DOI:
10.1039/C4IB00173G
(Perspective)
Integr. Biol., 2015,
7, 24-52
The simultaneous occurrence of both hypercoagulability and hypofibrinolysis in blood and serum during systemic inflammation, and the roles of iron and fibrin(ogen)
Received
21st July 2014
, Accepted 13th October 2014
First published on 14th October 2014
Abstract
Although the two phenomena are usually studied separately, we summarise a considerable body of literature to the effect that a great many diseases involve (or are accompanied by) both an increased tendency for blood to clot (hypercoagulability) and the resistance of the clots so formed (hypofibrinolysis) to the typical, ‘healthy’ or physiological lysis. We concentrate here on the terminal stages of fibrin formation from fibrinogen, as catalysed by thrombin. Hypercoagulability goes hand in hand with inflammation, and is strongly influenced by the fibrinogen concentration (and vice versa); this can be mediated via interleukin-6. Poorly liganded iron is a significant feature of inflammatory diseases, and hypofibrinolysis may change as a result of changes in the structure and morphology of the clot, which may be mimicked in vitro, and may be caused in vivo, by the presence of unliganded iron interacting with fibrin(ogen) during clot formation. Many of these phenomena are probably caused by electrostatic changes in the iron–fibrinogen system, though hydroxyl radical (OH˙) formation can also contribute under both acute and (more especially) chronic conditions. Many substances are known to affect the nature of fibrin polymerised from fibrinogen, such that this might be seen as a kind of bellwether for human or plasma health. Overall, our analysis demonstrates the commonalities underpinning a variety of pathologies as seen in both hypercoagulability and hypofibrinolysis, and offers opportunities for both diagnostics and therapies.
Insight, innovation, integration
The Biological Insight of this manuscript is that although they are usually studied separately (and thus typically described in separate papers) there is a considerable body of literature showing that both hypercoagulability and hypofibrinolysis are present in a large number of inflammatory, vascular diseases. This implies that they share common causes. Similarly, although these names reflect the changed kinetics of clot formation and lysis, there is also considerable evidence that the resistance to fibrinolysis comes because the nature (make-up and morphology) of the clot differs in these states. One established cause comes from the interaction of unliganded iron with fibrinogen. The Technological Innovation is the use of advanced microscopy techniques (including atomic force microscopy) to measure these changes. The Benefit of Integration comes (i) from bringing together these two separate readouts with a suggested major cause, viz. iron dysregulation, and (ii) by showing their commonality across a range of inflammatory vascular diseases we demonstrate this as Integrative Biology.
|
Introduction
Although the formation of blood clots and their subsequent removal are typical responses to the occurrence of damage in blood vessels,1,2 and this may also be part of the innate immune system,3–7 a common cause of thrombosis or thromboembolism (the formation of a blood clot that obstructs blood flow) is an excessive tendency toward blood clotting known8 as hypercoagulability.
Thromboembolic diseases are caused when a blood vessel is obstructed by a blood clot (embolus), whether formed locally or by one that has been carried in the bloodstream from the site of its formation. Thromboembolic diseases include both venous thromboembolism and arterial thrombosis, and these are treated differently (both intellectually and therapeutically) (e.g.ref. 9–13).
Thromboembolic events are responsible for approximately 80% of human strokes,14 specifically a type of stroke caused by the interruption of blood flow to a part of the brain due to the slow formation of a blood clot along the lumen of an artery. Cryptogenic ischaemic strokes are now thought to comprise about 25% of all ischaemic strokes and there is evidence that most cryptogenic strokes are thromboembolic.15 The thrombus is thought to originate from any of several well-established potential embolic sources, including minor-risk or covert cardiac sources, veins via paradoxical embolism, and non-occlusive atherosclerotic plaques in the aortic arch, cervical, or cerebral arteries.15 However, the most significant cause of thromboembolic stroke is considered to be due to a complication of atrial fibrillation (fibrillation of the muscles of the atria of the heart causing arrhythmia).16,17
Sudden occlusion of a cerebral blood vessel by a thrombus or embolism initiates a complex process of events that include excitotoxicity, oxidative stress, microvascular injury, blood brain barrier dysfunction and postischemic inflammation that ultimately leads to cell death.18 Also, most pertinently, thromboembolic events are associated with a hypercoagulable state.19,20
A great many ‘risk’ factors (including both genetic21–28 and environmental or ‘lifestyle’, as well as those accompanying medical interventions29–32) can contribute to this hypercoagulability,33–38 and understanding these (Fig. 1) and how they interact probably makes this a problem that is thus best considered using the tools and ideas (e.g.ref. 39–43) of systems biology. While many diseases of haemostasis also involve a tendency to haemorrhage and/or inadequate clot formation, our focus will be on those coagulopathies where the rate and/or nature of the clot formation are greater, and its relative resistance to fibrinolysis is less, than optimal. An overview of the manuscript is given in Fig. 2.
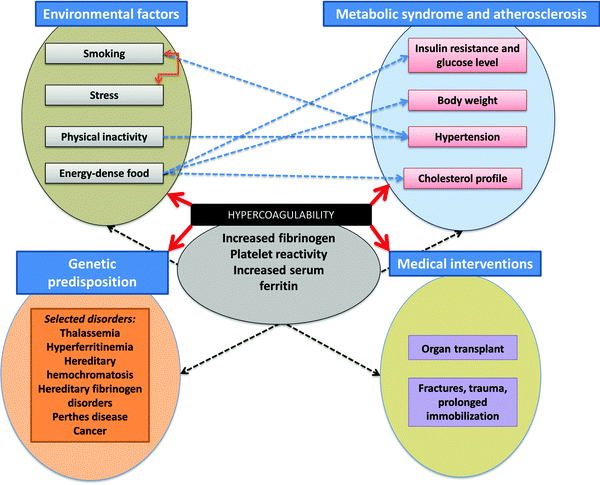 |
| Fig. 1 Some risk factors, associated with hypercoagulability. These include environmental factors, genetic predisposition, some lifestyle factors that an individual may have control over, potentially causing metabolic syndrome and atherosclerosis, as well as medical interventions. | |
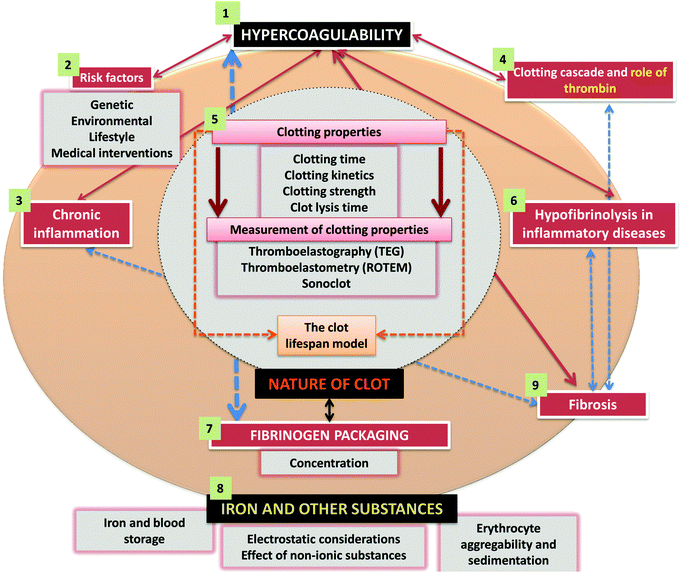 |
| Fig. 2 An overview of the manuscript. The first focus of the manuscript is hypercoagulability (1). We discuss risk factors (2) and chronic inflammation (3), and how the clotting cascade (4) is changed during hypercoagulability. We also focus on clotting properties that are measurable using the current leading methods of thromboelastography (TEG), thromboelastometry (ROTEM) and Sonoclot technology (5). We show how this provides insight into hypofibrinolysis associated with inflammation (6) and how fibrinogen packaging (7) is changed during inflammation. Here we also discuss various substances and molecules (8) that may influence clot structure and also the relationship between hypercoagulability and fibrosis (9). | |
Relationship between chronic inflammation and hypercoagulability
While a precise definition of chronic inflammation is slightly elusive, and is usually based around the observation of changes in various (‘inflammatory’) cytokines (e.g.ref. 44–46), there is abundant evidence that one of its hallmarks (or at least an extremely common co-existence in many circumstances) is a hypercoagulable state of some kind (e.g.ref. 10, 47–79).
Consequently, as we shall see, the long lists of inflammatory diseases that are additionally associated with iron dysregulation (e.g.ref. 80–85) are also those in which a hypercoagulable and/or hypofibrinolytic state may be observed.
The healthy clotting cascade, mainly focussing on the thrombin pathway and on factor XIII crosslinking
During healthy (normal) blood clotting, there are both ‘intrinsic’ and ‘extrinsic’ pathways (reviewed e.g. in ref. 86–88), but both converge on terminal steps in which prothrombin is converted to thrombin followed by the thrombin-catalysed conversion of individual molecules of the soluble protein fibrinogen, with the release of two fibrinopeptides, to an insoluble, multimolecular complex of these molecules in the form of fibrin, the chief component of the blood clot. Thrombin also activates factor XIII to produce factor XIIIa, which catalyses intermolecular crosslinks between fibrin fibrils to stabilise the clot so formed (e.g.ref. 89–91).
A useful series of systems biology clotting models has been produced by Duffull and colleagues (e.g.ref. 92–96). Those of Wajima et al.93 are available at the Biomodels database,97–99 and are most easily accessed in SBML100,101 form via the useful summary and commentary produced by Michael Schubert at http://www.ebi.ac.uk/biomodels-main/static-pages.do?page=ModelMonth%2F2011-07. Biomodels 338, 339 and 340 pertain at the Biomodels database. Biomodel 338 mimics the extrinsic pathway, where deficiencies affect the prothrombin time (PT), while Biomodel 339 includes the intrinsic pathway that allows simulation and calculations that mimic the results of the activated partial thromboplastin test (aPTT). (Biomodel 340 is concerned with the effects of warfarin.)
Although most modern models of coagulation incorporate the role of cells,1,87,102,103 we shall concentrate here on the stripped-down terminal elements of the extrinsic (and common) pathway, where thrombin plays a crucial role in initiating the polymerisation of fibrinogen. These terminal processes, that are our focus here, are shown in Fig. 3, along with the normal (as seen in healthy individuals) degradation pathway of the fibrin via (fibrino)lysis catalysed by plasmin formed from the tissue plasminogen activator-catalysed activation of plasminogen.
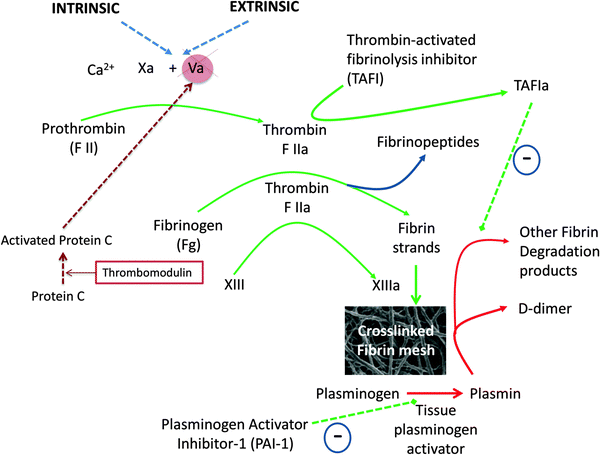 |
| Fig. 3 The latter stages of the various coagulation pathways, showing places where thrombin plays a crucial role in initiating the polymerisation of fibrinogen. | |
The viscoelastic measurement of clotting properties ex vivo: thromboelastography, thromboelastometry, Sonoclot and others
The importance of understanding clot formation and degradation has led to the development of a considerable variety of assays for these processes (e.g.ref. 104–108). Most of these methods are either optical or rheological/viscoelastometric in nature, and a number of commercial instruments exist. Some use plasma rather than whole blood, but plasma-based coagulation tests like prothrombin time (PT) and activated partial thromboplastin time (aPTT) have been seen as not entirely appropriate for monitoring coagulopathies,109 with a move to analyse whole blood and using methods that determine the viscoelastic properties directly. Nonetheless, PT and aPTT are pre-eminent in the clinic.
Viscoelastic haemostatic methods110 include thromboelastography (TEG),109,111–114 thromboelastometry (ROTEM)112,114–116 and the Sonoclot117–119 instruments. Some comparisons have been made,106,109,112–114,120–124 to the effect that results can be reasonably comparable in some circumstances but for detailed studies of specific effects it is probably wise to standardise on a particular instrument or technique.
Thromboelastography, thromboelastometry and Sonoclot instruments
In a typical thromboelastograph (a modern version is the Haemoscope) or rotational thermoelastometer (ROTEM), clotting is initiated and a trace generated where the ordinate represents the viscosity and the abscissa time. The differences are mainly as to whether a pin or the cup containing the blood is what is moved, and terminologies differ slightly according to Table 1 (based on ref. 106). Fig. 4 shows a schematic representation of a typical TEG and ROTEM readout (A), as well as viscoelastic haemostatic assay (VHA) tracings of a typical healthy trace (B) versus hypercoagulability (C), hypocoagulability (D), hyperfibrinolysis (E) and hypofibrinolysis (F).
Table 1 Terminologies of the variables measured in the TEG, ROTEM and Sonoclot instruments. Based on ref. 106, 109 and 125
Parameter |
TEG |
ROTEM |
Sonoclot |
Clot time
|
Period to 2 mm amplitude |
R (reaction time, min) |
CT (clotting time, s) |
ACT (activated clotting time, s) |
|
Clot kinetics
|
Period from 2–20 mm amplitude |
K (kinetics) |
CFT (clot formation time) |
CR (clot rate, U min−1) |
α (slope between R and K) |
α (slope of tangent at 2 mm amplitude) |
TP (time to peak, min) |
|
Clot strength
|
Maximum strength |
MA (max amplitude) |
MCF (max clot firmness) |
PA (peak amplitude, U) |
Clot elasticity |
G |
MCE (max clot elasticity) |
|
Clot lysis
|
Lysis (at fixed time) |
Ly30, Ly60 (amplitude reduction 3/60 min after MA) |
CL30, CL60 (amplitude reduction 30/60 min after MCF) |
|
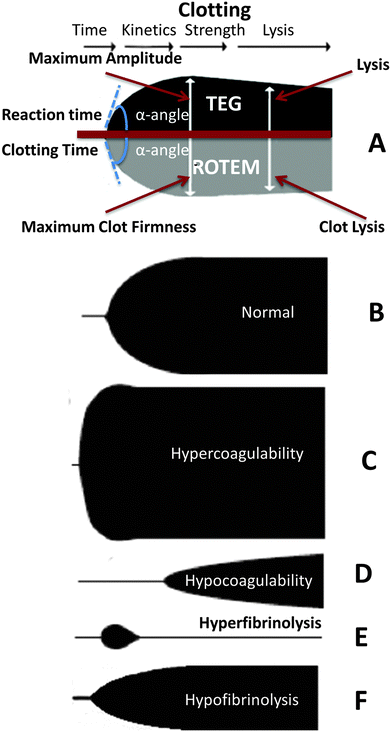 |
| Fig. 4 (A) Diagram comparing thromboelastography (TEG) with thromboelastometry (ROTEM), showing clotting/reaction parameters, including time, kinetics, strength and lysis of the clot. (B to F) Schematic representation of various viscoelastic haemostatic assay (VHA) tracings: (B) normal (healthy); (C) hypercoagulability; (D) hypocoagulability; (E) hyperfibrinolysis; and hypofibrinolysis (F). This diagram is based on a version in ref. 109. | |
A slightly different principle is embodied in the Sonoclot instruments, in that the viscoelastic probe vibrates vertically,118 and the shape of the trace is somewhat different, producing a ‘signature’ with various peaks106,125–127 (Fig. 5).
We compare typical reference values for clotting time, clot kinetics and clot strength for the TEG, ROTEM and Sonoclot in Table 2.
Table 2 Typical references values for clotting parameters of healthy blood and plasma as assessed using three different instruments. Based on ref. 106, 125 and 128. Note that the Sonoclot range varies considerably depending on whether activation includes glass beads
Parameter |
TEG |
ROTEM |
Sonoclot |
Clotting time |
Whole blood 4–8 min |
Citrated intrinsic 137–246 s, extrinsic 42–74 s |
137–353 s |
Citrated kaolin 3–8 min |
|
Clot kinetics |
Whole blood 1–4 min |
Citrated intrinsic 40–100 s, extrinsic 46–148 s |
CR 7.6–36 U min−1 |
Citrated kaolin 1–3 min |
TP 5.2–15.2 min |
|
Clot strengthening |
Whole blood α 47°–74° |
Citrated intrinsic 71°–82°, extrinsic 63°–81° |
|
Citrated kaolin α 55°–78° |
|
Maximum strength |
Whole blood 55–73 mm |
Citrated intrinsic 52–72 mm, extrinsic 49–71 mm |
PA 83–108 U |
Citrated kaolin 51–69 mm |
The clot lifespan model
A specific TEG assay that focuses rather more on the clot itself has been developed by Nielsen and his colleagues, and is known as the clot lifespan model129–132 (Fig. 6). It purposely combines a standardized clotting stimulus such as the tissue factor with a fibrinolytic stimulus such as the tissue type plasminogen activator to assess clot growth and disintegration via changes in clot resistance under the same incubation conditions. Its attraction from the present perspective is that it can assess both hypercoagulability and hypofibrinolysis in the same samples while admitting a reasonably swift assay time (∼30 min). In contrast to conventional TEG parameters, it also has the advantage of measuring the functionally relevant133,134 clot resistance directly.135
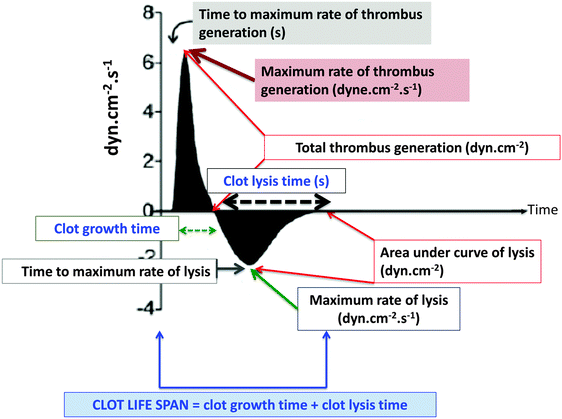 |
| Fig. 6 The clot lifespan model developed by Nielsen and colleagues.129–132 | |
We also note a number of approaches to the development of coagulometry based on the measurement of the passive electrical properties of the sample,136–142 a strategy that we ourselves have found useful,143 with radio-frequency measurements of cellular properties having a particular value.144–147 Finally, fibrin scatters light much more than does fibrinogen such that turbidimetry is often used to detect its formation (e.g.ref. 148–153), and a number of more specialist coagulometric instruments based on optical (e.g.ref. 154) or other155–161 properties have been proposed (see Table 2).
The relationship between hypercoagulability and hypofibrinolysis in selected inflammatory diseases
Hypercoagulability refers to the likelihood of or tendency towards making a clot, while hypofibrinolysis refers to a decreased tendency to remove it. Clearly either or both could be seen as coagulopathies. This said, and although they are usually studied separately, the common co-occurrence (e.g.Table 3 and ref. 162) of hypercoagulability and hypofibrinolysis can be interpreted to mean that both can have the same cause. Similarly, although the distinction (and their naming) reflects the changed kinetics of clot formation and lysis, there is also considerable evidence that the thermodynamics, i.e. properties related to the nature (make-up and morphology) of the clot differs in these states. (We note that sometimes fibrinolysis is assessed via D-dimer formation,163 but that when this is increased one cannot normally tell whether that is due to an increase in fibrinolysis or simply because there had previously been hypercoagulation.)
Table 3 The co-occurrence of hypercoagulation and hypofibrinolysis in some selected diseases
Disease |
Some references showing blood hypercoagulability |
Some references showing reduced clot permeability or decreased susceptibility of clot to (fibrino)lysis |
Alzheimer's disease |
58 and 164 (but contrast165) |
166–171
|
Cancer |
23, 172–186
|
23, 187 and 188
|
Cardiovascular disease |
72, 73, 79, 189–198
|
25, 162, 191, 192, 199–211
|
Chronic obstructive pulmonary disease |
212–214
|
215
|
Cushing's syndrome |
216–222
|
216, 217 and 220
|
Deep vein thrombosis |
7, 188, 223 and 224
|
162, 188, 223, 225 and 226
|
Diabetes mellitus, type 2 |
51, 227–233
|
34, 206, 228, 230, 232, 234–241
|
Heart failure |
61, 242 and 243
|
244
|
Inflammatory bowel disease |
59, 70, 245–252
|
246, 253–256
|
Metabolic syndrome |
257–260
|
25 and 258
|
Pre-eclampsia |
261–265
|
264 and 266
|
Pulmonary embolism |
153
|
188, 224 and 267
|
Retinal vein occlusion |
268
|
162, 268 and 269
|
Rheumatoid arthritis |
270–276
|
273 and 274
|
Sepsis |
277–284
|
277–280 and 284
|
Stroke |
56, 194, 195, 285–291
|
63, 152, 162, 292–299
|
Systemic lupus erythematosus |
300 and 301
|
266, 302 and 303
|
Thromboembolism |
304–307
|
307–310
|
Transient ischaemic attacks |
56, 290, 291 and 311
|
63 and 295
|
Trauma |
307, 312–319
|
315, 320–322
|
Note that in the case of β-thalassaemia there is a particularly noticeable amount of hypercoagulation323–335 but that fibrinolysis seems to be enhanced,324,336,337 albeit not by enough to overcome the hypercoagulation. The same seems to be true of psoriasis66,338–340 and of asthma.341–345 Interestingly, we have been unable to find any direct literature evidence for or against the role of hypercoagulability and/or hypofibrinolysis in the case of hereditary haemochromatosis, despite the fact that it is a well-known iron overload disease.346 DIC may be regarded as a contributor to a variety of syndromes, e.g. sepsis347 and multiple organ failure.78 There are also considerable changes in the coagulation variables during both healthy and compromised pregnancy.348–351 Finally, although we have listed the various diseases separately, there are a considerable number of comorbidities, such as IBD/cardiovascular events197 or chronic infection/multiple effects,352 as expected for multiple diseases with a broadly common cause.82
The nature of the clot and clot lysis time
In many ways, fibrinogen polymerisation following thrombin activation is a remarkable process (Fig. 7, redrawn below from ref. 208), initially involving the release of two fibrinopeptides that cause lateral and end-to-end interactions via ‘knobs’ and ‘holes’, ultimately strengthened via the inter-strand covalent cross-linkages introduced later by factor XIII. Our interest is focussed on what determines the sizes, and especially the diameter, of individual fibrin fibres, since these may be observed under the electron microscope (e.g.ref. 171, 294, 297, 345, 353–360 and see later), and indeed the optical microscope,361,362 directly. An individual fibrinogen molecule is an elongated coiled coil, with a length and diameter of approximately 45 and 5 nm, respectively.363,364 However, the diameter of ‘typical’ healthy fibrin fibres is of the other of 80–90 nm296,365 or more (as in Fig. 8). Thus to create every element of a fibre's width, even at the lower ‘normal’ diameter, requires the self-organisation of some 260 fibres (given that the formula for close-packing circles of diameter d to make, or fit into, a bigger circle of diameter D is N = 0.9069 × D2/d2). Our interest here lies in the recognition that variation in the kinetics or accretion (rate) of individual fibrinogen molecules and proto-fibrils changes not only the amount of a clot but the nature, diameter and architecture of the fibres of which it is formed, with considerable functional consequences for the performance (and ease of removal) of the clot itself. Thus in stroke, for instance, the fibrin fibres have an average (modal) diameter of some 35 nm,296i.e. less than one half of that of healthy fibrin, evidently involving less than one quarter of the fibrinogen molecules per unit length. It is obviously of interest to seek to understand what kinds of changes can effect such dramatic differences. In principle there can be at least three types: (i) changes in the amount of fibrinogen, (ii) changes in the amino acid sequence of fibrinogen, and (iii) changes in other substances in blood that interact with fibrinogen and affect its polymerisation. All three are known to occur.
