DOI:
10.1039/C3SC52622D
(Edge Article)
Chem. Sci., 2014,
5, 163-168
Benz[c]indeno[2,1-a]fluorene: a 2,3-naphthoquinodimethane incorporated into an indenofluorene frame†
Received
18th September 2013
, Accepted 7th October 2013
First published on 7th October 2013
Abstract
Synthesis of 13,14-dimesitylbenz[c]indeno[2,1-a]fluorene (9b), a 2,3-naphthoquinodimethane derivative isolated for the first time, was achieved. The X-ray crystallographic structure and spectroscopic and electrochemical properties of 9b indicate substantial singlet biradical character which is higher than that of 11,12-dimesitylindeno[2,1-a]fluorene (8b), in accord with the quantum chemical study. Cycloadditions with oxygen and dichlorodicyano-p-benzoquinone took place at the inner naphthalene carbons rather than the most reactive exocyclic carbons because of the effective steric protection by the mesityl groups at the latter positions. [4 + 2] Cyclodimerization also took place at the inner naphthalene position as a 4π component and at the outer naphthalene ring as a 2π component.
Introduction
Ortho- and para-quinodimethanes (o-QDMs and p-QDMs) have been attracting a great deal of interest for many years because of their unique electronic structures and as reactive intermediates in organic synthesis. Although o-QDMs have been utilised as versatile diene building blocks for the construction of six-membered rings,1 their physical organic studies have been much less studied compared to p-QDMs owing to their inherent high reactivity. In addition, the s-cis geometry of the exocyclic diene moiety renders steric protection difficult.2 In fact, whereas p-QDM congeners such as Thiele's hydrocarbon 13 and Tschitschibabin's hydrocarbon 24 have been known as stable compounds for over a century (Chart 1), o-QDMs such as tetraphenyl derivative 35 and pleiadene 46 have been shown to be elusive and detected only in rigid glass matrices. A series of tetraaryl o-QDMs incorporated into a phenanthrene framework such as 57 represent scarce examples of simple o-QDMs successfully isolated. The higher congener of o-QDM, 2,3-naphthoquinodimethane (NQD, 6a), is even more reactive. The parent NQD 6a and its bridged derivative 6c were only spectroscopically characterised in glass matrices at low temperatures, but they decomposed when the matrices were unfrozen.8 Heavily protected NQD derivatives such as 6d and 6e reported by Jones and co-workers were stable enough for UV-vis spectral measurement and for trapping by a dienophile in solution at room temperature, though they were not isolated due to facile air oxidation.9 Recently, o-QDMs and p-QDMs embedded in aromatic hydrocarbon frames have been a topic of intense interest because of their unique optical properties associated with singlet biradical characters,10 small band gaps and excellent electrochemical behaviours, which make them potential candidates for optoelectronic materials.11–13 Among these, indenofluorene congeners such as indeno[1,2-b]fluorene (7)11h–k and indeno[2,1-a]fluorene (8a)12 are of particular interest because of their simple frameworks consisting of consecutively fused six- and five-membered rings that would allow further structural as well as functional modification.13a Taking into account the stability of the mesityl derivative 8b,12b we envisioned that embedding NQD 6a into an indenofluorene frame (i.e.9a) would kinetically stabilise it by both electronic and steric means, though an attempt to synthesise the 13,14-diphenyl derivative 9c was reported to be unsuccessful.12aScheme 1 illustrates the Kekulé and open-shell biradical canonical resonance structures for 8a and 9a, which indicate that the radical centres should be located principally on the five-membered ring. For 9a, however, the spin density may also distribute to the inner naphthalene ring. Here we report the synthesis, structure, and physical properties of 13,14-dimesitylbenz[c]indeno[2,1-a]fluorene (9b), an NQD derivative that has been isolated for the first time.
