory. A summary of some overarching benefits noted from pre-laboratory activities is summarised in Table 1.
Table 1 Advantages of integrating pre-laboratory activities – synopsis of findings
Category |
Summary finding |
Overall |
Pre-laboratory activity tends to have a positive impact on learning in the laboratory. The nature and purpose of the activity will depend on the context and purpose of the laboratory in question. |
|
Experimental |
Pre-laboratory activity tends to increase the work requirements of students outside of formal class time, but once this is structured, students tend not to complain about this extra work. |
Pre-laboratory activity tends to increase the efficiency of students’ laboratory work, and reduce the time spent on experimental tasks. |
Pre-laboratory activity enables students to carry out routine experimental work in the lab, and reserve questions for more complex techniques. |
Pre-laboratory activity tends to result in fewer experimental errors being made by students. |
Pre-laboratory activity which require students to outline experimental approaches tends to result in improved understanding and efficiency of laboratory tasks, especially when students are prompted to consider overall approaches rather than stepwise instructions. |
|
Conceptual |
Pre-laboratory activity, even if it is focussed on experimental approaches, tended to result in students discussing conceptual aspects more or feeling better informed about conceptual aspects. |
Pre-laboratory activity which prepared students for conceptual aspects of laboratory work tends to result in students performing better in the laboratory. |
Pre-laboratory activity which presents conceptual ideas of laboratory work tends to lead students to feeling more autonomous about completing their laboratory work. |
|
Affective |
Pre-laboratory activity enables students to feel more confident about laboratory work, and/or reduces student anxiety about knowing what to do during their practical session. |
Pre-laboratory activity could motivate students about doing practical work, although there may need to be an extrinsic driver (such as assessment reward) to complete these activities. Activating interest in the purpose of the practical work can be a motivating factor. |
We have observed, in general terms, that pre-laboratory activity tends to focus on preparing students for experimental aspects of laboratory work or preparing them for understanding the underlying theoretical knowledge. An obvious question emerges: what kinds of information should we provide in advance? In the next section we draw upon a framework for learning in complex environments to consider how learning in complex environments can be supported, and in particular what kinds of information should be presented to learners as they embark on completion of practical activity.
2. A framework to support learning in a complex learning environment
A general finding from the literature on pre-laboratory activities is that incorporating some form of preparation has benefits. Given the emerging positive findings about the benefits of preparation for lecture activities – currently enjoying a lot of interest because of the “flipped” lecture movement – this should be of little surprise (Seery, 2015). The task now becomes one of describing an underlying framework to give a basis to the various reported benefits. The purpose here is to define criteria that may explain what aspects of preparation have benefit, so that those looking to prepare preparatory activities can approach their design with a clear rationale for their particular educational setting. In this section, a reported framework describing learning in a complex learning environment is presented and illustrated with particular reference to laboratory work by highlighting potential alignment with some of the studies and findings reported above.
2.1. The laboratory as a complex learning environment
One difficulty facing those looking to address issues regarding learning in the laboratory is that it is an extremely complex system, and indeed it has been recommended that that best way to study learning in the laboratory is as a complex ecological system, given the interdependence of various factors involved (McComas cited in Rollnick et al., 2001).
What does a complex learning environment mean? In the educational psychology literature, complex learning has been extensively studied, and one useful structure upon which to consider it is cognitive load theory. Cognitive load theory offers a description for the amount of mental effort that is expended in any given learning scenario. The “load” refers to the load on the working memory, which is of finite capacity. Load can be in the form of intrinsic load, which is related to the difficulty of the material, extraneous load, which is related to the difficulty of extracting required information from materials, and germane load which considers the integration of new knowledge with long term memory (Sweller et al., 2011). Long ago, Johnstone and Wham described the difficulty of laboratory work for learners in terms of working memory load (Johnstone and Wham, 1982):
Learning is severely hampered in a high information situation in which the working memory (of finite capacity) is overloaded with incoming data. From the teacher's point of view, the material he is trying to teach is well understood and well organised. To the learner, who does not yet have a grasp on the ideas, the position may look very different. The incoming information may have no apparent structure because the very idea being taught is needed at the start to organise the new information. This is a vicious circle in which the learner is trapped. He cannot discern what is important and what is incidental, what is the point of lesson and what is merely supportive or peripheral in nature. Nowhere is this more evident than in the laboratory.
The authors continue to suggest why practical work imposes a significant load (Fig. 2) and conclude:
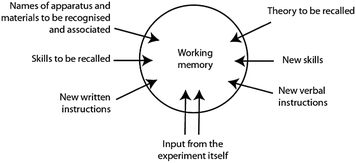 |
| Fig. 2 The demands of practical work as described by Johnstone and Wham mean that the working memory can become overloaded in laboratory learning environments. From Johnstone and Wham (1982). | |
It is our contention that, for many experiments, this information swamps the working memory leading to an unstable overload state which precludes systematic, intelligent working…
Johnstone argued for the reduction of what he described as “noise” from the students' instructions and activities, and proposed strategies for doing this. These included having clear goals for students and limiting the amount of new processes students were exposed to in any one setting (Johnstone and Wham, 1979, 1982). Such approaches came to be considered in terms of intrinsic and extraneous load in more recent years as cognitive load theory was used by several authors as a rationale for pre-laboratory activities (Johnstone et al., 1994; Limniou et al., 2007; Winberg and Berg, 2007; Abdulwahed and Nagy, 2009, 2011; Limniou et al., 2009; Kolk et al., 2012; Obenland et al., 2014; Box et al., 2017). Rollnick discusses the related issue of “preparedness” – students' ability to prepare depended on their conceptual and procedural skill of the laboratory as a whole (Rollnick et al., 2001). Cognitive load was also considered in terms of design of videos, so that their design was guided by Mayer's cognitive theory of multimedia learning (Schmidt-McCormack et al., 2017).
