Ruthenium-catalyzed C–H/O–H and C–H/N–H bond functionalizations: oxidative annulations of cyclopropyl-substituted alkynes†
Received
1st July 2012
, Accepted 11th October 2012
First published on 18th October 2012
Abstract
The chemical behavior of cyclopropyl-substituted alkynes has been probed using the reaction conditions of ruthenium-catalyzed oxidative C–H/O–H and C–H/N–H bond functionalizations. The oxidative annulations proceeded with complete conservation of all cyclopropane fragments and allowed for the one-step preparation of synthetically useful cyclopropyl-substituted isocoumarins and isoquinolones with high regioselectivities and chemical yields. The connectivities of the key heterocyclic products were unambiguously established by X-ray diffraction analysis.
Introduction
Transition metal-catalyzed reactions have matured to being among the most powerful tools in organic chemistry.1 Particularly, direct functionalizations2 of aromatic C–H bonds have received considerable recent attention as ecologically and economically friendly synthetic methods, that avoid the use of prefunctionalized starting materials. Yet, an even more sustainable strategy is represented by the simultaneous transition metal-catalyzed C–H/O–H or C–H/N–H bond functionalizations utilizing arenes or alkenes 1 (Scheme 1).
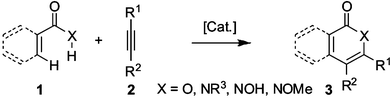 |
| Scheme 1 Oxidative annulations by C–H/O–H or C–H/N–H bond functionalizations. | |
This approach allowed for the one-pot preparation of synthetically useful heterocycles 3, such as substituted isoquinolinones, isoquinolones, isocoumarins, α-pyrones and 2-pyridones, many of which possess valuable biological activities3a–e and represent key structural scaffolds of naturally occurring compounds.3f–l Consequently, a number of valuable protocols for intermolecular oxidative annulations with alkynes 2 have been developed,4,5 with ruthenium-catalyzed4 cyclizations being among the most promising.
The attachment of a cyclopropyl fragment to heterocyclic molecules frequently modifies their pharmacological properties and significantly enhances their biological activities.6 For instance, a number of well-known antibacterial agents, such as Ciprofloxacin, Sparfloxacin, Grepafloxacin and WIN-57294, contain a 1-cyclopropylquinolin-4(1H)-one moiety.6e,f However, the inherently high molecular strain in cyclopropanes (28.1 kcal mol−1)7 is reflected by some specificity to the chemistry of cyclopropane-containing molecules. As a consequence, metal-mediated or -catalyzed reactions with participation of (cyclopropylcarbinyl) metal intermediates proceeded almost exclusively via opening of at least one cyclopropane ring,8 whereas formation of cyclopropane-containing compounds was observed as a side reaction only. Given the remarkable biological activity of cyclopropyl-decorated heterocycles along with the challenges associated with the use of cyclopropyl-substituted alkynes 2 in transition-metal catalysis, we, thus, became attracted to exploring their unprecedented oxidative annulations by metal-catalyzed C–H/Het–H bond functionalization with rather inexpensive ruthenium catalysts, on which we wish to report herein.
Results and discussion
To elucidate the synthetic versatility of the transformation as well as the influence and reactivity of cyclopropyl substituents in the alkynes 2, we set out to perform detailed synthetic and structural studies on atom- and step-economical ruthenium-catalyzed C–H/O–H and C–H/N–H bond functionalizations of representative benzoic acids 4a–d and benzamides 5a–i with various cyclopropylacetylenes 2 (Fig. 1).
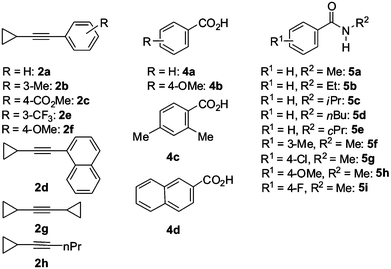 |
| Fig. 1 Substituted ethynylcyclopropanes 2, benzoic acids 4 and benzamides 5 used in C–H/Het–H bond functionalizations. | |
We initiated our studies by exploring the reactivity of substituted benzoic acids 4 and cyclopropylarylethynes 2 in ruthenium-catalyzed oxidative annulations4f (Scheme 2). Notably, arylalkynes displaying a cyclopropyl substituent yielded the desired product through the C–H/O–H bond functionalizations reaction manifold. Thus, the highest yield was observed for the alkyne 2a in the reaction with the acid 4c, while the yield decreased with alkyne 2e possessing an electron-withdrawing substituent. However, the influence of the substituents in benzoic acids 4 was found to be less pronounced.
![Ruthenium-catalyzed C–H/O–H bond functionalizations with benzoic acids 4 and (cyclopropylethynyl)benzenes 2. a[RuCl2(p-cymene)]2 (5 mol%).](/image/article/2013/OB/c2ob26250a/c2ob26250a-s2.gif) |
| Scheme 2 Ruthenium-catalyzed C–H/O–H bond functionalizations with benzoic acids 4 and (cyclopropylethynyl)benzenes 2. a[RuCl2(p-cymene)]2 (5 mol%). | |
No product formation was observed in the reactions of substrate 4a with alkyne 2d and when using starting materials 4d and 2a, while the corresponding isocoumarin 6dk was formed in the reaction of 4d with diethylethyne in 31% yield.†
The influence of the cyclopropyl moieties upon the course of chemical transformations by the stabilization of key reaction intermediates due to conjugation is well documented.9 However, several representative experiments with p-anisic acid (4b) and selected alkynes 2 (Table 1) indicated the cyclopropyl substituents in the alkynes 2 to translate into a reduction of their reactivity (entry 1), but demonstrated no significant influence onto the regioselectivity of the annulation. Indeed, the reaction with (pent-1-ynyl)cyclopropane (2h) furnished a 1
:
1 mixture of the two regioisomeric isocoumarins 6bh and 7bh (entry 2), thereby indicating a negligible effect of steric interactions10 in these annulations. Furthermore, the electronic effect of the cyclopropane appeared to be of less importance for the overall regioselectivity.