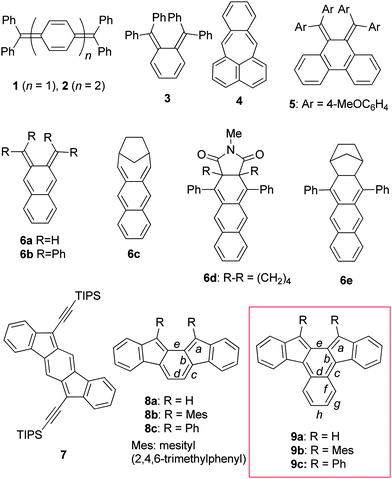 |
| Chart 1 Representative QDM derivatives 1–5, NQD derivatives 6a–e, indenofluorene isomers 7 and 8a–c, and benzindenofluorenes 9a–c. | |
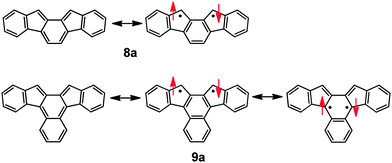 |
| Scheme 1 Canonical resonance structures for 8a and 9a. | |
Results and discussion
The synthesis of 9b was carried out as outlined in Scheme 2. Addition of mesitylmagnesium bromide to the known diketone 1014 gave diol 11,15 which was subjected to SnCl2-mediated dehydroxylation to give 9b. Pure samples of 9b were obtained by recrystallization from benzene–acetonitrile under argon as dark blue crystals in 8% yield. This compound was stable in the crystalline state or in degassed solution, but it decolorised in air even under protection from room light. Its half-life in the dark determined from the pseudo-first order decrease of its UV-vis spectra in CH2Cl2 (2.2 × 10−5 M) was 77 min (Fig. S1 in ESI†). The structure of the oxidation product, which was formed quantitatively as indicated by the presence of isosbestic points, was elucidated based on the correlation NMR spectra to be endoperoxide 12 (Fig. S2 and S3 in ESI†) and was confirmed by X-ray crystallographic analysis (Fig. 1). Note that the addition of oxygen took place at the inner naphthalene carbons but not at the exo-methylene positions which are expected to be most reactive.16 The regioselectivity of the addition is discussed later. Though the formation of endoperoxides by addition of ground-state oxygen is spin-symmetry forbidden, such reactions have been observed for highly reactive dienes.17
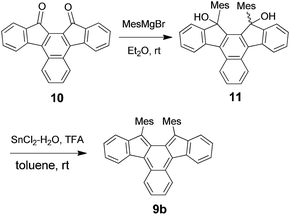 |
| Scheme 2 Synthesis of 9b. | |
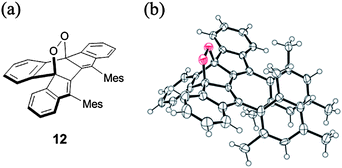 |
| Fig. 1 (a) Molecular structure and (b) ORTEP drawing of 12. | |
Although at room temperature 9b showed sharp signals in the 1H NMR spectrum, they broadened at high temperature such as 100 °C (Fig. S4 in ESI†). Since the signal broadening and sharpening are reversible, this behaviour is ascribed to the population of a small amount of thermally excited triplet state at high temperature. Some biradicaloid compounds incorporating an o-QDM or p-QDM substructure have small singlet–triplet (S–T) energy gaps and hence exhibit temperature-dependent line broadening in their 1H NMR spectra due to occupation of thermally excited triplet states at higher temperatures.11a,b,e–g,13c Indeed, the S–T gap estimated from the DFT calculations18 for the parent 9a (B3LYP/6-31G(d)) is 31.2 kJ mol−1, consistent with the observed NMR behaviour (Table S1 in ESI†). The calculated S–T gap for 9a is smaller than that for 8a (53.9 kJ mol−1),12b indicating the increased biradical character of the former. The chemical shifts of the all protons of the π-framework of 9b exhibit an upfield shift compared to its precursor 11 as expected from the anti-aromatic as-indacene core (Table S2 in ESI†). The NICS(1)19 values for 9a are more positive than those of 8a, indicating a stronger anti-aromaticity in 9a (Table S3 in ESI†).