2.2 Mechanisms to support learning in a complex environment
A framework to describe a complex learning environment consistent with cognitive load theory has been proposed in the education psychology literature. This defines complex learning as follows (van Merriënboer et al., 2003):
(i) Complex learning aims at the integration of knowledge, skills, and attitudes.
(ii) Complex learning involves the coördination of qualitatively different constituent skills.
(iii) Complex learning requires the transfer of what is learned to real settings.
It is feasible to consider how these general aims of complex learning transfer to the specific case of learning in the laboratory. We consider the main tenets of this framework as described by its authors below (van Merriënboer et al., 2003). In tandem, we aim to consider it specifically in the context of laboratory education, with the particular intent of considering the role pre-laboratory work can play to support the complex learning environment observed in laboratory work.
This framework considers: (a) how complex learning can be supported by scaffolding; and (b) the role and nature of presenting just-in-time information, in order to propose an approach for instructional design consistent with cognitive load theory. The intended goals are reducing intrinsic and extraneous load.
(a) Supporting a complex learning task by scaffolding.
Cognitive load theory considers how novice learners can be supported by means of scaffolding – approaches that support students' learning (Rosenshine and Meister, 1992). A key emphasis is that this support needs to be fully embedded into the learning activity. Not to do so means that learners who need most help will likely not use what they perceive to be additional supports for a task, because dividing their attention to that support will increase extraneous load (Carroll and Rosson cited in van Merriënboer et al., 2003). Thus the question arises: how best to integrate support for learners in a complex learning environment?
In general terms, simple-to-complex sequencing is one strategy to reduce intrinsic cognitive load. The intention is to provide learners with the simplest version of the intended task, and proceed to ever more complex iterations (van Merriënboer et al., 2003). A typical approach here is to support learners as they learn various components involved in a complex task, and subsequently combine learners' attention to being able to approach a whole task – called ‘part-task’ approach. However, the feasibility of this approach for a complex task has been questioned (Naylor and Briggs cited in van Merriënboer et al., 2003), because of the difficulty imposed by the integration and coördination of component skills, knowledge, and attitudes within a complex learning environment.
Instead, supporting ‘whole-task’ approaches is considered more beneficial for supporting complex tasks. This involves supporting the coördination of the constituent skills from the outset, with an emphasis on the overall view of the task at hand. Integration with the eventual overall task's complexity involves setting out with the simplest conditions of the whole task – one in which the overall basis of generalised knowledge is needed – followed by increasing complexity of “task classes”, characterised by requirements of more knowledge or more interactions between the components of the task (van Merriënboer 1997 in van Merriënboer et al., 2003).
In their work, Johnstone and Wham alludes to the problems laboratory work impose with regards to considering whole task approaches. Students faced with a whole task during their laboratory session, which comprises of a number of constituent tasks, will become overly focussed on particular tasks at hand. They use the example of students completing a rate experiment becoming overly focussed on the requirements of preparing dilutions, which obscures their focus on the main task of measuring rates of reaction of solutions of different concentrations (Johnstone and Wham, 1982). This may illustrate the general principle about difficulty of integrating various components of a whole task, as well as the problems generated by not providing students with a clear overall picture of the whole task in advance. The latter approach would intend that students can first be accustomed to this “task class” prior to incorporating more nuanced details that experimental work will require.
How might this be alleviated? Perhaps one of the reasons simulations have demonstrated benefit is that they enable familiarisation of the overall approach, without the specific requirements needed to conduct these approaches experimentally (Supasorn et al., 2008). Students can develop mental models and become familiar with the whole-task procedure in a simplified setting, before entering the laboratory and completing it in reality. Both Pickering (Pickering, 1987) and Smith (Smith, 1987) demonstrate that requiring students to think about planning laboratory tasks in advance of laboratories had benefit. In particular, Pickering illustrated with his work that students who conceived overall summaries that did not dwell on specific detail tended to perform better in the laboratory than those who prepared by outlining specific task details in advance. This observation could be linked to the idea of preparing for a task in terms of overall approach. Another possible strategy is video: reports on preparatory videos that distinguished between experimental, theoretical, and post-laboratory analysis aspects of laboratory work (Schmidt-McCormack et al., 2017) may be meritorious as they will allow students to engage with the specific experimental protocol in advance, before being required to integrate other more complex aspects of analysis. This is discussed further in the context of “just-in-time” resources, below.
Scaffolding learners by means of whole-task support is a strategy to reduce intrinsic cognitive load. Scaffolding by providing learners with structured approaches is a strategy to reduce extraneous cognitive load. In this case, the intention is to alleviate working memory demand caused by learners figuring out what processes they need to use to complete the task under consideration. A common strategy is to provide worked examples; guidance on the various stages involved in the completion of a task (Sweller et al., 2011). However, referring the issue of requiring such activities being embedded into learning noted above, it is found that worked examples can be passive; they do not require learners to study each stage carefully. If only consulted at the point of needing assistance, the resultant load of considering both the scenario and the worked example becomes very high (van Merriënboer et al., 2003).
Instead, completion tasks (fading) are advocated – tasks where a partial solution is provided and learners are required to complete it to arrive at a stated goal. Such tasks will require learners to be active, as they will need to study the partial example provided in the task. Some reported strategies on pre-laboratory activities may facilitate completion tasks, especially in the context of requiring students to become familiar with the overall laboratory strategy. Such completion tasks could be facilitated by strategies such as that reporting quizzes with individual feedback (Chittleborough et al., 2007), strategies requiring students to plan out procedures with guidance coming in the form of discussion (Isom and Rowsey, 1986; Pickering, 1987; Domin, 2007), or activities requiring students to complete some decision making based on some information provided (Moore et al., 1980).