Table 1 Ruthenium-catalyzed C–H/O–H bond functionalizations with p-anisic acid (4b) and selected substituted alkynes 2
Entry |
Alkyne |
R1 |
R2 |
Product 6/yield (%) |
Product 7/yield (%) |
1 |
2g
|
cPr |
cPr |
6bg = 7bg |
7bg/27 |
2 |
2h
|
cPr |
nPr |
6bh/7bh 1 : 1/53 |
3 |
2i
|
Et |
Ph |
6bi/47 |
7bi/5 |
It is noteworthy that the rhodium-catalyzed annulation of unsymmetrically substituted arylalkyl alkynes Alk–C
C–Ar delivered mixtures of 3- and 4-arylsubstituted regioisomeric products11,12 in ratios of 6
:
1 to 8
:
1.5i,j In spite of the previously reported almost exclusive formation of 3-arylsubstituted products,4e the cyclization of alkyne 2i afforded in our hands heterocycles 7bi and 6bi in a ratio of 9.4
:
1 (Table 1, entry 3).
In the ruthenium-catalyzed cyclizations of unsymmetrical cyclopropylacetylenes the ratios of regioisomeric products 6 and 7 varied from 1
:
1 to 13
:
1 (Scheme 2 and Table 1). To elaborate spectroscopic criteria† for this group of cyclopropyl-substituted heterocycles, the structures of cyclopropylisocoumarins 6aa, 6ae and 7aa have been unambiguously established by X-ray diffraction analysis (Fig. 2). Notably, both 3-aryl-4-cyclopropyl-substituted heterocycles 6aa and 6ae adopted essentially the same conformations with the dihedral angles C1–C2–C3–center (C4–C5) being equal to −99.5° and −96.8°, respectively, while the dihedral angles between heterocyclic and carbocyclic aromatic moieties were found to be 47.3 and 37.6°, respectively. In contrast, in 7aa the dihedral angle C1–C2–C3–centre (C4–C5) = 179.4° with the angle between aromatic planes of 66.5°, i.e. with almost ideal conditions for the conjugation of cyclopropyl and isocoumarin fragments.†
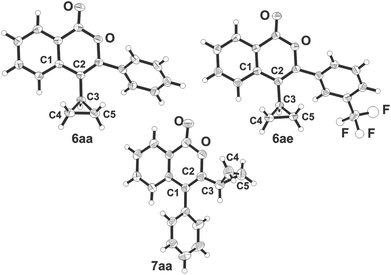 |
| Fig. 2 Molecular structures of isocoumarins 6aa, 6ae and 7aa in the crystal13 (numbering does not correspond to the IUPAC rules). | |
Somewhat similar tendencies were observed in ruthenium-catalyzed C–H/N–H bond functionalizations in benzamides 5 with (cyclopropylethynyl)benzenes 2, the results of which are summarized in Scheme 3. Generally, the oxidative annulations occurred with high yields and synthetically useful regioselectivity. While the catalytic system displayed a high functional group tolerance, the attempted cyclization of amide 5a with cyclopropylnaphthylalkyne 2d gave less satisfactory results.† Furthermore, cyclopropylaryl alkynes 2c and 2e possessing electron-withdrawing substituents on the aromatic rings delivered the corresponding products 8ac and 8ae, respectively, in somewhat lower yield. However, a number of oxidative cyclizations, including the reaction of the parent compounds 2a and 5a, proceeded in a regiospecific manner affording only regioisomers 8 (8aa, 8ac, 8ae, 8af and 8ca), while in the other cases the ratio of heterocycles 8 and 9 varied from 9.8 (8ia) to 3.6 (8ab). Surprisingly, the best yield (83%) was obtained when utilizing dicyclopropylacetylene (2g), which was less reactive in the ruthenium-catalyzed isocoumarin synthesis (vide supra). Unfortunately, the attempted synthesis of N-cyclopropyl-3(4)-cyclopropylisoquinolones 8ea and 9ea occurred less efficiently. Moreover, the benzamide 5e did not react with diphenylacetylene (tolane) under otherwise identical reaction conditions.
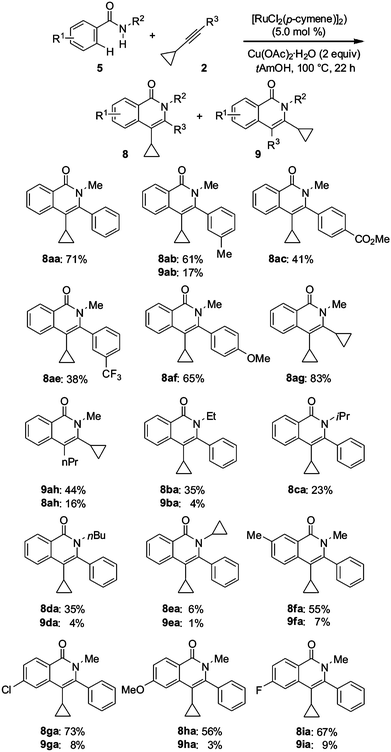 |
| Scheme 3 Ruthenium-catalyzed C–H/N–H bond functionalizations in benzamides 5 with (cyclopropylethynyl)benzenes 2. | |
Remarkably, the nitrogen substituent had a considerable effect on the outcome of the annulation. Thus, N-Me- (5a), N-Et- (5b), N-nBu- (5d) and N-iso-propylbenzamides (5c) furnished the corresponding isoquinolones 8 and 9 in the reactions with the alkyne 2a in 71, 39, 39 and 23% yield, respectively. This is in line with the values of steric substituent constants of these alkyl groups, which are equal to 0, 0.86, 0.86 and 2.29, respectively.10 Yet, in contrast to the synthesis of isocoumarins, the reaction of cyclopropyl-n-propyl alkyne (2h) with benzamide 5a afforded a 2.75
:
1 mixture of 3-cyclopropyl (9ah) and 4-cyclopropylisoquinolones (8ah) (Scheme 3), in spite of the steric substituent constants being equal to 0.89 (n-propyl) and 1.33 (cyclopropyl).10
The exact connectivities of the parent isoquinolone 8aa and of the fundamentally important compound 8ah have been established by X-ray diffraction analysis (Fig. 3). As for the analogous isocoumarins 6aa and 6ae, heterocycles 9aa and 9ah adopted essentially the same conformations with the dihedral angles C1–C2–C3–center (C4–C5) equal to 94.7° and 91.7°, respectively, i.e. without steric preconditions for conjugation, while the dihedral angle between heterocyclic and phenyl aromatic moieties in 8aa was determined to be 63.2°.