Compound 9b showed an absorption maximum at 697 nm (ε = 12
800 M−1 cm−1) in CH2Cl2 which extends into the NIR region (ca. 1050 nm) (Fig. 2). This is consistent with the strong absorption at 760 nm (f = 0.1148) and a weak one at 927 nm (f = 0.0074) predicted by the TD-DFT calculations (Table S4 in ESI†). Compared to 8b whose absorption maximum and end are at 537 and ca. 780 nm, respectively,12b9b exhibits a substantial bathochromic shift due to the smaller HOMO–LUMO gap as a result of the extended π-conjugation and greater singlet biradical character, which both contribute to lowering the excitation energy.20 The calculated HOMO–LUMO gap (B3LYP/6-31G*) for 9a (1.66 eV) is smaller than that for 8a (2.26 eV). The singlet biradical character (y) of 9a was calculated by the natural orbital occupation number (NOON) of the LUMO with spin-unrestricted calculations.21 The broken-symmetry UHF/6-31G* calculations of 9a gave a LUMO occupation number of 0.81. The index y was estimated to be 0.63 using the Yamaguchi scheme,22,23 which was considerably larger when compared to that of 8a (0.33).12b NQD 6a and its derivative 6c were reported to exhibit weak absorption at 541 and 588 nm, respectively, in solvent matrices.8 Moreover, Jones et al. reported the absorption maxima of sterically encumbered 6d and 6e at ca. 600 and 648 nm, respectively.9 The remarkable bathochromic shift in 9b compared to 6d and 6e can be ascribed to the extension of π-conjugation of the NQD chromophore to the planar indenofluorene framework. In cyclic voltammetry, 9b showed a smaller Eredox of 1.60 V than that of 8b (2.10 V),12b with reduction waves at −1.22 and −1.61 V and oxidation waves at +0.38, +0.77, and ca. +1.1 V (irreversible) (Fig. S6 in ESI†).
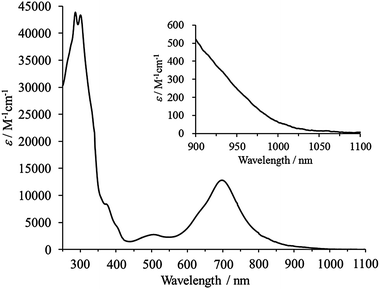 |
| Fig. 2 UV-vis NIR spectrum of 9b in CH2Cl2. | |
Crystals suitable for X-ray structure analysis were obtained by recrystallization from benzene–acetonitrile (Fig. 3). The bond lengths of the π-framework of 9b are listed in Table 1 together with those of 8b.12b For comparison, the theoretical bond lengths of 8a–b and 9a–b estimated by DFT calculations are summarised in Table S6 in ESI.† The calculated bond lengths of 9b agree well with those of the experimental data except for that of bond b which is longer in the crystal structure. The π-framework of 9b is almost planar and the two mesityl groups have large dihedral angles of 69.8° (average) to the backbone. The crystal structure of 9b shows characteristics due to the greater biradicaloid structure compared to that of 8b: the bond distance of the exo-methylene bond a (1.403(2) Å) of 9b is longer than that of 8b (1.391(2) Å). The same is true for bond c (1.398(2) vs. 1.359(3) Å), whereas bond b is shorter than that of 8b (1.468(2) vs. 1.480(2) Å). Another notable feature is the bond-length alternation in the outer naphthalene ring of 9b which has relatively long bonds f (1.441(2) Å) and h (1.419(2) Å) and a short bond g (1.363(3) Å). The observed bond length alternation is more significant than that of naphthalene24 and is comparable to that of the bicyclo[2.1.1]pentene-fused naphthalene derivative25 which exhibits a high degree of bond alternation due to the Mills–Nixon effect.26
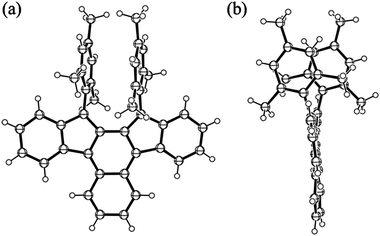 |
| Fig. 3 ORTEP drawings of 9b: (a) top view and (b) side view. | |
Table 1 Experimental bond lengths (Å) of the π-framework of indeno[2,1-a]fluorene 8b and benz[c]indeno[2,1-a]fluorene 9b
Bonda |
8b
|
9b
|
Bond positions are shown in Chart 1.
Ref. 12b.
Not applicable.
|
a
|
1.391(2) |
1.403(2) |
b
|
1.480(2) |
1.468(2) |
c
|
1.359(3) |
1.398(2) |
d
|
1.431(3) |
1.465(3) |
e
|
1.454(2) |
1.453(3) |
f
|
—c |
1.441(2) |
g
|
—c |
1.363(3) |
h
|
—c |
1.419(4) |
The kinetic stability of 9b is discussed by comparison with the hypothetical tetraphenyl NQD derivative 6b which has the same number of π-bonds as that of 9b yet has a highly twisted structure due to steric repulsion between the phenyl groups (Tables S1 and S6 in ESI†). The shorter distance of bond a in the theoretical structure of 9b than that of 6b indicates smaller biradical character in the former system. Indeed, the S–T gap of 9b is larger and the spin densities at the methylene carbons (C1 and C12) in 9b are smaller than those of 6b (Fig. S9, Tables S1 and S7 in ESI†). This is because of the planar framework of the indenofluorene system which makes 9b possess more singlet character than 6b. In addition to this electronic stabilization, the mesityl groups exert effective steric protection, thereby making the isolation of 9b possible.