(b) Providing information in advance of a complex learning scenario.
The previous section discussed how cognitive load theory provides guidance on how we can support learners in complex environments, and alluded to specific examples of how this might look in laboratory learning. In this section, we continue onto the second aspect proposed by van Merriënboer et al. (2003) to be considered with regards to learning in complex environments: provision of information needed by learners to complete a task. This will enable us to consider in more depth the role of pre-laboratory activity in supporting laboratory work.
In order for learners to complete any complex task presented, they will need information necessary to complete that task. A strategy therefore is to provide that information to learners in advance of completing the task, so that learners can draw on this when they are completing the task. In terms of cognitive load this can be considered as developing schema in the long term memory, so that the demands on the working memory are lowered when the task needs to be performed, as learners will be able to draw required knowledge from these schema. The consistent and repeated observation that pre-laboratory information reduces time on task in the laboratory can be considered in this context (Burewicz and Miranowicz, 2006; Jordan et al., 2015; Nadelson et al., 2015; Box et al., 2017), as can indeed the positive observations regarding the effect preparation has on student confidence and attitude about their laboratory work (Galloway and Bretz, 2016; Galloway et al., 2016; Jolley et al., 2016).
A second strategy for dealing with learning in a complex environment is to provide learners with information just as they need it so that it is available as they complete a task (van Merriënboer et al., 2003). Unfortunately, the authors report that there is not clarity on whether information in advance or information “just-in-time” is best. However, their discussion on what may be the most appropriate approach for particular scenarios does elicit some useful considerations which have obvious parallels with specific aspects we must consider regarding preparation for laboratory work. In order to elaborate on this, we describe two distinct types of information needed by learners in order to complete a task (van Merriënboer et al., 2003):
• Supportive information: experts in a discipline will have a body of knowledge relating to general information guiding approaches in that discipline. An experienced chemist will likely know the general principles of operation of a Schlenk line or a UV/visible spectrometer, or will have implicit schema regarding the kinds of results to expect when studying a kinetic reaction as a function of temperature. This understanding is of a general, abstract nature, and is in general parlance often called ‘theory’. Such information has a high intrinsic complexity.
• Procedural information: some task components will be consistent and recurrent. Our experts described above will easily, and perhaps automatically, be able to complete the tasks required to acquire a UV/visible spectrum on a given instrument, without devoting considerable attention to the process. Such information can be thought of as rules or procedures, and tends to have a low intrinsic complexity.
Having defined two types of information a learner needs, we can now proceed to consider what is recommended about how this information is presented. In describing the two types of information, the perspective of an expert learner was used. But what about a novice? A novice learner completing an experiment requiring the understanding and use of UV/visible spectroscopy will need to draw on information regarding the general principles of the instrument and why the approach they are taking uses it (supportive information) as well as the specific details of using the instrument and the procedure to follow as they complete the work (procedural information). Because the supportive information is of high(er) intrinsic complexity, presenting information to learners during their laboratory time while they are completing tasks would cause overload (Marcus et al., 1996). The procedural information is of low intrinsic complexity, involving routine procedural steps, and can be presented in the laboratory, “just-in-time” using, for example, the laboratory manual or guidance within the laboratory.
Because of the similarity in language, it is worth pausing here to reflect on what supportive and procedural information may mean in the context of learning in the chemistry laboratory, and with regards to the terms “theory” and “procedure”. Given the phrasing, it is tempting to consider that supportive information refers to the “underlying theory” of an experiment, so that in an experiment exploring the relationship between vapour pressures and temperature, such supportive information might discuss intermolecular forces and perhaps the role of the Clausius–Clapeyron equation in elucidating enthalpy of vapourisation. Subsequently, procedural information might then describe, for example, operation of a vacuum line, and other experimental details regarding the technical procedures involved in the experiment.
It is our assertion that the distinction between supportive information and procedural information is more nuanced than this dichotomy in the context of laboratory learning. If supportive information is considered to be an underlying knowledge base upon which the tasks at hand are grounded on, then in the scenario of our student completing an experiment about the relationship between vapour pressure and temperature, supportive information will indeed consider aspects of understanding intermolecular forces, given that this is the chemistry upon which it is grounded. But it will also include aspects related to the experimental protocol; for example: the understanding and general principles of use of a vacuum line. In parallel, while procedural knowledge will likely involve details about specific actions needed when conducting the experimental procedure, it will also include other procedures, such as the routine task of assembling data and preparing a Clausius–Clapeyron plot to determine enthalpy. In the context of the laboratory experiment, this is routine and recurrent. We summarise the supportive and procedural information for this hypothetical experiment in Table 2.
Table 2 An example of what might be considered supportive information and what might be considered procedural information for a typical experiment involving measuring the enthalpy of vapourisation of a volatile liquid using a vacuum line (a typical experiment described by Iannone (2006))
Supportive information (information presented in advance of the laboratory) |
Procedural Information (information presented just in time in the laboratory by manual or in-laboratory guidance/prompts) |
• Information about vapour pressure, intermolecular forces |
• Information about specific steps regarding use of vacuum line |
• Information about temperature dependence |
• Information about number and range of measurements to take |
• Information about vacuum line and its suitability for this technique |
• Information about approaches to data analysis and prompts for plotting data to obtain relevant experimental outcomes |
• Information about general operating principles of vacuum line |
|
• Information about overall experimental design |
|
Indeed, one of the interesting and exciting aspects of the work recently reported by the Cole group was the distinction between video presenting underlying theory and video presenting data analysis (Schmidt-McCormack et al., 2017); the latter is an aspect of procedural knowledge that students will draw on “just-in-time” as they are completing their analysis, rather than in advance of this experiment. Student usage in this way reflects their desire to (knowingly or unknowingly) moderate their cognitive load, and refer to resources as and when they consider them to be useful. Work in our studies of students use and value of pre-laboratory resources mirrors these observations (Agustian and Seery, unreported).