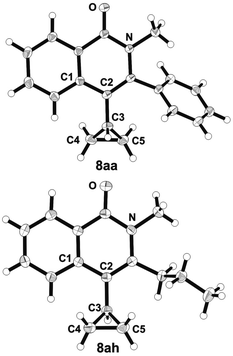 |
| Fig. 3 Molecular structures of isoquinolones 8aa and 8ah in the crystal13 (numbering does not correspond to the IUPAC rules). | |
It is particularly noteworthy that all the ruthenium-catalyzed oxidative C–H/Het–H bond functionalizations proceeded with complete conservation of all cyclopropane fragments.8,14 As a consequence, cyclopropylethynes can be utilized in ruthenium-catalyzed C–H/Het–H bond functionalizations for the preparation of valuable cyclopropyl-substituted heterocycles. According to the mechanistic rationalizations generally accepted for ruthenium-catalyzed C–H/Het–H bond functionalization with alkynes,4a,b,d–g the regiochemistry-determining step of these oxidative reactions is constituted by the insertion of alkyne 2 into the ruthenium–carbon bond of key intermediate 10 to give the seven-membered ruthenacycle 11 (Scheme 4). Thus, the observed regioselectivities can for instance be rationalized by the enhanced thermodynamic stability of regioisomer 11, in which the aryl substituent is in the neighboring position to the ruthenium.
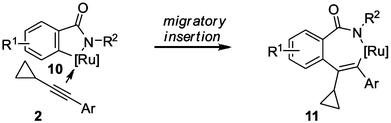 |
| Scheme 4 Regiochemistry-determining step of the ruthenium-catalyzed C–H/Het–H and C–H/N–H bond functionalizations. | |
Conclusions
In summary, we have examined the behavior of cyclopropyl-substituted acetylenes in the ruthenium-catalyzed oxidative C–H/O–H and C–H/N–H bond functionalizations and have found these reactions to proceed with complete conservation of all cyclopropane fragments. Thereby, these cyclizations allowed the one-step preparation of synthetically useful cyclopropyl-substituted isocoumarins and isoquinolones in high yields and regioselectivities.15
Experimental
Syntheses: general methods
Catalytic reactions were carried out using pre-dried glassware. Triethylamine and tAmOH were distilled over CaH2 and sodium, respectively. Anhydrous THF was obtained by distillation over sodium benzophenone ketyl. (Cyclopropylethynyl)benzene (2a), 1-(cyclopropylethynyl)-3-methylbenzene (2b), methyl 4-(cyclopropylethynyl)benzoate (2c), 1-(cyclopropylethynyl)naphthalene (2d), 1-(cyclopropylethynyl)-4-methoxylbenzene (2f), the previously unknown 1-(cyclopropylethynyl)-3-(trifluoromethyl)benzene (2e), (pent-1-ynyl)cyclopropane (2h), 1,2-dicyclopropylethyne (2g), N-cyclopropylbenzamide (5e), N,3-dimethylbenzamide (5f), 4-chloro-N-methylbenzamide (5g), 4-methoxy-N-methylbenzamide (5h), 4-fluoro-N-methylbenzamide (5i) and [RuCl2(p-cymene)]2 were synthesized as indicated in the ESI.† Other chemicals were obtained from commercial sources and were used without further purification. Yields refer to isolated compounds, estimated to be >95% pure as determined by 1H-NMR and GC. TLC: Macherey-Nagel, TLC plates Alugram® Sil G/UV254. Detection under UV light at 254 nm. Chromatography: Separations were carried out on Merck Silica 60 (0.040–0.063 mm, 70–230 mesh ASTM). All IR spectra were recorded on a BRUKER ALPHA-P spectrometer. MS: EI-MS: Finnigan MAT 95, 70 eV; ESI-MS: Finnigan LCQ. High resolution mass spectrometry (HRMS): APEX IV 7T FTICR, Bruker Daltonic. M.p.: Stuart® Melting Point Apparatus SMP3 melting point apparatus, values are uncorrected. 1H, 13C, 19F NMR-spectra were recorded at 300 (1H), 75.5 {13C, APT (Attached Proton Test)} and 283 MHz (19F), respectively, on a Varian Unity-300 instrument.
General procedure for ruthenium-catalyzed C–H/O–H bond functionalizations in benzoic acids 4 with ethynylbenzenes 2.