Finally, the reactivity of 9b was examined. Jones et al. reported the formation of [4 + 2] adducts of 6d and 6e with N-phenyltriazolinedione at the inner naphthalene ring and the exo-methylene carbons, respectively, depending on the degree of steric hindrance at each reaction site.9 We found that heating a toluene solution containing 9b (unpurified crude product prepared from diol 11) with 2,3-dichloro-5,6-dicyano-p-benzoquinone (DDQ) in toluene at 70 °C under argon afforded the [4 + 2] adduct 13 (Chart 2), which was isolated in 13% yield from 11. Alternatively, the same product was obtained in 58% yield when dihydro derivative 14, derived from 11 by reduction with triethylsilane and trifluoroacetic acid (ESI†), was treated with DDQ via initial dehydrogenation to generate 9b and subsequent [4 + 2] addition. The structure of 13 revealed by X-ray crystallography (Fig. S7 in ESI†) shows that the addition took place at the inner naphthalene ring. As described above, the addition of oxygen also took place at the inner naphthalene ring of 9b to give endoperoxide 12. This regioselectivity is in contrast to that observed for diphenylindenofluorene 8c which was reported to give both an oxygen adduct and a [4 + 2] adduct with maleic anhydride reacting at the exo-methylene positions.12a,16,27 The difference is ascribed to both electronic and steric reasons. In contrast with 8a,12b the relatively large HOMO coefficient and spin density are located at the inner naphthalene ring of 9a, though the largest ones are at the exo-methylene carbons as shown in Fig. 4 (Fig. S5, S9 and Table S7 in ESI†), indicating relatively high reactivity at the inner positions. Moreover, severe steric hindrance at the methylene carbons of 9b due to the mesityl groups precludes an approach of oxygen or a dienophile, thereby driving the reaction centre to the inner naphthalene ring.16,27
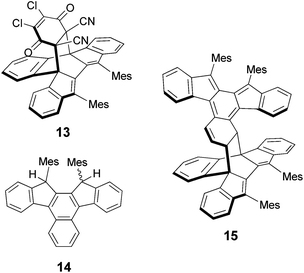 |
| Chart 2 Molecular structures of 13, 14 and 15. | |
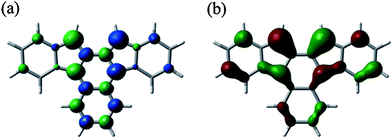 |
| Fig. 4 (a) Spin density of 9a. (b) HOMO of 9a calculated at the B3LYP/6-31G(d) level. | |
Moreover, we found that 9b underwent self-cyclodimerization in a [4 + 2] fashion. When a degassed solution of 9b in toluene-d8 was heated in a sealed tube at 80 °C for 48 h, the [4 + 2] dimer 15 was obtained in 98% yield. The structure of 15 was elucidated by X-ray crystallography (Fig. S8 in ESI†). In 15 the new bonds were formed at the inner naphthalene (4π component) and the outer naphthalene which served as a 2π component. As a consequence of the strong bond length alternation in the outer naphthalene ring of 9b, as discussed above for the X-ray structure of 9b, the double bond is highly activated to serve as a dienophile.
Conclusions
In conclusion, we succeeded in the isolation of 13,14-dimesitylbenz[c]indeno[2,1-a]fluorene (9b), which is the first 2,3-naphthoquinodimethane derivative isolated. 9b was found to be much more reactive compared to indenofluorene 8b due to its increased biradical character. However, incorporation of the 2,3-naphthoquinodimethane unit into the indenofluorene frame, together with effective steric protection exerted by the mesityl groups, kinetically stabilised the system thus allowing study of its characteristic physical and chemical properties.
Acknowledgements
This work was supported by Grant-in-Aid for Scientific Research from the Ministry of Education, Culture, Sports, Science and Technology (Japan). The synchrotron radiation experiments were performed at the BL38B1 of SPring-8 with the approval of the Japan Synchrotron Radiation Research Institute (JASRI) (proposal no 2012B1324). The authors acknowledge Prof. T. Kubo of Osaka University (Japan) for the use of some facilities. The authors are grateful to Dr K. Miura, Dr S. Baba, and Dr N. Mizuno for crystallographic data collection.