A final note on this point; the careful work of Winberg and Berg urge caution in the simple distinction between preparatory activities relating to theory and those relating to procedures – one of their findings was that preparation involving simulation of overarching experimental aspects by means of simulation, resulted in increased student engagement in what might be considered the theoretical component, observed through questions about conceptual matters (Winberg and Berg, 2007).
3. Pre-laboratory activities within a framework for supporting complex learning
Having summarised the findings from a survey from chemistry education literature on the benefits of pre-laboratory activities (Part 1), and described the constructs of a framework for supporting learning in a complex environment (Part 2), we now move to the conclusion and primary purpose of this article: to detail particular guidelines for considering preparation for laboratory work that should be considered in preparing students for learning in the complex environment that is the teaching laboratory. We hope that these will act as useful prompts for those wishing to design preparatory activities in their own practice.
In their work on defining the framework for considering complex learning, van Merrënboer and co-authors outline an instructional approach called the four-component instructional design model (van Merriënboer et al., 2002, 2003). The four components are those discussed in part 2: (a) learning tasks, (b) supportive information, (c) procedural information, and (d) part-task practice. Because our focus here is on preparation for learning in this complex learning environment, we consider this model in light of the aspects we should consider in helping learners prepare for laboratory work, and in the process indicate what aspects should be left to learning within the laboratory itself. Thus, we arrive at the following five recommendations for those considering the design of pre-laboratory activities:
1. Pre-laboratory activities benefit learning in the laboratory
Our first recommendation addresses the question: should pre-laboratory activities be considered as part of the curriculum design for laboratory work? Our answer is an emphatic agreement; we aim to have demonstrated with our survey of the literature that there is a substantial body of support for incorporating pre-laboratory work. Furthermore, we aim to have explained by means of a theoretical framework for complex learning, why pre-laboratory work has a positive impact on learning, considering in particular the significant cognitive load demands of practical work. We have surveyed various formats of pre-laboratory activities (Fig. 1) including video, simulation, quizzes and pre-laboratory discussion, but cannot make any conclusion as to the relative merits of any particular format, although particular advantages for given scenarios are highlighted below.
2. Pre-laboratory activities should be embedded into the overall laboratory learning process
A pre-laboratory activity should be part of the overall laboratory experience. We have identified two primary reasons for this; one emerging from reports from practice, and the other from the framework guiding our recommendations. Regarding practice, there are reports that students will not engage with pre-laboratory activities if they do not consider them of value, and indeed students will need some form of external motivation to complete the work (Rollnick et al., 2001). Similar findings have been observed regarding preparation for lecture work (Seery, 2015). Within the construct of the framework on learning in a complex environment, it is noted that supporting materials not embedded in the learning process may not be accessed by learners who need them most until they are in a situation of difficulty. At this point, the learner will be tasked with dealing with the load of the difficulty they are facing, as well as the load of integrating the information from the support material. The combination leads to a high load and an unsatisfactory learning scenario. Examples of embedding pre-laboratory activities that offer useful templates include the work on iterative quizzes with individual feedback (Chittleborough et al., 2007) and the work on advance preparation, and preparation incorporating discussion prior to laboratory work (Isom and Rowsey, 1986; Pickering, 1987; Domin, 2007). The attractiveness of the approach of considering laboratories within a flipped framework is that it makes the relationship between pre- and in-laboratory work explicit; one naturally reliant on the other (Teo et al., 2014). It follows that all of those involved in laboratory teaching (for example staff in the laboratory, teaching assistants/demonstrators) should be aware of what pre-laboratory activities students were required to complete, so that they can build on these activities within the laboratory.
3. Pre-laboratory activities should focus on the whole task, drawing learners' attention to overall strategy and approaches
Within the framework for complex learning, it is recommended that supporting learners by means of scaffolding is beneficial, but that such support should present learners with an overview of the whole task, rather than getting them to practice or prepare specific components of a task. This is advocated because, in complex scenarios, drawing together various tasks is difficult. In terms of preparing for laboratory learning, learners should be presented with the overall task that is required of them, but this presentation should be the simplest possible representation of this task, devoid of additional complexities or additional knowledge requirements necessary beyond the initial familiarisation needed. The purpose then is for learners to become familiar with the overall approach, so that as they become exposed to iterations or additional complexities within the laboratory itself, they will be able to draw upon their knowledge of the structure of the overall approach. In early work, Johnstone advocated the removal of “noise” from introductory laboratory instruction (Johnstone and Wham, 1982). It is likely that the use of simulations (e.g.Winberg and Berg, 2007) and video (e.g.Seery et al., 2017) offer the potential to provide learners with overall strategies.
4. Pre-laboratory activities should focus on supportive information
Much of the literature on pre-laboratory activity described above focuses on provision of information either relating to the underlying theory or information relating to experimental protocols. Instead of considering the nature of advance information in those terms, we advocate a more nuanced distinction deriving from the framework of learning in a complex learning environment: information that is supportive and information that is procedural. Supportive information relates to the information necessary to have an understanding of the whole laboratory task in general terms; a kind of theoretical framework in which the laboratory experiment operates. Such information may include information about the underlying theory, but may also include information about the rationale for particular experimental approaches in terms of how it fits within that theory; or indeed the reason a particular experimental approach is considered. Procedural information relates to the specific detail that is necessary to operate within the laboratory – procedural instructions that might best be provided by the laboratory manual or standard operating procedure, as well as the standard procedures for treatment and analysis of data. Work by Cole and co-workers in beginning to separate the identity of pre-laboratory work is an interesting direction in this context (Schmidt-McCormack et al., 2017).