A suspension of acid 4 (2.00 mmol), alkyne 2 (1.00 mmol), [RuCl2(p-cymene)]2 (15.5 mg, 2.5 mol%), KPF6 (36.8 mg, 20 mol%) and Cu(OAc)2·H2O (300 mg, 1.50 mmol) in tAmOH (3.0 mL) was stirred at 120 °C for 16 h. After cooling to ambient temperature, the reaction mixture was diluted with sat. aq. NH4Cl–NH3 solution (1
:
1, 50 mL) and extracted with EtOAc (4 × 25 mL). The combined organic layers were washed with NH4Cl–NH3 solution (1
:
1, 2 × 20 mL) and dried over MgSO4. After filtration and concentration of the solution under reduced pressure, the crude product was purified by column chromatography on silica gel (n-hexane–EtOAc 25
:
1) to yield 6 and 7 as colorless solids.
4-Cyclopropyl-3-phenyl-1H-isochromen-1-one (6aa) and 3-cyclopropyl-4-phenyl-1H-isochromen-1-one (7aa).
According to the general procedure, benzoic acid (4a) (490 mg, 4.0 mmol), alkyne (2a) (284 mg, 2.0 mmol), [RuCl2(p-cymene)]2 (62.0 mg, 100 μmol), KPF6 (73 mg, 0.4 mmol) and Cu(OAc)2·H2O (599 mg, 3.0 mmol) yielded compounds 6aa (353 mg, 67%) and 7aa (64 mg, 11%) as colorless solids. 6aa: m.p. = 115–117 °C; 1H NMR (300 MHz, CDCl3) δ = 8.33 (dd, J = 8.0, 1.4 Hz, 1H), 8.12 (d, J = 8.2, 1H), 7.78 (td, J = 7.7, 1.41 Hz, 1H), 7.76–7.72 (m, 2H), 7.50 (td, J = 7.7, 1.1 Hz, 1H), 7.46–7.37 (m, 3H), 1.91 (tt, J = 8.1, 5.5 Hz, 1H), 1.00–0.87 (m, 2H), 0.26–0.06 (m, 2H); 13C NMR (75 MHz, CDCl3) δ = 162.4 (C), 153.4 (C), 139.7 (C), 134.4 (CH), 133.2 (C), 129.5 (CH), 129.4 (CH), 129.3 (CH), 127.8 (CH), 127.7 (CH), 124.5 (CH), 120.6 (C), 114.5 (C), 9.9 (CH2), 9.0 (CH); IR (ATR): 3011, 2961, 2922, 2855, 1718, 1629, 1078, 700 cm−1; MS (EI) m/z (%): 262 (M+, 100), 247 (50), 234 (35), 165 (65), 69 (25); HRMS (EI) m/z calcd for C18H14O2 (M+) 262.0994, found 262.0988.
7aa: m.p. = 100–102 °C; 1H NMR (300 MHz, CDCl3) δ = 8.20 (dd, J = 7.9, 1.6 Hz, 1H), 7.57–7.33 (m, 7H), 6.95 (ddd, J = 8.1, 1.1, 0.5 Hz, 1H), 1.70–1.56 (m, 1H), 1.21–1.12 (m, 2H), 0.81–0.70 (m, 2H); 13C NMR (75 MHz, CDCl3) δ 162.0 (C), 154.2 (C), 138.8 (C), 134.4 (CH), 134.2 (C), 131.0 (2CH), 129.3 (CH), 128.8 (CH), 127.9 (CH), 126.7 (CH), 123.9 (CH), 119.5 (C), 115.0 (C), 12.0 (CH), 7.6 (CH2); IR (ATR): 3084, 3049, 3008, 2984, 1727, 1604, 1075, 672 cm−1; MS (EI) m/z (relative intensity): 262 (M+, 50), 217 (45), 185 (40), 105 (55), 77 (100); HRMS (EI) calcd for C18H14O2 (M+) 262.0994, found 262.0996.
The connectivities of compounds 6aa and 7aa were established by X-ray crystal structure analysis.13
4-Cyclopropyl-6-methoxy-3-phenyl-1H-isochromen-1-one (6ba) and 3-cyclopropyl-6-methoxy-4-phenyl-1H-isochromen-1-one (7ba).
According to the general procedure, p-anisic acid (4b) (152 mg, 4.0 mmol), alkyne 2a (284 mg, 2.0 mmol), [RuCl2(p-cymene)]2 (31 mg, 50 μmol), KPF6 (73 mg, 0.4 mmol) and Cu(OAc)2·H2O (599 mg, 3.0 mmol) yielded 6ba (308 mg, 53%) and 7ba (23 mg, 4%) after column chromatography as colorless solids. 6ba: m.p. = 144–146 °C; 1H NMR (300 MHz, CDCl3) δ = 8.27 (d, J = 8.8 Hz, 1H), 7.75–7.71 (m, 1H), 7.50 (d, J = 2.5 Hz, 1H), 7.46–7.40 (m, 2H), 7.06 (dd, J = 8.8, 2.5 Hz, 1H), 3.97 (s, 2H), 1.88 (tt, J = 8.1, 5.5 Hz, 1H), 0.98–0.88 (m, 1H), 0.23–0.15 (m, 1H); 13C NMR (75 MHz, CDCl3) δ = 164.6 (C), 162.3 (C), 154.2 (C), 142.2 (C), 133.4 (C), 131.9 (CH), 129.6 (CH), 129.6 (CH), 129.5 (CH), 127.9 (CH), 115.5 (CH), 114.4 (C), 113.9 (C), 107.7 (CH), 55.7 (CH3), 10.0 (CH2), 9.2 (CH); IR (ATR): 3005, 2956, 2856, 1711, 1600, 1444, 1226, 831 cm−1; MS (EI) m/z (%): 292 (M+, 95), 217 (36), 215 (77), 105 (90), 77 (100); HRMS (EI) calcd for C19H16O3 (M+) 292.1099; found 292.1099.