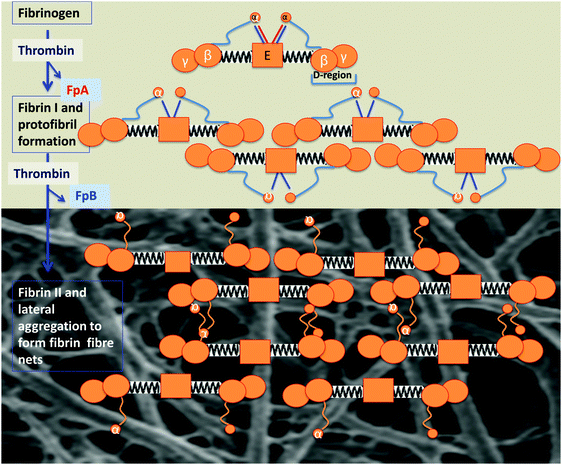 |
| Fig. 7 Fibrinogen polymerisation following thrombin activation, indicating how the molecular structure of the fibrinogen changes and self-assembles to form macroscopic fibrin fibre nets, following the removal of fibrinopeptides A and B under the action of thrombin. Fibrinogen consists of 2 Aα, 2 Bβ, and 2 γ-chains, with a central E-region and D-regions, which are connected with an E-region by a coiled coil segment, composed of the β- and γ-chain C-termini; the α-chain C-termini fold back on the coiled coil and interact with the E-region. Fibrinopeptides A and B are released by the action of thrombin, and this initiates the polymerization of the fibrin protofibrils (redrawn from ref. 208). | |
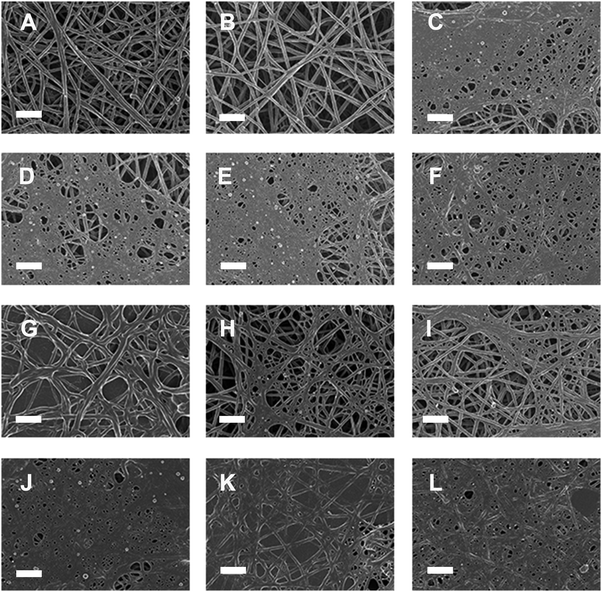 |
| Fig. 8 Representative micrographs of platelet rich plasma (PRP) from 6 healthy individuals whose iron levels (iron, transferrin, % saturation and serum ferritin) were within the healthy ranges. (A) PRP + thrombin; (B) PRP + thiourea + thrombin; (C) PRP + phytic acid + thrombin; (D) PRP + FeCl3 + thrombin; (E) PRP + AlCl3 + thrombin; (F) PRP + YCl3 + thrombin; (G) PRP + FeCl3 + thiourea + thrombin; (H) PRP + AlCl3 + thiourea + thrombin; (I) PRP + YCl3 + thiourea + thrombin; (J) PRP + FeCl3 + phytic acid + thrombin; (K) PRP + AlCl3 + phytic acid + thrombin; and (L) PRP + YCl3 + phytic acid + thrombin. Scale bar = 1 μm. See also Table 5 and text. | |
Effects of fibrinogen concentration on the nature of the clot
Fibrinogen itself is a well-known inflammatory marker, and as inflammatory markers, fibrinogen levels are themselves of course also associated with a variety of diseases (see e.g.ref. 88, 366–376), and fibrin clot properties also vary in this way with various diseases (e.g.ref. 191 and 377). The nature of the clot structure also depends on a complex interplay of entropic and enthalpic mechanisms accompanying structural changes and that underpin the nonlinear mechanical response in fibrin networks undergoing compressive deformation.378
On simple kinetic grounds alone, changes in the fibrinogen concentration might be expected to have significant effects on the nature of the clot, and this is indeed observed,34,113,126,238,292,379 with, for instance, higher fibrinogen concentrations leading to faster clotting times and hence148 smaller clot pores.149,380,381
In addition to changes in fibrinogen levels, one might suppose that changes in the fibrinogen molecule itself would have substantial effects, and this too is found. In one very nice study, a common variant BβArg448Lys in the C-terminal region of the fibrinogen beta-chain had major effects on the nature of the clot,192 and the fact that this was also true for recombinant protein implied that the changes were due to interactions with substances in the blood itself.
The role of iron ions and other substances in affecting fibrinogen and clot formation
Fibrin(ogen) and clot formation is affected by a plethora of circulating plasma molecules, including collagen, fibronectin, lipoprotein, albumin, thrombospondin, von Willebrand factor, fibulin, fibroblast growth factor-2, interleukin-1, and calcium, zinc and iron ions (see Table 4).382–395
Table 4 Selected plasma molecules that influence fibrin(ogen) and clot formation
Molecules |
Selected references |
Calcium ions bound both strongly and weakly to fibrin(ogen) have been localized, but this binding and interactions are only beginning to be discovered. |
380, 392, 398 and 399
|
Fibrinogen/fibrin can bind to native collagen type I (Col I) and may use the Col I fiber network as a base to provide a functional interface matrix that connects cells to the Col I. |
394
|
|
Fibroblast growth factor-2 (FGF-2) is a critical growth factor in normal and malignant cell proliferation and tumor-associated angiogenesis. Fibrinogen and fibrin bind to FGF-2 high affinity to fibrin(ogen) and modulate FGF-2 functions and this binding plays an important role in augmented angiogenesis. |
386 and 387
|
|
Fibronectin may also affect clot properties, as it binds extracellular matrix components such as collagen and fibrin, and sometimes serves as a general cell adhesion molecule. Along with fibrin, plasma fibronectin is deposited at the site of injury, forming a blood clot that stops bleeding and protects the underlying tissue. An increase in fibronectin concentration results in thinner and denser fibers in the fibrin matrices as it is covalently and non-covalently bound to fibrin matrices. |
393
|
|
Fibulins are a family of extracellular matrix and blood proteins presently having two members designated as fibulin-1 and -2 and fibulin-1 can bind to fibrinogen; it was also found to be thrombi associated with human atherectomy specimens. |
382
|
Similarly, the inflammatory mediator IL-1beta binds with high affinity to fibrin(ogen) and demonstrates increased activity in the bound form; and compared with free form, fibrinogen-bound IL-1beta stimulated increased activation of endothelial cell nuclear factor kappaB (NF-kappaB), monocyte chemo-attractant protein-1 (MCP-1) secretion, and nitric oxide (NO) synthesis. |
384
|
|
There is also evidence that elevated lipoprotein(a) (Lp(a)) levels are associated with dense fibrin clots, reduced clot permeability and prolonged lysis time. |
395
|
Low serum albumin was a modest marker of increased VTE risk. |
388 and 390
|
|
Thrombospondins are proteins with antiangiogenic abilities and is a major platelet glycoprotein, which is released from platelets during blood coagulation and co-polymerizes with fibrin during blood coagulation and may be an important modulator of the clot structure. |
400–402
|
|
von Willebrand factor-binding protein secreted by Staphylococcus aureus activates host prothrombin and form fibrin cables, thereby promoting the establishment of infectious lesions. |
391
|
|
Zinc ions bind to the negatively charged fibrinogen, decrease thrombin's activity, but accelerate fibrin polymerisation, forming larger fibres. |
383, 403–405
|
A number of clot properties can vary under the influence of various molecules mentioned in the previous paragraph. These molecules may interact with fibrinogen and change the mechanical properties of clots, which are essential to the effective function of fibrin. Clot properties that may vary include the pore size, fibrin diameter, rigidity, ease of fibrinolysis, and so on208,396 (and some of these are correlated361,397).
Important to this review is that fibrinogen directly recognizes iron ion, the PPIX ring and metal ions complexed with the hemin (iron–protoporphyrin IX: PPIX) ring.389
Electrostatic considerations
The pI of fibrinogen is 5.5, i.e. it is negatively charged at neutral pH (as are the surfaces of erythrocytes). As one might expect, changes in ionic strength decrease the clot pore size.149,380,381 As one would also expect from Debye–Hückel theory, it binds divalent cations such as Ca2+
380,392,398,399 and Zn2+
404; the trivalent iron ion is especially effective.389,406 It has also been suggested that added ferric iron can carry out Fenton chemistry by reacting with peroxide that may be formed in a variety of ways.171,353,359,407,408 Thus both electrostatic phenomena and hydroxyl radical formation may modify the fibrinogen structure in such a way that its kinetics of polymerisation, and the structure of the fibrin products, is altered substantially.
The action of phytic acid and thiourea in the presence of Fe3+, Al3+ and Y3+
Phytic acid (myoinositol hexaphosphate) has 12 exchangeable protons, giving it a strong ability to complex with multivalent cations409 (and indeed positively charged proteins). Trivalent ions are of special interest from an electrostatics point of view, and can be chosen to have very different chemistries and solvent interactions410 such that if their behaviour is similar it is likely their electrostatic rather than chemical properties that underpin this.411 Phytic acid, therefore, has the potential to remove metal ions such as Fe3+, Al3+ and Y3+ in the form of a metal–phytate complex, but not to serve as a hydroxyl radical trapper.412 By contrast, thiourea is not thought to be a metal chelator, but traps hydroxyl radicals (e.g.ref. 413). To this end, we studied the effects of these substances on fibrinogen polymerisation.
AlCl3, FeCl3 and YCl3 (final concentration 15 μM) were mixed with platelet rich plasma (PRP) from 6 healthy individuals prepared from blood drawn in citrate tubes. Full iron analysis showed that their basal iron levels (iron, serum ferritin transferrin and % saturation) were within the normal ranges as specified for healthy individuals. Thrombin with and without either phytic acid or thiourea (final concentrations 30 μM) was added to PRP (pH: 7.38 (physiological pH)). See Table 5 for sample preparation and the mean diameter in nm ± SD. Fibrin fibres were prepared for SEM according to methods published previously.359,360
Table 5 Sample preparation for and statistical analysis (to nearest nm) of Fig. 8. Where present, final concentrations of FeCl3, AlCl3 and YCl3 were 15 μM, and of thiourea and phytic acid 30 μM
Components and (figure letter in parentheses) |
Mean fibre diameter (nm) (n = 50) ± SD |
10 μL PRP + 5 μL thrombin (8A) |
105 ± 3 |
10 μL PRP + 5 μL thiourea + 5 μL thrombin (8B) |
117 ± 4 |
10 μL PRP + 5 μL phytic acid + 5 μL thrombin (8C) |
46 ± 2 |
10 μL PRP + 5 μL FeCl3 + 5 μL thrombin (8D) |
45 ± 3 |
10 μL PRP + 5 μL AlCl3 + 5 μL thiourea (8E) |
39 ± 1 |
10 μL PRP + 5 μL YCl3 + 5 μL thrombin (8F) |
50 ± 2 |
10 μL PRP + 5 μL FeCl3 + 5 μL thiourea + 5 μL thrombin (8G) |
146 ± 4 |
10 μL PRP + 5 μL AlCl3 + 5 μL thiourea + 5 μL thrombin (8H) |
66 ± 5 |
10 μL PRP + 5 μL YCl3 + 5 μL thiourea + 5 μL thrombin (8I) |
69 ± 5 |
10 μL PRP + 5 μL FeCl3 + 5 μL phytic acid + 5 μL thrombin (8J) |
47 ± 3 |
10 μL PRP + 5 μL AlCl3 + 5 μL phytic acid + 5 μL thrombin (8K) |
184 ± 8 |
10 μL PRP + 5 μL YCl3 + 5 μL phytic acid + 5 μL thrombin (8L) |
65 ± 4 |
SEM analysis of healthy fibrin fibre morphology (Fig. 8A) shows individual fibres that form a typical netlike structure. When thiourea is added to healthy PRP (Fig. 8B), no changes in fibrin fibre structure are noted; however, in the presence of phytic acid, instead of a healthy fibre structure, a dense netted layer (Fig. 8C) is formed. This netted appearance of the fibrin fibres was also noted with the addition of FeCl3, AlCl3 and YCl3 to healthy PRP (Fig. 8D to F) (final concentration of these substances in PRP was 15 μM), while the fibre diameters were approximately halved (as also seen in stroke296). When thiourea was added to a final concentration of 30 μM to PRP and FeCl3, AlCl3 and YCl3, respectively (Fig. 8G to I), the fibrin structure was either protected or reverted back to a morphology more similar to that of healthy PRP. When phytic acid was added to a final concentration of 30 μM to PRP and FeCl3, AlCl3 and YCl3, respectively, the fibrin structure changed to a thick, matted layer (Fig. 8J to L). This structure was denser than when only phytic acid was added to PRP (Fig. 8C) or when just FeCl3, AlCl3 or YCl3 were added to PRP (Fig. 8D to F).
The above observations suggest that thiourea added to PRP does not have an electrostatic effect (as would indeed be expected in that it is uncharged). However, the addition of phytic acid to PRP (Fig. 8C) and AlCl3 (Fig. 8E) might suggest a purely electrostatic effect of both excessive cations and anions. The effects of PRP with FeCl3 and thiourea (Fig. 8G) could be interpreted as showing that at least in part the changes are due to OH˙ – but that is on the basis of thiourea not chelating. This suggests that the structural changes in the fibres that take place when FeCl3 is added to PRP and thrombin (Fig. 8D) might be contingent upon both an electrostatic (mainly) and an OH˙ (partly) effect.
Interestingly, it seems as if thiourea also stops the effect that AlCl3 (Al3+) has on the structure of the fibres (Fig. 8H). Al3+ does not on its own effect Fenton chemistry; however, under conditions where there might be iron present (as will be the case in PRP of healthy individuals) it can actually contribute to Fenton chemistry.414,415
Overall, these observations (and literature) are consistent with the view that a great many substances can have substantial effects on the rate of fibrinogen polymerisation and on the nature and structure of the fibres and clots formed. In some senses, fibrin structure might be considered to be a kind of bellwether of plasma health.
Effects of non-ionic substances
The nature of the clot formed upon thrombin activation can also be modulated by a variety of other substances416 besides trivalent ions. One such substance is glucose – and in diabetes the changes are more likely due to glycation of fibrinogen.236,416–418 Hormonal fluctuations also influence fibrin fibre morphology. Swanepoel and co-workers in 2014 were the first to show that oestrogen changes the ultrastructure of fibrin fibres.419,420 They found that the external and internal structure of the fibrin strands differed throughout the menstrual cycle. These changes coincided with the normal oestrogen peaks associated with the menstrual cycle. An increase in oestrogen during specifically the pre-ovulatory phase of the menstrual cycle resulted in a granular appearance of the fibrin network on high magnification. During pregnancy, which is associated with even more elevated oestrogen levels, the same granular appearance was typical, with an additional increase in dense matted deposits. These morphological changes persisted till at least 8 weeks post-partum. Oestrogen therefore may play a significant part in the hypercoagulable state associated with pregnancy.
The many oxidative and nitrosative stresses leading to protein modifications and their role in diseases are given e.g. by Dalle-Donne and colleagues.421–424 Unsurprisingly, substances that can react with fibrinogen directly can also have significant effects on clotting; indeed, aspirin affects the nature of the clot directly via acetylation,150 while peroxynitrite can inhibit fibrinolysis significantly,425 and fibrinogen nitration is pro-thrombotic.426,427
Iron and blood storage
Although not the entire focus of this article, we note that it is becoming increasingly recognised that as blood ages during storage it has a tendency to release iron and that this may give it properties that are suboptimal.428–438 As we have shown in the previous paragraphs, free iron can have a substantial role in determining the outcome of the clotting process, and this will need to be borne in mind as blood ages. The same is likely true for blood that ages in individuals.439,440
Inflammation, fibrinogen and red cell aggregability as assessed via the erythrocyte sedimentation rate and other means
As indicated, inflammation, fibrinogen levels and hypercoagulability are intimately connected. In a similar vein, fibrinogen levels are one of the strongest contributors to red cell aggregability and aggregation,441 and thence to the erythrocyte sedimentation rate, another classical indicator of inflammation.442–448 It should be noted that the difference between RBC aggregation and RBC aggregability is that the latter describes an intrinsic RBC property and the former describes the effect that results from this intrinsic cell property together with other factors (e.g. plasma proteins, temperature, pH, shear rate, blood flow), resulting in RBC aggregation. In this regard it is accepted that fibrinogen increases RBC aggregation, not aggregability.
This can have a significant diagnostic and indeed prognostic value (e.g.ref. 449–454). Here, the Laser-Assisted Optical Rotational Red Cell Analyzer (LORRCA)455–458 (marketed by Mechatronics) provides a convenient measurement, while atomic force microscopy assesses deformability at the level of the single cell.459–463
Similar comments apply to the changes in red cell distribution width also commonly seen in inflammatory diseases (e.g.ref. 464–474).
Cytokines linking inflammation, hypercoagulability and hypofibrinolysis
Given the considerable evidence noted above for the co-occurrence of inflammation, hypercoagulability and hypofibrinolysis, one might note in general terms that the relationship between two variables A and B that co-vary can take at least four forms: A affects B, B affects A, both A and B affect each other, and D affects both A and B. More complex variants such as A affects D and D affects B are not considered; these are known as ‘indirect effects’, and have proved problematic in studies of covariance (e.g. in protein folding based on phylogenetics,475 although considerable progress is being made in disentangling them476–480).
When looking for the potential biochemical changes or co-occurrences that might serve to link coagulation and inflammation, the chief players seem to be IL-1β481,482 and, in particular, IL-6,61,65,338,481–483 that seems to be involved in causing an iterative cycle of coagulability and inflammation. One important mechanism involving the (IL-1-mediated) activation of IL-6 that in turn induces fibrinogen synthesis by hepatocytes484–486 and other cells482,487–490 is especially pertinent. IL-6 is also involved in iron (dys)regulation, in particular by its ability to induce hepcidin biosynthesis (e.g.ref. 491–499). An overview of the role of these cytokines is shown in Fig. 9.
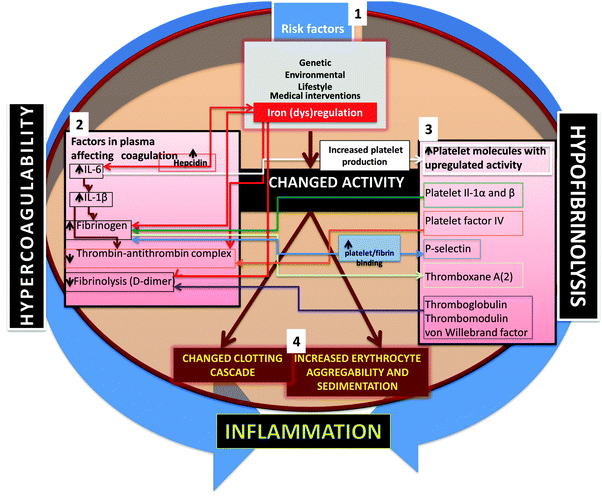 |
| Fig. 9 Hypercoagulation, hypofibrinolysis and inflammation: a brief overview of the roles of plasma and platelet factors. Risk factors (1) cause a changed coagulation factor profile in plasma (2), as well as an upregulation activity of platelet products (3) which feeds back to affect each coagulation (indicated by arrows between 2 and 3). This results in a changed clotting profile and increased erythrocyte aggregability and sedimentation rate (4) that may be observed macroscopically. | |
Relationship between inflammation and fibrosis
Fibrosis refers to the laying down of pathological variants of a variety of proteins in an insoluble form,500 and also occurs in response to inflammation. Although we are concentrating here on events in blood, it is not a coincidence that ‘fibrosis’ shares the same etymological root as fibrin(oge)n. Most pertinently, fibrosis can be seen under the same hypercoagulable conditions as discussed here,501–506 and may be considered to have a common cause (and, potentially, cure).
Concluding remarks
In the spirit of Integrative Biology, we have brought together a considerable body of literature on the effect that many diseases share a state of both hypercoagulability and hypofibrinolysis, and that part of this is due to the fact that they also share common causes in the form of inflammation and increased levels of substances such as inflammatory cytokines, fibrinogen and iron. Measuring these effects in blood or plasma may provide some useful and convenient approaches to diagnostics, and minimising them may provide some innovative system approaches to innovative therapies, to the benefit of all. Morphological methods cannot study changes or variations or conformational flexibility and variability of the specific forms (e.g. monomeric form) of the fibrin molecule, although this might indeed impact structural differences and polymerization characteristics. Therefore, ultrastructural investigation is proposed as a complementary methodology, together with methods described in this manuscript, to truly support an integrative approach to study clot formation (hypercoagulability) and hypofibrinolysis.
Acknowledgements
We thank the Biotechnology and Biological Sciences Research Council (BB/L025752/1) for supporting this collaboration. Janette Bester and Natasha Vermeulen prepared the samples for scanning electron microscopy.
References
- M. Hoffman and D. M. Monroe, Coagulation 2006: a modern view of hemostasis, Hematol. Oncol. Clin. North Am., 2007, 21, 1–11 CrossRef PubMed.
- M. R. Falvo, O. V. Gorkun and S. T. Lord, The molecular origins of the mechanical properties of fibrin, Biophys. Chem., 2010, 152, 15–20 CrossRef CAS PubMed.
- M. Delvaeye and E. M. Conway, Coagulation and innate immune responses: can we view them separately?, Blood, 2009, 114, 2367–2374 CrossRef CAS PubMed.