5. Pre-laboratory activities should address the affective domain
While there is limited work on the formal study of the affective dimension of laboratory work, numerous reports demonstrate that an unintended outcome of introducing preparative activities is that students report their confidence and motivation about laboratory work has increased. Reports that formally consider student attitude point to the importance of developing strategies that address the affective domain (Keen-Rocha, 2008; Supasorn et al., 2008; Galloway and Bretz, 2016; Galloway et al., 2016). Because of the lack of literature in this regard, we draw from a wider set of guidelines regarding instructional design. Mayer has proposed a series of principles for e-resources, including three relating to the concept of fostering positive attitude towards e-resources (Mayer, 2017). The first is the personalisation principle, centring on the notion of generating a conversational rather than a formal style. Work done where resources have been student-generated (Jordan et al., 2015; Box et al., 2017) or where videos have been made in the setting learners will later use (Schmidt-McCormack et al., 2017; Seery et al., 2017) may be ways to incorporate this principle. The second principle is the voice principle, advocating the use of a human voice, rather than a computer-generated voice. The final principle is the embodiment principle, which advocates the presentation of diagrams and graphs as they form in real time instead of what they look like. The argument is that this mimics a real, social interaction, and may mean that for example, if experimental overviews are being presented, a video of a particular transformation or spectrum acquisition is shown in real time.
4. Limitations
The literature on pre-laboratory learning has been used to identify the general approaches reported to prepare students for learning in the laboratory. This work was considered in the context of a theoretical framework describing a complex learning environment, and the combination used to derive five principles that should be considered when designing pre-laboratory activities.
We are confident that these principles have a good grounding in the literature and will offer useful guidance to those wishing to design pre-laboratory activities in their own practice, or those involved in researching pre-laboratory learning. However, we do acknowledge that there may be some limitations with this discussion. These are presented below, along with some brief discussion as to how these limitations were considered within our framework.
The first limitation is that we have used a framework for learning in complex environments on the basis of the definition for complex learning proposed by the authors of that framework. While we considered other frameworks, the appeal of this one rested in the fact that, as well as finding resonance with the description of complex learning environments proposed, several authors writing about pre-laboratory work ground their rationale in cognitive load theory (e.g.Winberg and Berg, 2007). Therefore it seemed sensible to choose a framework that aligned with cognitive load theory for the purposes of using prior literature to generate some recommendations about laboratory work. However, we acknowledge that others have proposed alternative frameworks as rationales for their particular study. Self-regulated learning was proposed by Cole and co-workers, who describes the three phases of this framework: Forethought Phase, Performance Phase, and Self-Reflection Phase (Schmidt-McCormack et al., 2017) They propose that provision of pre-laboratory materials will scaffold students' focus during the forethought phase, in which learners should complete planning and thinking about learning how to do a task. Another framework proposed is Kolb's experiential learning theory (Abdulwahed and Nagy, 2009), in which the authors posit that pre-laboratory work aligns with the prehension phase of the experiential cycle, thus activating the transformation dimension. While these frameworks are clearly different, we contend that there is uniformity across these frameworks regarding the nature and relevance of activation. Because cognitive load theory subsequently invokes active strategies for managing learning in a complex environment, we opted to pursue the framework advocated here.
A second limitation is that in our discussion, there is little description about the nature of laboratory work being conducted, and hence being prepared for. Indeed there is an implicit assumption that the laboratories under consideration are expository laboratories. (For a discussion of laboratory instruction styles, see Domin (1999)). This is partly true, if only because most of the reports surveyed in the literature either made no attempt to differentiate the laboratory teaching style in their report from the assumed expository style or were for expository style laboratories. Therefore, a concern is that the value of our recommendations is limited to expository style laboratories. We have little data to counter this argument, but do make the following contention: two of the most common alternatives to expository laboratories are inquiry laboratories and problem-based laboratories, whose characteristics are also described by Domin (Domin, 1999). However, reports of examples of both inquiry (Szalay and Toth, 2016) and problem-based laboratories (McDonnell et al., 2007; Chopra et al., 2017) indicate that the environment before and in the laboratory is of even greater complexity than one finds in expository laboratories, and that students are not able to just rely on surface-level detail by following the recipes in the laboratory manuals, as characterised by Johnstone and Wham (1982). Therefore the need to assist preparing learners for this environment of increased complexity is even more pressing than it may be for expository laboratories. Indeed one of the outcomes noted upon introducing problem-based approach was that learners emphasis shifted from procedural knowledge to having to absorb responsibility for the approaches taken in laboratory time (Chopra et al., 2017), which echoes with the distinctions between supportive and procedural information discussed above.
Finally, one might query what extent of preparation is feasible. Given the complexity of laboratory learning, particularly in cases where laboratories are running for advanced courses and are perhaps out of sync with corresponding lecture courses (Schmidt-McCormack et al., 2017), it is necessary to question how much preparation can be feasibly achieved by students in the time available. This is a complex issue, requiring consideration of prior knowledge and curriculum design, and one that needs much more research. However the reports on preparatory activity for advanced laboratories do indicate benefit, and as with the discussion above, in these situations the cognitive load is likely to be higher than the generic case considered, thus making the requirements of preparation all the more necessary.