7ba: m.p. = 126–128 °C; 1H NMR (300 MHz, CDCL3) δ = 8.22 (d, J = 8.8 Hz, 1H), 7.54–7.34 (m, 5H), 6.94 (dd, J = 8.8, 2.5 Hz, 1H), 6.35 (d, J = 2.5 Hz, 1H), 3.72 (s, 3H), 1.67–1.55 (m, 1H), 1.23–1.13 (m, 2H), 0.85–0.74 (m, 2H); 13C NMR (75 MHz, CDCl3) δ = 164.6 (C), 161.9 (C), 155.0 (C), 141.4 (C), 134.5 (C), 131.9 (CH), 131.1 (CH), 129.0 (CH), 128.0 (C), 115.1 (C), 114.6 (CH), 113 0 (C), 107.1 (CH), 55.6 (CH3), 12.2 (CH), 7.8 (CH2); IR (ATR): 3009, 2925, 1713, 1600, 1231, 1044, 849, 723 cm−1; MS (EI) m/z (%): 292 (M+, 100), 277 (32), 264 (55), 195 (35), 152 (50); HRMS (EI) calcd for C19H16O3 (M+) 292.1099, found 292.1105.
Synthesis of cyclopropyl-substituted isoquinolones 8 and 9
General procedure for ruthenium-catalyzed C–H/N–H bonds functionalizations in benzamides 5 with (cyclopropylethynyl)benzenes 2.
A mixture of benzamide 5 (0.50 mmol), acetylene 2 (1.00 mmol), [RuCl2(p-cymene)]2 (15.3 mg, 25 μmol, 5.0 mol%) and Cu(OAc)2·H2O (200 mg, 1.00 mmol) in tAmOH (2.0 mL) was stirred at 100 °C under a nitrogen atmosphere for 22 h. After cooling the mixture to ambient temperature, the reaction mixture was diluted with aq. NH3 solution (75 mL, 1.0 wt%) and extracted with EtOAc (3 × 75 mL). The combined organic phase was washed with brine (50 mL) and dried over Mg2SO4. After filtration and concentration of the solution under reduced pressure, the crude product was purified by column chromatography on silica gel (n-hexane–EtOAc 4
:
1) to yield 8 and 9 as colorless solids.
4-Cyclopropyl-2-methyl-3-phenylisoquinolin-1(2H)-one (8aa).
According to the general procedure, N-methylbenzamide (5a) (135 mg, 1.0 mmol), alkyne 2a (284 mg, 2.0 mmol), [RuCl2(p-cymene)]2 (31 mg, 50 μmol), and Cu(OAc)2·H2O (399 mg, 2.0 mmol) yielded 8aa (185 mg, 71%) after column chromatography as a colorless solid, m.p. = 139–141 °C; 1H NMR (300 MHz, CDCl3) δ = 8.49 (ddd, J = 8.0, 1.5, 0.6 Hz, 1H), 8.19 (ddd, J = 8.3, 1.2, 0.6 Hz, 1H), 7.68 (ddd, J = 8.4, 7.1, 1.5 Hz, 1H), 7.51–7.39 (m, 4H), 7.31–7.27 (m, 2H), 3.28 (s, 3H), 1.62 (tt, J = 8.3, 5.6 Hz, 1H), 0.69–0.55 (m, 2H), 0.17–0.05 (m, 2H); 13C NMR (75 MHz, CDCl3) δ = 162.8 (C), 142.9 (C), 138.0 (C), 135.7 (C), 133.7 (CH), 129.9 (CH), 128.4 (CH), 128.4 (CH), 127.9 (CH), 126.2 (CH), 125.1 (C), 124.4 (CH), 115.0 (C), 34.2 (CH3), 10.5 (CH), 8.7 (CH2); IR (ATR): 3081, 2994, 2957, 2924, 1643, 1587, 1025, 759 cm−1. MS (EI) m/z (%): 275 (M+, 32), 274 (100), 246 (15), 198 (27), 77 (30); HRMS (EI) calcd for C19H17NO (M+) 275.1310, found 275.1298. The structure of compound 8aa was established by X-ray crystal structure analysis.13
4-Cyclopropyl-6-methoxy-2-methyl-3-phenylisoquinolin-1(2H)-one (8ha) and 3-cyclopropyl-6-methoxy-2-methyl-4-phenylisoquinolin-1(2H)-one (9ha).
According to the general procedure, 4-methoxy-N-methylbenzamide (5h) (165 mg, 1.0 mmol), alkyne 2a (284 mg, 2.0 mmol), [RuCl2(p-cymene)]2 (31 mg, 50 μmol), and Cu(OAc)2·H2O (399 mg, 2.0 mmol) yielded 8ha (173 mg, 56%) and 9ha (9 mg, 3%) after column chromatography as colorless solids. 8ha: m.p. = 135–137 °C; 1H NMR (300 MHz, CDCl3) δ = 8.39 (d, J = 8.9 Hz, 1H), 7.53 (d, J = 2.5 Hz, 1H), 7.48–7.37 (m, 3H), 7.29–7.23 (m, 2H), 7.04 (dd, J = 8.9, 2.5 Hz, 1H), 3.91 (d, J = 1.5 Hz, 3H), 3.24 (d, J = 1.4 Hz, 3H), 1.57 (tt, J = 8.2, 5.6 Hz, 1H), 0.63–0.54 (m, 2H), 0.13–0.05 (m, 2H); 13C NMR (75 MHz, CDCl3) δ = 162.3 (C), 162.2 (C), 143.5 (C), 140.0 (C), 135.6 (C), 129.9 (CH), 129.8 (CH), 128.3 (CH), 119.0 (C), 115.1 (C), 114.4 (C), 109.1 (C), 106.9 (CH), 55.2 (CH3), 33.9 (CH3), 10.4 (CH), 8.6 (CH2); IR (ATR): 2991, 2939, 2914, 1639, 1604, 1275, 1027, 779, 725, 687 cm−1; MS (EI) m/z (%): 305 (M+, 52), 304 (84), 274 (100), 228 (68), 185 (20), 118 (30), 84 (40), 77 (46); HRMS (EI) calcd for C20H19NO2 (M+) 305.1416; found: 305.1416.