Notes and references
- For reviews, see:
(a) W. Oppolzer, Synthesis, 1978, 793–802 CrossRef CAS;
(b) J. L. Charlton and M. M. Alauddin, Tetrahedron, 1987, 43, 2873–2889 CrossRef CAS;
(c) J. L. Segura and N. Martín, Chem. Rev., 1999, 99, 3199–3246 CrossRef CAS PubMed;
(d)
H. Sano and J. Nishimura, Science of Synthesis, ed. J. Siegel and Y. Tobe, Thieme, Stuttgart, 2009, vol. 45a, pp. 483–506 Search PubMed.
-
(a) C. R. Flynn and J. Michl, J. Am. Chem. Soc., 1974, 96, 3280–3288 CrossRef CAS;
(b) R. D. Miller, J. Kolec and J. Michl, J. Am. Chem. Soc., 1976, 98, 8510–8514 CrossRef CAS.
- J. Thiele and H. Balhorn, Chem. Ber., 1904, 37, 1463–1470 CrossRef.
-
(a) A. E. Tschitschibabin, Chem. Ber., 1907, 40, 1810–1819 CrossRef CAS;
(b) L. K. Montgomery, J. C. Huffman, E. A. Jurczak and M. P. Grendze, J. Am. Chem. Soc., 1986, 108, 6004–6011 CrossRef CAS PubMed.
-
(a) G. Quinkert, W.-W. Wiersdorff, M. Finke and K. Opitz, Tetrahedron Lett., 1966, 7, 2193–2200 CrossRef;
(b) G. Quinkert, W.-W. Wiersdorf, M. Finke, K. Opitz and F.-G. von der Haar, Chem. Ber., 1968, 101, 2302–2325 CrossRef CAS.
-
(a) J. Kolc and J. Michl, J. Am. Chem. Soc., 1970, 92, 4147–4148 CrossRef CAS;
(b) J. Kolc and J. Michl, J. Am. Chem. Soc., 1973, 95, 7391–7401 CrossRef CAS.
-
(a) S. Iwashita, E. Ohta, H. Higuchi, H. Kawai, K. Fujiwara, K. Ono, M. Takenaka and T. Suzuki, Chem. Commun., 2004, 2076–2077 RSC;
(b) T. Suzuki, Y. Sakano, T. Iwai, S. Iwashita, Y. Miura, R. Katoono, H. Kawai, K. Fujiwara, Y. Tsuji and T. Fukushima, Chem.–Eur. J., 2013, 19, 117–123 CrossRef CAS PubMed.
-
(a) M. Gisin and J. Wirz, Helv. Chim. Acta, 1976, 59, 2273–2277 CrossRef CAS;
(b) R. P. Steiner, R. D. Miller, H. J. Dewey and J. Michl, J. Am. Chem. Soc., 1979, 101, 1820–1826 CrossRef CAS;
(c) W. P. Cofino, M. Engelsma, D. A. Kamminga, G. Ph. Hoornweg, C. Gooijer, C. MacLean and N. H. Velthost, Spectrochim. Acta, Part A, 1984, 40, 269–273 CrossRef.
- D. W. Jones, A. Pomfret and R. L. Wife, J. Chem. Soc., Perkin Trans. 1, 1983, 459–465 RSC.
- For recent reviews on open-shell singlet biradical hydrocarbons, see:
(a) C. Lambert, Angew. Chem., Int. Ed., 2011, 50, 1756–1758 CrossRef CAS PubMed;
(b) Z. Sun and J. Wu, J. Mater. Chem., 2012, 22, 4151–4160 RSC;
(c) Z. Sun, Q. Ye, C. Chi and J. Wu, Chem. Soc. Rev., 2012, 41, 7857–7889 RSC;
(d) Z. Sun, Z. Zeng and J. Wu, Chem.–Asian J., 2013 DOI:10.1002/asia.201300560.