5. Conclusions
We have drawn together literature reports of pre-laboratory activities in chemistry in higher education and a framework for learning in complex environments to generate some recommendations for the design of pre-laboratory activities consistent with cognitive load theory. While we do not have data to advocate particular strategies as to how these activities might manifest in practice, we intend that our guidelines will be applicable for whatever type of strategy (e.g. video, simulation, discussion) is used in a particular scenario. We consider that there is a need for a renewed emphasis for inclusion of pre-laboratory activities within the overall framework of laboratory learning, and encourage those responsible for laboratory work in undergraduate curricula to incorporate these activities. In this regard, we hope that our guidelines prove to be of value.
Conflicts of interest
There are no conflicts to declare.
Notes and references
- Abdulwahed M. and Nagy Z. K., (2009), Applying Kolb's experiential learning cycle for laboratory education, J. Eng. Educ., 98(3), 283–294.
- Abdulwahed M. and Nagy Z. K., (2011), The TriLab, a novel ICT-based triple access mode laboratory education model, Comput. Educ., 56, 262–274.
- ACS, (2015), Undergraduate Professional Education in Chemistry: ACS Guidelines and Evaluation Procedures for Bachelor's Degree Programs, Washington: American Chemical Society.
- Alaimo P. J., Langenhan J. M., Tanner M. J. and Ferrenberg S. M., (2010), Safety teams: an approach to engage students in laboratory safety, J. Chem. Educ., 87(8), 856–861.
- Anderson R. O., (1976), The experience of science: a new perspective for laboratory teaching. New York: Teachers College Press, Columbia University.
- Barrie S. C., Bucat R. B., Buntine M. A., Burke da Silva K., Crisp G. T., George A. V., Pyke S. M., et al., (2015), Development, evaluation and use of a student experience survey in undergraduate science laboratories: the advancing science by enhancing learning in the laboratory student laboratory learning experience survey, Int. J. Sci. Educ., 37(11), 1795–1814.
- Beasley W. F., (1979), The effect of physical and mental practice of psychomotor skills on chemistry student laboratory performance, J. Res. Sci. Teach., 16(5), 473–479.
- Beasley W. F., (1985), Improving student laboratory performance: How much practice makes perfect? Sci. Educ., 69(4), 567–576.
- Beasley W. F. and Heikkinen H. W., (1983), Mental practice as a technique to improve laboratory skill development, J. Chem. Educ., 60(6), 488.
- Boud D., Dunn J. and Hegarty-Hazel E., (1986), Teaching in laboratories, Surrey, UK: Society for Research into Higher Education & NFER-Nelson Guildford.
- Box M. C., Dunnagan C. L., Hirsh L. A. S., Cherry C. R., Christianson K. A., Gibson R. J., Gallardo-Williams M. T., et al., (2017), Qualitative and Quantitative Evaluation of Three Types of Student-Generated Videos as Instructional Support in Organic Chemistry Laboratories, J. Chem. Educ., 94(2), 164–170.
- Burewicz A. and Miranowicz N., (2006), Effectiveness of multimedia laboratory instruction, Chem. Educ. Res. Pract., 7(1), 1–12.
- Chaytor J. L., Al Mughalaq M. and Butler H., (2017), Development and Use of Online Prelaboratory Activities in Organic Chemistry To Improve Students' Laboratory Experience, J. Chem. Educ., 94(7), 859–866.
- Chittleborough G. D., Mocerino M. and Treagust D. F., (2007), Achieving greater feedback and flexibility using online pre-laboratory exercises with non-major chemistry students. J. Chem. Educ., 84(5), 884–888.
- Chopra I., O'Connor J., Pancho R., Chrzanowski M. and Sandi-Urena S., (2017), Reform in a general chemistry laboratory: how do students experience change in the instructional approach? Chem. Educ. Res. Pract., 18(1), 113–126.
- Davidowitz B., Rollnick M. and Fakudze C., (2003), Increasing the Signal to Noise Ratio in a Chemistry Laboratory-Improving a Practical for Academic Development Students, S. Afr. J. Chem., 56, 47–53.
- DeMeo S., (2001), Teaching Chemical Technique. A Review of the Literature, J. Chem. Educ., 78(3), 373–379.
- Domin D. S., (1999), A review of laboratory instruction styles, J. Chem. Educ., 76(4), 543–547.
- Domin D. S., (2007), Students' perceptions of when conceptual development occurs during laboratory instruction, Chem. Educ. Res. Pract., 8(2), 140–152.
- Fine L. W., Harpp D. N., Krakower E. and Snyder J. P., (1977), Lap-dissolve slides: multiple-use formats for pre-laboratory instruction, J. Chem. Educ., 54(2), 72–74.
- Fung F. M., (2015), Using first-person perspective filming techniques for a chemistry laboratory demonstration to facilitate a flipped pre-lab, J. Chem. Educ., 92(9), 1518–1521.
- Galloway K. R. and Bretz S. L., (2016), Video episodes and action cameras in the undergraduate chemistry laboratory: eliciting student perceptions of meaningful learning, Chem. Educ. Res. Pract., 17(1), 139–155.
- Galloway K. R., Malakpa Z. and Bretz S. L., (2016), Investigating affective experiences in the undergraduate chemistry laboratory: students' perceptions of control and responsibility, J. Chem. Educ., 93(2), 227–238.
- Gammon S. D. and Hutchinson S. G., (2001), Using the internet to individualize laboratory questions, J. Chem. Educ., 78(3), 412–413.
- Gryczka P., Klementowicz E., Sharrock C., Maxfield M. and Montclare J. K., (2016), LabLessons: Effects of Electronic Prelabs on Student Engagement and Performance, J. Chem. Educ., 93(12), 2012–2017.