9ha: m.p. = 145–147 °C; 1H NMR (300 MHz, CDCl3) δ = 8.39 (d, J = 8.9 Hz, 1H), 7.47–7.33 (m, 3H), 7.28 (d, J = 1.7 Hz, 1H), 7.25 (t, J = 1.5 Hz, 1H), 6.99 (dd, J = 8.9, 2.5 Hz, 1H), 6.59 (d, J = 2.5 Hz, 1H), 3.81 (s, 3H), 3.68 (s, 3H), 1.72 (tt, J = 8.3, 5.7 Hz, 1H), 0.75–0.66 (m, 2H), 0.34–0.25 (m, 2H); 13C NMR (75 MHz, CDCl3) δ = 162.6 (C), 162.3 (C), 140.8 (C), 138.9 (C), 137.3(C), 131.4 (CH2), 129.9 (CH), 128.3 (CH), 127.1 (CH), 119.4 (C), 118.7 (C), 115.0 (CH), 106.5 (CH), 55.2 (CH3), 32.1 (CH3), 14.0 (CH), 10.2 (CH2); IR (ATR): 3057, 2974, 2939, 2914, 1643, 1586, 1225, 1025, 781, 705 cm−1; MS (EI) m/z (%): 305 (M+, 94), 290 (100), 277 (33), 152 (21), 82 (13), 41 (10); HRMS (EI) calcd for C20H19NO2 (M+) 305.1416; found: 305.1417.
Acknowledgements
Support by Astra-Zeneca and the EPSRC (UK) is gratefully acknowledged.
Notes and references
-
(a)
Metal-Catalyzed Cross-Coupling Reactions, ed. A. de Meijere and F. Diederich, Wiley-VCH, Weinheim, 2nd edn, 2004 Search PubMed;
(b)
Transition Metals for Organic Synthesis, ed. M. Beller and C. Bolm, Wiley-VCH, Weinheim, 2nd edn, 2004 Search PubMed.
- For selected recent reviews on C–H bond functionalization, see:
(a)
, Acc. Chem. Res., 2012, 45 Search PubMed , special issue 6 “C–H Functionalization”; ;
(b)
L. Ackermann, A. R. Kapdi, H. K. Potukuchi and S. I. Kozhushkov, in Handbook of Green Chemistry, ed. C.-J. Li, Wiley-VCH, Weinheim, 2012, pp. 259–305 Search PubMed;
(c) L. Ackermann, Chem. Rev., 2011, 111, 1315–1345 CrossRef CAS;
(d) C. S. Yeung and V. M. Dong, Chem. Rev., 2011, 111, 1215–1292 CrossRef CAS;
(e) C.-L. Sun, B.-J. Li and Z.-J. Shi, Chem. Rev., 2011, 111, 1293–1314 CrossRef CAS;
(f) O. Baudoin, Chem. Soc. Rev., 2011, 40, 4902–4911 RSC;
(g) S. H. Cho, J. Y. Kim, J. Kwak and S. Chang, Chem. Soc. Rev., 2011, 40, 5068–5083 RSC;
(h) Y. Nakao, Synthesis, 2011, 3209–3219 CrossRef CAS;
(i) L. Ackermann and H. K. Potukuchi, Org. Biomol. Chem., 2010, 8, 4503–4513 RSC;
(j)
, Chem. Rev., 2010, 110 Search PubMed , special issue 2 “Selective Functionalization of C–H Bonds” ;
(k) L. Ackermann, R. Vicente and A. Kapdi, Angew. Chem., Int. Ed., 2009, 48, 9792–9826 CrossRef CAS;
(l) X. Chen, K. M. Engle, D.-H. Wang and J.-Q. Yu, Angew. Chem., Int. Ed., 2009, 48, 5094–5115 CrossRef CAS;
(m) F. Bellina and R. Rossi, Tetrahedron, 2009, 65, 10269–10310 CrossRef CAS;
(n) A. A. Kulkarni and O. Daugulis, Synthesis, 2009, 4087–4109 CAS;
(o) L. Joucla and L. Djakovitch, Adv. Synth. Catal., 2009, 351, 673–714 CrossRef CAS;
(p)
Modern Arylation Methods, ed. L. Ackermann, Wiley-VCH, Weinheim, 2009 Search PubMed;
(q) L. Ackermann, Top. Organmet. Chem., 2007, 24, 35–60 CrossRef CAS;
(r) D. Alberico, M. E. Scott and M. Lautens, Chem. Rev., 2007, 107, 174–238 CrossRef CAS;
(s) D. R. Stuart and K. Fagnou, Aldrichimica Acta, 2007, 40, 35–41 Search PubMed;
(t) I. V. Seregin and V. Gevorgyan, Chem. Soc. Rev., 2007, 36, 1173–1193 RSC;
(u) L. Ackermann, Synlett, 2007, 507–526 CrossRef CAS;
(v) T. Satoh and M. Miura, Chem. Lett., 2007, 36, 200–205 CrossRef CAS.