- For p-QDM congeners, biphenalenes:
(a) T. Kubo, A. Shimizu, M. Sakamoto, M. Uruichi, K. Yakushi, M. Nakano, D. Shiomi, K. Sato, T. Takui, Y. Morita and K. Nakasuji, Angew. Chem., Int. Ed., 2005, 44, 6564–6568 CrossRef CAS PubMed;
(b) T. Kubo, A. Shimizu, M. Uruichi, K. Yakushi, M. Nakano, D. Shiomi, K. Sato, T. Takui, Y. Morita and K. Nakasuji, Org. Lett., 2007, 9, 81–84 CrossRef CAS PubMed;
(c) A. Shimizu, Y. Hirao, K. Matsumoto, H. Kurata, T. Kubo, M. Uruichi and K. Yakushi, Chem. Commun., 2012, 48, 5629–5631 RSC. For zethrene homologs:
(d) Z. Sun, K.-W. Huang and J. Wu, J. Am. Chem. Soc., 2011, 133, 11896–11899 CrossRef CAS PubMed;
(e) Y. Li, W.-K. Heng, B. S. Lee, N. Aratani, J. L. Zafra, N. Bao, R. Lee, Y. M. Sung, Z. Sun, K.-W. Huang, R. D. Webster, J. T. L. Navarrete, D. Kim, A. Osuka, J. Casado, J. Ding and J. Wu, J. Am. Chem. Soc., 2012, 134, 14913–14922 CrossRef CAS PubMed. For anthenes:
(f) A. Konishi, Y. Hirao, M. Nakano, A. Shimizu, E. Botek, B. Champagne, D. Shiomi, K. Sato, T. Takui, K. Matsumoto, H. Kurata and T. Kubo, J. Am. Chem. Soc., 2010, 132, 11021–11023 CrossRef CAS PubMed;
(g) A. Konishi, Y. Hirao, K. Matsumoto, H. Kurata, R. Kishi, Y. Shigeta, M. Nakano, K. Tokunaga, K. Kamada and T. Kubo, J. Am. Chem. Soc., 2013, 135, 1430–1437 CrossRef CAS PubMed. For indeno[1,2-b]fluorenes:
(h) Q. Zhou, P. J. Carroll and T. M. Swager, J. Org. Chem., 1994, 59, 1294–1301 CrossRef CAS;
(i) D. T. Chase, B. D. Rose, S. P. McClintock, L. N. Zakharov and M. M. Haley, Angew. Chem., Int. Ed., 2011, 50, 1127–1130 CrossRef CAS PubMed;
(j) D. T. Chase, A. G. Fix, S. J. Kang, B. D. Rose, C. D. Weber, Y. Zhong, L. N. Zakharov, M. C. Lonergan, C. Nuckolls and M. M. Haley, J. Am. Chem. Soc., 2012, 134, 10349–10352 CrossRef CAS PubMed;
(k) J. Nishida, S. Tsukaguchi and Y. Yamashita, Chem.–Eur. J., 2012, 18, 8964–8970 CrossRef CAS PubMed.
- For an o-QDM congener, indeno[2,1-a]fluorenes:
(a) A. Le Berre, Ann. Chim., 1957, 13, 371–425 Search PubMed;
(b) A. Shimizu and Y. Tobe, Angew. Chem., Int. Ed., 2011, 50, 6906–6910 CrossRef CAS PubMed.
- For a review on indenofluorene isomers:
(a) A. G. Fix, D. T. Chase and M. M. Haley, Top. Curr. Chem. DOI:10.1007/128_2012_376. Recently, Haley and co-workers added a new member of p-QDM, indeno[2,1-c]fluorene, to the indenofluorene family:
(b) A. G. Fix, P. E. Deal, C. L. Vonnegut, B. D. Rose, L. N. Zakharov and M. M. Haley, Org. Lett., 2013, 15, 1362–1365 CrossRef CAS PubMed. We also reported indeno[2,1-b]fluorene, a meta-quinodimethane congener:
(c) A. Shimizu, R. Kishi, M. Nakano, D. Shiomi, K. Sato, T. Takui, I. Hisaki, M. Miyata and Y. Tobe, Angew. Chem., Int. Ed., 2013, 52, 6076–6079 CrossRef CAS PubMed.
- C. F. Koelsch and H. J. Richter, J. Org. Chem., 1938, 3, 465–472 CrossRef CAS.
- Though diol 11 was obtained as a single isomer, its stereochemistry was not determined.
- It should be pointed out that for 11,12-diphenylindeno[2,1-a]fluorene (8c), Le Berre reported that oxygen added to the exo-methylene carbons yielding the corresponding cyclic peroxide which further degradated to give bond cleaved products.12a Similarly, attempts to prepare NQD derivative 9c resulted in the formation of decomposition products derived from oxygenation at the exo-methylene carbons12a.