- S. Massberg, L. Grahl, M. L. von Bruehl, D. Manukyan, S. Pfeiler, C. Goosmann, V. Brinkmann, M. Lorenz, K. Bidzhekov, A. B. Khandagale, I. Konrad, E. Kennerknecht, K. Reges, S. Holdenrieder, S. Braun, C. Reinhardt, M. Spannagl, K. T. Preissner and B. Engelmann, Reciprocal coupling of coagulation and innate immunity via neutrophil serine proteases, Nat. Med., 2010, 16, 887–896 CrossRef CAS PubMed.
- C. T. Esmon, J. Xu and F. Lupu, Innate immunity and coagulation, J. Thromb. Haemostasis, 2011, 9, 182–188 CrossRef CAS PubMed.
- B. Engelmann and S. Massberg, Thrombosis as an intravascular effector of innate immunity, Nat. Rev. Immunol., 2013, 13, 34–45 CrossRef CAS PubMed.
- C. Schulz, B. Engelmann and S. Massberg, Crossroads of coagulation and innate immunity: the case of deep vein thrombosis, J. Thromb. Haemostasis, 2013, 11(suppl 1), 233–241 CrossRef PubMed.
- F. Cacciapuoti, Some considerations about the hypercoagulable states and their treatments, Blood Coagulation Fibrinolysis, 2011, 22, 155–159 CrossRef CAS PubMed.
- N. Mackman, Triggers, targets and treatments for thrombosis, Nature, 2008, 451, 914–918 CrossRef CAS PubMed.
- A. G. G. Turpie and C. Esmon, Venous and arterial thrombosis--pathogenesis and the rationale for anticoagulation, Thromb. Haemostasis, 2011, 105, 586–596 CrossRef CAS PubMed.
- N. Mackman, New insights into the mechanisms of venous thrombosis, J. Clin. Invest., 2012, 122, 2331–2336 CrossRef CAS PubMed.
- F. Horsted, J. West and M. J. Grainge, Risk of venous thromboembolism in patients with cancer: a systematic review and meta-analysis, PLoS Med., 2012, 9, e1001275 Search PubMed.
- N. Mackman, Mouse models, risk factors, and treatments of venous thrombosis, Arterioscler., Thromb., Vasc. Biol., 2012, 32, 554–555 CrossRef CAS PubMed.
- Y. Zhang, S. Fan, Y. Yao, J. Ding, Y. Wang, Z. Zhao, L. Liao, P. Li, F. Zang and G. J. Teng, In vivo near-infrared imaging of fibrin deposition in thromboembolic stroke in mice, PLoS One, 2012, 7, e30262 CAS.
- R. G. Hart, H. C. Diener, S. B. Coutts, J. D. Easton, C. B. Granger, M. J. O'Donnell, R. L. Sacco and S. J. Connolly, Embolic strokes of undetermined source: the case for a new clinical construct, Lancet Neurol., 2014, 13, 429–438 CrossRef.
- C. Madias and R. G. Trohman, The link between atrial fibrillation and stroke in women, Women's Health, 2011, 7, 375–382 Search PubMed.
- N. N. Lang and D. T. Connelly, Novel oral anticoagulants for the prevention of thromboembolism in patients with atrial fibrillation, J. R. Coll. Physicians Edinb., 2013, 43, 151–158 CrossRef CAS PubMed.
- S. Ansar, E. Chatzikonstantinou, R. Thiagarajah, L. Tritschler, M. Fatar, M. G. Hennerici and S. Meairs, Pro-inflammatory mediators and apoptosis correlate to rt-PA response in a novel mouse model of thromboembolic stroke, PLoS One, 2014, 9, e85849 Search PubMed.
- S. R. Levine, Hypercoagulable states and stroke: a selective review, CNS Spectr., 2005, 10, 567–578 Search PubMed.
- C. Medi, G. J. Hankey and S. B. Freedman, Stroke risk and antithrombotic strategies in atrial fibrillation, Stroke, 2010, 41, 2705–2713 CrossRef PubMed.
- R. F. Franco and P. H. Reitsma, Genetic risk factors of venous thrombosis, Hum. Genet., 2001, 109, 369–384 CrossRef CAS PubMed.
- R. A. S. Ariëns, M. de Lange, H. Snieder, M. Boothby, T. D. Spector and P. J. Grant, Activation markers of coagulation and fibrinolysis in twins: heritability of the prethrombotic state, Lancet, 2002, 359, 667–671 CrossRef.
- P. H. Viale, Abnormal clotting in cancer: an overview of pathophysiology and etiology, Semin. Oncol. Nurs., 2005, 21, 12–20 CrossRef PubMed.
- I. Martinelli, Thromboembolism in women, Semin. Thromb. Hemostasis, 2006, 32, 709–715 CrossRef CAS PubMed.
- A. M. Carter, C. M. Cymbalista, T. D. Spector and P. J. Grant, Heritability of clot formation, morphology, and lysis: the EuroCLOT study, Arterioscler., Thromb., Vasc. Biol., 2007, 27, 2783–2789 CrossRef CAS PubMed.
- W. Tang, M. Teichert, D. I. Chasman, J. A. Heit, P. E. Morange, G. Li, N. Pankratz, F. W. Leebeek, G. Pare, M. de Andrade, C. Tzourio, B. M. Psaty, S. Basu, R. Ruiter, L. Rose, S. M. Armasu, T. Lumley, S. R. Heckbert, A. G. Uitterlinden, M. Lathrop, K. M. Rice, M. Cushman, A. Hofman, J. C. Lambert, N. L. Glazer, J. S. Pankow, J. C. Witteman, P. Amouyel, J. C. Bis, E. G. Bovill, X. Kong, R. P. Tracy, E. Boerwinkle, J. I. Rotter, D. A. Trégouët, D. W. Loth, B. H. Stricker, P. M. Ridker, A. R. Folsom and N. L. Smith, A genome-wide association study for venous thromboembolism: the extended cohorts for heart and aging research in genomic epidemiology (CHARGE) consortium, Genet. Epidemiol., 2013, 37, 512–521 CrossRef PubMed.
- P. de Moerloose, A. Casini and M. Neerman-Arbez, Congenital Fibrinogen Disorders: An Update, Semin. Thromb. Hemostasis, 2013, 39, 585–595 CrossRef CAS PubMed.
- P. Woratanarat, C. Thaveeratitharm, T. Woratanarat, C. Angsanuntsukh, J. Attia and A. Thakkinstian, Meta-analysis of hypercoagulability genetic polymorphisms in Perthes disease, J. Orthop. Res., 2014, 32, 1–7 CrossRef CAS PubMed.
- M. Levi and S. M. Opal, Coagulation abnormalities in critically ill patients, Crit. Care, 2006, 10, 222 CrossRef PubMed.
- P. Meybohm, K. Zacharowski and C. F. Weber, Point-of-care coagulation management in intensive care medicine, Crit. Care, 2013, 17, 218 CrossRef PubMed.
- F. Arshad, T. Lisman and R. J. Porte, Hypercoagulability as a contributor to thrombotic complications in the liver transplant recipient, Liver Int., 2013, 33, 820–827 CrossRef CAS PubMed.
- J. K. Hennigs, H. J. Baumann, N. Lüneburg, G. Quast, L. Harbaum, J. Heyckendorf, K. Sydow, B. Schulte-Hubbert, M. Halank and H. Klose, Fibrinogen plasma concentration is an independent marker of haemodynamic impairment in chronic thromboembolic pulmonary hypertension, Sci. Rep., 2014, 4, 4808 Search PubMed.
- C. M. Johnson, L. Mureebe and D. Silver, Hypercoagulable states: a review, Vasc. Endovascular Surg., 2005, 39, 123–133 CrossRef PubMed.
- S. H. Alzahrani and R. A. Ajjan, Coagulation and fibrinolysis in diabetes, Diab. Vasc. Dis. Res., 2010, 7, 260–273 CrossRef CAS PubMed.
- J. A. M. Anderson and J. I. Weitz, Hypercoagulable states, Clin. Chest Med., 2010, 31, 659–673 CrossRef PubMed.
- R. Houbballah and G. M. LaMuraglia, Clotting Problems: Diagnosis and Management of Underlying Coagulopathies, Semin.
Vasc. Surg., 2010, 23, 221–227 CrossRef PubMed.
- A. J. Chu, Tissue factor, blood coagulation, and beyond: an overview, Int. J. Inflammation, 2011, 2011, 367284 Search PubMed.
- P. H. Reitsma, H. H. Versteeg and S. Middeldorp, Mechanistic view of risk factors for venous thromboembolism, Arterioscler., Thromb., Vasc. Biol., 2012, 32, 563–568 CrossRef CAS PubMed.
-
E. Klipp, R. Herwig, A. Kowald, C. Wierling and H. Lehrach, Systems biology in practice: concepts, implementation and clinical application, Wiley/VCH, Berlin, 2005 Search PubMed.
-
U. Alon, An introduction to systems biology: design principles of biological circuits, Chapman and Hall/CRC, London, 2006 Search PubMed.
-
D. B. Kell and J. D. Knowles, The role of modeling in systems biology, in System modeling in cellular biology from concepts to nuts and bolts, ed. Z. Szallasi, J. Stelling and V. Periwal, MIT Press, Cambridge, 2006, pp. 3–18 Search PubMed.
- D. B. Kell, Metabolomics, modelling and machine learning in systems biology: towards an understanding of the languages of cells. The 2005 Theodor Bücher lecture, FEBS J., 2006, 273, 873–894 CrossRef CAS PubMed.
-
B. Ø. Palsson, Systems biology: properties of reconstructed networks, Cambridge University Press, Cambridge, 2006 Search PubMed.
- E. H. S. Choy and G. S. Panayi, Mechanisms of disease: Cytokine pathways and joint inflammation in rheumatoid arthritis., N. Engl. J. Med., 2001, 344, 907–916 CrossRef CAS PubMed.
- W. W. Lin and M. Karin, A cytokine-mediated link between innate immunity, inflammation, and cancer, J. Clin. Invest., 2007, 117, 1175–1183 CrossRef CAS PubMed.
- M. Kopf, M. F. Bachmann and B. J. Marsland, Averting inflammation by targeting the cytokine environment, Nat. Rev. Drug Discovery, 2010, 9, 703–718 CrossRef CAS PubMed.
- C. Cicala and G. Cirino, Linkage between inflammation and coagulation: an update on the molecular basis of the crosstalk, Life Sci., 1998, 62, 1817–1824 CrossRef CAS.
- M. Levi and H. ten Cate, Disseminated intravascular coagulation, N. Engl. J. Med., 1999, 341, 586–592 CrossRef CAS PubMed.
- S. R. Coughlin, Thrombin signalling and protease-activated receptors, Nature, 2000, 407, 258–264 CrossRef CAS PubMed.
- P. Libby and D. I. Simon, Inflammation and thrombosis: the clot thickens, Circulation, 2001, 103, 1718–1720 CrossRef CAS.
- M. Thor, A. Yu and J. Swedenborg, Markers of inflammation and hypercoagulability in diabetic and nondiabetic patients with lower extremity ischemia, Thromb. Res., 2002, 105, 379–383 CrossRef CAS.
- D. S. G. Conway, P. Buggins, E. Hughes and G. Y. H. Lip, Predictive value of indexes of inflammation and hypercoagulability on success of cardioversion of persistent atrial fibrillation, Am. J. Cardiol., 2004, 94, 508–510 CrossRef PubMed.
- M. Levi, T. van der Poll and H. R. Buller, Bidirectional relation between inflammation and coagulation, Circulation, 2004, 109, 2698–2704 CrossRef PubMed.
- M. Van de Wouwer, D. Collen and E. M. Conway, Thrombomodulin-protein C-EPCR system: integrated to regulate coagulation and inflammation, Arterioscler., Thromb., Vasc. Biol., 2004, 24, 1374–1383 CrossRef CAS PubMed.
- A. J. Chu, Tissue factor mediates inflammation, Arch. Biochem. Biophys., 2005, 440, 123–132 CrossRef CAS PubMed.
- P. Dusitanond, J. W. Eikelboom, G. J. Hankey, J. Thom, G. Gilmore, K. Loh, Q. Yi, C. J. M. Klijn, P. Langton, F. M. van Bockxmeer, R. Baker and K. Jamrozik, Homocysteine-lowering treatment with folic acid, cobalamin, and pyridoxine does not reduce blood markers of inflammation, endothelial dysfunction, or hypercoagulability in patients with previous transient ischemic attack or stroke: a randomized substudy of the VITATOPS trial, Stroke, 2005, 36, 144–146 CrossRef CAS PubMed.
- C. T. Esmon, The interactions between inflammation and coagulation, Br. J. Haematol., 2005, 131, 417–430 CrossRef CAS PubMed.
- A. Gupta, A. Watkins, P. Thomas, R. Majer, N. Habubi, G. Morris and K. Pansari, Coagulation and inflammatory markers in Alzheimer's and vascular dementia, Int. J. Clin. Pract., 2005, 59, 52–57 CrossRef CAS PubMed.
- O. A. Hatoum and D. G. Binion, The vasculature and inflammatory bowel disease: contribution to pathogenesis and clinical pathology, Inflammatory Bowel Dis., 2005, 11, 304–313 CrossRef.
- G. Choi, M. J. Schultz, M. Levi and T. van der Poll, The relationship between inflammation and the coagulation system, Swiss Med. Wkly., 2006, 136, 139–144 Search PubMed.
- R. Marcucci, A. M. Gori, F. Giannotti, M. Baldi, V. Verdiani, S. Del Pace, C. Nozzoli and R. Abbate, Markers of hypercoagulability and inflammation predict mortality in patients with heart failure, J. Thromb. Haemostasis, 2006, 4, 1017–1022 CrossRef CAS PubMed.
- G. Aksu, C. Ozturk, K. Kavakli, F. Genel and N. Kutukculer, Hypercoagulability: interaction between inflammation and coagulation in familial Mediterranean fever, Clin. Rheumatol., 2007, 26, 366–370 CrossRef PubMed.
- S. Anžej, M. Božič, A. Antovič, P. Peternel, N. Gašperšič, U. Rot, G. Tratar and M. Stegnar, Evidence of hypercoagulability and inflammation in young patients long after acute cerebral ischaemia, Thromb. Res., 2007, 120, 39–46 CrossRef PubMed.
- K. Tomobe, The relation between adiponectin and four hypercoagulable, inflammatory biomarkers during normal pregnancy, Dokkyo J. Med. Sci., 2007, 34, 69–77 CAS.
- E. Chertok-Shacham, A. Ishay, I. Lavi and R. Luboshitzky, Biomarkers of hypercoagulability and inflammation in primary hyperparathyroidism, Med. Sci. Monit., 2008, 14, CR628–CR632 Search PubMed.
- O. Karabudak, R. E. Ulusoy, A. A. Erikci, E. Solmazgul, B. Dogan and Y. Harmanyeri, Inflammation and hypercoagulable state in adult psoriatic men, Acta Derm.-Venereol., 2008, 88, 337–340 CAS.
- Z. N. Kon, E. N. Brown, M. C. Grant, T. Ozeki, N. S. Burris, M. J. Collins, M. H. Kwon and R. S. Poston, Warm ischemia provokes inflammation and regional hypercoagulability within the heart during off-pump coronary artery bypass: a possible target for serine protease inhibition, Eur. J. Cardiothorac. Surg., 2008, 33, 215–221 CrossRef PubMed.
- R. Medzhitov, Origin and physiological roles of inflammation, Nature, 2008, 454, 428–435 CrossRef CAS PubMed.
- P. A. Gurbel, K. P. Bliden, R. P. Kreutz, J. Dichiara, M. J. Antonino and U. S. Tantry, The link between heightened thrombogenicity and inflammation: pre-procedure characterization of the patient at high risk for recurrent events after stenting, Platelets, 2009, 20, 97–104 CrossRef CAS PubMed.
- H. Yoshida and D. N. Granger, Inflammatory Bowel Disease: A Paradigm for the Link Between Coagulation and Inflammation, Inflammatory Bowel Dis., 2009, 15, 1245–1255 CrossRef PubMed.
- M. Levi and T. van der Poll, Inflammation and coagulation, Crit. Care Med., 2010, 38, S26–S34 CrossRef CAS PubMed.
- U. S. Tantry, K. P. Bliden, T. A. Suarez, R. P. Kreutz, J. Dichiara and P. A. Gurbel, Hypercoagulability, platelet function, inflammation and coronary artery disease acuity: results of the Thrombotic RIsk Progression (TRIP) study, Platelets, 2010, 21, 360–367 CrossRef CAS PubMed.
- J. I. Borissoff, H. M. H. Spronk and H. ten Cate, The Hemostatic System as a Modulator of Atherosclerosis, N. Engl. J. Med., 2011, 364, 1746–1760 CrossRef CAS PubMed.
- C. S. Kitchens, D. Erkan, L. R. Brandão, S. Hahn, A. H. James, R. Kulkarni, M. Pericak-Vance, J. Vance and T. L. Ortel, Thrombotic Storm Revisited: Preliminary Diagnostic Criteria Suggested by the Thrombotic Storm Study Group, Am. J. Med., 2011, 124, 290–296 CrossRef PubMed.
- J. Petäjä, Inflammation and coagulation. An overview, Thromb. Res., 2011, 127(suppl 2), S34–S37 CrossRef.
- T. van der Poll, J. D. de Boer and M. Levi, The effect of inflammation on coagulation and vice versa, Curr. Opin. Infect. Dis., 2011, 24, 273–278 CrossRef CAS PubMed.
- C. T. Esmon, Molecular circuits in thrombosis and inflammation, Thromb. Haemostasis, 2013, 109, 416–420 CrossRef CAS PubMed.
- M. Levi and T. van der Poll, Disseminated intravascular coagulation: a review for the internist, Intern. Emerg. Med., 2013, 8, 23–32 CrossRef PubMed.
- C. T. Esmon, Targeting factor Xa and thrombin: impact on coagulation and beyond, Thromb. Haemostasis, 2014, 111, 625–633 CrossRef CAS PubMed.
- E. D. Weinberg, Iron out-of-balance: a risk factor for acute and chronic diseases, Hemoglobin, 2008, 32, 117–122 CrossRef CAS PubMed.
- D. B. Kell, Iron behaving badly: inappropriate iron chelation as a major contributor to the aetiology of vascular and other progressive inflammatory and degenerative diseases, BMC Med. Genomics, 2009, 2, 2 CrossRef PubMed.
- D. B. Kell, Towards a unifying, systems biology understanding of large-scale cellular death and destruction caused by poorly liganded iron: Parkinson's, Huntington's, Alzheimer's, prions, bactericides, chemical toxicology and others as examples, Arch. Toxicol., 2010, 577, 825–889 CrossRef PubMed.
- E. D. Weinberg, The hazards of iron loading, Metallomics, 2010, 2, 732–740 RSC.
- T. D. Coates, Physiology and pathophysiology of iron in hemoglobin-associated diseases, Free Radical Biol. Med., 2014, 72C, 23–40 CrossRef PubMed.
- P. J. Urrutia, N. P. Mena and M. T. Núñez, The interplay between iron accumulation, mitochondrial dysfunction, and inflammation during the execution step of neurodegenerative disorders, Front. Pharmacol., 2014, 5, 38 Search PubMed.
- L. A. Norris, Blood coagulation, Best Pract. Res. Clin. Obstet. Gynaecol., 2003, 17, 369–383 CrossRef.
- S. A. Smith, The cell-based model of coagulation, J. Vet. Emerg. Crit. Care, 2009, 19, 3–10 CrossRef PubMed.
- E. Pretorius and D. B. Kell, Diagnostic morphology: biophysical indicators for iron-driven inflammatory diseases, Integr. Biol., 2014, 6, 486–510 RSC.
- S. Narayanan, Multifunctional roles of thrombin, Ann. Clin. Lab. Sci., 1999, 29, 275–280 CAS.
- S. S. Adam, N. S. Key and C. S. Greenberg, D-dimer antigen: current concepts and future prospects, Blood, 2009, 113, 2878–2887 CrossRef CAS PubMed.
- J. M. Siller-Matula, M. Schwameis, A. Blann, C. Mannhalter and B. Jilma, Thrombin as a multi-functional enzyme. Focus on in vitro and in vivo effects, Thromb. Haemostasis, 2011, 106, 1020–1033 CrossRef CAS PubMed.
- P. P. Tanos, G. K. Isbister, D. G. Lalloo, C. M. Kirkpatrick and S. B. Duffull, A model for venom-induced consumptive coagulopathy in snake bite, Toxicon, 2008, 52, 769–780 CrossRef CAS PubMed.
- T. Wajima, G. K. Isbister and S. B. Duffull, A comprehensive model for the humoral coagulation network in humans, Clin. Pharmacol. Ther., 2009, 86, 290–298 CrossRef CAS PubMed.
- A. Gulati, J. M. Faed, G. K. Isbister and S. B. Duffull, Development and evaluation of a prototype of a novel clotting time test to monitor enoxaparin, Pharm. Res., 2012, 29, 225–235 CrossRef CAS PubMed.
- A. Gulati, G. K. Isbister and S. B. Duffull, Effect of Australian elapid venoms on blood coagulation: Australian Snakebite Project (ASP-17), Toxicon, 2013, 61, 94–104 CrossRef CAS PubMed.
- A. Gulati, G. K. Isbister and S. B. Duffull, Scale reduction of a systems coagulation model with an application to modeling pharmacokinetic-pharmacodynamic data, CPT: Pharmacometrics Syst. Pharmacol., 2014, 3, e90 CrossRef CAS PubMed.
- N. Le Novère, B. Bornstein, A. Broicher, M. Courtot, M. Donizelli, H. Dharuri, L. Li, H. Sauro, M. Schilstra, B. Shapiro, J. L. Snoep and M. Hucka, BioModels Database: a free, centralized database of curated, published, quantitative kinetic models of biochemical and cellular systems, Nucleic Acids Res., 2006, 34, D689–D691 CrossRef PubMed.
- C. Li, M. Donizelli, N. Rodriguez, H. Dharuri, L. Endler, V. Chelliah, L. Li, E. U. He, A. Henry, M. I. Stefan, J. L. Snoep, M. Hucka, N. Le Novère and C. Laibe, BioModels Database: An enhanced, curated and annotated resource for published quantitative kinetic models, BMC Syst. Biol., 2010, 4, 92 CrossRef PubMed.
- C. Li, M. Courtot, N. Le Novère and C. Laibe, BioModels.net Web Services, a free and integrated toolkit for computational modelling software, Briefings Bioinf., 2010, 11, 270–277 CrossRef CAS PubMed.