- For selected examples, lifespan-altering activity towards eukaryotic organisms:
(a)
D. S. Goldfarb, US Pat, US 20090163545 A1, 2009, antituberculosis properties Search PubMed;
(b) I. V. Ukrainets, L. V. Sidorenko, O. V. Gorokhova, V. B. Rybakov, V. V. Chernyshev and E. V. Kolesnik, Visn. Farm., 2004, 7–12 CAS ; modulation of PI3 kinase: ;
(c)
P. Ren, Y. Liu, T. E. Wilson, L. Li, K. Chan and C. Rommel, PCT Int. Appl., WO 2011008302 A1, 2011 Search PubMed;
(d)
P. Ren, Y. Liu, T. E. Wilson, L. Li, K. Chan and C. Rommel, U.S. Pat. Appl., US 20090312319 A1, 2009; antifungal, antibacterial, and antidiabetic effects Search PubMed;
(e) S. Cai, F. Wang and C. Xi, J. Org. Chem., 2012, 77, 2331–2336 CrossRef CAS , and references cited therein; ;
(f) V. Rukachaisirikul, A. Rodglin, Y. Sukpondma, S. Phongpaichit, J. Buatong and J. Sakayaroj, J. Nat. Prod., 2012, 75, 853–858 CrossRef CAS;
(g) W. K. Liu, Y. H. Ling, F. W. K. Cheung and C.-T. Che, J. Nat. Prod., 2012, 75, 586–590 CrossRef CAS;
(h) E.-J. Park, E. Kiselev, M. Conda-Sheridan, M. Cushman and J. M. Pezzuto, J. Nat. Prod., 2012, 75, 378–384 CrossRef CAS;
(i) R. J. R. Jaeger and P. Spiteller, J. Nat. Prod., 2010, 73, 1350–1354 CrossRef CAS;
(j) M. Kumarihamy, F. R. Fronczek, D. Ferreira, M. Jacob, S. I. Khan and N. P. D. Nanayakkara, J. Nat. Prod., 2010, 73, 1250–1253 CrossRef CAS;
(k) J. Kornsakulkarn, C. Thongpanchang, S. Lapanun and K. Srichomthong, J. Nat. Prod., 2009, 72, 1341–1343 CrossRef CAS;
(l) E. D. de Silva, A.-S. Geiermann, M. I. Mitova, P. Kuegler, J. W. Blunt, A. L. J. Cole and M. H. G. Munro, J. Nat. Prod., 2009, 72, 477–479 CrossRef.
- For selected recent reports on ruthenium-catalyzed C–H/O–H and C–H/N–H bond functionalization, see:
(a) K. Parthasarathy, N. Senthilkumar, J. Jayakumar and C.-H. Cheng, Org. Lett., 2012, 14, 3478–3481 CrossRef CAS;
(b) V. S. Thirunavukkarasu, M. Donati and L. Ackermann, Org. Lett., 2012, 14, 3416–3419 CrossRef CAS;
(c) R. K. Chinnagolla, S. Pimparkar and M. Jeganmohan, Org. Lett., 2012, 14, 3032–3035 CrossRef CAS;
(d) L. Ackermann and A. V. Lygin, Org. Lett., 2012, 14, 764–767 CrossRef CAS;
(e) R. K. Chinnagolla and M. Jeganmohan, Chem. Commun., 2012, 2030–2032 RSC;
(f) L. Ackermann, J. Pospech, K. Graczyk and K. Rauch, Org. Lett., 2012, 14, 930–933 CrossRef CAS;
(g) L. Ackermann, L. Wang and A. V. Lygin, Chem. Sci., 2012, 3, 177–180 RSC;
(h) B. Li, H. Feng, S. Xu and B. Wang, Chem.–Eur. J., 2011, 17, 12573–12577 CrossRef CAS;
(i) L. Ackermann and S. Fenner, Org. Lett., 2011, 13, 6548–6551 CrossRef CAS;
(j) L. Ackermann, A. V. Lygin and N. Hofmann, Org. Lett., 2011, 13, 3278–3281 CrossRef CAS;
(k) L. Ackermann, A. V. Lygin and N. Hofmann, Angew. Chem., Int. Ed., 2011, 50, 6379–6382 CrossRef CAS . For recent reviews on ruthenium-catalyzed C–H bond functionalization, see: ;
(l) P. B. Arockiam, C. Bruneau and P. H. Dixneuf, Chem. Rev., 2012, 112 DOI:10.1021/cr300153j;
(m) L. Ackermann, Pure Appl. Chem., 2010, 82, 1403–1413 CrossRef CAS;
(n) L. Ackermann and R. Vicente, Top. Curr. Chem., 2010, 292, 211–229 CrossRef CAS.
- For reviews on intermolecular C–H/O–H and C–H/N–H cyclizations with alkynes catalyzed by metals other than ruthenium see:
(a) C. Zhu, R. Wang and J. R. Falck, Chem.–Asian J., 2012, 7, 1502–1514 CrossRef CAS;
(b) J. Wencel-Delord, T. Dröge, F. Liu and F. Glorius, Chem. Soc. Rev., 2011, 40, 4740–4761 RSC;
(c) T. Satoh and M. Miura, Synthesis, 2010, 3395–3409 CrossRef CAS;
(d) T. Satoh, K. Ueura and M. Miura, Pure Appl. Chem., 2008, 80, 1127–1134 CrossRef CAS . Representative communications: ;
(e) B.-J. Li, H.-Y. Wang, Q.-L. Zhu and Z.-J. Shi, Angew. Chem., Int. Ed., 2012, 51, 3948–3952 CrossRef CAS;
(f) H. Zhong, D. Yang, S. Wang and J. Huang, Chem. Commun., 2012, 3236–3238 RSC;
(g) N. Guimond, S. I. Gorelsky and K. Fagnou, J. Am. Chem. Soc., 2011, 133, 6449–6457 CrossRef CAS;
(h) T. Satoh and M. Miura, Chem.–Eur. J., 2010, 16, 11212–11222 CrossRef CAS;
(i) K. Ueura, T. Satoh and M. Miura, J. Org. Chem., 2007, 72, 5362–5367 CrossRef CAS;
(j) K. Ueura, T. Satoh and M. Miura, Org. Lett., 2007, 9, 1407–1409 CrossRef CAS.