- Hendry G., (2013), Integrating feedback with classroom teaching, in Merry S., Price M., Carless D. and Taras M. (ed.), Reconceptualising Feedback in Higher Education: Developing Dialogue with Students, Routledge, pp. 133–134.
- Hensiek S., DeKorver B. K., Harwood C. J., Fish J., O'Shea K. and Towns M., (2016), Improving and Assessing Student Hands-On Laboratory Skills through Digital Badging, J. Chem. Educ., 93(11), 1847–1854.
- Hofstein A. and Lunetta V. N., (2004), The laboratory in science education: Foundations for the twenty-first century, Sci. Educ., 88(1), 28–54.
- Iannone M., (2006), Vapor pressure measurements in a closed system, J. Chem. Educ., 83(1), 97–98.
- Isom F. S. and Rowsey R. E., (1986), The effect of a new prelaboratory procedure on students' achievement in chemistry, J. Res. Sci. Teach., 23(3), 231–235.
- Johnstone A. H. and Al-Shuaili A., (2001), Learning in the laboratory; some thoughts from the literature, Univ. Chem. Educ., 5(2), 42–51.
- Johnstone A. H. and Wham A. J. B., (1979), A model for undergraduate practical work, Educ. Chem., 16(1), 16–17.
- Johnstone A. H. and Wham A. J. B., (1982), The demands of practical work, Educ. Chem., 19(3), 71–73.
- Johnstone A. H., Sleet R. J. and Vianna J. F., (1994), An information processing model of learning: its application to an undergraduate laboratory course in chemistry, Stud. High. Educ., 19(1), 77–87.
- Jolley D. F., Wilson S. R., Kelso C., O'Brien G. and Mason C. E., (2016), Analytical Thinking, Analytical Action: Using Prelab Video Demonstrations and e-Quizzes To Improve Undergraduate Preparedness for Analytical Chemistry Practical Classes, J. Chem. Educ., 93(11), 1855–1862.
- Jordan J. T., Box M. C., Eguren K. E., Parker T. A., Saraldi-Gallardo V. M., Wolfe M. I. and Gallardo-Williams M. T., (2015), Effectiveness of student-generated video as a teaching tool for an instrumental technique in the organic chemistry laboratory, J. Chem. Educ., 93(1), 141–145.
- Keen-Rocha L. S., (2008), Personal epistemological growth in a college chemistry laboratory environment, (PhD), University of South Florida. Retrieved from http://scholarcommons.usf.edu/etd/327.
- Kempa R. and Palmer C., (1974),
The Effectiveness of Video-tape Recorded Demonstrations in the Learning of Manipulative Skills in Practical Chemistry, Br. J. Educ. Technol., 5(1), 62–71.
- Kirk M. K. and Layman J. W., (1996), A pre-lab guide for general chemistry: improving student understanding of chemical concepts and processes. Paper presented at the 69th Annual Meeting of the National Association for Research in Science, St Louis.
- Kirschner P. A., (1992), Epistemology, practical work and academic skills in science education, Sci. Educ., 1(3), 273–299.
- Koehler B. P. and Orvis J. N., (2003), Internet-based prelaboratory tutorials and computer-based probes in general chemistry, J. Chem. Educ., 80(6), 606–608.
- Kolk K. V. D., Beldman G., Hartog R. and Gruppen H., (2012), Students Using a Novel Web-Based Laboratory Class Support System: A Case Study in Food Chemistry Education, J. Chem. Educ., 89(1), 103–108.
- Kolodny N. H. and Bayly R., (1983), Enhancing the laboratory experience in introductory chemistry with Apple-based pre-lab quizzes, J. Chem. Educ., 60(10), 896–897.
- Limniou M., Papadopoulos N., Giannakoudakis A., Roberts D. and Otto O., (2007), The integration of a viscosity simulator in a chemistry laboratory, Chem. Educ. Res. Pract., 8(2), 220–231.
- Limniou M., Papadopoulos N. and Whitehead C., (2009), Integration of simulation into pre-laboratory chemical course: computer cluster versus WebCT, Comput. Educ., 52(1), 45–52.
- Marcus N., Cooper M. and Sweller J., (1996), Understanding instructions, J. Educ. Psychol., 88(1), 49–63.
- Mayer R., (2017), Using multimedia for e-learning, J. Comput. Assist. Learn. DOI:10.1111/jcal.12197.
- McDonnell C., O'Connor C. and Seery M. K., (2007), Developing practical chemistry skills by means of student-driven problem based learning mini-projects, Chem. Educ. Res. Pract., 8(2), 130–139.
- McKelvy G. M., (2000), Preparing for the chemistry laboratory: an internet presentation and assessment tool, Univ. Chem. Educ., 4(2), 46–49.
- McNaught C., Grant H., Fritze P., McTigue P., Barton J. and Prosser R., (1993), Improving quantitative volumetric analysis skills in first year university chemistry courses, Res. Sci. Educ., 23(1), 189–198.
- Meester M. A. M. and Maskill R., (1993), The practical side of chemistry, Educ. Chem., 30(6), 156–159.
- Meester M. A. M. and Maskill R., (1995), First-year chemistry practicals at universities in England and Wales: organizational and teaching aspects, Int. J. Sci. Educ., 17(6), 705–719.
- Merritt M. V., Schneider M. J. and Darlington J. A., (1993), Experimental design in the general chemistry laboratory, J. Chem. Educ., 70(8), 660–662.
- Miller G. J., Heideman S. A. and Greenbowe T. J., (2000), Introducing proper chemical hygiene and safety in the general chemistry curriculum, J. Chem. Educ., 77(9), 1185.
- Moore C., Smith S. and Avner R. A., (1980), Facilitation of laboratory performance through CAI, J. Chem. Educ., 57(3), 196–198.