- M. Hucka, A. Finney, H. M. Sauro, H. Bolouri, J. C. Doyle, H. Kitano, A. P. Arkin, B. J. Bornstein, D. Bray, A. Cornish-Bowden, A. A. Cuellar, S. Dronov, E. D. Gilles, M. Ginkel, V. Gor, I. I. Goryanin, W. J. Hedley, T. C. Hodgman, J. H. Hofmeyr, P. J. Hunter, N. S. Juty, J. L. Kasberger, A. Kremling, U. Kummer, N. Le Novere, L. M. Loew, D. Lucio, P. Mendes, E. Minch, E. D. Mjolsness, Y. Nakayama, M. R. Nelson, P. F. Nielsen, T. Sakurada, J. C. Schaff, B. E. Shapiro, T. S. Shimizu, H. D. Spence, J. Stelling, K. Takahashi, M. Tomita, J. Wagner and J. Wang, The systems biology markup language (SBML): a medium for representation and exchange of biochemical network models, Bioinformatics, 2003, 19, 524–531 CrossRef CAS PubMed.
- M. Hucka, A. Finney, B. J. Bornstein, S. M. Keating, B. E. Shapiro, J. Matthews, B. L. Kovitz, M. J. Schilstra, A. Funahashi, J. C. Doyle and H. Kitano, Evolving a lingua franca and associated software infrastructure for computational systems biology: the Systems Biology Markup Language (SBML) project, Syst. Biol., 2004, 1, 41–53 CrossRef CAS.
- M. Hoffman and D. M. Monroe, 3rd, A cell-based model of hemostasis, Thromb. Haemostasis, 2001, 85, 958–965 CAS.
- D. M. Monroe and M. Hoffman, What does it take to make the perfect clot?, Arterioscler., Thromb., Vasc. Biol., 2006, 26, 41–48 CrossRef CAS PubMed.
- S. M. Bates and J. I. Weitz, Coagulation assays, Circulation, 2005, 112, e53–e60 CrossRef PubMed.
- E. Berntorp and G. L. Salvagno, Standardization and clinical utility of thrombin-generation assays, Semin. Thromb. Hemostasis, 2008, 34, 670–682 CrossRef CAS PubMed.
- M. T. Ganter and C. K. Hofer, Coagulation monitoring: Current techniques and clinical use of viscoelastic point-of-care coagulation devices, Anesth. Analg., 2008, 106, 1366–1375 Search PubMed.
- M. A. McMichael and S. A. Smith, Viscoelastic coagulation testing: technology, applications, and limitations, Vet. Clin. Pathol., 2011, 40, 140–153 CrossRef PubMed.
- M. Chitlur, Challenges in the laboratory analyses of bleeding disorders, Thromb. Res., 2012, 130, 1–6 CrossRef CAS PubMed.
- P. I. Johansson, T. Stissing, L. Bochsen and S. R. Ostrowski, Thrombelastography and tromboelastometry in assessing coagulopathy in trauma, Scand. J. Trauma Resusc. Emerg. Med., 2009, 17, 45 CrossRef PubMed.
- R. J. Luddington, Thrombelastography/thromboelastometry, Clin. Lab. Haematol., 2005, 27, 81–90 CrossRef CAS PubMed.
- H. Reikvam, E. Steien, B. Hauge, K. Liseth, K. G. Hagen, R. Storkson and T. Hervig, Thrombelastography, Transfus. Apher. Sci., 2009, 40, 119–123 CrossRef PubMed.
- A. Afshari, A. Wikkelsø, J. Brok, A. M. Moller and J. Wetterslev, Thrombelastography (TEG) or thromboelastometry (ROTEM) to monitor haemotherapy versus usual care in patients with massive transfusion, Cochrane Database Syst. Rev., 2011, CD007871 Search PubMed.
- D. Bolliger, M. D. Seeberger and K. A. Tanaka, Principles and practice of thromboelastography in clinical coagulation management and transfusion practice, Transfus. Med. Rev., 2012, 26, 1–13 CrossRef PubMed.
- A. Sankarankutty, B. Nascimento, L. Teodoro da Luz and S. Rizoli, TEG(R) and ROTEM(R) in trauma: similar test but different results?, World J. Emerg. Surg., 2012, 7(suppl 1), S3 CrossRef PubMed.
- B. Sørensen and J. Ingerslev, Tailoring haemostatic treatment to patient requirements – an update on monitoring haemostatic response using thrombelastography, Haemophilia, 2005, 11(suppl 1), 1–6 CrossRef PubMed.
- J. J. van Veen, A. Gatt, A. E. Bowyer, P. C. Cooper, S. Kitchen and M. Makris, Calibrated automated thrombin generation and modified thromboelastometry in haemophilia A, Thromb. Res., 2009, 123, 895–901 CrossRef CAS PubMed.
- U. Kjellberg and M. Hellgren, Sonoclot signature during normal pregnancy, Intensive Care Med., 2000, 26, 206–211 CrossRef CAS.
- D. A. Hett, D. Walker, S. N. Pilkington and D. C. Smith, Sonoclot analysis, Br. J. Anaesth., 1995, 75, 771–776 CrossRef CAS PubMed.
- S. Sharma, J. Uprichard, A. Moretti, H. Boyce, R. Szydlo and G. Stocks, Use of thromboelastography to assess the combined role of pregnancy and obesity on coagulation: a prospective study, Int. J. Obstet. Anesth., 2013, 22, 113–118 CrossRef CAS PubMed.
- M. Hellgren, Hemostasis during normal pregnancy and puerperium, Semin. Thromb. Hemostasis, 2003, 29, 125–130 CrossRef CAS PubMed.
- V. G. Nielsen, A comparison of the Thrombelastograph and the ROTEM, Blood Coagulation Fibrinolysis, 2007, 18, 247–252 CrossRef PubMed.
- G. N. B. Jackson, K. J. Ashpole and S. M. Yentis, The TEG((R)) vs the ROTEM((R)) thromboelastography/thromboelastometry systems, Anaesthesia, 2009, 64, 212–215 CrossRef CAS PubMed.
- T. Tomori, D. Hupalo, K. Teranishi, S. Michaud, M. Hammett, D. Freilich, R. McCarron and F. Arnaud, Evaluation of coagulation stages of hemorrhaged swine: comparison of thromboelastography and rotational elastometry, Blood Coagulation Fibrinolysis, 2010, 21, 20–27 CrossRef PubMed.
- L. F. Venema, W. J. Post, H. G. Hendriks, R. C. Huet, J. T. de Wolf and A. J. de Vries, An assessment of clinical interchangeability of TEG and RoTEM thromboelastographic variables in cardiac surgical patients, Anesth. Analg., 2010, 111, 339–344 Search PubMed.
- M. Furuhashi, N. Ura, K. Hasegawa, H. Yoshida, K. Tsuchihashi, T. Miura and K. Shimamoto, Sonoclot coagulation analysis: new bedside monitoring for determination of the appropriate heparin dose during haemodialysis, Nephrol., Dial., Transplant., 2002, 17, 1457–1462 CrossRef CAS PubMed.
- J. J. Liszka-Hackzell and G. Ekback, Analysis of the information content in Sonoclot data and reconstruction of coagulation test variables, J. Med. Syst., 2002, 26, 1–8 CrossRef.
- T. Yamada, N. Katori, K. A. Tanaka and J. Takeda, Impact of Sonoclot hemostasis analysis after cardiopulmonary bypass on postoperative hemorrhage in cardiac surgery, J. Anesth., 2007, 21, 148–152 CrossRef PubMed.
- K. R. Brandy, R. M. Meyer, X. Luo, G. H. Rao, Y. H. Datta and A. A. Divani, Evaluation of the coagulation profile among oral and vaginal combined hormonal contraceptive users using sonoclot coagulation analyzer, Clin. Appl. Thromb./Hemostasis, 2012, 18, 576–581 CrossRef PubMed.
- V. G. Nielsen, B. M. Cohen and E. Cohen, Elastic modulus-based thrombelastographic quantification of plasma clot fibrinolysis with progressive plasminogen activation, Blood Coagulation Fibrinolysis, 2006, 17, 75–81 CrossRef CAS PubMed.
- V. G. Nielsen, Beyond cell based models of coagulation: analyses of coagulation with clot “lifespan” resistance-time relationships, Thromb. Res., 2008, 122, 145–152 CrossRef CAS PubMed.
- V. G. Nielsen, Corn trypsin inhibitor decreases tissue-type plasminogen activator-mediated fibrinolysis of human plasma, Blood Coagulation Fibrinolysis, 2009, 20, 191–196 CrossRef CAS PubMed.
- V. G. Nielsen, J. K. Kirklin, W. L. Holman and B. L. Steenwyk, Clot lifespan model analysis of the effects of warfarin on thrombus growth and fibrinolysis: role of contact protein and tissue factor initiation, ASAIO J., 2009, 55, 33–40 CrossRef CAS PubMed.
- V. G. Nielsen, R. T. Lyerly, 3rd and W. Q. Gurley, The effect of dilution on plasma coagulation kinetics determined by thrombelastography is dependent on antithrombin activity and mode of activation, Anesth. Analg., 2004, 99, 1587–1592 CAS , table of contents.
- G. E. Rivard, K. E. Brummel-Ziedins, K. G. Mann, L. Fan, A. Hofer and E. Cohen, Evaluation of the profile of thrombin generation during the process of whole blood clotting as assessed by thrombelastography, J. Thromb. Haemostasis, 2005, 3, 2039–2043 CrossRef CAS PubMed.
- T. C. Ellis, V. G. Nielsen, M. B. Marques and J. K. Kirklin, Thrombelastographic measures of clot propagation: a comparison of alpha with the maximum rate of thrombus generation, Blood Coagulation Fibrinolysis, 2007, 18, 45–48 CrossRef PubMed.
- T. M. Wilson, The conductivity of blood in coagulation, Biochem. J., 1907, 2, 377–382 CAS.
- A. Ur, Changes in Electrical Impedance of Blood during Coagulation, Nature, 1970, 226, 269–270 CrossRef CAS.
- A. Ur, Detection of Clot Retraction through Changes of Electrical Impedance of Blood during Coagulation, Am. J. Clin. Pathol., 1971, 56, 713–718 CAS.
- A. Ur, Analysis and Interpretation of Impedance Blood-Coagulation Curve, Am. J. Clin. Pathol., 1977, 67, 470–476 CAS.
- H. Berney and J. J. O'Riordan, Impedance measurement monitors blood coagulation, Analog Dialogue, 2008, 42–08, 1–3 Search PubMed.
- Y. Hayashi, Y. Katsumoto, S. Omori, A. Yasuda, K. Asami, M. Kaibara and I. Uchimura, Dielectric coagulometry: a new approach to estimate venous thrombosis risk, Anal. Chem., 2010, 82, 9769–9774 CrossRef CAS PubMed.
- B. Ramaswamy, Y. T. T. Yeh and S. Y. Zheng, Microfluidic device and system for point-of-care blood coagulation measurement based on electrical impedance sensing, Sens. Actuators, B, 2013, 180, 21–27 CrossRef CAS PubMed.
- H. Beving, L. E. G. Eriksson, C. L. Davey and D. B. Kell, Dielectric properties of human blood and erythrocytes at radio frequencies (0.2–10 MHz): dependence on medium composition, Eur. Biophys. J., 1994, 23, 207–215 CrossRef CAS.
- R. Pethig and D. B. Kell, The passive electrical properties of biological systems: their significance in physiology, biophysics and biotechnology, Phys. Med. Biol., 1987, 32, 933–970 CrossRef CAS.
- C. M. Harris, R. W. Todd, S. J. Bungard, R. W. Lovitt, J. G. Morris and D. B. Kell, The dielectric permittivity of microbial suspensions at radio frequencies: a novel method for the estimation of microbial biomass, Enzyme Microb. Technol., 1987, 9, 181–186 CrossRef CAS.
- C. L. Davey, H. M. Davey, D. B. Kell and R. W. Todd, An Introduction to The Dielectric Estimation of Cellular Biomass in Real Time, with Special Emphasis on Measurements at High Volume Fractions, Anal. Chim. Acta, 1993, 279, 155–161 CrossRef.
-
C. L. Davey and D. B. Kell, The Low-Frequency Dielectric Properties of Biological Cells, in Bioelectrochemistry of Cells and Tissues, ed. D. Walz, H. Berg and G. Milazzo, Birkauser, Zürich, 1995, pp.159–207 Search PubMed.
- B. Blombäck and M. Okada, Fibrin gel structure and clotting time, Thromb. Res., 1982, 25, 51–70 CrossRef.
- B. Blombäck, K. Carlsson, B. Hessel, A. Liljeborg, R. Procyk and N. Åslund, Native Fibrin Gel Networks Observed by 3d Microscopy, Permeation and Turbidity, Biochim. Biophys. Acta, 1989, 997, 96–110 CrossRef.
- R. A. Ajjan, K. F. Standeven, M. Khanbhai, F. Phoenix, K. C. Gersh, J. W. Weisel, M. T. Kearney, R. A. S. Ariëns and P. J. Grant, Effects of aspirin on clot structure and fibrinolysis using a novel in vitro cellular system, Arterioscler., Thromb., Vasc. Biol., 2009, 29, 712–717 CrossRef CAS PubMed.
- E. Pretorius, S. Briedenhann, J. Marx, E. Smit, C. Van Der Merwe, M. Pieters and C. Franz, Ultrastructural comparison of the morphology of three different platelet and fibrin fiber preparations, Anat. Rec., 2007, 290, 188–198 CrossRef CAS PubMed.
- A. Undas, A. Slowik, P. Wolkow, A. Szczudlik and W. Tracz, Fibrin clot properties in acute ischemic stroke: relation to neurological deficit, Thromb. Res., 2010, 125, 357–361 CrossRef CAS PubMed.
- M. R. Martinez, A. Cuker, A. M. Mills, A. Crichlow, R. T. Lightfoot, I. N. Chernysh, C. Nagaswami, J. W. Weisel and H. Ischiropoulos, Enhanced lysis and accelerated establishment of viscoelastic properties of fibrin clots are associated with pulmonary embolism, Am. J. Physiol., 2014, 306, L397–L404 CAS.
- M. M. Tripathi, Z. Hajjarian, E. M. Van Cott and S. K. Nadkarni, Assessing blood coagulation status with laser speckle rheology, Biomed. Opt. Express, 2014, 5, 817–831 CrossRef PubMed.
- H. C. Chang, T. J. Cheng, T. H. Wu and T. M. Lin, Determination of coagulation time in whole blood containing anticoagulant by piezoelectric quartz crystal sensor, Sens. Actuators, B, 2000, 66, 296–298 CrossRef CAS.
- L. G. Puckett, G. Barrett, D. Kouzoudis, C. Grimes and L. G. Bachas, Monitoring blood coagulation with magnetoelastic sensors, Biosens. Bioelectron., 2003, 18, 675–681 CrossRef CAS.
- L. G. Puckett, J. K. Lewis, A. Urbas, X. D. Cui, D. Y. Gao and L. G. Bachas, Magnetoelastic transducers for monitoring coagulation, clot inhibition, and fibrinolysis, Biosens. Bioelectron., 2005, 20, 1737–1743 CrossRef CAS PubMed.
- T. M. Lin, T. J. Cheng, T. H. Wu and H. C. Chang, Comparing a piezoelectric quartz crystal with an optical coagulometer in monitoring high-dose heparin therapy by determining whole blood activated partial thromboplastin time and activated clotting time, Sens. Actuators, B, 2005, 109, 270–277 CrossRef CAS PubMed.
- L. F. Harris, V. Castro-Lopez and A. J. Killard, Coagulation monitoring devices: Past, present, and future at the point of care, TrAC, Trends Anal. Chem., 2013, 50, 85–95 CrossRef CAS PubMed.
- C. L. Yang, S. J. Huang, C. W. Chou, Y. C. Chiou, K. P. Lin, M. S. Tsai and K. C. Young, Design and evaluation of a portable optical-based biosensor for testing whole blood prothrombin time, Talanta, 2013, 116, 704–711 CrossRef CAS PubMed.
- C. Yao, L. Qu and W. Fu, Detection of fibrinogen and coagulation factor VIII in plasma by a quartz crystal microbalance biosensor, Sensors, 2013, 13, 6946–6956 CrossRef CAS PubMed.
- A. Undas, Fibrin clot properties and their modulation in thrombotic disorders, Thromb. Haemostasis, 2014, 112, 32–42 CrossRef CAS PubMed.
- E. Danese, M. Montagnana, G. Cervellin and G. Lippi, Hypercoagulability, D-dimer and atrial fibrillation: an overview of biological and clinical evidence, Ann. Med., 2014, 1–8 Search PubMed.
- A. Gupta and K. Pansari, The association between blood coagulation markers, atherothrombosis and dementia, Int. J. Clin. Pract., 2003, 57, 107–111 CAS.
- L. Vaccarino, G. I. Forte, M. Palmeri, G. Misiano, E. Porcellini, M. Chiappelli, L. Scola, C. Caruso, F. Licastro and D. Lio, Role of prothrombotic polymorphisms in successful or unsuccessful aging, Biogerontology, 2011, 12, 445–450 CrossRef CAS PubMed.
- N. Purandare, A. Burns, K. J. Daly, J. Hardicre, J. Morris, G. Macfarlane and C. McCollum, Cerebral emboli as a potential cause of Alzheimer's disease and vascular dementia: case-control study, BMJ, 2006, 332, 1119–1122 CrossRef PubMed.
- M. Cortes-Canteli and S. Strickland, Fibrinogen, a possible key player in Alzheimer's disease, J. Thromb. Haemostasis, 2009, 7, 146–150 CrossRef CAS PubMed.
- H. J. Ahn, D. Zamolodchikov, M. Cortes-Canteli, E. H. Norris, J. F. Glickman and S. Strickland, Alzheimer's disease peptide beta-amyloid interacts with fibrinogen and induces its oligomerization, Proc. Natl. Acad. Sci. U. S. A., 2010, 107, 21812–21817 CrossRef CAS PubMed.
- M. Cortes-Canteli, D. Zamolodchikov, H. J. Ahn, S. Strickland and E. H. Norris, Fibrinogen and Altered Hemostasis in Alzheimer's Disease, J. Alzheimer's Dis., 2012, 32, 599–608 Search PubMed.
- N. Purandare, A. Burns, J. Morris, E. P. Perry, J. Wren and C. McCollum, Association of Cerebral Emboli With Accelerated Cognitive Deterioration in Alzheimer's Disease and Vascular Dementia, Am. J. Psychiatry, 2012, 169, 300–308 CrossRef PubMed.
- B. Lipinski and E. Pretorius, The Role of Iron-Induced Fibrin in the Pathogenesis of Alzheimer's Disease and the Protective Role of Magnesium, Front. Hum. Neurosci., 2013, 7, 735 CrossRef PubMed.
- J. L. Francis, D. A. Francis and G. J. Gunathilagan, Assessment of hypercoagulability in patients with cancer using the Sonoclot Analyzer and thromboelastography, Thromb. Res., 1994, 74, 335–346 CrossRef CAS.
- F. R. Rickles and M. N. Levine, Epidemiology of thrombosis in cancer, Acta Haematol., 2001, 106, 6–12 CrossRef CAS PubMed.
- F. R. Rickles and A. Falanga, Molecular basis for the relationship between thrombosis and cancer, Thromb. Res., 2001, 102, V215–V224 CrossRef CAS.
- G. J. Caine, P. S. Stonelake, G. Y. H. Lip and S. T. Kehoe, The hypercoagulable state of malignancy: pathogenesis and current debate, Neoplasia, 2002, 4, 465–473 CrossRef CAS PubMed.
- H. C. Kwaan, S. Parmar and J. Wang, Pathogenesis of increased risk of thrombosis in cancer, Semin. Thromb. Hemostasis, 2003, 29, 283–290 CrossRef CAS PubMed.
- A. Y. Y. Lee and M. N. Levine, Venous thromboembolism and cancer: risks and outcomes, Circulation, 2003, 107, I17–I21 Search PubMed.
- R. S. Cunningham, Therapeutic options for the treatment of cancer-associated thrombosis, Semin. Oncol. Nurs., 2005, 21, 21–40 CrossRef PubMed.
- P. K. Gupta, V. D. Charan and H. Kumar, Cancer related thrombophilia: clinical importance and management strategies, J. Assoc. Physicians India, 2005, 53, 877–882 CAS.
- L. R. Zacharski, Hypercoagulability preceding cancer. The iron hypothesis, J. Thromb. Haemostasis, 2005, 3, 585–588 CrossRef CAS PubMed.
- F. R. Rickles, Cancer and thrombosis in women – molecular mechanisms, Thromb. Res., 2009, 123(suppl 2), S16–S20 CrossRef.
- E. G. Aoun, K. M. Musallam, M. Abou-Ghazal and A. T. Taher, Malignancy and hypercoagulability: a two-way association revisited, J. Thromb. Thrombolysis, 2010, 30, 340–341 CrossRef PubMed.
- L. G. Lima and R. Q. Monteiro, Activation of blood coagulation in cancer: implications for tumour progression, Biosci. Rep., 2013, 33, e00064 CrossRef PubMed.
- A. Falanga, L. Russo and C. Verzeroli, Mechanisms of thrombosis in cancer, Thromb. Res., 2013, 131(suppl 1), S59–S62 CrossRef CAS.
- J. F. Timp, S. K. Braekkan, H. H. Versteeg and S. C. Cannegieter, Epidemiology of cancer-associated venous thrombosis, Blood, 2013, 122, 1712–1723 CrossRef CAS PubMed.
- E. D'Asti, N. Magnus, B. Meehan, D. Garnier and J. Rak, Genetic basis of thrombosis in cancer, Semin. Thromb. Hemostasis, 2014, 40, 284–295 CrossRef PubMed.
- J. S. Chen, D. C. Chan, M. J. Chung, L. C. Chang and V. C. Yang, Altered fibrinolytic activities in gastric cancer and uremic patients after intervention, Clin. Nephrol., 2009, 72, 468–472 CrossRef CAS.
- C. J. Glueck, C. Richardson-Royer, R. Schultz, T. Burger, D. Bowe, J. Padda and P. Wang, Testosterone therapy, thrombophilia-hypofibrinolysis, and hospitalization for deep venous thrombosis-pulmonary embolus: an exploratory, hypothesis-generating study, Clin. Appl. Thromb./Hemostasis, 2014, 20, 244–249 CrossRef CAS PubMed.
- S. Sallah, Review. Right ventricle thrombosis and the hypercoagulable states, Clin. Adv. Hematol. Oncol., 2004, 2, 180 Search PubMed.
- C. J. Song, A. Nakagomi, S. Chandar, H. Cai, I. G. S. Lim, H. P. McNeil, S. B. Freedman and C. L. Geczy, C-reactive protein contributes to the hypercoagulable state in coronary artery disease, J. Thromb. Haemostasis, 2006, 4, 98–106 CrossRef CAS PubMed.
- R. Ajjan and P. J. Grant, Coagulation and atherothrombotic disease, Atherosclerosis, 2006, 186, 240–259 CrossRef CAS PubMed.
- R. Ajjan, B. C. Lim, K. F. Standeven, R. Harrand, S. Dolling, F. Phoenix, R. Greaves, R. H. Abou-Saleh, S. Connell, D. A. Smith, J. W. Weisel, P. J. Grant and R. A. S. Ariëns, Common variation in the C-terminal region of the fibrinogen beta-chain: effects on fibrin structure, fibrinolysis and clot rigidity, Blood, 2008, 111, 643–650 CrossRef CAS PubMed.