- Reviews:
(a) C. J. Thibodeaux, W.-C. Chang and H.-W. Liu, Chem. Rev., 2012, 112, 1681–1709 CrossRef CAS;
(b) F. Brackmann and A. de Meijere, Chem. Rev., 2007, 107, 4493–4583 CrossRef CAS;
(c) L. A. Wessjohann, W. Brandt and T. Thiemann, Chem. Rev., 2003, 103, 1625–1648 CrossRef CAS;
(d) F. Gnad and O. Reiser, Chem. Rev., 2003, 103, 1603–1624 CrossRef CAS;
(e) J. Salaün, Top. Curr. Chem., 2000, 207, 1–67 CrossRef;
(f) J. Salaün and M. S. Baird, Curr. Med. Chem., 1995, 2, 511–542 Search PubMed.
-
(a) P. von R. Schleyer, J. E. Williams Jr. and K. P. Blanchard, J. Am. Chem. Soc., 1970, 92, 2377–2386 CrossRef;
(b) R. D. Bach and O. Dmitrienko, J. Am. Chem. Soc., 2004, 126, 4444–4452 CrossRef CAS , and references cited therein.
- Selected reviews on metal-promoted reactions of substituted cyclopropanes:
(a) C. Aïssa, Synthesis, 2011, 3389–3407 CrossRef;
(b) M. Rubin, M. Rubina and V. Gevorgyan, Chem. Rev., 2007, 107, 3117–3179 CrossRef CAS;
(c) I. Nakamura and Y. Yamamoto, Adv. Synth. Catal., 2002, 344, 111–129 CrossRef CAS , and references cited therein. Selected rare metal-catalyzed reactions, not resulting in an opening of at least one cyclopropane ring: ;
(d) L. Ackermann, S. I. Kozhushkov and D. S. Yufit, Chem.–Eur. J., 2012, 18, 12068–12077 CrossRef CAS;
(e) T.-L. Liu, Z.-L. He, H.-Y. Tao, Y.-P. Cai and C.-J. Wang, Chem. Commun., 2011, 2616–2618 RSC;
(f) Y. Fall, H. Doucet and M. Santelli, Tetrahedron, 2010, 66, 2181–2188 CrossRef CAS;
(g) M. Shirakura and M. Suginome, J. Am. Chem. Soc., 2009, 131, 5060–5061 CrossRef CAS;
(h) S. I. Kozhushkov, D. S. Yufit and L. Ackermann, Org. Lett., 2008, 10, 3409–3412 CrossRef CAS and references cited therein.
- Selected examples:
(a) S. I. Kozhushkov, T. Späth, M. Kosa, Y. Apeloig, D. S. Yufit and A. de Meijere, Eur. J. Org. Chem., 2003, 4234–4242 CrossRef CAS , and references cited therein; ;
(b)
G. Boche and H. M. Walborsky, Cyclopropane Derived Reactive Intermediates, ed. S. Patai and Z. Rappoport, Wiley, Chichester, 1990, pp. 117–173 Search PubMed;
(c) A. de Meijere, Angew. Chem., Int. Ed. Engl., 1979, 18, 809–826 CrossRef.
- Steric substituent constants of the n-propyl and cyclopropyl moieties are equal to 0.89 and 1.33, respectively: H. D. Beckhaus, Angew. Chem., Int. Ed. Engl., 1978, 17, 593–594 CrossRef.
- The structures of selected regioisomers have been established by X-ray crystal structure analysis: J. Langer, M. Gärtner, H. Görls and D. Walther, Synthesis, 2006, 2697–2706 CAS ; or by their properties with those of the probes, independently prepared by alternative procedures.12.
-
(a) R. Rossi, A. Carpita, F. Bellina, P. Stabile and L. Mannina, Tetrahedron, 2003, 59, 2067–2081 CrossRef CAS;
(b) J. Delaunay and J. Simonet, Tetrahedron Lett., 1988, 29, 543–544 CrossRef CAS;
(c) J. N. Chatterjea, S. K. Mukherjee, C. Bhakta, H. C. Jha and F. Zilliken, Chem. Ber., 1980, 113, 3927–3931 CrossRef CAS;
(d) H. E. Zimmerman and R. D. Simkin, Tetrahedron Lett., 1964, 1847–1851 CrossRef CAS.
- CCDC 901337 (6aa), 901338 (6ae), 901339 (7aa), 901340 (8aa) and 901341 (8ah) contain the supplementary crystallographic data for this paper.
- Only in the reaction of substituted alkyne 4b with 2g we have observed traces of a by-product with the same molecular mass as the one of product 7bg.†.
- Several representative cyclopropyl-substituted isocoumarins and isoquinolones were prepared by multistep syntheses through cyclizations of functionalized ortho-alkynylbenzoates under gold, palladium and rhodium catalysis:
(a) A. S. K. Hashmi, C. Lothschütz, R. Döpp, M. Ackermann, J. De Buck Becker, M. Rudolph, C. Scholz and F. Rominger, Adv. Synth. Catal., 2012, 354, 133–147 CrossRef CAS;
(b) A. Fürstner and P. W. Davies, J. Am. Chem. Soc., 2005, 127, 15024–15025 CrossRef;
(c) P. Zhao, D. Chen, G. Song, K. Han and X. Li, J. Org. Chem., 2012, 77, 1579–1584 CrossRef CAS.
|
This journal is © The Royal Society of Chemistry 2013 |