-
(a) D. W. Jones and A. Pomfret, J. Chem. Soc., Perkin Trans. 1, 1991, 263–267 RSC;
(b) M. Seip and H.-D. Brauer, J. Am. Chem. Soc., 1992, 114, 4486–4490 CrossRef CAS;
(c) C.-H. Chou and W. S. Trahanovsky, J. Org. Chem., 1995, 60, 5449–5451 CrossRef CAS;
(d) D. Ghereg, S. E.-C. E. Kettani, M. Lazraq, H. Ranaivonjatovo, W. W. Schoeller, J. Escudié and H. Gornitzka, Chem. Commun., 2009, 4821–4823 RSC.
- Y. Takano, T. Taniguchi, H. Isobe, T. Kubo, Y. Morita, K. Yamamoto, K. Nakasuji, T. Takui and K. Yamaguchi, J. Am. Chem. Soc., 2002, 124, 11122–11130 CrossRef CAS PubMed.
-
(a) P. v. R. Schleyer, H. Jiao, N. J. R. v. E. Hommes, V. G. Malkin and O. L. Malkina, J. Am. Chem. Soc., 1997, 119, 12669–12670 CrossRef CAS;
(b) P. v. R. Schleyer, M. Manoharan, Z.-X. Wang, B. Kiran, H. Jiao, R. Puchta and N. J. R. v. E. Hommes, Org. Lett., 2001, 3, 2465–2468 CrossRef CAS PubMed.
-
(a) L. Salem and C. Rowland, Angew. Chem., Int. Ed. Engl., 1972, 11, 92–111 CrossRef CAS;
(b) V. Bonačić-Koutecký, J. Koutecký and J. Michl, Angew. Chem., Int. Ed. Engl., 1987, 26, 170–189 CrossRef;
(c) J. Fabian, H. Nakazumi and M. Matsuoka, Chem. Rev., 1992, 92, 1197–1226 CrossRef CAS.
-
(a) D. Döhnert and J. Koutecký, J. Am. Chem. Soc., 1980, 102, 1789–1796 CrossRef;
(b) Y. Jung and M. Head-Gordon, ChemPhysChem, 2003, 4, 522–525 CrossRef CAS PubMed.
- K. Yamaguchi, Chem. Phys. Lett., 1975, 33, 330–335 CrossRef CAS.
- For the geometry optimisation of 8a and 9a, RB3LYP/6–31G(d) and UB3LYP/6-31G(d) levels of theory were used, respectively.
- C. P. Brock and J. D. Dunitz, Acta Crystallogr., Sect. B: Struct. Crystallogr. Cryst. Chem., 1982, 38, 2218–2228 CrossRef.
- T. Uto, T. Nishinaga, A. Matsuura, R. Inoue and K. Komatsu, J. Am. Chem. Soc., 2005, 127, 10162–10163 CrossRef CAS PubMed.
- For discussions on the Mills–Nixon effect, see:
(a) A. Stanger, J. Am. Chem. Soc., 1991, 113, 8277–8280 CrossRef CAS;
(b) K. K. Baldridge and J. S. Siegel, J. Am. Chem. Soc., 1992, 114, 9583–9587 CrossRef CAS.
- Le Berre reported that maleic anhydride added to the exo-methylene carbons of 11,12-diphenylindeno[2,1-a]fluorene (8c), though the structure was not concretely established.12a Similarly, an attempt to prepare NQD derivative 9c in the presence of maleic anhydride gave an adduct at the exo-methylene carbons.12a On the other hand, we reported that dimesityl derivative 8b did not react with maleic anhydride when a toluene solution of them was heated at 100 °C,12b showing the efficient protecting effect of the mesityl groups.
Footnotes |
† Electronic supplementary information (ESI) available: Experimental procedures, analytical data for compounds prepared in this work, cyclic voltammetry, and theoretical calculations. CCDC 960644, 960645, 960647, and 960648. For ESI and crystallographic data in CIF or other electronic format see DOI: 10.1039/c3sc52622d |
‡ Present address: Department of Chemistry, Graduate School of Science, Nagoya University, Nagoya 464-8602, Japan. |
§ Present address: Department of Synthetic and Biological Chemistry, Graduate School of Engineering, Kyoto University, Kyoto 615-8510, Japan. |
|
This journal is © The Royal Society of Chemistry 2014 |