- M. Y. Chan, F. Andreotti and R. C. Becker, Hypercoagulable states in cardiovascular disease, Circulation, 2008, 118, 2286–2297 CrossRef PubMed.
- M. R. Di Tullio, S. Homma, Z. Jin and R. L. Sacco, Aortic atherosclerosis, hypercoagulability, and stroke the APRIS (Aortic Plaque and Risk of Ischemic Stroke) study, J. Am. Coll. Cardiol., 2008, 52, 855–861 CrossRef PubMed.
- M. R. Di Tullio, C. Russo, Z. Z. Jin, R. L. Sacco, J. P. Mohr and S. Homma, P. F. O. C. S, Aortic Arch Plaques and Risk of Recurrent Stroke and Death, Circulation, 2009, 119, 2376–2382 CrossRef PubMed.
- J. I. Borissoff, S. Heeneman, E. Kilinç, P. Kassák, R. Van Oerle, K. Winckers, J. W. P. Govers-Riemslag, K. Hamulyák, T. M. Hackeng, M. J. A. P. Daemen, H. Ten Cate and H. M. H. Spronk, Early Atherosclerosis Exhibits an Enhanced Procoagulant State, Circulation, 2010, 122, 821–830 CrossRef CAS PubMed.
- A. J. Yarur, A. R. Deshpande, D. M. Pechman, L. Tamariz, M. T. Abreu and D. A. Sussman, Inflammatory bowel disease is associated with an increased incidence of cardiovascular events, Am. J. Gastroenterol., 2011, 106, 741–747 CrossRef PubMed.
- R. Loeffen, H. M. H. Spronk and H. ten Cate, The impact of blood coagulability on atherosclerosis and cardiovascular disease, J. Thromb. Haemostasis, 2012, 10, 1207–1216 CrossRef CAS PubMed.
- I. Juhan-Vague, M. C. Alessi and P. E. Morange, Hypofibrinolysis and increased PAI-1 are linked to atherothrombosis via insulin resistance and obesity, Ann. Med., 2000, 32(suppl 1), 78–84 CAS.
- J. P. Collet, Y. Allali, C. Lesty, M. L. Tanguy, J. Silvain, A. Ankri, B. Blanchet, R. Dumaine, J. Gianetti, L. Payot, J. W. Weisel and G. Montalescot, Altered fibrin architecture is associated with hypofibrinolysis and premature coronary atherothrombosis, Arterioscler., Thromb., Vasc. Biol., 2006, 26, 2567–2573 CrossRef CAS PubMed.
- A. Undas, M. Celinska-Löwenhoff, T. Löwenhoff and A. Szczeklik, Statins, fenofibrate, and quinapril increase clot permeability and enhance fibrinolysis in patients with
coronary artery disease, J. Thromb. Haemostasis, 2006, 4, 1029–1036 CrossRef CAS PubMed.
- A. Undas, D. Plicner, E. Stepien, R. Drwila and J. Sadowski, Altered fibrin clot structure in patients with advanced coronary artery disease: a role of C-reactive protein, lipoprotein(a) and homocysteine, J. Thromb. Haemostasis, 2007, 5, 1988–1990 CrossRef CAS PubMed.
- N. Bhasin, R. A. S. Ariëns, R. M. West, D. J. Parry, P. J. Grant and D. J. A. Scott, Altered fibrin clot structure and function in the healthy first-degree relatives of subjects with intermittent claudication, J. Vasc. Surg., 2008, 48, 1497–1503 CrossRef PubMed , 1503 e1491.
- A. Undas, K. Szuldrzynski, E. Stepien, J. Zalewski, J. Godlewski, W. Tracz, M. Pasowicz and K. Zmudka, Reduced clot permeability and susceptibility to lysis in patients with acute coronary syndrome: effects of inflammation and oxidative stress, Atherosclerosis, 2008, 196, 551–557 CrossRef CAS PubMed.
- D. J. A. Scott, P. Prasad, H. Philippou, S. T. Rashid, S. Sohrabi, D. Whalley, A. Kordowicz, Q. Tang, R. M. West, A. Johnson, J. Woods, R. A. Ajjan and R. A. S. Ariëns, Clot architecture is altered in abdominal aortic aneurysms and correlates with aneurysm size, Arterioscler., Thromb., Vasc. Biol., 2011, 31, 3004–3010 CrossRef CAS PubMed.
- M. Bochenek, J. Zalewski, J. Sadowski and A. Undas, Type 2 diabetes as a modifier of fibrin clot properties in patients with coronary artery disease, J. Thromb. Thrombolysis, 2013, 35, 264–270 CrossRef CAS PubMed.
- A. L. Cilia La Corte, H. Philippou and R. A. S. Ariëns, Role of fibrin structure in thrombosis and vascular disease, Adv. Protein Chem. Struct. Biol., 2011, 83, 75–127 Search PubMed.
- A. Undas and R. A. S. Ariëns, Fibrin clot structure and function: a role in the pathophysiology of arterial and venous thromboembolic diseases, Arterioscler., Thromb., Vasc. Biol., 2011, 31, e88–e99 CrossRef CAS PubMed.
- A. Undas, Acquired dysfibrinogenemia in atherosclerotic vascular disease, Pol. Arch. Med. Wewn., 2011, 121, 310–319 CAS.
- Z. de Lange, M. Pieters, J. C. Jerling, A. Kruger and D. C. Rijken, Plasma clot lysis time and its association with cardiovascular risk factors in black Africans, PLoS One, 2012, 7, e48881 CAS.
- R. A. S. Ariëns, Fibrin(ogen) and thrombotic disease, J. Thromb. Haemostasis, 2013, 11(suppl 1), 294–305 CrossRef PubMed.
- C. Alessandri, S. Basili, F. Violi, P. Ferroni, P. P. Gazzaniga and C. Cordova, Hypercoagulability state in patients with chronic obstructive pulmonary disease. Chronic Obstructive Bronchitis and Haemostasis Group, Thromb. Haemostasis, 1994, 72, 343–346 CAS.
- U. P. Kodavanti, M. C. Schladweiler, A. D. Ledbetter, R. V. Ortuno, M. Suffia, P. Evansky, J. H. Richards, R. H. Jaskot, R. Thomas, E. Karoly, Y. C. Huang, D. L. Costa, P. S. Gilmour and K. E. Pinkerton, The spontaneously hypertensive rat: an experimental model of sulfur dioxide-induced airways disease, Toxicol. Sci., 2006, 94, 193–205 CrossRef CAS PubMed.
- F. L. Fimognari, S. Scarlata, M. E. Conte and R. A. Incalzi, Mechanisms of atherothrombosis in chronic obstructive pulmonary disease, Int. J. Chronic Obstruct. Pulm. Dis., 2008, 3, 89–96 Search PubMed.
- A. Undas, P. Kaczmarek, K. Sladek, E. Stępień, W. Skucha, M. Rzeszutko, I. Gorkiewicz-Kot and W. Tracz, Fibrin clot properties are altered in patients with chronic obstructive pulmonary disease. Beneficial effects of simvastatin treatment, Thromb. Haemostasis, 2009, 102, 1176–1182 CAS.
- C. Erem, I. Nuhoglu, M. Yilmaz, M. Kocak, A. Demirel, O. Ucuncu and H. O. Ersoz, Blood coagulation and fibrinolysis in patients with Cushing's syndrome: Increased plasminogen activator inhibitor-1, decreased tissue factor pathway inhibitor, and unchanged thrombin-activatable fibrinolysis inhibitor levels, J. Endocrinol. Invest., 2009, 32, 169–174 CrossRef CAS.
- B. Van Zaane, E. Nur, A. Squizzato, O. M. Dekkers, M. T. Twickler, E. Fliers, V. E. Gerdes, H. R. Buller and D. P. Brandjes, Hypercoagulable state in Cushing's syndrome: a systematic review, J. Clin. Endocrinol. Metab., 2009, 94, 2743–2750 CrossRef CAS PubMed.
- D. Kastelan, T. Dusek, I. Kraljevic, O. Polasek, Z. Giljevic, M. Solak, S. Z. Salek, J. Jelcic, I. Aganovic and M. Korsic, Hypercoagulability in Cushing's syndrome: the role of specific haemostatic and fibrinolytic markers, Endocrine, 2009, 36, 70–74 CrossRef CAS PubMed.
- L. Trementino, G. Arnaldi, G. Appolloni, V. Daidone, C. Scaroni, A. Casonato and M. Boscaro, Coagulopathy in Cushing's Syndrome, Neuroendocrinology, 2010, 92, 55–59 CrossRef CAS PubMed.
- R. van der Pas, C. de Bruin, F. W. Leebeek, M. P. de Maat, D. C. Rijken, A. M. Pereira, J. A. Romijn, R. T. Netea-Maier, A. R. Hermus, P. M. Zelissen, F. H. de Jong, A. J. van der Lely, W. W. de Herder, S. W. Lamberts, L. J. Hofland and R. A. Feelders, The hypercoagulable state in Cushing's disease is associated with increased levels of procoagulant factors and impaired fibrinolysis, but is not reversible after short-term biochemical remission induced by medical therapy, J. Clin. Endocrinol. Metab., 2012, 97, 1303–1310 CrossRef CAS PubMed.
- D. Kastelan, T. Dusek, I. Kraljevic and I. Aganovic, Hypercoagulable state in Cushing's syndrome is reversible following remission, Clin. Endocrinol., 2013, 78, 102–106 CrossRef CAS PubMed.
- R. van der Pas, F. W. G. Leebeek, L. J. Hofland, W. W. de Herder and R. A. Feelders, Hypercoagulability in Cushing's syndrome: prevalence, pathogenesis and treatment, Clin. Endocrinol., 2013, 78, 481–488 CrossRef CAS PubMed.
- J. H. Smalberg, M. J. Kruip, H. L. Janssen, D. C. Rijken, F. W. Leebeek and M. P. de Maat, Hypercoagulability and hypofibrinolysis and risk of deep vein thrombosis and splanchnic vein thrombosis: similarities and differences, Arterioscler., Thromb., Vasc. Biol., 2011, 31, 485–493 CrossRef CAS PubMed.
- C. J. Glueck, J. Friedman, A. Hafeez, A. Hassan and P. Wang, Testosterone, thrombophilia, thrombosis, Blood Coagulation Fibrinolysis, 2014, 25, 683–687 CrossRef CAS PubMed.
- G. Cesarman-Maus and K. A. Hajjar, Molecular mechanisms of fibrinolysis, Br. J. Haematol., 2005, 129, 307–321 CrossRef CAS PubMed.
- T. Lisman, P. G. de Groot, J. C. Meijers and F. R. Rosendaal, Reduced plasma fibrinolytic potential is a risk factor for venous thrombosis, Blood, 2005, 105, 1102–1105 CrossRef CAS PubMed.
- M. E. Carr, Diabetes mellitus: a hypercoagulable state, J. Diabetes Complications, 2001, 15, 44–54 CrossRef CAS.
- J. D. Banga, Coagulation and fibrinolysis in diabetes, Semin. Vasc. Med., 2002, 2, 75–86 CrossRef CAS PubMed.
- R. Aras, J. R. Sowers and R. Arora, The proinflammatory and hypercoagulable state of diabetes mellitus, Rev. Cardiovasc. Med., 2005, 6, 84–97 Search PubMed.
- P. J. Grant, Diabetes mellitus as a prothrombotic condition, J. Intern. Med., 2007, 262, 157–172 CrossRef CAS PubMed.
- A. Tripodi, A. Branchi, V. Chantarangkul, M. Clerici, G. Merati, A. Artoni and P. M. Mannucci, Hypercoagulability in patients with type 2 diabetes mellitus detected by a thrombin generation assay, J. Thromb. Thrombolysis, 2011, 31, 165–172 CrossRef CAS PubMed.
- Y. Ye, J. R. Perez-Polo, D. Aguilar and Y. Birnbaum, The potential effects of anti-diabetic medications on myocardial ischemia-reperfusion injury, Basic Res. Cardiol., 2011, 106, 925–952 CrossRef CAS PubMed.
- H. J. B. H. Beijers, I. Ferreira, H. M. H. Spronk, B. Bravenboer, J. M. Dekker, G. Nijpels, H. ten Cate and C. D. A. Stehouwer, Impaired glucose metabolism and type 2 diabetes are associated with hypercoagulability: potential role of central adiposity and low-grade inflammation--the Hoorn Study, Thromb. Res., 2012, 129, 557–562 CrossRef CAS PubMed.
- M. Cucuianu, T. Fekete, C. Marcusiu, R. Mosler and A. Dutu, Fibrinolysis in diabetes mellitus. Role of overweight and hypertriglyceridemia, Med. Interne, 1984, 22, 171–177 CAS.
- Y. Yano, N. Kitagawa, E. C. Gabazza, K. Morioka, H. Urakawa, T. Tanaka, A. Katsuki, R. Araki-Sasaki, Y. Hori, K. Nakatani, O. Taguchi, Y. Sumida and Y. Adachi, Increased plasma thrombin-activatable fibrinolysis inhibitor levels in normotensive type 2 diabetic patients with microalbuminuria, J. Clin. Endocrinol. Metab., 2003, 88, 736–741 CrossRef CAS PubMed.
- E. J. Dunn, R. A. S. Ariëns and P. J. Grant, The influence of type 2 diabetes on fibrin structure and function, Diabetologia, 2005, 48, 1198–1206 CrossRef CAS PubMed.
- E. J. Dunn, H. Philippou, R. A. S. Ariëns and P. J. Grant, Molecular mechanisms involved in the resistance of fibrin to clot lysis by plasmin in subjects with type 2 diabetes mellitus, Diabetologia, 2006, 49, 1071–1080 CrossRef CAS PubMed.
- S. H. Alzahrani, K. Hess, J. F. Price, M. Strachan, P. D. Baxter, R. Cubbon, F. Phoenix, T. Gamlen, R. A. S. Ariëns, P. J. Grant and R. A. Ajjan, Gender-specific alterations in fibrin structure function in type 2 diabetes: associations with cardiometabolic and vascular markers, J. Clin. Endocrinol. Metab., 2012, 97, E2282–E2287 CrossRef CAS PubMed.
- K. Hess, S. H. Alzahrani, M. Mathai, V. Schroeder, A. M. Carter, G. Howell, T. Koko, M. W. Strachan, J. F. Price, K. A. Smith, P. J. Grant and R. A. Ajjan, A novel mechanism for hypofibrinolysis in diabetes: the role of complement C3, Diabetologia, 2012, 55, 1103–1113 CrossRef CAS PubMed.
- M. Walus-Miarka, P. Wolkow, K. Cyganek, B. Mirkiewicz-Sieradzka, M. T. Malecki and A. Undas, Altered fibrin-clot properties are associated with retinopathy in type 2 diabetes mellitus, Diabetes Metab., 2012, 38, 462–465 CAS.
- M. Konieczynska, K. Fil, M. Bazanek and A. Undas, Prolonged duration of type 2 diabetes is associated with increased thrombin generation, prothrombotic fibrin clot phenotype and impaired fibrinolysis, Thromb. Haemostasis, 2014, 111, 685–693 CrossRef CAS PubMed.
- A. Nair, B. Sealove, J. L. Halperin, G. Webber and V. Fuster, Anticoagulation in patients with heart failure: who, when, and why?, Eur. Heart J. Suppl., 2006, 8, E32–E38 CrossRef PubMed.
- A. Mongirdienė, L. Kuršvietienė and A. Kašauskas, The coagulation system changes in patients with chronic heart failure, Medicina, 2010, 46, 642–647 Search PubMed.
- V. G. Nielsen, J. K. Kirklin, W. L. Holman, B. L. Steenwyk, J. F. George, F. Zhou, D. A. Parks and T. C. Ellis, Mechanical circulatory device thrombosis: a new paradigm linking hypercoagulation and hypofibrinolysis, ASAIO J., 2008, 54, 351–358 CrossRef PubMed.
- G. Twig, G. Zandman-Goddard, M. Szyper-Kravitz and Y. Shoenfeld, Systemic thromboembolism in inflammatory bowel disease: mechanisms and clinical applications, Ann. N. Y. Acad. Sci., 2005, 1051, 166–173 CrossRef CAS PubMed.
- S. Danese, A. Papa, S. Saibeni, A. Repici, A. Malesci and M. Vecchi, Inflammation and coagulation in inflammatory bowel disease: the clot thickens, Am. J. Gastroenterol., 2007, 102, 174–186 CrossRef CAS PubMed.
- H. Alkim, S. Ayaz, C. Alkim, A. Ulker and B. Sahin, Continuous active state of coagulation system in patients with nonthrombotic inflammatory bowel disease, Clin. Appl. Thromb./Hemostasis, 2011, 17, 600–604 CrossRef CAS PubMed.
- M. Lazzerini, M. Bramuzzo, M. Maschio, S. Martelossi and A. Ventura, Thromboembolism in Pediatric Inflammatory Bowel Disease: Systematic Review, Inflammatory Bowel Dis., 2011, 17, 2174–2183 CrossRef PubMed.
- A. Deutschmann, A. Schlagenhauf, B. Leschnik, K. M. Hoffmann, A. Hauer and W. Muntean, Increased Procoagulant Function of Microparticles in Pediatric Inflammatory Bowel Disease: Role in Increased Thrombin Generation, J. Pediatr. Gastroenterol. Nutr., 2013, 56, 401–407 CrossRef CAS PubMed.
- C. M. Nylund, A. Goudie, J. M. Garza, G. Crouch and L. A. Denson, Venous thrombotic events in hospitalized children and adolescents with inflammatory bowel disease, J. Pediatr. Gastroenterol. Nutr., 2013, 56, 485–491 CrossRef PubMed.
- D. Kohoutova, M. Pecka, M. Cihak, J. Cyrany, J. Maly and J. Bures, Prevalence of hypercoagulable disorders in inflammatory bowel disease, Scand. J. Gastroenterol., 2014, 49, 287–294 CrossRef CAS PubMed.
- D. Owczarek, D. Cibor, M. K. Glowacki, T. Rodacki and T. Mach, Inflammatory bowel disease: epidemiology, pathology and risk factors for hypercoagulability, World J. Gastroenterol., 2014, 20, 53–63 CrossRef CAS PubMed.
- J. C. Gris, J. F. Schved, C. Raffanel, A. Dubois, P. Aguilar-Martinez, A. Arnaud, N. Sanchez, C. Sarlat and J. L. Balmes, Impaired fibrinolytic capacity in patients with inflammatory bowel disease, Thromb. Haemostasis, 1990, 63, 472–475 CAS.
- E. de Jong, R. J. Porte, E. A. Knot, J. H. Verheijen and J. Dees, Disturbed fibrinolysis in patients with inflammatory bowel disease. A study in blood plasma, colon mucosa, and faeces, Gut, 1989, 30, 188–194 CrossRef CAS.
- J. Shen, Z. H. Ran, Y. Zhang, Q. Cai, H. M. Yin, X. T. Zhou and S. D. Xiao, Biomarkers of altered coagulation and fibrinolysis as measures of disease activity in active inflammatory bowel disease: a gender-stratified, cohort analysis, Thromb. Res., 2009, 123, 604–611 CrossRef CAS PubMed.
- D. Owczarek, D. Cibor, K. Salapa, M. K. Glowacki, T. Mach and A. Undas, Reduced plasma fibrin clot permeability and susceptibility to lysis in patients with inflammatory bowel disease: a novel prothrombotic mechanism, Inflammatory Bowel Dis., 2013, 19, 2616–2624 CrossRef PubMed.
- F. Dentali, E. Romualdi and W. Ageno, The metabolic syndrome and the risk of thrombosis, Haematologica, 2007, 92, 297–299 CrossRef CAS.
- M.-C. Alessi and I. Juhan-Vague, Metabolic syndrome, haemostasis and thrombosis, Thromb. Haemostasis, 2008, 99, 995–1000 CAS.
- D. Vykoukal and M. G. Davies, Vascular biology of metabolic syndrome, J. Vasc. Surg., 2011, 54, 819–831 CrossRef PubMed.
- F. Dentali, M. N. di Minno, M. Gianni, G. di Minno, A. Squizzato and W. Ageno, The role of the metabolic syndrome in patients with provoked venous thromboembolic events, Thromb. Haemostasis, 2013, 109, 759–761 CrossRef CAS PubMed.
- S. He, K. Bremme and M. Blomback, Acquired deficiency of antithrombin in association with a hypercoagulable state and impaired function of liver and/or kidney in preeclampsia, Blood Coagulation Fibrinolysis, 1997, 8, 232–238 CrossRef CAS PubMed.
- A. Kher, R. Bauersachs and J. D. Nielsen, The management of thrombosis in pregnancy: role
of low-molecular-weight heparin, Thromb. Haemostasis, 2007, 97, 505–513 CAS.
- A. S. Ducloy-Bouthors, Clotting disorders and preeclampsia (in French), Ann. Fr. Anesth. Reanim., 2010, 29, e121–e134 CrossRef PubMed.
- L. M. Dusse, D. R. A. Rios, M. B. Pinheiro, A. J. Cooper and B. A. Lwaleed, Pre-eclampsia: relationship between coagulation, fibrinolysis and inflammation, Clin. Chim. Acta, 2011, 412, 17–21 CrossRef CAS PubMed.
- C. W. G. Redman, D. S. Tannetta, R. A. Dragovic, C. Gardiner, J. H. Southcombe, G. P. Collett and I. L. Sargent, Review: Does size matter? Placental debris and the pathophysiology of pre-eclampsia, Placenta, 2012, 33(suppl), S48–S54 CrossRef PubMed.
- M. A. Martínez-Zamora, D. Tassies, F. Carmona, G. Espinosa, R. Cervera, J. C. Reverter and J. Balasch, Clot lysis time and thrombin activatable fibrinolysis inhibitor in severe preeclampsia with or without associated antiphospholipid antibodies, J. Reprod. Immunol., 2010, 86, 133–140 CrossRef PubMed.
- C. J. Glueck, R. A. Freiberg, R. N. Fontaine, T. Tracy and P. Wang, Hypofibrinolysis, thrombophilia, osteonecrosis, Clin. Orthop. Relat. Res., 2001, 19–33 CrossRef CAS PubMed.
- A. P. Cellai, D. Lami, S. Fedi, R. Marcucci, L. Mannini, C. Cenci, A. Rogolino, A. Sodi, U. Menchini, R. Abbate and D. Prisco, A hypercoagulable and hypofibrinolytic state is detectable by global methods in patients with retinal vein occlusion, Atherosclerosis, 2012, 224, 97–101 CrossRef CAS PubMed.
- I. Karska-Basta, A. Kubicka-Trząska, B. Romanowska-Dixon and A. Undas, Altered fibrin clot properties in patients with retinal vein occlusion, J. Thromb. Haemostasis, 2011, 9, 2513–2515 CrossRef CAS PubMed.