- Nadelson L. S., Scaggs J., Sheffield C. and McDougal O. M., (2015), Integration of video-based demonstrations to prepare students for the organic chemistry laboratory, J. Sci. Educ. Technol., 24(4), 476–483.
- Neerinck D. and Palmer C., (1977), The Effective of Video-Taped Recorder Demonstrations in the Learning of Manipulative Skills in Practical Chemistry: Part II, Br. J. Educ. Technol., 8(2), 124–131.
- Nicholls B. S., (1998), Post-laboratory support using dedicated courseware, Univ. Chem. Educ., 2(1), 10–15.
- Nichols B. S., (1999), Pre-laboratory support using dedicated software, Univ. Chem. Educ., 3(1), 22–27.
- Obenland C. A., Kincaid K. and Hutchinson J. S., (2014), A general chemistry laboratory course designed for student discussion, J. Chem. Educ., 91(9), 1446–1450.
- Pickering M., (1987), What goes on in students' heads in lab? J. Chem. Educ., 64(6), 521–523.
- Pogačnik L. and Cigić B., (2006), How To Motivate Students To Study before They Enter the Lab, J. Chem. Educ., 83(7), 1094–1098.
- Polles J. S., (2006), The Chemistry Teaching Laboratory: The Student Perspective, PhD thesis, Purdue University.
- Powell C. B. and Mason D. S., (2013), Effectiveness of podcasts delivered on mobile devices as a support for student learning during general chemistry laboratories, J. Sci. Educ. Technol., 22(2), 148–170.
- Reid N. and Shah I., (2007), The role of laboratory work in university chemistry, Chem. Educ. Res. Pract., 8(2), 172–185.
- Rollnick M., Zwane S., Staskun M., Lotz S. and Green G., (2001), Improving pre-laboratory preparation of first year university chemistry students, Int. J. Sci. Educ., 23(10), 1053–1071.
- Rosenshine B. V. and Meister C., (1992), The use of scaffolds for teaching less structured cognitive tasks, Educ. Leadership, 49(7), 26–33.
- RSC, (2015), Accreditation of Degree Programmes, Cambridge: Royal Society of Chemistry.
- Sadler D. R., (1989), Formative assessment and the design of instructional systems, Instruct. Sci., 18(2), 119–144.
- Schmidt-McCormack J. A., Muniz M. N., Keuter E. C., Shaw S. K. and Cole R. S., (2017), Design and Implementation of Instructional Videos for Upper-Division Undergraduate Laboratory Courses, Chem. Educ. Res. Pract. 10.1039/C7RP00078B.
- Seery M. K., (2015), Flipped learning in higher education chemistry: emerging trends and potential directions, Chem. Educ. Res. Pract., 16(4), 758–768.
- Seery M. K., Agustian H. Y., Doidge E. D., Kucharski M. M., O'Connor H. M. and Price A., (2017), Developing laboratory skills by incorporating peer-review and digital badges, Chem. Educ. Res. Pract., 18(3), 403–419.
- Simpson P., (1973), Videotapes in laboratory teaching, Educ. Chem., 10(5), 174–175.
- Smith J., (1987), Student preparation for prelab sessions, J. Chem. Educ., 64(7), 621–622.
- Starkey R. and Kieper D., (1983), A Computer-based Pre-laboratory Quiz for Organic Chemistry, J. Chem. Educ., 60(10), 897–898.
- Supasorn S., Suits J. P., Jones L. L. and Vibuljan S., (2008), Impact of a pre-laboratory organic-extraction simulation on comprehension and attitudes of undergraduate chemistry students, Chem. Educ. Res. Pract., 9(2), 169–181.
- Sweller J., Ayres P. and Kalyuga S., (2011), Cognitive load theory, vol. 1, Springer.
- Szalay L. and Toth Z., (2016), An inquiry-based approach of traditional ‘step-by-step’ experiments, Chem. Educ. Res. Pract., 17(4), 923–961.
- Teo T. W., Tan K. C. D., Yan Y. K., Teo Y. C. and Yeo L. W., (2014), How flip teaching supports undergraduate chemistry laboratory learning, Chem. Educ. Res. Pract., 15(4), 550–567.
- Towns M., Harwood C. J., Robertshaw M. B., Fish J. and O'Shea K., (2015), The Digital Pipetting Badge: A Method To Improve Student Hands-On Laboratory Skills, J. Chem. Educ., 92(12), 2038–2044.
- van Merriënboer J. J. G., Clark R. E. and de Croock M. B. M., (2002), Blueprints for complex learning: the 4C/ID*-model, Educ. Technol., Res. Dev., 50(2), 39–64.
- van Merriënboer J. J. G., Kirschner P. A. and Kester L., (2003), Taking the load off a learner's mind: instructional design for complex learning, Educ. Psychol., 38(1), 5–13.
- Walters A. U., Lawrence W. and Jalsa N. K., (2017), Chemical laboratory safety awareness, attitudes and practices of tertiary students, Safety Sci., 96, 161–171.
- Watson J. R., (1977), Videotapes in undergraduate chemistry laboratories, Educ. Chem., 14(3), 84–86.
- Weibel J. D., (2016), Working towards a paperless undergraduate physical chemistry teaching laboratory, J. Chem. Educ., 93(4), 781–784.
- Winberg T. M. and Berg C. A. R., (2007), Students' cognitive focus during a chemistry laboratory exercise: effects of a computer-simulated prelab, J. Res. Sci. Teach., 44(8), 1108–1133.
- Woolnough B. E. and Allsop T., (1985), Practical work in science, Cambridge University Press.
|
This journal is © The Royal Society of Chemistry 2017 |