- Y. Mukubo and M. Kawamata, Perioperative hypercoagulability in patients with rheumatoid arthritis: Sonoclot study, J. Anesth., 2004, 18, 62–64 CrossRef PubMed.
- Y. Shoenfeld, R. Gerli, A. Doria, E. Matsuura, M. M. Cerinic, N. Ronda, L. J. Jara, M. Abu-Shakra, P. L. Meroni and Y. Sherer, Accelerated atherosclerosis in autoimmune rheumatic diseases, Circulation, 2005, 112, 3337–3347 CrossRef PubMed.
- R. J. Bisoendial, E. S. G. Stroes and P. P. Tak, Where the immune response meets the vessel wall, Neth. J. Med., 2009, 67, 328–333 CAS.
- R. J. Bisoendial, M. Levi, P. P. Tak and E. S. G. Stroes, The prothrombotic state in rheumatoid arthritis: an additive risk factor for adverse cardiovascular events, Semin. Thromb. Hemostasis, 2010, 36, 452–457 CrossRef CAS PubMed.
- R. J. Bisoendial, E. S. G. Stroes, J. J. Kastelein and P. P. Tak, Targeting cardiovascular risk in rheumatoid arthritis: a dual role for statins, Nat. Rev. Rheumatol., 2010, 6, 157–164 CrossRef CAS PubMed.
- R. J. Bisoendial, E. S. G. Stroes and P. P. Tak, Critical determinants of cardiovascular risk in rheumatoid arthritis, Curr. Pharm. Des., 2011, 17, 21–26 CrossRef CAS.
- W. S. Chung, C. L. Peng, C. L. Lin, Y. J. Chang, Y. F. Chen, J. Y. Chiang, F. C. Sung and C. H. Kao, Rheumatoid arthritis increases the risk of deep vein thrombosis and pulmonary thromboembolism: a nationwide cohort study, Ann. Rheum. Dis., 2014, 73, 1774–1780 CrossRef PubMed.
- J. F. Hesselvik, M. Blomback, B. Brodin and R. Maller, Coagulation, fibrinolysis, and kallikrein systems in sepsis: relation to outcome, Crit. Care Med., 1989, 17, 724–733 CrossRef CAS PubMed.
- G. R. Bernard, E. W. Ely, T. J. Wright, J. Fraiz, J. E. Stasek, Jr., J. A. Russell, I. Mayers, B. A. Rosenfeld, P. E. Morris, S. B. Yan and J. D. Helterbrand, Safety and dose relationship of recombinant human activated protein C for coagulopathy in severe sepsis, Crit. Care Med., 2001, 29, 2051–2059 CrossRef CAS PubMed.
- G. R. Bernard, J. L. Vincent, P. F. Laterre, S. P. LaRosa, J. F. Dhainaut, A. Lopez-Rodriguez, J. S. Steingrub, G. E. Garber, J. D. Helterbrand, E. W. Ely and C. J. Fisher, Jr., Efficacy and safety of recombinant human activated protein C for severe sepsis, N. Engl. J. Med., 2001, 344, 699–709 CrossRef CAS PubMed.
- M. A. Matthay, Severe sepsis--a new treatment with both anticoagulant and antiinflammatory properties, N. Engl. J. Med., 2001, 344, 759–762 CrossRef CAS PubMed.
- S. M. Opal and C. T. Esmon, Bench-to-bedside review: functional relationships between coagulation and the innate immune response and their respective roles in the pathogenesis of sepsis, Crit. Care, 2003, 7, 23–38 CrossRef.
- S. Biswal and D. G. Remick, Sepsis: redox mechanisms and therapeutic opportunities, Antioxid. Redox Signaling, 2007, 9, 1959–1961 CrossRef CAS PubMed.
- D. G. Remick, Pathophysiology of sepsis, Am. J. Pathol., 2007, 170, 1435–1444 CrossRef CAS PubMed.
- R. J. Langley, E. L. Tsalik, J. C. van Velkinburgh, S. W. Glickman, B. J. Rice, C. Wang, B. Chen, L. Carin, A. Suarez, R. P. Mohney, D. H. Freeman, M. Wang, J. You, J. Wulff, J. W. Thompson, M. A. Moseley, S. Reisinger, B. T. Edmonds, B. Grinnell, D. R. Nelson, D. L. Dinwiddie, N. A. Miller, C. J. Saunders, S. S. Soden, A. J. Rogers, L. Gazourian, L. E. Fredenburgh, A. F. Massaro, R. M. Baron, A. M. Choi, G. R. Corey, G. S. Ginsburg, C. B. Cairns, R. M. Otero, V. G. Fowler, Jr., E. P. Rivers, C. W. Woods and S. F. Kingsmore, An integrated clinico-metabolomic model improves prediction of death in sepsis, Sci. Transl. Med., 2013, 5, 195ra195 Search PubMed.
- A. P. Fletcher, N. Alkjaersig, A. Davies, M. Lewis, J. Brooks, W. Hardin, W. Landau and M. E. Raichle, Blood coagulation and plasma fibrinolytic enzyme system pathophysiology in stroke, Stroke, 1976, 7, 337–348 CrossRef CAS.
- G. Landi, A. D'Angelo, E. Boccardi, L. Candelise, P. M. Mannucci, E. Nobile Orazio and A. Morabito, Hypercoagulability in acute stroke: prognostic significance, Neurology, 1987, 37, 1667–1671 CrossRef CAS.
- K. Takano, T. Yamaguchi, Y. Okada, K. Uchida, W. Kisiel and H. Kato, Hypercoagulability in acute ischemic stroke: analysis of the extrinsic coagulation reactions in plasma by a highly sensitive automated method, Thromb. Res., 1990, 58, 481–491 CrossRef CAS.
- N. Matijevic and K. K. Wu, Hypercoagulable states and strokes, Curr. Atheroscler. Rep., 2006, 8, 324–329 CrossRef CAS PubMed.
- T. J. Bernard, L. Z. Fenton, S. D. Apkon, R. Boada, G. N. Wilkening, C. C. Wilkinson, J. B. Soep, S. D. Miyamoto, M. Tripputi, J. Armstrong-Wells, T. A. Benke, M. J. Manco-Johnson and N. A. Goldenberg, Biomarkers of hypercoagulability and inflammation in childhood-onset arterial ischemic stroke, J. Pediatr., 2010, 156, 651–656 CrossRef CAS PubMed.
- K. L. Furie, S. E. Kasner, R. J. Adams, G. W. Albers, R. L. Bush, S. C. Fagan, J. L. Halperin, S. C. Johnston, I. Katzan, W. N. Kernan, P. H. Mitchell, B. Ovbiagele, Y. Y. Palesch, R. L. Sacco, L. H. Schwamm, S. Wassertheil-Smoller, T. N. Turan and D. Wentworth, Guidelines for the prevention of stroke in patients with stroke or transient ischemic attack: a guideline for healthcare professionals from the American heart association/American stroke association, Stroke, 2011, 42, 227–276 CrossRef PubMed.
- P. Martínez-Sánchez, M. Martínez-Martínez, B. Fuentes, M. V. Cuesta, L. Cuéllar-Gamboa, L. Idrovo-Freire, J. Fernández-Dominguez and E. Diéz-Tejedor, Migraine and hypercoagulable states in ischemic stroke, Cephalalgia, 2011, 31, 1609–1617 CrossRef PubMed.
- B. Kristensen, J. Malm, T. K. Nilsson, J. Hultdin, B. Carlberg and T. Olsson, Increased fibrinogen levels and acquired hypofibrinolysis in young adults with ischemic stroke, Stroke, 1998, 29, 2261–2267 CrossRef CAS.
- B. Kristensen, J. Malm, T. K. Nilsson, J. Hultdin, B. Carlberg, G. Dahlén and T. Olsson, Hyperhomocysteinemia and hypofibrinolysis in young adults with ischemic stroke, Stroke, 1999, 30, 974–980 CrossRef CAS.
- B. Lipinski, E. Pretorius, H. M. Oberholzer and W. J. Van Der Spuy, Iron enhances generation of fibrin fibers in human blood: Implications for pathogenesis of stroke, Microsc. Res. Tech., 2012, 75, 1185–1190 CrossRef CAS PubMed.
- B. Lipinski, E. Pretorius, H. M. Oberholzer and W. J. van der Spuy, Interaction of fibrin with red blood cells: the role of iron, Ultrastruct. Pathol., 2012, 36, 79–84 CrossRef PubMed.
- E. Pretorius, H. Steyn, M. Engelbrecht, A. C. Swanepoel and H. M. Oberholzer, Differences in fibrin fiber diameters in healthy individuals and thromboembolic ischemic stroke patients, Blood Coagulation Fibrinolysis, 2011, 22, 696–700 CrossRef PubMed.
- E. Pretorius and B. Lipinski, Differences in morphology of fibrin clots induced with thrombin and ferric ions and its pathophysiological consequences, Heart, Lung Circ., 2013, 22, 447–449 CrossRef CAS PubMed.
- E. Rooth, N. H. Wallen, M. Blomback and S. He, Decreased fibrin network permeability and impaired fibrinolysis in the acute and convalescent phase of ischemic stroke, Thromb. Res., 2011, 127, 51–56 CrossRef CAS PubMed.
- A. Undas, P. Podolec, K. Zawilska, M. Pieculewicz, I. Jedliński, E. Stępień, E. Konarska-Kuszewska, P. Weglarz, M. Duszynska, E. Hanschke, T. Przewlocki and W. Tracz, Altered fibrin clot structure/function in patients with cryptogenic ischemic stroke, Stroke, 2009, 40, 1499–1501 CrossRef CAS PubMed.
- S. M. Abo and V. A. DeBari, Laboratory evaluation of the antiphospholipid syndrome, Ann. Clin. Lab. Sci., 2007, 37, 3–14 CAS.
- F. R. Dembitzer, M. R. Ledford Kraemer, P. Meijer and E. I. Peerschke, Lupus anticoagulant testing: performance and practices by North American clinical laboratories, Am. J. Clin. Pathol., 2010, 134, 764–773 CrossRef CAS PubMed.
- M. Jurado, J. A. Paramo, M. Gutierrez-Pimentel and E. Rocha, Fibrinolytic potential and antiphospholipid antibodies in systemic lupus erythematosus and other connective tissue disorders, Thromb. Haemostasis, 1992, 68, 516–520 CAS.
- K. A. Krone, K. L. Allen and K. R. McCrae, Impaired fibrinolysis in the antiphospholipid syndrome, Curr. Rheumatol. Rep., 2010, 12, 53–57 CrossRef CAS PubMed.
- J. M. P. de Godoy, M. F. de Godoy and D. M. Braile, Recurrent thrombosis in patients with deep vein thrombosis and/or venous thromboembolism associated with anticardiolipin antibodies, Angiology, 2006, 57, 79–83 CrossRef PubMed.
- J. A. Caprini, Risk assessment as a guide for the prevention of the many faces of venous thromboembolism, Am. J. Surg., 2010, 199, S3–S10 CrossRef PubMed.
- M. Bern and N. McCarthy, Multiple markers of hypercoagulation in patients with history of venous
thromboembolic disease, Blood Coagulation Fibrinolysis, 2013, 24, 59–63 CrossRef CAS PubMed.
- R. M. Van Haren, E. J. Valle, C. M. Thorson, J. M. Jouria, A. M. Busko, G. A. Guarch, N. Namias, A. S. Livingstone and K. G. Proctor, Hypercoagulability and other risk factors in trauma intensive care unit patients with venous thromboembolism, J. Trauma Acute Care Surg., 2014, 76, 443–449 CrossRef CAS PubMed.
- M. M. Aleman, B. L. Walton, J. R. Byrnes and A. S. Wolberg, Fibrinogen and red blood cells in venous thrombosis, Thromb. Res., 2014, 133(suppl 1), S38–S40 CrossRef PubMed.
- J. Skov, J. J. Sidelmann, E. M. Bladbjerg, J. Jespersen and J. Gram, Difference in fibrinolytic capacity in young patients with venous thrombosis or ischaemic stroke, Blood Coagulation Fibrinolysis, 2014, 25, 61–66 CrossRef CAS PubMed.
- H. Watanabe, I. Kikkawa, S. Madoiwa, H. Sekiya, S. Hayasaka and Y. Sakata, Changes in blood coagulation-fibrinolysis markers by pneumatic tourniquet during total knee joint arthroplasty with venous thromboembolism, J. Arthroplasty, 2014, 29, 569–573 CrossRef PubMed.
- M. S. Siket and J. Edlow, Transient ischemic attack: an evidence-based update, Emerg. Med. Pract., 2013, 15, 1–26 Search PubMed.
- D. J. Dries, Activation of the clotting system and complement after trauma, New Horiz., 1996, 4, 276–288 CAS.
- D. T. Engelman, S. G. Gabram, L. Allen, G. E. Ens and L. M. Jacobs, Hypercoagulability following multiple trauma, World J. Surg., 1996, 20, 5–10 CrossRef CAS.
- J. R. Hess, K. Brohi, R. P. Dutton, C. J. Hauser, J. B. Holcomb, Y. Kluger, K. Mackway-Jones, M. J. Parr, S. B. Rizoli, T. Yukioka, D. B. Hoyt and B. Bouillon, The coagulopathy of trauma: a review of mechanisms, J. Trauma, 2008, 65, 748–754 CrossRef CAS PubMed.
- R. Selby, W. Geerts, F. A. Ofosu, S. Craven, L. Dewar, A. Phillips and J. P. Szalai, Hypercoagulability after trauma: hemostatic changes and relationship to venous thromboembolism, Thromb. Res., 2009, 124, 281–287 CrossRef CAS PubMed.
- J. A. Differding, S. J. Underwood, P. Y. Van, R. A. Khaki, N. J. Spoerke and M. A. Schreiber, Trauma induces a hypercoagulable state that is resistant to hypothermia as measured by thrombelastogram, Am. J. Surg., 2011, 201, 587–591 CrossRef PubMed.
- C. T. Esmon and N. L. Esmon, The link between vascular features and thrombosis, Annu. Rev. Physiol., 2011, 73, 503–514 CrossRef CAS PubMed.
- M. S. Park, B. A. Owen, B. A. Ballinger, M. G. Sarr, H. J. Schiller, S. P. Zietlow, D. H. Jenkins, M. H. Ereth, W. G. Owen and J. A. Heit, Quantification of hypercoagulable state after blunt trauma: microparticle and thrombin generation are increased relative to injury severity, while standard markers are not, Surgery, 2012, 151, 831–836 CrossRef PubMed.
- B. C. Branco, K. Inaba, C. Ives, O. Okoye, I. Shulman, J. S. David, H. Schöchl, P. Rhee and D. Demetriades, Thromboelastogram evaluation of the impact of hypercoagulability in trauma patients, Shock, 2014, 41, 200–207 CrossRef PubMed.
- D. N. Kapsch, M. Metzler, M. Harrington, F. L. Mitchell and D. Silver, Fibrinolytic response to trauma, Surgery, 1984, 95, 473–478 CAS.
- C. Kluft, J. H. Verheijen, A. F. Jie, D. C. Rijken, F. E. Preston, H. M. Sue-Ling, J. Jespersen and A. O. Aasen, The postoperative fibrinolytic shutdown: a rapidly reverting acute
phase pattern for the fast-acting inhibitor of tissue-type plasminogen activator after trauma, Scand. J. Clin. Lab. Invest., 1985, 45, 605–610 CrossRef CAS PubMed.
- B. L. Enderson, J. P. Chen, R. Robinson and K. I. Maull, Fibrinolysis in multisystem trauma patients, J. Trauma, 1991, 31, 1240–1246 CrossRef CAS PubMed.
- M. D. Cappellini, L. Robbiolo, B. M. Bottasso, R. Coppola, G. Fiorelli and A. P. Mannucci, Venous thromboembolism and hypercoagulability in splenectomized patients with thalassaemia intermedia, Br. J. Haematol., 2000, 111, 467–473 CrossRef CAS.
- A. Eldor and E. A. Rachmilewitz, The hypercoagulable state in thalassemia, Blood, 2002, 99, 36–43 CrossRef CAS PubMed.
- K. I. Ataga, M. D. Cappellini and E. A. Rachmilewitz, Beta-thalassaemia and sickle cell anaemia as paradigms of hypercoagulability, Br. J. Haematol., 2007, 139, 3–13 CrossRef CAS PubMed.
- M. Bhattacharyya, M. Kannan, V. P. Chaudhry, M. Mahapatra, H. Pati and R. Saxena, Hypercoagulable state in five thalassemia intermedia patients, Clin. Appl. Thromb./Hemostasis, 2007, 13, 422–427 CrossRef CAS PubMed.
- A. T. Taher, Z. K. Otrock, I. Uthman and M. D. Cappellini, Thalassemia and hypercoagulability, Blood Rev., 2008, 22, 283–292 CrossRef PubMed.
- M. Karimi, H. Bagheri, F. Rastgu and E. A. Rachmilewitz, Magnetic resonance imaging to determine the incidence of brain ischaemia in patients with beta-thalassaemia intermedia, Thromb. Haemostasis, 2010, 103, 989–993 CrossRef CAS PubMed.
- A. T. Taher, K. M. Musallam and A. Inati, The hypercoagulable state in thalassemia intermedia, Hemoglobin, 2009, 33(suppl 1), S160–S169 CrossRef CAS PubMed.
- A. Tripodi, M. D. Cappellini, V. Chantarangkul, L. Padovan, M. R. Fasulo, A. Marcon and P. M. Mannucci, Hypercoagulability in splenectomized thalassemic patients detected by whole-blood thromboelastometry, but not by thrombin generation in platelet-poor plasma, Haematologica, 2009, 94, 1520–1527 CrossRef CAS PubMed.
- M. D. Cappellini, I. Motta, K. M. Musallam and A. T. Taher, Redefining thalassemia as a hypercoagulable state, Ann. N. Y. Acad. Sci., 2010, 1202, 231–236 CrossRef CAS PubMed.
- M. D. Cappellini, K. M. Musallam and A. T. Taher, Thalassaemia as a hypercoagulable state, Touch Briefings, 2011, 214–216 Search PubMed.
- M. D. Cappellini, E. Poggiali, A. T. Taher and K. M. Musallam, Hypercoagulability in beta-thalassemia: a status quo, Expert Rev. Hematol., 2012, 5, 505–511 CrossRef CAS PubMed ; quiz 512.
- M. D. Cappellini, K. M. Musallam, E. Poggiali and A. T. Taher, Hypercoagulability in non-transfusion-dependent thalassemia, Blood Rev., 2012, 26(suppl 1), S20–S23 CrossRef.
- S. Haghpanah and M. Karimi, Cerebral thrombosis in patients with beta-thalassemia: a systematic review, Blood Coagulation Fibrinolysis, 2012, 23, 212–217 CrossRef CAS PubMed.
- S. Kemahli, C. Gürman, Y. Eğin, Y. Yildirmak, T. Sipahi, Z. Uysal, N. Akar, S. Cin and A. Arcasoy, Hypercoagulability in children with thalassemia major, Clin. Appl. Thromb./Hemostasis, 1997, 3, 129–132 CrossRef PubMed.
- G. M. Mokhtar, R. M. Matter, H. Shawki and M. M. Abdel Aziz, Thrombin activatable fibrinolysis inhibitor (TAFI): relationship to hemostatic alteration in patients with beta-thalassemia, Pediatr. Hematol. Oncol., 2010, 27, 363–373 CrossRef CAS PubMed.
- M. Cugno, A. Tedeschi, C. Crosti and A. V. Marzano, Activation of blood coagulation in autoimmune skin disorders, Expert Rev. Clin. Immunol., 2009, 5, 605–613 CrossRef CAS PubMed.
- M. Cugno, A. Tedeschi, R. Asero, P. L. Meroni and A. V. Marzano, Skin autoimmunity
and blood coagulation, Autoimmunity, 2010, 43, 189–194 CrossRef CAS PubMed.
- M. Gaeta, S. Castelvecchio, C. Ricci, P. Pigatto, G. Pellissero and R. Cappato, Role of psoriasis as independent predictor of cardiovascular disease: a meta-regression analysis, Int. J. Cardiol., 2013, 168, 2282–2288 CrossRef PubMed.
- A. J. Paidhungat, D. S. Salgaonkar, S. T. Nabar, M. Y. Nadkar, V. I. Chari, D. P. Khatri and S. R. Prabhu, Effect of inhaled terbutaline on platelet activity in bronchial asthma, J. Assoc. Physicians India, 1994, 42, 113–115 CAS.
- H. B. Pandit, C. R. Spillert and R. D. Shih, Determination of hypercoagulable state in acute bronchospasm, J. Am. Osteopath. Assoc., 1999, 99, 203–206 CAS.
- M. J. Utell and M. W. Frampton, Acute health effects of ambient air pollution: the ultrafine particle hypothesis, J. Aerosol Med., 2000, 13, 355–359 CrossRef CAS.
- R. M. Laryushkina, A. I. Ryvkin, T. G. Glazova, Y. Linkova, A. T. G. Reshetova, E. O. Safronova, T. B. Sokolova and I. K. Tomilova, Role of disorders in coagulofibrinolythic link of blood clotting and role of dyslipidemia in persistent course of pediatric bronchial asthma, Pediatriya, 2003, 6, 22–26 Search PubMed.
- E. Pretorius and H. M. Oberholzer, Ultrastructural changes of platelets and fibrin networks in human asthma: a qualitative case study, Blood Coagulation Fibrinolysis, 2009, 20, 146–149 CrossRef PubMed.
-
W. Burke, P. D. Phatak and E. D. Weinberg, The Iron Disorders Institute guide to hemochromatosis, Cumberland House, Nashville, TN, 2001 Search PubMed.
- G. T. Kinasewitz, J. G. Zein, G. L. Lee, S. A. Nazir and F. B. Taylor, Jr., Prognostic value of a simple evolving disseminated intravascular coagulation score in patients with severe sepsis, Crit. Care Med., 2005, 33, 2214–2221 CrossRef.
- M. N. O'Riordan and J. R. Higgins, Haemostasis in normal and abnormal pregnancy, Best Pract. Res. Clin. Obstet. Gynaecol., 2003, 17, 385–396 CrossRef.
- E. H. Uchikova and I. I. Ledjev, Changes in haemostasis during normal pregnancy, Eur. J. Obstet. Gynecol. Reprod. Biol., 2005, 119, 185–188 CrossRef CAS PubMed.
- P. B. Szecsi, M. Jorgensen, A. Klajnbard, M. R. Andersen, N. P. Colov and S. Stender, Haemostatic reference intervals in pregnancy, Thromb. Haemostasis, 2010, 103, 718–727 CrossRef CAS PubMed.
- P. Thornton and J. Douglas, Coagulation in pregnancy, Best practice & research, Clin. Obstet. Gynecol., 2010, 24, 339–352 Search PubMed.
- S. G. Deeks, R. Tracy and D. C. Douek, Systemic effects of inflammation on health during chronic HIV infection, Immunity, 2013, 39, 633–645 CrossRef CAS PubMed.
- B. Lipinski and E. Pretorius, Iron-induced fibrin in cardiovascular disease, Curr. Neurovasc. Res., 2013, 10, 269–274 CrossRef CAS.
- E. Pretorius, U. B. Windberger, H. M. Oberholzer and R. E. Auer, Comparative ultrastructure of fibrin networks of a dog after thrombotic ischaemic stroke, Onderstepoort J. Vet. Res., 2010, 77, E1–E4 Search PubMed.
- E. Pretorius, H. M. Oberholzer, W. J. van der Spuy and J. H. Meiring, The changed ultrastructure of fibrin networks during use of oral contraception and hormone replacement, J. Thromb. Thrombolysis, 2010, 30, 502–506 CrossRef CAS PubMed.
- E. Pretorius, The use of a desktop scanning electron microscope as a diagnostic tool in studying fibrin networks of thrombo-embolic ischemic stroke, Ultrastruct. Pathol., 2011, 35, 245–250 CrossRef PubMed.
- E. Pretorius, H. M. Oberholzer, W. J. van der Spuy, A. C. Swanepoel and P. Soma, Qualitative scanning electron microscopy analysis of fibrin networks and platelet abnormalities in diabetes, Blood Coagulation Fibrinolysis, 2011, 22, 463–467 CrossRef CAS PubMed.
- E. Pretorius, J. Olivier, H. M. Oberholzer and W. J. Van der Spuy, Scanning electron microscopy
investigation of fibrin networks after thermal injury, Onderstepoort J. Vet. Res., 2011, 78, 244 Search PubMed.
- E. Pretorius, N. Vermeulen, J. Bester, B. Lipinski and D. B. Kell, A novel method for assessing the role of iron and its functional chelation in fibrin fibril formation: the use of scanning electron microscopy, Toxicol. Mech. Methods, 2013, 23, 352–359 CrossRef CAS PubMed.
- E. Pretorius, J. Bester, N. Vermeulen, B. Lipinski, G. S. Gericke and D. B. Kell, Profound morphological changes in the erythrocytes and fibrin networks of patients with hemochromatosis or with hyperferritinemia, and their normalization by iron chelators and other agents, PLoS One, 2014, 9, e85271 Search PubMed.
- B. Blombäck, K. Carlsson, K. Fatah, B. Hessel and R. Procyk, Fibrin in human plasma: gel architectures governed by rate and nature of fibrinogen activation, Thromb. Res., 1994, 75, 521–538 CrossRef.
- B. Blombäck and N. Bark, Fibrinopeptides and fibrin gel structure, Biophys. Chem., 2004, 112, 147–151 CrossRef PubMed.
- J. H. Brown, N. Volkmann, G. Jun, A. H. Henschen-Edman and C. Cohen, The crystal structure of modified bovine fibrinogen, Proc. Natl. Acad. Sci. U. S. A., 2000, 97, 85–90 CrossRef CAS.
- M. W. Mosesson, Fibrinogen and fibrin structure and functions, J. Thromb. Haemostasis, 2005, 3, 1894–1904 CrossRef CAS PubMed.
- K. M. Weigandt, N. White, D. Chung, E. Ellingson, Y. Wang, X. Y. Fu and D. C. Pozzo, Fibrin Clot Structure and Mechanics Associated with Specific Oxidation of Methionine Residues in Fibrinogen, Biophys. J., 2012, 103, 2399–2407 CrossRef CAS PubMed.
- J. Danesh, R. Collins, P. Appleby and R. Peto, Association of fibrinogen, C-reactive protein, albumin, or leukocyte count with coronary heart disease: meta-analyses of prospective studies, JAMA, J. Am. Med. Assoc., 1998, 279, 1477–1482 CrossRef CAS.
- N. Ossei-Gerning, I. J. Wilson and P. J. Grant, Sex differences in coagulation and fibrinolysis in subjects with coronary artery disease, Thromb. Haemostasis, 1998, 79, 736–740 CAS.
- C. Bickel, H. J. Rupprecht, S. Blankenberg, C. Espiniola-Klein, A. Schlitt, G. Rippin, G. Hafner, R. Treude, H. Othman, K. P. Hofmann, J. Meyer and A. Investigators, Relation of markers of inflammation (C-reactive protein, fibrinogen, von Willebrand factor, and leukocyte count) and statin therapy to long-term mortality in patients with angiographically proven coronary artery disease, Am. J. Cardiol., 2002, 89, 901–908 CrossRef CAS.
- E. Réganon, V. Vila, V. Martínez-Sales, A. Vaya and J. Aznar, Inflammation, fibrinogen and thrombin generation in patients with previous myocardial infarction, Haematologica, 2002, 87, 740–745 Search PubMed.
- P. Jousilahti, V. Salomaa, V. Rasi, E. Vahtera and T. Palosuo, Association of markers of systemic inflammation, C reactive protein, serum amyloid A, and fibrinogen, with socioeconomic status, J. Epidemiol. Community Health, 2003, 57, 730–733 CrossRef CAS.
- C. Zoccali, F. A. Benedetto, F. Mallamaci, G. Tripepi, S. Cutrupi, S. Parlongo, L. S. Malatino, G. Bonanno, F. Rapisarda, P. Fatuzzo, G. Seminara, G. Nicocia and M. Buemi, Fibrinogen, inflammation and concentric left ventricular hypertrophy in chronic renal failure, Eur. J. Clin. Invest., 2003, 33, 561–566 CrossRef CAS.
- J. Danesh, S. Lewington, S. G. Thompson, G. D. O. Lowe, R. Collins and Fibrinogen Studies Collaboration, Plasma fibrinogen level and the risk of major cardiovascular diseases and nonvascular mortality – An individual participant meta-analysis, JAMA, J. Am. Med. Assoc., 2005, 294, 1799–1809 CAS.
- D. Green, C. Chan, J. Kang, K. Liu, P. Schreiner, N. S. Jenny and R. P. Tracy, Longitudinal assessment of fibrinogen in relation to subclinical cardiovascular disease: the CARDIA study, J. Thromb. Haemostasis, 2010, 8, 489–495 CrossRef CAS PubMed.
- D. Zapryanova, L. Lazarov, T. M. Georgieva, J. Nikolov, N. Goranov, I. Dinev and I. Stoycheva, Markers of inflammation in experimentally induced pancreatitis in dogs (Part III): Blood plasma protein profiles and fibrinogen, Rev. Med. Vet., 2011, 162, 449–453 CAS.
- D. Davalos and K. Akassoglou, Fibrinogen as a key regulator of inflammation in disease, Semin. Immunopathol., 2012, 34, 43–62 CrossRef CAS PubMed.
- M. Swarowska, A. Janowska, A. Polczak, A. Klimkowicz-Mrowiec, J. Pera, A. Slowik and T. Dziedzic, The Sustained Increase of Plasma Fibrinogen During Ischemic Stroke Predicts Worse Outcome Independently of Baseline Fibrinogen Level, Inflammation, 2014, 37, 1142–1147 CrossRef CAS PubMed.
- G. Jörneskog, N. Egberg, B. Fagrell, K. Fatah, B. Hessel, H. Johnsson, K. Brismar and M. Blombäck, Altered properties of the fibrin gel structure in patients with IDDM, Diabetologia, 1996, 39, 1519–1523 CrossRef.
- O. V. Kim, R. I. Litvinov, J. W. Weisel and M. S. Alber, Structural basis for the nonlinear mechanics of fibrin networks under compression, Biomaterials, 2014, 35, 6739–6749 CrossRef CAS PubMed.
- R. D. McBane, R. M. Hardison, 2nd and B. E. Sobel, Comparison of plasminogen activator inhibitor-1, tissue type plasminogen activator antigen, fibrinogen, and D-dimer levels in various age decades in patients with type 2 diabetes mellitus and stable coronary artery disease (from the BARI 2D trial), Am. J. Cardiol., 2010, 105, 17–24 CrossRef CAS PubMed.
- M. Okada and B. Blombäck, Calcium and fibrin gel structure, Thromb. Res., 1983, 29, 269–280 CrossRef CAS.
- M. Okada and B. Blomback, Factors influencing fibrin gel structure studied by flow measurement, Ann. N. Y. Acad. Sci., 1983, 408, 233–253 CrossRef CAS PubMed.
- H. Tran, A. Tanaka, S. V. Litvinovich, L. V. Medved, C. C. Haudenschild and W. S. Argraves, The interaction of fibulin-1 with fibrinogen. A potential role in hemostasis and thrombosis, J. Biol. Chem., 1995, 270, 19458–19464 CrossRef CAS PubMed.
- G. Marx, Zinc binding to fibrinogen and fibrin, Arch. Biochem. Biophys., 1988, 266, 285–288 CrossRef CAS.
- A. Sahni, M. Guo, S. K. Sahni and C. W. Francis, Interleukin-1beta but not IL-1alpha binds to fibrinogen and fibrin and has enhanced activity in the bound form, Blood, 2004, 104, 409–414 CrossRef CAS PubMed.
- J. W. Weisel, Fibrinogen and fibrin, Adv. Protein Chem., 2005, 70, 247–299 CrossRef CAS.
- A. Sahni, A. A. Khorana, R. B. Baggs, H. Peng and C. W. Francis, FGF-2 binding to fibrin(ogen) is required for augmented angiogenesis, Blood, 2006, 107, 126–131 CrossRef CAS PubMed.
- A. Sahni, P. J. Simpson-Haidaris, S. K. Sahni, G. G. Vaday and C. W. Francis, Fibrinogen synthesized by cancer cells augments the proliferative effect of fibroblast growth factor-2 (FGF-2), J. Thromb. Haemostasis, 2008, 6, 176–183 CrossRef CAS PubMed.
- A. R. Folsom, P. L. Lutsey, S. R. Heckbert and M. Cushman, Serum albumin and risk of venous thromboembolism, Thromb. Haemostasis, 2010, 104, 100–104 CrossRef CAS PubMed.
- K. Orino, Functional binding analysis of human fibrinogen as an iron- and heme-binding protein, BioMetals, 2013, 26, 789–794 CrossRef CAS PubMed.
- E. Pretorius, B. Lipinski, J. Bester, N. Vermeulen and P. Soma, Albumin stabilizes fibrin fiber ultrastructure in low serum albumin type 2 diabetes, Ultrastruct. Pathol., 2013, 37, 254–257 CrossRef PubMed.
- L. Thomer, O. Schneewind and D. Missiakas, Multiple ligands of von Willebrand factor-binding protein (vWbp) promote Staphylococcus aureus clot formation in human plasma, J. Biol. Chem., 2013, 288, 28283–28292 CrossRef CAS PubMed.
- J. W. Weisel and R. I. Litvinov, Mechanisms of fibrin polymerization and clinical implications, Blood, 2013, 121, 1712–1719 CrossRef CAS PubMed.
- A. Ramanathan and N. Karuri, Fibronectin alters the rate of formation and structure of the fibrin matrix, Biochem. Biophys. Res. Commun., 2014, 443, 395–399 CrossRef CAS PubMed.
- V. Reyhani, P. Seddigh, B. Guss, R. Gustafsson, L. Rask and K. Rubin, Fibrin binds to collagen and provides a bridge for alphaVbeta3 integrin-dependent contraction of collagen gels, Biochem. J., 2014, 462, 113–123 CrossRef CAS PubMed.
- C. M. Rowland, C. R. Pullinger, M. M. Luke, D. Shiffman, L. Green, I. Movsesyan, J. J. Devlin, M. J. Malloy, J. P. Kane and A. Undas, Lipoprotein (a), LPA Ile4399Met, and Fibrin Clot Properties, Thromb. Res., 2014, 133, 863–867 CrossRef CAS PubMed.
- J. Jankun, P. Landeta, E. Pretorius, E. Skrzypczak-Jankun and B. Lipinski, Unusual clotting dynamics of plasma supplemented with iron(III), Int. J. Mol. Med., 2014, 33, 367–372 CAS.
- B. Blombäck, Fibrinogen structure, activation, polymerization and fibrin gel structure, Thromb. Res., 1994, 75, 327–328 CrossRef.
- M. S. Kostelansky, K. C. Lounes, L. F. Ping, S. K. Dickerson, O. V. Gorkun and S. T. Lord, Calcium-binding site beta 2, adjacent to the “b” polymerization site, modulates lateral aggregation of protofibrils during fibrin polymerization, Biochemistry, 2004, 43, 2475–2483 CrossRef CAS PubMed.
- M. S. Kostelansky, K. C. Lounes, L. F. Ping, S. K. Dickerson, O. V. Gorkun and S. T. Lord, Probing the gamma2 calcium-binding site: studies with gammaD298,301A fibrinogen reveal changes in the gamma294-301 loop that alter the integrity of the “a” polymerization site, Biochemistry, 2007, 46, 5114–5123 CrossRef CAS PubMed.
- M. D. Bale, L. G. Westrick and D. F. Mosher, Incorporation of thrombospondin into fibrin clots, J. Biol. Chem., 1985, 260, 7502–7508 CAS.
- M. D. Bale and D. F. Mosher, Effects of thrombospondin on fibrin polymerization and structure, J. Biol. Chem., 1986, 261, 862–868 CAS.
- M. D. Bale, Noncovalent and covalent interactions of thrombospondin with polymerizing fibrin, Semin. Thromb. Hemostasis, 1987, 13, 326–334 CrossRef CAS PubMed.
- P. Hopmeier, M. Halbmayer, M. Fischer and G. Marx, Zinc modulates thrombin adsorption to fibrin, Thromb. Res., 1990, 58, 293–301 CrossRef CAS.
- G. Marx, P. Hopmeier and D. Gurfel, Zinc alters fibrin ultrastructure, Thromb. Haemostasis, 1987, 57, 73–76 CAS.
- G. Marx and P. Hopmeier, Zinc inhibits FPA release and increases fibrin turbidity, Am. J. Hematol., 1986, 22, 347–353 CrossRef CAS.
- V. G. Nielsen and E. Pretorius, Iron Enhanced Coagulation is Attenuated by Chelation: A Thrombelastographic and Ultrastructural Analysis, Blood Coagulation Fibrinolysis, 2014 DOI:10.1097/MBC.0000000000000160.
- B. Lipinski, Hydroxyl radical and its scavengers in health and disease, Oxid. Med. Cell. Longevity, 2011, 2011, 809696 Search PubMed.
- B. Lipinski and E. Pretorius, Hydroxyl radical-modified fibrinogen as a marker of thrombosis: the role of iron, Hematology, 2012, 17, 241–247 CrossRef CAS PubMed.
- G. Rimbach, J. Pallauf, J. Moehring, K. Kraemer and A. M. Minihane, Effect of dietary phytate and microbial phytase on mineral and trace element bioavailability: a literature review, Curr. Top. Nutraceutical Res., 2008, 6, 131–144 CAS.
- Y. Won, Force Field for Monovalent, Divalent, and Trivalent Cations Developed under the Solvent Boundary Potential, J. Phys. Chem. A, 2012, 116, 11763–11767 CrossRef CAS PubMed.
- F. J. Zhang, F. Roosen-Runge, A. Sauter, M. Wolf, R. M. J. Jacobs and F. Schreiber, Reentrant condensation, liquid-liquid phase separation and crystallization in protein solutions induced by multivalent metal ions, Pure Appl. Chem., 2014, 86, 191–202 CAS.
- G. Rimbach and J. Pallauf, Phytic acid inhibits free radical formation in vitro but does not affect liver oxidant or antioxidant status in growing rats, J. Nutr., 1998, 128, 1950–1955 CAS.
- M. A. Kohanski, M. A. DePristo and J. J. Collins, Sublethal antibiotic treatment leads to multidrug resistance via radical-induced mutagenesis, Mol. Cell, 2010, 37, 311–320 CrossRef CAS PubMed.
- F. Ruipérez, J. I. Mujika, J. M. Ugalde, C. Exley and X. Lopez, Pro-oxidant activity of aluminum: promoting the Fenton reaction by reducing Fe(III) to Fe(II), J. Inorg. Biochem., 2012, 117, 118–123 CrossRef PubMed.
- C. Exley, The coordination chemistry of aluminium in neurodegenerative disease, Coord. Chem. Rev., 2012, 256, 2142–2146 CrossRef CAS PubMed.
- M. Hoffman, Alterations of fibrinogen structure in human disease, Cardiovasc. Hematol. Agents Med. Chem., 2008, 6, 206–211 CrossRef CAS.
- M. Pieters, N. Covic, T. Loots du, F. H. van der Westhuizen, D. G. van Zyl, P. Rheeder, J. C. Jerling and J. W. Weisel, The effect of glycaemic control on fibrin network structure of type 2 diabetic subjects, Thromb. Haemostasis, 2006, 96, 623–629 CAS.
- M. Pieters, N. Covic, F. H. van der Westhuizen, C. Nagaswami, Y. Baras, D. Toit Loots, J. C. Jerling, D. Elgar, K. S. Edmondson, D. G. van Zyl, P. Rheeder and J. W. Weisel, Glycaemic control improves fibrin network characteristics in type 2 diabetes – a purified fibrinogen model, Thromb. Haemostasis, 2008, 99, 691–700 CAS.
- A. C. Swanepoel, B. G. Lindeque, P. J. Swart, Z. Abdool and E. Pretorius, Estrogen causes ultrastructural changes of fibrin networks during the menstrual cycle: a qualitative investigation, Microsc. Res. Tech., 2014, 77, 594–601 CrossRef CAS PubMed.
- A. C. Swanepoel, B. G. Lindeque, P. J. Swart, Z. Abdool and E. Pretorius, Ultrastructural changes of fibrin networks during three phases of pregnancy: a qualitative investigation, Microsc. Res. Tech., 2014, 77, 602–608 CrossRef PubMed.
- I. Dalle-Donne, A. Scaloni, D. Giustarini, E. Cavarra, G. Tell, G. Lungarella, R. Colombo, R. Rossi and A. Milzani, Proteins as biomarkers of oxidative/nitrosative stress in diseases: The contribution of redox proteomics, Mass Spectrom. Rev., 2005, 24, 55–99 CrossRef CAS PubMed.
- I. Dalle-Donne, R. Rossi, R. Colombo, D. Giustarini and A. Milzani, Biomarkers of oxidative damage in human disease, Clin. Chem., 2006, 52, 601–623 CAS.
- A. Bachi, I. Dalle-Donne and A. Scaloni, Redox proteomics: chemical principles, methodological approaches and biological/biomedical promises, Chem. Rev., 2013, 113, 596–698 CrossRef CAS PubMed.
- D. A. Butterfield and I. Dalle-Donne, Redox Proteomics: From Protein Modifications to Cellular Dysfunction and Disease, Mass Spectrom. Rev., 2014, 33, 1–6 CrossRef CAS PubMed.
- V. G. Nielsen, J. P. Crow, F. Zhou and D. A. Parks, Peroxynitrite inactivates tissue plasminogen activator, Anesth. Analg., 2004, 98, 1312–1317 CAS , table of contents.
- I. Parastatidis, L. Thomson, A. Burke, I. Chernysh, C. Nagaswami, J. Visser, S. Stamer, D. C. Liebler, G. Koliakos, H. F. Heijnen, G. A. Fitzgerald, J. W. Weisel and H. Ischiropoulos, Fibrinogen beta-chain tyrosine nitration is a prothrombotic risk factor, J. Biol. Chem., 2008, 283, 33846–33853 CrossRef CAS PubMed.
- Y. Ding, Y. Luo and J. Fu, Effects of Mn (II) on peroxynitrite nitrifying fibrinogen, Bio-Med. Mater. Eng., 2014, 24, 901–907 CAS.
- E. A. Hod, G. M. Brittenham, G. B. Billote, R. O. Francis, Y. Z. Ginzburg, J. E. Hendrickson, J. Jhang, J. Schwartz, S. Sharma, S. Sheth, A. N. Sireci, H. L. Stephens, B. A. Stotler, B. S. Wojczyk, J. C. Zimring and S. L. Spitalnik, Transfusion of human volunteers with older, stored red blood cells produces extravascular hemolysis and circulating non-transferrin-bound iron, Blood, 2011, 118, 6675–6682 CrossRef CAS PubMed.
- E. A. Hod and S. L. Spitalnik, Stored red blood cell transfusions: Iron, inflammation, immunity, and infection, Transfus. Clin. Biol., 2012, 19, 84–89 CrossRef CAS PubMed.
- D. Wang, J. Sun, S. B. Solomon, H. G. Klein and C. Natanson, Transfusion of older stored blood and risk of death: a meta-analysis, Transfusion, 2012, 52, 1184–1195 CrossRef PubMed.
- C. Aubron, A. Nichol, D. J. Cooper and R. Bellomo, Age of red blood cells and transfusion in critically ill patients, Ann. Intensive Care, 2013, 3, 2 CrossRef PubMed.
- L. T. Goodnough, J. H. Levy and M. F. Murphy, Concepts of blood transfusion in adults, Lancet, 2013, 381, 1845–1854 CrossRef.
- A. C. Hubbard, S. Bandyopadhyay, B. S. Wojczyk, S. L. Spitalnik, E. A. Hod and K. A. Prestia, Effect of dietary iron on fetal growth in pregnant mice, Comp. Med., 2013, 63, 127–135 CAS.
- S. B. Solomon, D. Wang, J. Sun, T. Kanias, J. Feng, C. C. Helms, M. A. Solomon, M. Alimchandani, M. Quezado, M. T. Gladwin, D. B. Kim-Shapiro, H. G. Klein and C. Natanson, Mortality increases after massive exchange transfusion with older stored blood in canines with experimental pneumonia, Blood, 2013, 121, 1663–1672 CrossRef CAS PubMed.
- A. Shander and L. T. Goodnough, Can blood transfusion be not only ineffective, but also injurious?, Ann. Thorac. Surg., 2014, 97, 11–14 CrossRef PubMed.
- L. T. Goodnough, Iron-related adverse effects from stored blood, Blood, 2014, 123, 1287–1289 CrossRef CAS PubMed.
- I. Cortés-Puch, D. Wang, J. Sun, S. B. Solomon, K. E. Remy, M. Fernandez, J. Feng, T. Kanias, L. Bellavia, D. Sinchar, A. Perlegas, M. A. Solomon, W. E. Kelley, M. A. Popovsky, M. T. Gladwin, D. B. Kim-Shapiro, H. G. Klein and C. Natanson, Washing older blood units before transfusion reduces plasma iron and improves outcomes in experimental canine pneumonia, Blood, 2014, 123, 1403–1411 CrossRef PubMed.
- D. Wang, I. Cortés-Puch, J. Sun, S. B. Solomon, T. Kanias, K. E. Remy, J. Feng, M. Alimchandani, M. Quezado, C. Helms, A. Perlegas, M. T. Gladwin, D. B. Kim-Shapiro, H. G. Klein and C. Natanson, Transfusion of older stored blood worsens outcomes in canines depending on the presence and severity of pneumonia, Transfusion, 2014, 54, 1712–1724 CrossRef PubMed.
- L. Katsimpardi, N. K. Litterman, P. A. Schein, C. M. Miller, F. S. Loffredo, G. R. Wojtkiewicz, J. W. Chen, R. T. Lee, A. J. Wagers and L. L. Rubin, Vascular and neurogenic rejuvenation of the aging mouse brain by young systemic factors, Science, 2014, 344, 630–634 CrossRef CAS PubMed.
- S. A. Villeda, K. E. Plambeck, J. Middeldorp, J. M. Castellano, K. I. Mosher, J. Luo, L. K. Smith, G. Bieri, K. Lin, D. Berdnik, R. Wabl, J. Udeochu, E. G. Wheatley, B. Zou, D. A. Simmons, X. S. Xie, F. M. Longo and T. Wyss-Coray, Young blood reverses age-related impairments in cognitive function and synaptic plasticity in mice, Nat. Med., 2014 Search PubMed.
- P. J. Nestel, N. Straznicky, N. A. Mellett, G. Wong, D. P. De Souza, D. L. Tull, C. K. Barlow, M. T. Grima and P. J. Meikle, Specific plasma lipid classes and phospholipid fatty acids indicative of dairy food consumption associate with insulin sensitivity, Am. J. Clin. Nutr., 2014, 99, 46–53 CrossRef CAS PubMed.
- M. B. Andresdottir, N. Sigfusson, H. Sigvaldason and V. Gudnason, Erythrocyte sedimentation rate, an independent predictor of coronary heart disease in men and women – The Reykjavik study, Am. J. Epidemiol., 2003, 158, 844–851 CrossRef PubMed.
- J. Crawford, M. K. Eye-Boland and H. J. Cohen, Clinical utility of erythrocyte sedimentation rate and plasma protein analysis in the elderly, Am. J. Med., 1987, 82, 239–246 CrossRef CAS.
- G. Erikssen, K. Liestol, J. V. Bjornholt, H. Stormorken, E. Thaulow and J. Erikssen, Erythrocyte sedimentation rate: a possible marker of atherosclerosis and a strong predictor of coronary heart disease mortality, Eur. Heart J., 2000, 21, 1614–1620 CrossRef CAS PubMed.
- T. A. Kermani, J. Schmidt, C. S. Crowson, S. R. Ytterberg, G. G. Hunder, E. L. Matteson and K. J. Warrington, Utility of erythrocyte sedimentation rate and C-reactive protein for the diagnosis of giant cell arteritis, Semin. Arthritis Rheum., 2012, 41, 866–871 CrossRef CAS PubMed.
- M. Pavlović, D. Babić, P. Rastović and I. Ljevak, Association of erythrocyte sedimentation rate and fibrinogen concentration with metabolic syndrome in schizophrenic patients, Psychiatr. Danubina, 2013, 25(suppl 1), 51–55 Search PubMed.
- S. Yardim-Akaydin, E. Caliskan-Can, H. Firat, S. Ardic and B. Simsek, Influence of Gender on C-Reactive Protein, Fibrinogen, and Erythrocyte Sedimentation Rate in Obstructive Sleep Apnea, Anti-Inflammatory Anti-Allergy Agents Med. Chem., 2014, 13, 56–63 CrossRef CAS.
- I. Yildirim, E. Hur and F. Kokturk, Inflammatory Markers: C-Reactive Protein, Erythrocyte Sedimentation Rate, and Leukocyte Count in Vitamin D Deficient Patients with and without Chronic Kidney Disease, Int. J. Endocrinol., 2013, 2013, 802165 Search PubMed.
- F. J. Neumann, H. A. Katus, E. Hoberg, P. Roebruck, M. Braun, H. M. Haupt, H. Tillmanns and W. Kübler, Increased plasma viscosity and erythrocyte aggregation: indicators of an unfavourable clinical outcome in patients with unstable angina pectoris, Br. Heart J., 1991, 66, 425–430 CrossRef CAS.
- A. Luquita, L. Urli, M. J. Svetaz, A. M. Gennaro, R. Volpintesta, S. Palatnik and M. Rasia, Erythrocyte aggregation in rheumatoid arthritis: Cell and plasma factor's role, Clin. Hemorheol. Microcirc., 2009, 41, 49–56 CAS.
- M. Piagnerelli, K. Z. Boudjeltia, M. Vanhaeverbeek and J. L. Vincent, Red blood cell rheology in sepsis, Intensive Care Med., 2003, 29, 1052–1061 CrossRef CAS PubMed.
- N. Bolokadze, I. Lobjanidze, N. Momtselidze, R. Shakarishvili and G. Mchedlishvili, Comparison of erythrocyte aggregability changes during ischemic and hemorrhagic stroke, Clin. Hemorheol. Microcirc., 2006, 35, 265–267 CAS.
- in Handbook of Hemorheology and Hemodynamics, ed. O. K. Baskurt, M. R. Hardeman, M. W. Rampling and H. J. Meiselman, IOS Press, Amsterdam, 2007 Search PubMed.
-
O. Baskurt, B. Neu and H. J. Meiselman, Red Blood Cell Aggregation, CRC Press, Boca Raton, 2011 Search PubMed.
- M. R. Hardeman, P. T. Goedhart, J. G. G. Dobbe and K. P. Lettinga, Laser-Assisted Optical Rotational Cell Analyzer (Lorca). 1. A New Instrument for Measurement of Various Structural Hemorheological Parameters, Clin. Hemorheol., 1994, 14, 605–618 Search PubMed.
- M. R. Hardeman, P. T. Goedhart and N. H. Schut, Laser-Assisted Optical Rotational Cell Analyzer (Lorca). 2. Red-Blood-Cell Deformability – Elongation Index Versus Cell Transit-Time, Clin. Hemorheol., 1994, 14, 619–630 Search PubMed.
- M. R. Hardeman, J. G. Dobbe and C. Ince, The Laser-assisted Optical Rotational Cell Analyzer (LORCA) as red blood cell aggregometer, Clin. Hemorheol. Microcirc., 2001, 25, 1–11 CAS.
- M. R. Hardeman, G. A. J. Besselink, I. Ebbing, D. de Korte, C. Ince and A. J. Verhoeven, Laser-assisted optical rotational cell analyzer measurements reveal early changes in human RBC deformability induced by photodynamic treatment, Transfusion, 2003, 43, 1533–1537 CrossRef.
- J. Bester, A. V. Buys, B. Lipinski, D. B. Kell and E. Pretorius, High ferritin levels have major effects on the morphology of erythrocytes in Alzheimer's disease, Front. Aging Neurosci., 2013, 5, 00088 Search PubMed.
- A. V. Buys, M. J. Van Rooy, P. Soma, D. Van Papendorp, B. Lipinski and E. Pretorius, Changes in red blood cell membrane structure in type 2 diabetes: a scanning electron and atomic force microscopy study, Cardiovasc. Diabetol., 2013, 12, 25 CrossRef PubMed.
- J. N. Du Plooy, A. Buys, W. Duim and E. Pretorius, Comparison of platelet ultrastructure and elastic properties in thrombo-embolic ischemic stroke and smoking using atomic force and scanning electron microscopy, PLoS One, 2013, 8, e69774 CAS.
- E. Pretorius, J. N. du Plooy, P. Soma, I. Keyser and A. V. Buys, Smoking and fluidity of erythrocyte membranes: A high resolution scanning electron and atomic force microscopy investigation, Nitric Oxide, 2013, 35C, 42–46 CrossRef PubMed.
- W. J. van der Spuy and E. Pretorius, Interaction of red blood cells adjacent to and within a thrombus in experimental cerebral ischaemia, Thromb. Res., 2013, 132, 718–723 CrossRef CAS PubMed.
- M. E. Emans, C. A. J. M. Gaillard, R. Pfister, M. W. Tanck, S. M. Boekholdt, N. J. Wareham and K. T. Khaw, Red cell distribution width is associated with physical inactivity and heart failure, independent of established risk factors, inflammation or iron metabolism; the EPIC-Norfolk study, Int. J. Cardiol., 2013, 168, 3550–3555 CrossRef PubMed.
- Z. Förhécz, T. Gombos, G. Borgulya, Z. Pozsonyi, Z. Prohászka and L. Jánoskuti, Red cell distribution width in heart failure: prediction of clinical events and relationship with markers of ineffective erythropoiesis, inflammation, renal function, and nutritional state, Am. Heart J., 2009, 158, 659–666 CrossRef PubMed.
- Z. A. Öztürk, A. Ünal, R. Yiğiter, Y. Yesil, M. E. Kuyumcu, M. Neyal and Y. Kepekçi, Is increased red cell distribution width (RDW) indicating the inflammation in Alzheimer's disease (AD)?, Arch. Gerontol. Geriatr., 2013, 56, 50–54 CrossRef PubMed.
- K. Shrestha, A. G. Borowski, R. W. Troughton, A. L. Klein and W. H. Tang, Association between systemic neutrophil gelatinase-associated lipocalin and anemia, relative hypochromia, and inflammation in chronic systolic heart failure, Congestive Heart Failure, 2012, 18, 239–244 CrossRef CAS PubMed.
- L. A. Allen, G. M. Felker, M. R. Mehra, J. R. Chiong, S. H. Dunlap, J. K. Ghali, D. J. Lenihan, R. M. Oren, L. E. Wagoner, T. A. Schwartz and K. F. Adams, Jr., Validation and potential mechanisms of red cell distribution width as a prognostic marker in heart failure, J. Card. Failure, 2010, 16, 230–238 CrossRef PubMed.
- Y. Koma, A. Onishi, H. Matsuoka, N. Oda, N. Yokota, Y. Matsumoto, M. Koyama, N. Okada, N. Nakashima, D. Masuya, H. Yoshimatsu and Y. Suzuki, Increased red blood cell distribution width associates with cancer stage and prognosis in patients with lung cancer, PLoS One, 2013, 8, e80240 CAS.
- W. S. Lee and T. Y. Kim, Relation between red blood cell distribution
width and inflammatory biomarkers in rheumatoid arthritis, Arch. Pathol. Lab. Med., 2010, 134, 505–506 Search PubMed.
- G. Lippi, G. Targher, M. Montagnana, G. L. Salvagno, G. Zoppini and G. C. Guidi, Relation between red blood cell distribution width and inflammatory biomarkers in a large cohort of unselected outpatients, Arch. Pathol. Lab. Med., 2009, 133, 628–632 CAS.
- M. Montagnana, G. Cervellin, T. Meschi and G. Lippi, The role of red blood cell distribution width in cardiovascular and thrombotic disorders, Clin. Chem. Lab. Med., 2012, 50, 635–641 CAS.
- C. S. Song, D. I. Park, M. Y. Yoon, H. S. Seok, J. H. Park, H. J. Kim, Y. K. Cho, C. I. Sohn, W. K. Jeon and B. I. Kim, Association between red cell distribution width and disease activity in patients with inflammatory bowel disease, Dig. Dis. Sci., 2012, 57, 1033–1038 CrossRef CAS PubMed.
- A. Vaya, J. L. Hernández, E. Zorio and D. Bautista, Association between red blood cell distribution width and the risk of future cardiovascular events, Clin. Hemorheol. Microcirc., 2012, 50, 221–225 Search PubMed.
- A. S. Lapedes, B. G. Giraud, L. C. Liu and G. D. Stormo, Correlated mutations in models of protein sequences: phylogenetic and structural effects, IMS Lect. Notes, 1999, 33, 236–256 Search PubMed.
- H. Ashkenazy and Y. Kliger, Reducing phylogenetic bias in correlated mutation analysis, PEDS, 2010, 23, 321–326 CAS.
- D. S. Marks, L. J. Colwell, R. Sheridan, T. A. Hopf, A. Pagnani, R. Zecchina and C. Sander, Protein 3D structure computed from evolutionary sequence variation, PLoS One, 2011, 6, e28766 CAS.
- D. T. Jones, D. W. Buchan, D. Cozzetto and M. Pontil, PSICOV: Precise structural contact prediction using sparse inverse covariance estimation on large multiple sequence alignments, Bioinformatics, 2012, 28, 184–190 CrossRef CAS PubMed.
- D. S. Marks, T. A. Hopf and C. Sander, Protein structure prediction from sequence variation, Nat. Biotechnol., 2012, 30, 1072–1080 CrossRef CAS PubMed.
- T. Kosciolek and D. T. Jones,
De novo structure prediction of globular proteins aided by sequence variation-derived contacts, PLoS One, 2014, 9, e92197 Search PubMed.
- A. Yasim, M. Kilinc, M. Aral, H. Oksuz, M. Kabalci, E. Eroglu and S. Imrek, Serum concentration of procoagulant, endothelial and oxidative stress markers in early primary varicose veins, Phlebology, 2008, 23, 15–20 CrossRef CAS.
- H. Yang, H. J. Ko, J. Y. Yang, J. J. Kim, S. U. Seo, S. G. Park, S. S. Choi, J. K. Seong and M. N. Kweon, Interleukin-1 promotes coagulation, which is necessary for protective immunity in the lung against Streptococcus pneumoniae infection, J. Infect. Dis., 2013, 207, 50–60 CrossRef CAS PubMed.
- M. N. Mannila, P. Eriksson, K. Leander, B. Wiman, U. de Faire, A. Hamsten and A. Silveira, The association between fibrinogen haplotypes and myocardial infarction in men is partly mediated through pleiotropic effects on the serum IL-6 concentration, J. Intern. Med., 2007, 261, 138–147 CrossRef CAS PubMed.
- Z. X. Zhang, N. L. Fuentes and G. M. Fuller, Characterization of the Il-6 Responsive Elements in the Gamma-Fibrinogen Gene Promoter, J. Biol. Chem., 1995, 270, 24287–24291 CrossRef CAS PubMed.
- S. Ohta, M. Okazaki, M. Maruyama and K. Oguchi, Involvement of IL-6 and IL-6 receptor in fibrinogen synthesis in the liver of triton WR-1339-induced hyperlipidemic rats, In Vivo, 2004, 18, 203–211 CAS.
- Y. Ohsugi, Recent advances in immunopathophysiology of interleukin-6: an innovative therapeutic drug, tocilizumab (recombinant humanized anti-human interleukin-6 receptor antibody), unveils the mysterious etiology of immune-mediated inflammatory diseases, Biol. Pharm. Bull., 2007, 30, 2001–2006 CAS.
- H. O. Duan and P. J. Simpson-Haidaris, Functional analysis of interleukin 6 response elements (IL-6REs) on the human gamma-fibrinogen promoter: binding of hepatic Stat3 correlates negatively with transactivation potential of type II IL-6REs, J. Biol. Chem., 2003, 278, 41270–41281 CrossRef CAS PubMed.
- H. O. Duan and P. J. Simpson-Haidaris, Cell type-specific differential induction of the human gamma-fibrinogen promoter by interleukin-6, J. Biol. Chem., 2006, 281, 12451–12457 CrossRef CAS PubMed.
- C. L. Carty, P. Heagerty, S. R. Heckbert, G. P. Jarvik, L. A. Lange, M. Cushman, R. P. Tracy and A. P. Reiner, Interaction between Fibrinogen and IL-6 Genetic Variants and Associations with Cardiovascular Disease Risk in the Cardiovascular Health Study, Ann. Hum. Genet., 2010, 74, 1–10 CrossRef CAS PubMed.
- D. I. Swerdlow, M. V. Holmes, K. B. Kuchenbaecker, J. E. L. Engmann, T. Shah, R. Sofat, Y. R. Guo, C. Chung, A. Peasey, R. P. Ster, S. P. Mooijaart, H. A. Ireland, M. Leusink, C. Langenberg, K. Li, J. Palmen, P. Howard, J. A. Cooper, F. Drenos, J. Hardy, M. A. Nalls, Y. R. Li, G. Lowe, M. Stewart, S. J. Bielinski, J. Peto, N. J. Timpson, J. Gallacher, M. Dunlop, R. Houlston, I. Tomlinson, I. Tzoulaki, J. Luan, J. M. A. Boer, N. G. Forouhi, N. C. Onland-Moret, Y. T. van der Schouw, R. B. Schnabel, J. A. Hubacek, R. Kubinova, M. Baceviciene, A. Tamosiunas, A. Pajak, R. Topor-Madry, S. A. Malyutina, D. Baldassarre, B. Sennblad, E. Tremoli, U. de Faire, L. Ferrucci, S. Bandenelli, T. Tanaka, J. F. Meschia, A. Singleton, G. Navis, I. M. Leach, S. J. L. Bakker, R. T. Gansevoort, I. Ford, S. E. Epstein, M. S. Burnett, J. M. Devaney, J. W. Jukema, R. G. J. Westendorp, G. J. de Borst, Y. van der Graaf, P. A. de Jong, A. H. Maitland-van der Zee, O. H. Klungel, A. de Boer, P. A. Doevendans, J. W. Stephens, C. B. Eaton, J. G. Robinson, J. E. Manson, F. G. R. Fowkes, T. M. Frayling, J. F. Price, P. H. Whincup, R. W. Morris, D. A. Lawlor, G. D. Smith, Y. Ben-Shlomo, S. Redline, L. A. Lange, M. Kumari, N. J. Wareham, W. M. M. Verschuren, E. J. Benjamin, J. C. Whittaker, A. Hamsten, F. Dudbridge, J. A. C. Delaney, A. Wong, D. Kuh, R. Hardy, B. A. Castillo, J. J. Connolly, P. van der Harst, E. J. Brunner, M. G. Marmot, C. L. Wassel, S. E. Humphries, P. J. Talmud, M. Kivimaki, F. W. Asselbergs, M. Voevoda, M. Bobak, H. Pikhart, J. G. Wilson, H. Hakonarson, A. P. Reiner, B. J. Keating, N. Sattar, A. D. Hingorani and J. P. Casas, I.-R. M. R The interleukin-6 receptor as a target for prevention of coronary heart disease: a mendelian randomisation analysis, Lancet, 2012, 379, 1214–1224 CrossRef.
- E. Nemeth, S. Rivera, V. Gabayan, C. Keller, S. Taudorf, B. K. Pedersen and T. Ganz, IL-6 mediates hypoferremia of inflammation by inducing the synthesis of the iron regulatory hormone hepcidin, J. Clin. Invest., 2004, 113, 1271–1276 CrossRef CAS.
- N. V. Gorbunov, S. J. McFaul, A. Januszkiewicz and J. L. Atkins, Pro-inflammatory alterations and status of blood plasma iron in a model of blast-induced lung trauma, Int. J. Immunopathol. Pharmacol., 2005, 18, 547–556 CAS.
- R. G. DePalma, V. W. Hayes, B. K. Chow, G. Shamayeva, P. E. May and L. R. Zacharski, Ferritin levels, inflammatory biomarkers, and mortality in peripheral arterial disease: A substudy of the Iron (Fe) and Atherosclerosis Study (FeAST) Trial, J. Vasc. Surg., 2010, 51, 1498–1503 CrossRef PubMed.
- F. Marques, A. M. Falcao, J. C. Sousa, G. Coppola, D. Geschwind, N. Sousa, M. Correia-Neves and J. A. Palha, Altered iron metabolism is part of the choroid plexus response to peripheral inflammation, Endocrinology, 2009, 150, 2822–2828 CrossRef CAS PubMed.
- K. Broedbaek, V. Siersma, J. T. Andersen, M. Petersen, S. Afzal, B. Hjelvang, A. Weimann, R. D. Semba, L. Ferrucci and H. E. Poulsen, The association between low-grade inflammation, iron status and nucleic acid oxidation in the elderly, Free Radical Res., 2011, 45, 409–416 CrossRef CAS PubMed.
- S. Uysal, F. Armutcu, T. Aydogan, K. Akin, M. Ikizek and M. R. Yigitoglu, Some inflammatory cytokine levels, iron metabolism and oxidan stress markers in subjects with nonalcoholic steatohepatitis, Clin. Biochem., 2011, 44, 1375–1379 CrossRef CAS PubMed.
- A. C. Chua, B. R. Klopcic, D. S. Ho, S. K. Fu, C. H. Forrest, K. D. Croft, J. K. Olynyk, I. C. Lawrance and D. Trinder, Dietary Iron Enhances Colonic Inflammation and IL-6/IL-11-Stat3 Signaling Promoting Colonic Tumor Development in Mice, PLoS One, 2013, 8, e78850 CAS.
- J. López-Prieto, E. González-Reimers, M. R. Alemán-Valls, M. J. de la Vega-Prieto, P. Abreu-González, R. Pelazas-González, R. Hernández-Luis, C. Jorge-Ripper and F. Santolaria-Fernández, Iron and proinflammatory cytokines in chronic hepatitis C virus infection, Biol. Trace Elem. Res., 2013, 155, 5–10 CrossRef PubMed.
- S.-N. J. Song, M. Iwahashi, N. Tomosugi, K. Uno, J. Yamana, S. Yamana, T. Isobe, H. Ito, H. Kawabata and K. Yoshizaki, Comparative evaluation of the effects of treatment with tocilizumab and TNF-alpha inhibitors on serum hepcidin, anemia response and disease activity in rheumatoid arthritis patients, Arthritis Res. Ther., 2013, 15, R141 Search PubMed.
- L. A. Borthwick, T. A. Wynn and A. J. Fisher, Cytokine mediated tissue fibrosis, Biochim. Biophys. Acta, 2013, 1832, 1049–1060 CrossRef CAS PubMed.
- R. C. Chambers and G. J. Laurent, Coagulation cascade proteases and tissue fibrosis, Biochem. Soc. Trans., 2002, 30, 194–200 CrossRef CAS.
- B. D. Bringardner, C. P. Baran, T. D. Eubank and C. B. Marsh, The role of inflammation in the pathogenesis of idiopathic pulmonary fibrosis, Antioxid. Redox Signaling, 2008, 10, 287–301 CrossRef CAS PubMed.
- R. C. Chambers, Procoagulant signalling mechanisms in lung inflammation and fibrosis: novel opportunities for pharmacological intervention?, Br. J. Pharmacol., 2008, 153(suppl 1), S367–S378 CAS.
- T. A. Wynn and T. R. Ramalingam, Mechanisms of fibrosis: therapeutic translation for fibrotic disease, Nat. Med., 2012, 18, 1028–1040 CrossRef CAS PubMed.
- J. S. Duffield, M. Lupher, V. J. Thannickal and T. A. Wynn, Host responses in tissue repair and fibrosis, Annu. Rev. Pathol.: Mech. Dis., 2013, 8, 241–276 CrossRef CAS PubMed.
- P. F. Mercer and R. C. Chambers, Coagulation and coagulation signalling in fibrosis, Biochim. Biophys. Acta, 2013, 1832, 1018–1027 CrossRef CAS PubMed.
- V. Aggarwal, P. M. Kapoor, M. Choudhury, U. Kiran and U. Chowdhury, Utility of Sonoclot analysis and tranexamic acid in tetralogy of Fallot patients undergoing intracardiac repair, Ann. Card. Anaesth., 2012, 15, 26–31 CrossRef PubMed.
|
This journal is © The Royal Society of Chemistry 2015 |